DOI:
10.1039/D1RA06873C
(Paper)
RSC Adv., 2021,
11, 38054-38059
Synthesis, characterization, and functional evaluation of branched dodecyl phenol polyoxyethylene ethers: a novel class of surfactants with excellent wetting properties†
Received
14th September 2021
, Accepted 2nd November 2021
First published on 25th November 2021
Abstract
A series of branched dodecyl phenol polyoxyethylene ethers (b-DPEOn) were successfully synthesized via alkylation and ethylene oxide addition reactions. The alkylation reaction was conducted by using a branched internal olefin as the raw material. Furthermore, the conversion rate of the branched dodecene was measured to be 98.1% and the selectivity towards branched dodecyl phenol (b-DP) was 95.9%. Moreover, b-DPEOn (b-DPEO7, b-DPEO10, b-DPEO12) were obtained via the reaction of ethylene oxide with b-DP. Notably, b-DPEO10 can efficiently reduce the surface tension of water below 31.55 mN m−1 at the critical micelle concentration (cmc) and the cmc value in water was approximately 1.3 × 10−2 g L−1 at 25 °C. The preferable wetting ability of b-DPEO10 was superior to that of commercialized dodecyl phenol polyoxyethylene ether (c-DPEOn), so it will be promoted and used in the textile and pesticide industries.
Introduction
Surface tension is caused by the imbalance of the forces on the molecules at the interface of the two phases. Surfactant molecules usually feature a hydrophilic group at one end and a lipophilic group at the other, which can form an arrangement on the surface of the solution to improve the unbalanced state of the surface molecules, thereby reducing the surface tension of the solution.1–4 Non-ionic surfactants represent an important subgroup of surfactants, whose hydrophobic groups are generally composed of alkylphenols, fatty alcohols, etc. On the other hand, their hydrophilic groups consist of polyoxyethylene (EO) or polyoxypropylene (PO) chains.5–8 The properties of non-ionic surfactants can be adjusted by changing the lengths of hydrophobic or hydrophilic groups and by modifying the structures of the hydrophobic moiety.9,10
At present, commercialized alkylphenol polyoxyethylene ethers are obtained from alpha olefins (primarily the linear ones), while only a few branched alkylphenol polyoxyethylene ethers have been reported. However, studies have revealed that the hydrophobic base with a branched structure show better wetting and penetrating properties than those of linear ones, even when their types and molecular sizes are comparable.11–15 Sha and coworkers successfully synthesized a novel branched fluorinated anionic surfactant 4-(3,3,4,4,5,5,5-heptafluoro-2,2-bis-trifluoromethyl-pentyl)-benzene lithium phosphonate via a four-step route by using perfluoro-2-methyl-2-pentene as a starting material.16 This branched fluorinated anionic surfactant exhibits excellent surface activity, which reduces the surface tension of water to be 19.2 mN m−1. Zhang et al. successfully obtained novel anionic surfactants alcohol polyoxyethylene ether carboxylates with three branched chains.17 One of these novel branched surfactants features allows for the reduction of surface tension of water to around 27 mN m−1. Compared with linear surfactants, branched surfactants exhibit preferable wetting ability toward potential application, which was mainly caused by the difference in diffusion rate.18–20
Although high-branched alkyl tails may lead to poor biodegradability, branched surfactants with limited short side-chains have been testified to have negligible effect on their biodegradability.21 With respect to the linear isomer, a branched alkyl amine oxide surfactant (C10DAO-branched) showed the ability to form concentrated solutions without generating lyotropic liquid crystals22 or to tune supramolecular aggregation upon pH stimuli.23 Moreover, C10DAO-branched can keep low viscosity even in mixtures with other linear surfactants, thereby the rational design of branched alkyl structures has become an efficient strategy to increase the concentration of surfactants in detergent formulations.24
In this research, we present a series of branched dodecyl phenol polyoxyethylene ether (b-DPEOn), which were obtained through alkylation and ethylene oxide addition reaction in high yields, as shown in Scheme 1. It is noteworthy that the raw material non-terminal dodecene is a by-product of the butene oligomerization unit and contains some branched olefins. Their ability to reduce surface tension and wetting performance were investigated and compared with the commercialized dodecyl phenol polyoxyethylene ether (c-DPEOn).
