DOI:
10.1039/D1RA07413J
(Paper)
RSC Adv., 2021,
11, 36222-36229
Formation and enhancement of negative thermal quenching in emission of KGdF4:Eu3+, Yb3+@GQDs†
Received
6th October 2021
, Accepted 2nd November 2021
First published on 9th November 2021
Abstract
In order to obtain a red emitting phosphor with good luminescence thermal stability, a series of KGdF4:Eu3+, Yb3+@GQD (GQD: Cl-containing graphene quantum dots) red emitting phosphors have been synthesized by the co-precipitation method, and their luminescence thermal properties have also been studied in detail. It is intriguing that the negative thermal quenching (NTQ) effect is induced by the double doping of Yb3+, and the effect is further enhanced by GQD coating. The strongest integrated PL intensities of the optimal double doped sample and the optimal GQD-coated sample are at 130 and 170 °C, in which the corresponding integrated PL intensities are presented as 117.7 and 156.5% of the initial value at 30 °C, respectively. The NTQ effect makes the optimal GQD coated sample have good luminescent thermal stability, so it can be applied for high-power WLEDs. A mechanism of energy conversion from heat to light is discussed and suggested for the effect.
1. Introduction
White light emitting diodes (WLEDs) have gradually become the mainstream lighting source due to their characteristics of high efficiency, low energy consumption, low heat dissipation, long life, good light quality, energy saving and environmental protection.1–3 The traditional method to obtain white light is to coat the yellow emitting phosphor on the blue LED chip, and realize the white light emission by mixture of the blue light of the chip and the yellow light of the phosphor. But there are some serious problems in this system, such as thermal quenching, poor color reproducibility, high color temperature (CCT > 5000 K), and low color rendering index (CRI < 80) due to the lack of red emitting components, which have a great impact on practical application.4–6 Another method to obtain white light is to coat three primary color (blue, yellow and red color) phosphors on near-ultraviolet (near-UV) light LED chip (370–410 nm) and achieve white light emission by mixture of the three primary color lights. The phosphors are the focus of current research on luminescent materials. However, the red emitting phosphors obtained in this way are mainly Y2O2S:Eu3+. Their luminescent efficiency is far lower than that of blue and green phosphors and their chemical properties are unstable. It decomposes easily at WLEDs working temperatures to release toxic hydrogen sulfide and reduce the service life of WLEDs.7 Therefore, it is urgent to develop a red emitting phosphor with high luminescent thermal stability, which is suitable for WLEDs excited by near-UV light (370–410 nm).
Eu3+ ion is generally recognized as a good activator for red phosphor. Under near-UV (370–410 nm) excitation, 5D0 → 7Fj (j = 0, 1, 2, 3, 4) transitions emit red light, so that many researchers are trying to develop Eu3+ as activator to develop red emitting phosphor. But because of the shielding effect of 5s and 5p electrons in the outer shell, Eu3+ has weak luminescent intensity and small absorption cross section. So it is necessary to find an appropriate sensitizer to improve the luminescence intensity.8–34 Eu3+ and Yb3+ often co-doped in various kinds of matrix, for example: Lu2O3,13 CaF2,14 Gd2O3,15 KGdF3 (ref. 16) and NaYF4,17 etc., and emission of Eu3+ is enhanced by absorbing the infrared light though Yb3+. But, as best as we know, that under near-UV (370–410 nm) excitation, the emission enhancement of Eu3+ by co-doping of Yb3+ has been rarely reported.
In addition to luminescent intensity, thermal quenching of phosphors is also an important factor affecting the luminescent performance, and the Eu3+ doped red emitting phosphors are no exception. The luminescent intensity of Eu3+ will decrease with the increase of temperature in most matrices, such as Ba3Bi(PO4)3:Eu3+,18 Sr2Al3O6F:Eu3+,19 K4CaSi3O9:Eu3+,20 Ca2LaTaO6:Eu3+,21 Ba3ZrNb4O15:Eu3+.22 There are only a few reports to show that emission of Eu3+ can produce NTQ with the increase of temperature. For example, emission intensity of K5Y(P2O7):Eu3+ (ref. 23) increases with the increase of temperature before 100 °C, and emission intensity of Ca18Li3Bi0.4Eu0.6 (PO4)14:Eu3+ (ref. 24) at 60 °C is 230.4% of that initial value at 30 °C. Therefore, more studies are needed to improve the thermal stability of the Eu3+ doped phosphors.
