DOI:
10.1039/D1RA06827J
(Paper)
RSC Adv., 2021,
11, 38108-38114
Copper-catalyzed in situ oxidative-coupling for one-pot synthesis of 5-aryl-1,4-disubstituted 1,2,3-triazoles under mild conditions†
Received
11th September 2021
, Accepted 7th November 2021
First published on 25th November 2021
Abstract
A new reaction system with CuCl as catalyst, TEA as base and O2/chloramine-T as oxidant was developed for one-pot in situ oxidative-coupling to synthesize 5-aryl-1,4-disubstituted 1,2,3-triazoles in this paper. A variety of 5-arylated-1,2,3-triazole compounds could be efficiently prepared directly from the readily accessible organic azides, terminal alkynes and arylboronic acids. Advantages of the method include use of low-cost catalyst, clean oxidant, less-toxic additive, and low reaction temperature. Importantly, due to avoiding harsh strong basic reagents and high temperatures, the presented method can offer mild conditions for multi-component synthesis of 5-aryl-1,2,3-triazoles from the designed structurally complicated alkynyl or azide donors bearing natural product motifs and sensitive functional groups.
Introduction
1,2,3-Triazoles have not only demonstrated extensive anticancer, anti-inflammatory, and antibacterial activities,1,2 but also been widely used as promising potential building blocks for energetic materials, lubricants, dyes and light stabilizers in materials science.3,4 The increasing interest in the applications of 1,2,3-triazoles calls for concise and convenient synthesis methods for preparing structurally diverse 1,2,3-triazole derivatives. Copper-catalyzed cycloadditions of azide and terminal alkynes (CuAAC) reported in 2002, have given easy access to 1,4-substituted 1,2,3-triazoles.5,6 Since then, a huge number of efforts have been made for improving catalytic systems for CuAAC reaction toward high efficiency, mild conditions and application for a wide scope of substrates.7,8 In contrast, only a few of reaction systems have been reported to access to 5-aryl-1,4-disubstituted 1,2,3-triazoles.9–16 Traditionally, the reaction of aryl alkyne and azide gives 1,4- and 1,5-substituted isomers under heating conditions.7 Fokin group reported a Ru-catalysis approach for constructing 5-aryl-1,4-disubstituted 1,2,3-triazoles from the cycloaddition of diphenylacetylene and benzylazide in the presence of Cp*RuCl(PPh3)2.9 More recently, a C–H activation method for synthesis of 5-aryl-1,4-disubstituted 1,2,3-triazoles from pre-prepared 1,4-disubstituted 1,2,3-triazole and aryl halides was reported by Ackermann group in the presence of Pd catalyst and tetrabutylammonium acetate in PEG20000.11 Other reported arylation methods including uses of bromobenzene15,16 and aryl tosylate10 also showed effective for incorporations of aryl groups on C-5 of 1,2,3-triazoles. However, most of these methods are involved expensive noble metal catalysts, complex ligands, harsh reaction conditions or high temperatures.
On the other hand, a multi-component one-pot reaction strategy based on in situ capture of triazole compounds has been developed in our and other groups.17–21 By utilizing the nucleophilicity of in situ formed copper triazolides, a range of electrophilic groups such as acyl, allyl, iodine, bromine etc. could be introduced into the C-5 position of various complex 1,2,3-triazoles derivatives under mild conditions.17,18,19a Furthermore, the oxidative coupling reactions based on the copper triazolides were also found to be feasible synthetic route for incorporations of different nucleophilic groups e.g. phosphate and fluoroalkyl onto the C-5 position of 1,2,3-triazoles.19c,d Different from the reaction with electrophilic reagents, however, the oxidative coupling reaction of copper triazolides with nucleophilic reagents often suffer from undesirable efficiency and selectivity, obsessed by the self-oxidative couplings to give bis-triazoles, 1,4-diynes, 5-alkynyl triazoles and other by-products.22 To address the problems, careful optimizations of oxidants, and choice of ligands are suggested, and strong basic reagents for deprotonation were necessary in some cases before achieving desirable yields, but which inevitably leads to tedious manipulations and harsh reaction environments.