 |
| Scheme 1 The synthesis process of the b-DPEOn. | |
Experimental
Materials
Branched non-terminal dodecenes were obtained from Sinopec Maoming Petrochemical Company. The composition analysis of the dodecenes was provided by Sinopec Maoming Petrochemical Company and analyzed by GC-MS and NMR instruments. The composition is as follows: the content of low-carbon chain components such as octylphenol and octane is less than 0.5%, the content of dodecene is more than 98%, and the content of dodecane and high-carbon olefins is less than 1%. The approximate content of terminal dodecenes was ca. 10%. The catalyst (sulfonic acid resin, DA330) was purchased from Dandong Mingzhu Special Resin Co., Ltd. Ethylene oxide was industrial pure grade. Phenol, methanol, KOH, and lactic acid were all analytical pure grade. The c-DPEOn used for comparison was purchased from Jiangsu Hai'an Petrochemical Company.
Synthesis of branched dodecyl phenol (b-DP)
The specified molar ratio of phenol and branched non-terminal dodecene was added in a 250 mL flask. Added the catalyst DA330 to the flask and fixed the stirring device. When the reaction device was prepared, the raw materials were heated to melt through the oil bath, and then stirred. The reaction time was started being counted when the temperature reached the specified temperature. The resulting products were analyzed for the composition by GC.
Conversion rate: X = 1 − m |
where m was the remaining content of dodecene in the product, n was the content of the b-DP.
Synthesis of branched dodecyl phenol polyoxyethylene ether (b-DPEOn)
The b-DP and 0.06% wt KOH were added to a 0.3 L pressure reactor. N2 was used to replace the air in the reactor for three times. For the first hour, water removal was proceeded from the reactor under the condition of reduced pressure at 100 ± 5 °C. Then the temperature was increased to about 160 °C, and EO was introduced from the bottom of the reactor. The reaction temperature was set at 175 ± 5 °C and the pressure at 0.3–0.4 MPa until all EO had been added. Finally, after cooling to ca. 70 °C, the unreacted EO was removed, and the products were obtained by adding lactic acid to neutralize. (mKOH
:
m85% lactic acid = 1
:
1.89).
Test conditions of GC
Test instrument. Shimadzu GC-2010AF.Agilent J&W GC column: DB-1MS, 30 m × 0.25 mm × 0.25 μm.
Setting parameters. The initial temperature of the chromatographic column was 60 °C, maintained for 2 minutes and then increased to 280 °C at a rate of 20 °C min−1, and then maintained for 15 minutes. The temperature of the inlet was 250 °C, and the temperature of the FID detector was 280 °C. N2: 30 mL min−1, air: 400 mL min−1, H2: 40 mL min−1.
Test of surface tension
Test instrument. K100 automatic surface tension instrument from KRUSS, Germany.
Test conditions.
Platinum plate method. The concentration of the starting and the final solution was 500 and 1 mg L−1, respectively, with the starting volume of 60 mL. A total of 12 points had been tested upon the addition of deionized water.A series of parameters such as surface tension reduction efficiency (pc20), efficiency (Πcmc), saturated adsorption capacity (Γmax) and saturated adsorption area (Amin) can be obtained by the following formulas:
|
pc20 = −lg c20
| (2) |
|
 | (3) |
|
 | (4) |
where
γ0 was the surface tension of pure water at 25 °C, approximately 72.0 mN m
−1,
c20 was the mass concentration of surfactant (g L
−1) when
γ0 was less than 20 mN m
−1.
R was the gas constant with the value of 8.314 J (mol K)
−1, and
NA was Avogadro's constant.
Test of hydroxyl value
The hydroxyl value was tested by titration. In this method, acetic anhydride and pyridine were selected as acylation reagents. The hydroxyl value I(OH) (mg KOH per g) was calculated according to the following formula:
where V0 was the volume of KOH solution consumed in the blank experiment, V1 was the volume of KOH solution consumed by the sample, c was the concentration of KOH solution, and m0 was the mass of the sample.
Test of wetting ability
The coarse canvas is used in the test, and the specification is C/C 21/2 × 8/2. The canvas is cut into round cloth pieces with a diameter of 30 mm, with a mass range of 0.44 to 0.45 g. The 0.5 g L−1 solution of surfactant in water is prepared, and the temperature is kept at 25 °C after dissolution. The round cloth pieces were put into the solution horizontally. The time from entering the solution to the beginning of sinking was denoted as the wetting time, and three parallel tests were carried out for each surfactant.