In this paper, we reported a simple copper-catalyzed O2/chloramine-T system to effectively promote the three-component reaction of organic azide, terminal alkyne and arylboronic acid for one-pot synthesis of 5-aryl-1,4-disubstituted 1,2,3-triazoles under mild conditions (Fig. 1D). In comparison with the literature methods,11,20,21 our method avoids the uses of noble metal catalysts, high reaction temperatures as well as strong basic reagents, thus permitting to apply not only for simple organic compounds, but also for complex bioactive molecules such as ribose and estrone derivatives.
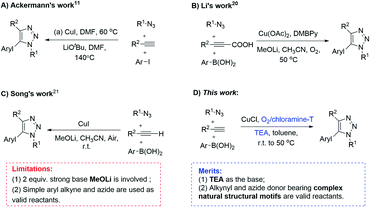 |
| Fig. 1 The reactions for multi-component synthesis of 5-aryl-1,2,3-triazoles. (A) Synthesis of 5-arly-1,2,3-triazoles from iodobenzenes, azides and alkynes developed in Ackermann's lab. (B) Synthesis of 5-arly-1,2,3-triazoles from propiolic acids, azides, and arylboronic acids developed in Li's lab. (C) Synthesis of 5-arly-1,2,3-triazoles from arylboronic acids, organic azides and alkynes developed in Song's lab. (D) Synthesis of 5-arly-1,2,3-triazoles from arylboronic acids, organic azides and alkynes developed in the current work. | |
Results and discussion
The initial attempt was carried out in the air at room temperature in the presence of CH2Cl2 with 10 mol% Cu(I) salt and 2 equivalents of Et3N (entries 1 and 2 in Table 1). Only 24% yield of 5-aryl-1,4-disubstituted 1,2,3-triazole 4a was obtained after 24 hours with CuI as the catalyst. Then, we studied the influence of Cu salt. A little higher 39% yield of 5-aryl-1,4-disubstituted 1,2,3-triazole 4a could be given when CuI was replaced by CuCl, but considerable by-products were also obtained including protonated 1,4-disubstituted triazole and phenylethynyl triazole. Different bases including DIPEA, pyridine, DIPA, DBU, Na2CO3, NaOH were next screened for the reaction, but all of them were unable to increasing the yield of target compound. Solvent effects were also investigated. The reaction in toluene demonstrated the highest yield 54% than in other solvents such as DMSO, CH3CN, dioxane, MeOH and CH3Cl. There are a large number of additives have been used for promoting oxidative reactions23 Based on the literatures, we further investigated the detailed effects of oxidant additives for the one-pot reaction to prepare 5-aryl-1,4-disubstituted 1,2,3-triazole 4a with CuCl as the catalyst, triethylamine (TEA) as the base and toluene as the solvent (entry 13 to entry 20 in Table 1). The first group of additives such as DDQ, m-CPBA caused the oxidation of Cu(I) to Cu(II), thus losing the catalytic capacity for CuAAC reaction with no any triazole-type products obtained. The second group including di-t-butyl peroxide (DTBP), NIS, NBS, Oxone and K2Cr2O7 reduced the reaction efficiency of CuAAC reaction, with 11–23% yields of 4a. Interestingly, the third group of additives, selectfluor and chloramine-T were found to promote the reaction to give 4a with 56% and 68% yield respectively. With the air/chloramine-T oxidant system, a higher 84% yield was given at 50 °C reaction temperature. Since oxygen in the air might play a role of oxidant for the one-pot reaction, we attempted to replace air atmosphere by pure oxygen. Gratefully, a desirable 90% yield of 4a was finally obtained (entry 24 in Table 1).
Table 1 Optimization of reaction conditions

|
Entrya |
Temperature |
Catalyst |
Oxidant additive |
Base |
Solvent |
Productb (%) |
0.15 mmol azide, 0.6 mmol arylboronic acid, 0.17 mmol terminal alkyne, 0.015 mmol CuX were used as substrates in the presence of 2.0 equivalent of base and 1.0 equivalent of the oxidant. Isolated yields. Oxygen environment was added. DCM (dichloromethane). DIPEA (N,N-diisopropylethylamine). DIPA (diisopropanolamine). DBU (1,8-diazabicyclo[5.4.0]undec-7-ene). DMSO (dimethyl sulfoxide). DDQ (2,3-dichloro-5,6-dicyano-1,4-benzoquinone). NBS (N-bromosuccinimide). m-CPBA (3-chloroperbenzoic acid). DTBP (di-tert-butyl peroxide). NIS (N-iodosuccinimide). |
1 |
rt |
CuI |
— |
Et3N |
DCMd |
24 |
2 |
rt |
CuCl |
— |
Et3N |
DCM |
39 |
3 |
rt |
CuCl |
— |
DIPEAd |
DCM |
27 |
4 |
rt |
CuCl |
— |
Pyridine |
DCM |
15 |
5 |
rt |
CuCl |
— |
DIPAd |
DCM |
31 |
6 |
rt |
CuCl |
— |
NaHCO3 |
DCM |
12 |
7 |
rt |
CuCl |
— |
DBUd |
DCM |
20 |
8 |
rt |
CuCl |
— |
NaOH |
DCM |
— |
9 |
rt |
CuCl |
— |
Et3N |
DMSOd |
14 |
10 |
rt |
CuCl |
— |
Et3N |
CH3CN |
11 |
11 |
rt |
CuCl |
— |
Et3N |
CHCl3 |
30 |
12 |
rt |
CuCl |
— |
Et3N |
Toluene |
32 |
13 |
rt |
CuCl |
Selectfluor |
Et3N |
Toluene |
56 |
14 |
rt |
CuCl |
DDQd |
Et3N |
Toluene |
— |
15 |
rt |
CuCl |
NBSd |
Et3N |
Toluene |
15 |
16 |
rt |
CuCl |
m-CPBAd |
Et3N |
Toluene |
— |
17 |
rt |
CuCl |
DTBPd |
Et3N |
Toluene |
11 |
18 |
rt |
CuCl |
NISd |
Et3N |
Toluene |
15 |
19 |
rt |
CuCl |
Oxone |
Et3N |
Toluene |
23 |
20 |
rt |
CuCl |
K2Cr2O7 |
Et3N |
Toluene |
20 |
21 |
rt |
CuCl |
Chloramine-T |
Et3N |
Toluene |
68 |
22 |
40 |
CuCl |
Chloramine-T |
Et3N |
Toluene |
77 |
23 |
50 |
CuCl |
Chloramine-T |
Et3N |
Toluene |
84 |
24c |
50 |
CuCl |
Chloramine-T |
Et3N |
Toluene |
90 |
With the optimized conditions in hand, we investigated the substrate scope of the one-pot reaction by using variant organic azides, terminal alkynes and arylboronic acids. Firstly, aryl alkynes with electron-withdrawing substituents (such as –F, –Cl and –Br) and electron-donating substituents (such as –CH3, –OCH3), reacted smoothly with benzyl azides 2a and arylboronic acids 3a to give target product 5-aryl-1,2,3-triazoles 4a to 4e with the yields from 80%∼90% (entry 1 to entry 5 in Table 2). Secondly, various alkyl azides (2a to 2g) bearing different substituted groups such as –NO2, –F, –OCH3, reacted smoothly with benzyl azides 2a and arylboronic acids 3a to give target product 5-aryl-1,2,3-triazoles 4l to 4t with the yields from 68%∼80% (entry 12 to entry 20 in Table 2). Among these azides, azide 2g that contained 2,5-difluorobenzyl motifs that occurs in the clinic drug rufinamide24 could react smoothly with terminal alkynes and arylboronic acids to give 2,5-difluorobenzyl 5-aryl-1,2,3-triazoles 4s and 4t, implying the potential applications of the method for drug