Test of polyethylene glycol content
Test instrument. Agilent Technologies 1260 Infinity LC.
Results and discussion
Synthesis of b-DP
Excess phenol was typically added in the alkylation reactions with the presence of olefin and phenol as raw materials to reduce the content of multi-substituted products and increase the yield of mono-substituted ones.25 In this paper, the conversion rate of the reaction was calculated based on the remaining content of dodecene in the products, showing that the main by-products in the reaction were multi-substituted alkylphenol. The alkylation reaction also yielded other organics including decyl phenol and cetyl phenol. However, their contents were less than 0.5% wt and therefore they were not discussed in this study. As shown in Fig. 1, lowering the reaction temperature, increasing the molar ratio of phenol to olefin, and reducing the reaction time exclusively resulted in the reduction of the selectivity of multi-substituted products. As a consequence, conversion rate of olefins decreased and the difficulty of the separation and purification increased. To maintain the high conversion rate of olefins and high selectivity of mono-substituted products, the reaction condition was optimized. The amount of catalyst added was 5%, the molar ratio of phenol to olefin was 4
:
1, the reaction temperature was 90 °C, and the reaction time was 5 h. Under this condition, the conversion rate of branched non-terminal dodecene and the selectivity of b-DP was determined to be 98.1% and 95.9%, respectively.
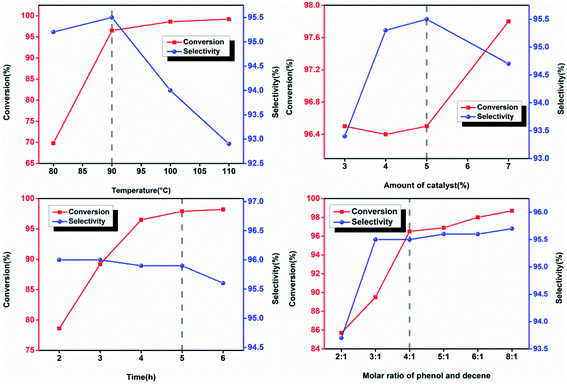 |
| Fig. 1 Effects on the synthesis of b-DP. | |
Synthesis of b-DPEOn.
Characterization of b-DPEOn. After separating and purifying, b-DPs further reacted with ethylene oxide to yield three kinds of b-DPEOn (b-DPEO7, b-DPEO10, and b-DPEO12). The structures were analyzed by FTIR and 1H NMR, and the results were shown in Fig. 2 and S1,† respectively.
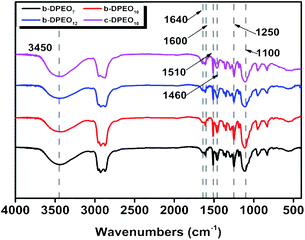 |
| Fig. 2 FTIR spectra. | |
The main characteristic absorption peaks of the DPEOn can be found in the FTIR spectra. The peak of 3450 cm−1 corresponds to the stretching vibration of the O–H group in the phenolic hydroxyl group, and the peaks of 1640, 1600, 1510, and 1460 cm−1 can be assigned to the stretching vibration of the benzene ring. The anti-symmetric stretching vibration peak of C–O–C group and the symmetric stretching vibration peak locate at 1250 cm−1 and 1100 cm−1, respectively. Combined with the analysis of the 1H NMR, it can be seen that b-DPEO10 and c-DPEO10 have similar chemical shifts regions of phenyl H, methyl H and methylene H, which further confirm their structural relevance. However, the branched chain in b-DPEO10 leads to more complicated chemical environments for the H on the hydrocarbon chain, as exemplified by the larger integration in the range of 0–2 ppm due to the presence of more methyl H in the branched one.
Properties of b-DPEOn. The hydroxyl value can be used to define the average molecular weight of the product. Furthermore, the contents of polyethylene glycol may affect the surface activity of the product. These two parameters are summarized in Table 1.