screening. Besides of benzyl azides, naphthylmethyl azide can also effectively react with various alkynes and arylboronic acids to provide 5-aryl-1,2,3-triazoles with a yield as high as 89% (entry 6 to entry 11 in Table 2). Other azides including phenyl ether 2c, naphthyl 2d and phenylethyl azide 2f were also the effective substrates of the reaction to prepare diverse 5-aryl-1,2,3-triazoles. In addition, we tested alkyl alkyne 1g for the three-component reaction, and the target product 4x could be obtained in the yield of 64% (entry 24 in Table 2). Finally, we investigated different phenylboronic acids with electron-donating substituents (–Me) and electron-withdrawing substituents (–F and –Br) for the reaction. 5-p-Methylphenyl-1,4-disubstituted 1,2,3-triazole 4u was obtained with 87%, 5-p-fluorophenyl-1,4-disubstituted 1,2,3-triazole 4v was obtained with 67%, and 5-p-bromophenyl-1,4-disubstituted 1,2,3-triazole 4v was obtained with 64%. Therefore, the current method has shown a wide scope of substrates including variant alkynes, azides and arylboronic acids.
Table 2 Substrate scope of the reaction
Inspired by the results shown in Table 2, we then studied the potential applications of this reaction to synthesize compounds with more complex structures, by using azides and aromatic alkynes containing natural product skeleton (Scheme 1). Glucosinolates containing 1,2,3-triazole motif represent a class of broad-spectrum antibacterial agents.25,26 Here, we proved that 5-aryl-1,2,3-triazole nucleoside can be directly synthesized from glucosyl alkyne, aryl azide and phenylboronic acid in one pot, and the target compound 4y was obtained with a yield of 61%. During the reaction, the glucose motif remained intact without isomerization of glycosidic bond. Another example is the azido-containing estrone motif, which contains ketone groups sensitive to strong basic conditions and possibly suffer side-reactions in the presence of strong oxidative reagents.27,28 While in the current reaction system, it can also be used as an effective substrate to provide the target 5-aryl-1,2,3-triazole 4z in the yield of 74% and no any side-reactions related to estrone motifs were detected. In the reported reaction conditions for the preparations of 5-aryl 1,2,3-triazole (Fig. 1A to C), ether over 100 °C reaction temperatures or strong basic reagents like lithium tert-butoxide or lithium methoxide were used, which could be hardly used for acetyl protected glucoses, base-sensitive ketones and other complicated substrates bearing base-sensitive functional groups. In contrast, the method presented in this paper can be proceeded well at 50 °C with weak organic base TEA, catalytic amount of CuCl and oxygen-chloramine-T oxidant system, thus offering the mildest conditions for one-pot preparation of 5-aryl-1,4-disubstituted 1,2,3-triazoles.
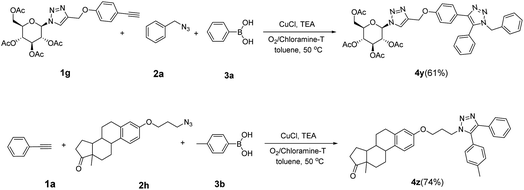 |
| Scheme 1 Applications of the one-pot reaction for syntheses of complex molecules. | |
The possible role of chloramine-T during the in situ oxidative coupling reaction was also investigated by control experiments. In replace of oxygen atmosphere with nitrogen atmosphere, only 5-H-1,2,3-triazole product could be obtained (eqn (1) in Scheme 2), which indicated the oxygen was the essential oxidant for the reaction. Increasing the amount of chloramine-T did not enhance the yield of 5-aryl-1,2,3-trazoles, suggesting chloramine-T may actually act as an additive for promoting aerobic coupling reaction. Additional control experiments were also conducted, including the reaction of 5-H-1,2,3-triazole with arylboronic acids and the reaction of diaryl alkyne with azide under the current conditions, both of which could not lead to the generation of target 5-aryl-1,2,3-trazoles (eqn (2) and (3) in Scheme 2). Trapping the reaction intermediates by nucleophilic allyl bromide demonstrated the existence of triazolide in the current reaction procedure (eqn (4) in Scheme 2). On the basis of the above experimental results and the known oxidative coupling mechanism of triazolide copper(I),3e a plausible mechanism of the in situ multi-component oxidative coupling reaction for synthesis of 5-aryl-1,2,3-triazoles was proposed as shown in Scheme 2. Briefly, the reaction proceeded through a tandem click-coupling and oxidative coupling reaction, the Cu-catalyzed click-coupling of an azide with alkyne (1a to 5′), followed by aerobic oxidative coupling of 5′ with arylboronic acids (5′ to 4a). Copper(I) acetylide intermediate 6 is formed from terminal alkyne 1a in the presence of TEA as the base, the intermediate 6 react with benzyl azide 2a via the CuAAC reaction pathway to give triazolide copper 5′. Under these oxidative conditions, transmetalation of intermediate 5′ with phenylboronic acids, generating 8 should be reasonable,20,21 and the transmetalation must take place before the protonation of 5′. However, the detailed mechanism of chloramine-T-activated aerobic oxidative coupling reaction, such as the intermediates of potential reactions of chloramine-T with O2 is still not clear at present.
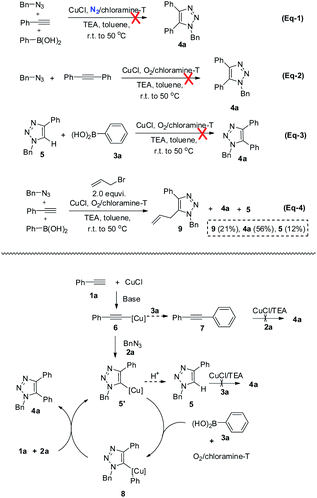 |
| Scheme 2 Control experiments and plausible reaction mechanism. | |
Conclusions
In summary, we reported a new reaction system for one-pot synthesis of 5-aryl-1,4-disubstituted 1,2,3-triazoles with low-cost CuCl as catalyst, clean oxygen as oxidant and less-toxic chloramine-T as additive in this paper. A wide scope of substituted aryl alkynes, alkynyl alkynes, benzyl and alkyne azides, substituted arylboronic acids can be used as the effective reaction substrates for the reaction to prepare structurally diverse 5-aryl-1,2,3-triazoles in modest to good yields. Importantly, the presented reaction system avoids the uses of strong basic reagents and high temperature, and thus can be used not only for simple molecules but also for the structurally complicated alkynyl or azide donors bearing natural product motifs and sensitive functional groups. Therefore, it presented the latest mild conditions for multicomponent synthesis of 5-aryl-1,2,3-triazoles. The applications of this method in designing more complex bioactive compounds are undergoing in our lab.
Data availability
Source data are provided with this paper. Methods and additional results are provided in the ESI.†
Conflicts of interest
The authors declare no conflicts of interest.
Acknowledgements
This work was funded by the National Natural Science Foundation of China (22077027, 21778015, 21801257), Central Plains Science and Technology Innovation Leader Project (214200510008), Doctoral Initiation Fund (qd18018), and Henan Provincial University Science and Technology Innovation Team (21IRTSTHN001).
Notes and references
-
(a) H. C. Kolb and K. B. Sharpless, Drug Discov. Today., 2003, 8, 1128–1137 CrossRef CAS PubMed;
(b) D. Dheer, V. Singh and R. Shankar, Bioorg. Chem., 2017, 71, 30–54 CrossRef CAS PubMed;
(c) E. Bonandi, M. S. Christodoulou, G. Fumagalli, D. Perdicchia, G. Rastelli and D. Passarella, Drug Discov. Today., 2017, 22, 1572–1581 CrossRef CAS PubMed;
(d) P. Thirumurugan, D. Matosiuk and K. Jozwiak, Chem. Rev., 2013, 113, 4905–4979 CrossRef CAS PubMed.