Table 1 Hydroxyl values and polyethylene glycol contents
Sample |
Hydroxyl value/mg KOH g−1 |
Polyethylene glycol content/% wt |
Theoretical hydroxyl value/mg KOH g−1 |
b-DPEO7 |
96.07 |
1.81 |
98.37 |
b-DPEO10 |
80.26 |
2.13 |
79.89 |
b-DPEO12 |
73.17 |
2.76 |
70.93 |
c-DPEO10 |
85.79 |
2.60 |
79.89 |
It can be seen from Table 1 that all the hydroxyl values of the synthesized samples are close to the theoretical values, while the hydroxyl value of b-DPEO12 is higher than the calculated value. This result combined with the polyethylene glycol content suggests that the hydroxyl value of b-DPEO12 is higher because the reaction time was longer and more polyethylene glycol was formed. The hydroxyl value of c-DPEO10 is higher than the theoretical value, which is mainly caused by the naming convention in the industry. According to the hydroxyl value calculation, its actual degree of polymerization should be around 9. The molecular weights of the b-DPEOn were in a reasonable range based on the theoretical hydroxyl values, which allows for the measurement of surface tension and test of performance.
Wetting ability of b-DPEOn. As depicted in Fig. 4, the wetting ability of b-DPEOn was tested under three different mass concentrations, showing that the performance of b-DPEOn are better than c-DPEO10. Specifically, b-DPEOn shows good wetting ability at the concentration of 0.1 g L−1 and the its performance can be optimized at a concentration of 0.5 g L−1. The pc20 of b-DPEOn is higher, implying that b-DPEOn has a higher efficiency of reducing surface tension. At the same time, the sizes of b-DPEOn in water are smaller than that of the linear surfactant c-DPEO10. Branched surfactant can penetrate to the surface of the internal fibers more quickly to complete the directional arrangement, which give rise to a better wetting ability than the linear one. In contrast, a recent work showed that the cmc of a branched amine-oxide was higher than that of the linear analogue.23 It appears that the effect of branches depends on the final architecture of the tail, which regulates the critical packing parameter of the surfactants bearing short side chains.
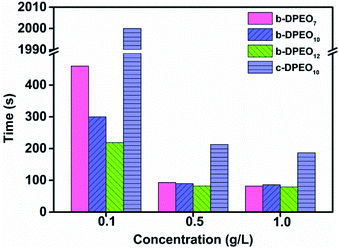 |
| Fig. 4 Wetting ability of b-DPEOn. | |
Conclusions
The b-DP was obtained through the reaction of branched non-terminal dodecenes and phenol. The optimal reaction condition was as follows: the amount of catalyst added is 5%, the molar ratio of phenol to branched dodecene is 4
:
1, the reaction temperature is 90 °C, and the reaction time is 5 h. Under this condition, the conversion rate of branched non-terminal dodecene is 98.1%, and the selectivity of b-DP is 95.9%. A series novel surfactants b-DPEOn were synthesized by the ethylene oxide addition reaction of b-DP. The characterizations of FTIR and 1H NMR confirmed that the structures of b-DPEOn and the commercialized linear sample are similar. It was found that the b-DPEO10 exhibits excellent surface activity to reduce the surface tension of water below to 31.55 mN m−1 at the cmc and the cmc value in water is about 1.3 × 10−2 g L−1 at 25 °C. Compared with c-DPEOn, b-DPEOn exhibited preferable wetting ability. This work can provide a feasible solution for the application of internal olefins, by-products of the olefin oligomerization unit.
Author contributions
Xing Wang: data curation, writing-original draft, formal analysis. Junfeng Qian: conceptualization, methodology, validation. Zhonghua Sun: investigation, methodology. Zhihui Zhang: writing-review & editing. Mingyang He: project administration, conceptualization, funding acquisition.
Conflicts of interest
The authors declare no conflict of interest.
Acknowledgements
We gratefully acknowledge the financial support from A Project funded by the Priority Academic Program Development of Jiangsu Higher Education Institutions (PAPD) and Jiangsu Key Laboratory of Advanced Catalytic Materials and Technology (grant BM2012110).
Notes and references
- J. W. Hu, X. Y. Zhang and Z. W. Wang, Int. J. Mol. Sci., 2010, 11, 1020–1047 CrossRef CAS PubMed.
- M. A. Migahed and A. M. Al-Sabagh, Chem. Eng. Commun., 2009, 196, 1054–1075 CrossRef CAS.
- Saroj and L. Sharma, Mini-Rev. Org. Chem., 2018, 15, 404–411 CrossRef CAS.
- L. C. Wang, X. S. Wang, J. C. Zhang, X. W. Song, X. L. Cao and Z. Q. Li, J. Dispersion Sci. Technol., 2014, 35, 641–646 CrossRef CAS.