-
(a) D. Dheer, C. Behera, D. Singh, M. Abdullaha, G. Chashoo, S. B. Bharate, P. N. Gupta and R. Shankar, Eur. J. Med. Chem., 2020, 207, 112813 CrossRef CAS PubMed;
(b) Z. Xu, S. Zhao and Y. Liu, Eur. J. Med. Chem., 2019, 183, 111700 CrossRef CAS PubMed;
(c) F. Pagliai, T. Pirali, E. D. Grosso, R. D. Brisco, G. C. Tron, G. Sorba and A. A. Genazzani, J. Med. Chem., 2006, 49, 467–470 CrossRef CAS PubMed;
(d) N. Ahmed, N. K. Konduru, S. Ahmad and M. Owais, Eur. J. Med. Chem., 2014, 82, 552–564 CrossRef CAS PubMed;
(e) T. Ostrowski, P. Januszczyk, M. Cieslak, J. Kazmierczak-Baransk, B. Nawrot, E. Bartoszak-Adamska and J. Zeidler, Bioorg. Med. Chem., 2011, 19, 4386–4398 CrossRef CAS PubMed;
(f) D. Goyard, A. S. Chajistamatiou, A. I. Sotiropoulou, E. D. Chrysina, J.-P. Praly and S. Vidal, Chem. –Eur. J., 2014, 20, 5423–5432 CrossRef CAS PubMed.
-
(a) J. E. Moses and A. D. Moorhouse, Chem. Soc. Rev., 2007, 36, 1249–1262 RSC;
(b) F. Ahmed and H. Xiong, Dyes Pigments, 2021, 185, 108905 CrossRef CAS;
(c) S. Kantheti, R. Narayana and K. V. S. N. Raju, RSC Adv., 2015, 5, 3687–3708 RSC;
(d) L. D. Rodrigues, D. Sunil, D. Chaithra and P. Bhagavath, J. Mol. Liq., 2020, 297, 111909 CrossRef CAS;
(e) D. Fournier, R. Hoogenboom and U. S. Schubert, Chem. Soc. Rev., 2007, 36, 1369–1380 RSC.
-
(a) J. Niskanen, M. N. Tousignant, A. J. Peltekoff and B. H. Lessard, Polymer, 2021, 212, 123144 CrossRef CAS;
(b) M. Pulst, J. Balko, Y. Golitsyn, D. Reichert, K. Busse and J. Kressler, Phys. Chem. Chem. Phys., 2016, 18, 6153–6163 RSC;
(c) E. Güzel, RSC Adv., 2019, 9, 10854–10864 RSC.
- V. V. Rostovtsev, L. G. Green, V. V. Fokin and K. B. Sharpless, Angew. Chem., Int. Ed., 2002, 41, 2596–2599 CrossRef CAS PubMed.
- C. W. Tornøe, C. Christensen and M. Meldal, J. Org. Chem., 2002, 67, 3057–3064 CrossRef PubMed.
- C. W. Tornøe and M. Meldal, Chem. Rev., 2008, 108, 2952–3015 CrossRef PubMed.
-
(a) S. Neumann, M. Biewend, S. Rana and W. H. Binder, Macromol. Rapid Commun., 2019, 41, 1900359 CrossRef PubMed;
(b) M. Meldal and F. Diness, Trends in Chemistry, 2020, 2, 569–584 CrossRef CAS.
- L. Zhang, X. Chen, P. Xue, H. H. Y. Sun, I. D. Williams, K. B. Sharpless, V. V. Fokin and G. Jia, J. Am. Chem. Soc., 2005, 127, 15998–15999 CrossRef CAS PubMed.
- L. Ackermann, A. Althammer and S. Fenner, Angew. Chem., Int. Ed., 2009, 48, 201–204 CrossRef CAS PubMed.
- L. Ackermann and R. Vicente, Org. Lett., 2009, 11, 4922–4925 CrossRef CAS PubMed.
- L. Ackermann, H. K. Potukuchi, D. Landsberg and R. Vicente, Org. Lett., 2008, 39, 3081–3084 CrossRef PubMed.
- R. Chen, L. Zeng, Z. Lai and S. Cui, Adv. Syn. Catal., 2019, 361, 989–994 CrossRef CAS.
- L. Li, T. Shang, X. Ma, H. Guo, A. Zhu and G. Zhang, Synlett, 2015, 26, 695–699 CrossRef CAS.
- S. Chuprakov, N. Chernyak, A. S. Dudnik and V. Gevorgyan, Org. Lett., 2007, 9, 2333–2336 CrossRef CAS PubMed.
- F. Wei, H. Li, C. Song, Y. Ma, L. Zhou, C.-H. Tung and Z. Xu, Org. Lett., 2015, 17, 2860–2863 CrossRef CAS PubMed.
- Y.-M. Wu, J. Deng, Y. Li and Q.-Y. Chen, Synthesis, 2005, 8, 1314–1318 CrossRef.
-
(a) F. Wu, W. Zhou, K. Chen, W. Chen, M. Liu and H. Wu, Adv. Synth. Catal., 2018, 360, 2435–2439 CrossRef CAS;
(b) F. Cui, J. Chen, Z. Mo, S. Su, Y. Chen, X. Ma, H. Tang, H. Wang, Y. Pan and Y. Xu, Org. Lett., 2018, 20, 925–929 CrossRef CAS PubMed;
(c) W. Wang, F. Wei, Y. Ma, C. Tung and Z. Xu, Org. Lett., 2016, 18, 4158–4161 CrossRef CAS PubMed;
(d) X. Deng, J. Liang, B. B. Allison, C. Dvorak, H. McAllister, B. M. Savall and N. S. Mani, J. Org. Chem., 2015, 80, 11003–11012 CrossRef CAS PubMed;
(e) R. Yan, K. Sander, E. Galante, V. Rajkumar, A. Badar, M. Robson, E. El-Emir, M. F. Lythgoe, R. B. Pedley and E. Arstad, J. Am. Chem. Soc., 2013, 135, 703–709 CrossRef CAS PubMed;
(f) J. C. Morris, J. Chiche, C. Grellier, M. Lopez, L. F. Bornaghi, A. Maresca, C. T. Supuran, J. Pouyssegur and S.-A. Poulsen, J. Med. Chem., 2011, 54, 6905–6918 CrossRef CAS PubMed;
(g) W. Wang, X. Peng, F. Wei, C.-H. Tung and Z. Xu, Angew. Chem., Int. Ed., 2016, 55, 649–653 CrossRef CAS PubMed;
(h) B. Wang, N. Liu, W. Chen, D. Huang, X. Wang and Y. Hu, Adv. Synth. Catal., 2015, 357, 401–407 CrossRef CAS;
(i) Z. Zhang, Q. Zhou, F. Ye, Y. Xia, G. Wu, M. L. Hossain, Y. Zhang and J. Wang, Adv. Synth. Catal., 2015, 357, 2277–2286 CrossRef CAS;
(j) W. S. Brotherton, R. J. Clark and L. Zhu, J. Org. Chem., 2012, 77, 6443–6455 CrossRef CAS PubMed;
(k) X. Jian and Q. Song, Org. Chem. Front., 2017, 4, 938–942 RSC.
-
(a) L. Li, G. Zhang, A. Zhu and L. Zhang, J. Org. Chem., 2008, 73, 3630–3633 CrossRef CAS PubMed;
(b) L. Li, S. Ding, Y. Yang, A. Zhu, X. Fan, M. Cui, C. Chen and G. Zhang, Chem. –Eur. J., 2017, 23, 1166–1172 CrossRef CAS PubMed;
(c) L. Li, G. Hao, A. Zhu, X. Fan, G. Zhang and L. Zhang, Chem. –Eur. J., 2013, 19, 14403–14406 CrossRef CAS PubMed;
(d) A. Zhu, X. Xing, S. Wang, D. Yuan, G. Zhu, M. Geng, Y. Guo, G. Zhang and L. Li, Green Chem., 2019, 21, 3407–3412 RSC.