- B. Simončič and M. Kert, Dyes Pigm., 2008, 76, 104–112 CrossRef.
- Z. W. Wang, G. Z. Li, J. H. Mu, X. Y. Zhang and A. J. Lou, Chin. Chem. Lett., 2002, 13, 363–366 CAS.
- W. J. Zhang, L. G. Zhou and Z. Y. Ding, J. Dispersion Sci. Technol., 2009, 30, 1161–1166 CrossRef CAS.
- M. A. Abd El-Ghaffar, M. H. Sherif and A. Taher El-Habab, J. Surfactants Deterg., 2016, 20, 117–128 CrossRef.
- I. D. Robb and P. S. Stevenson, Langmuir, 2000, 16, 7939–7945 CrossRef CAS.
- V. Mirchi, S. Saraji, M. Akbarabadi, L. Goual and M. Piri, Ind. Eng. Chem. Res., 2017, 56, 13677–13695 CrossRef CAS.
- S. W. Wang and R. E. Marchant, Macromolecules, 2004, 37, 3353–3359 CrossRef CAS PubMed.
- N. A. Jamaluddin, A. Mohamed, S. Abu Bakar, T. Ardyani, M. Sagisaka, S. Suhara, M. Hafiz Mamat, M. K. Ahmad, S. M. King, S. E. Rogers and J. Eastoe, Phys. Chem. Chem. Phys., 2020, 22, 12732–12744 RSC.
- Z. Y. Liu, N. Wei, C. Wang, H. Zhou, L. Zhang, Q. Liao and L. Zhang, AIP Adv., 2015, 5, 1–8 CAS.
- P. C. Bian, D. P. Zhang, H. Z. Gang, J. F. Liu, B. Z. Mu and S. Z. Yang, Acta Phys. - Chim. Sin., 2016, 32, 2753–2760 CAS.
- H. K. Wu, J. Q. Zhong, H. M. Shen and H. X. Shi, J. Fluorine Chem., 2013, 156, 5–8 CrossRef CAS.
- M. Sha, R. Pan, L. Zhan, P. Xing and B. Jiang, Chin. J. Chem., 2014, 32, 995–998 CrossRef CAS.
- Q. H. Zhang, Y. L. Li, Y. B. Song, J. Li and Z. F. Wang, Mini-Rev. Org. Chem., 2018, 258, 34–39 CAS.
- B. Simončič and V. Rozman, Colloids Surf., A, 2007, 292, 236–245 CrossRef.
- Q. Jiang, Y. Du, L. Zhang, W. Ma, F. Yan, L. Zhang and S. Zhao, Molecules, 2021, 26, 863 CrossRef CAS PubMed.
- A. Fabozzi, R. Vitiello, I. Russo Krauss, M. Iuliano, G. De Tommaso, A. Amoresano, G. Pinto, L. Paduano, C. Jones, M. Di Serio and G. D'Errico, J. Surfactants Deterg., 2019, 22, 115–124 CrossRef CAS.
- A. Marcomini, G. Pojana, C. Carrer, L. Cavalli, G. Cassani and M. Lazzarin, Environ. Toxicol. Chem., 2000, 19, 555–560 CrossRef CAS.
- A. Fabozzi, I. Russo Krauss, R. Vitiello, M. Fornasier, L. Sicignano, S. King, S. Guido, C. Jones, L. Paduano, S. Murgia and G. D'Errico, J. Colloid Interface Sci., 2019, 552, 448–463 CrossRef CAS PubMed.
- L. Scermino, A. Fabozzi, G. De Tommaso, A. J. M. Valente, M. Iuliano, L. Paduano and G. D'Errico, J. Mol. Liq., 2020, 316 Search PubMed.
- L. Savignano, A. Fabozzi, R. Vitiello, M. Fornasier, S. Murgia, S. Guido, V. Guida, L. Paduano and G. D'Errico, Colloids Surf., A, 2021, 613, 126091 CrossRef CAS.
- S. Sarish, B. Devassy, W. Bohringer, J. Fletcher and S. Halligudi, J. Mol. Catal. A: Chem., 2005, 240, 123–131 CAS.
Footnote |
† Electronic supplementary information (ESI) available: 1H NMR are included. See DOI: 10.1039/d1ra06873c |
|
This journal is © The Royal Society of Chemistry 2021 |
Click here to see how this site uses Cookies. View our privacy policy here.