- X.-X. Wang, Y. Xin, Y. Li, W.-J. Xia, B. Zhou, R.-R. Ye and Y.-M. Li, J. Org. Chem., 2020, 85, 3576–3586 CrossRef CAS PubMed.
- X. Yu, J. Xu, Y. Zhou and Q. Song, Org. Chem. Front., 2018, 5, 2463–2467 RSC.
-
(a) B. Schulze and U. S. Schubert, Chem. Soc. Rev., 2014, 43, 2522–2571 RSC;
(b) J. E. Hein and V. V. Fokin, Chem. Soc. Rev., 2010, 39, 1302–1315 RSC.
-
(a) L. D. S. Yadav, V. P. Srivasta and R. Patel, Synlett, 2010, 1047–1050 CrossRef CAS;
(b) Y. Yang, J. Lan and J. You, Chem. Rev., 2017, 117, 8787–8863 CrossRef CAS PubMed.
-
(a) L. A. Sorbera, P. A. Leeson, X. Rabasseda and J. Castaner, Drugs Future, 2000, 25, 1145 CrossRef CAS;
(b) M. A. Rogawski, Epilepsy Res, 2006, 69, 273–294 CrossRef CAS PubMed;
(c) C. E. Stafstrom, Neuropsychiatr. Dis. Treat., 2009, 5, 547–551 CrossRef CAS PubMed.
-
(a) J. C. Morris, J. Chiche, C. Grellier, M. Lopez, L. F. Bornaghi, A. Maresca, C. T. Supuran, J. Pouyssegur and S.-A. Poulsen, J. Med. Chem., 2011, 54, 6905–6918 CrossRef CAS PubMed;
(b) J.-B. He, H.-F. He, L. Zhao, L. Zhang, G.-Y. You, L.-L. Feng, J. Wan and H.-W. He, Bioorg. Med. Chem., 2015, 23, 1395–1401 CrossRef CAS PubMed.
-
(a) K. T. Andrews, G. M. Fisher, S. D. M. Sumanadasa, T. Skinner-Adams, J. Moeker, M. Lopez and S.-A. Poulsen, Bioorg. Med. Chem. Lett., 2013, 23, 6114–6117 CrossRef CAS PubMed;
(b) N. Ahmed, N. K. Konduru, S. Ahmad and M. Owais, Eur. J. Med. Chem., 2014, 82, 552–564 CrossRef CAS PubMed;
(c) V. R. Sirivolu, S. K. V. Vernekar, T. Ilina, N. S. Myshakina, M. A. Parniak and Z. Q. Wang, J. Med. Chem., 2013, 56, 8765–8780 CrossRef CAS PubMed.
-
(a) B. Ildikó, K. Csilla, O. A. Boglárka, K. Gábor, R. Réka, Ö.-L. Csilla and M. Erzsébet, Molecules, 2018, 23, 821 CrossRef PubMed;
(b) A. Gupta, B. S. Kumar and A. S. Negi, J. Steroid
Biochem. Mol. Biol., 2013, 137, 242–270 CrossRef CAS PubMed.
-
(a) P. C. Meltzer, D. Butler, J. R. Deschamps and B. K. Madras, J. Med. Chem., 2006, 49, 1325–1345 CrossRef PubMed;
(b) J. R. Hanson, Nat. Prod. Rep., 2007, 24, 1342–1349 RSC.
Footnote |
† Electronic supplementary information (ESI) available. See DOI: 10.1039/d1ra06827j |
|
This journal is © The Royal Society of Chemistry 2021 |
Click here to see how this site uses Cookies. View our privacy policy here.