In our previous work,33 it has been reported that KGdF4:Eu3+:Sm3+ phosphor was induced to have the NTQ effect by coating with GQDs. It's implied that after Eu3+ and different rare earth ions co-doped phosphors are coated with GQDs, they may also have the NTQ effect. Rare earth fluoride of KGdF4 is considered to be a good matrix due to its high ionicity, low phonon energy, good optical stability and high refractive index. So, it is widely used as matrix for various down-conversion and up-conversion materials.27–30
In addition, the ionic radius difference between Gd3+ and Eu3+ or Yb3+ is within 15%. Based on the Vegard Law,7 the metal ion radius does not exceed 15%, they can easily replace each other, which facilitates co-doping of Eu3+ and Yb3+. Therefore, KGdF4 is a good matrix for doping of Eu3+ ion.
So, as a part of our system research, in this paper, based on KGdF4:Eu3+, Yb3+ obtained via a co-precipitation method, GQDs is coated on the surface of KGdF4:Eu3+, Yb3+ to form KGdF4:Eu3+, Yb3+@GQDs hybrid composite luminescent materials. Some novel effects induced by co-doping of Yb3+ and coating of GQDs have been observed, which the NTQ effect was formed after co-doping of Yb3+ and it was further obviously enhanced by coating of GQDs.
2. Experimental procedure and methods
Experimental section is described in ESI.† Experimental section includes reagents, apparatus, and synthesis of the samples. Properties of GQDs are also described in (Table 1S, and Fig. 1S–6S†).
3. Results and discussion
3.1 Structure, morphology and composition
The ICP elemental analysis data of samples (i–iii) are listed in Table 1, which the corresponding molecular formulae of the samples (i–iii) are KGF:0.20Eu3+, KGF:0.20Eu3+, 0.002Yb3+, KGF:0.20Eu3+, 0.002Yb3+@GQDs2 mg mol−1, respectively.
Table 1 ICP element analysis results of samples (i–iii)a
No. |
K/% |
Gd/% |
Eu/% |
Yb/% |
Atomic ratios of K : Gd : Eu : Yb |
Calculated molecular formulae |
(i) KGF:0.20Eu3+, (ii) KGF:0.20Eu3+, 0.002Yb3+, (iii) KGF:0.20Eu3+, 0.002Yb3+@GQDs2 mg mol−1. |
(i) |
14.327 |
46.455 |
11.237 |
0.000 |
0.996 : 0.803 : 0.201 : 0.0000 |
KGF:0.20Eu3+ |
(ii) |
14.311 |
46.172 |
11.068 |
0.146 |
0.995 : 0.798 : 0.198 : 0.0023 |
KGF:0.20Eu3+, 0.002Yb3+ |
(iii) |
14.354 |
46.149 |
10.957 |
0.134 |
0.998 : 0.798 : 0.196 : 0.0021 |
KGF:0.20Eu3+, 0.002Yb3+@GQDs2 mg mol−1 |
Fig. 1 shows the XRD patterns of the samples (i–iii) ((i) KGF:0.20Eu3+, (ii) KGF:0.20Eu3+, 0.002Yb3+, (iii) KGF: 0.20Eu3+, 0.002Yb3+@GQDs2 mg mol−1) and the standard spectra of NaGdF4. Indexed results of Fig. 1 are listed in Table 2, which the crystal structures of all the three samples are identical to be close to that of cubic NaGdF4 (space group Fm
m (225), PDF # 27-0697), which are supported by other authors' reports.34–38 It can be seen from Fig. 1 and Table 2 that: first, doping of Eu3+, Yb3+ and coating of GQDs do not change the structure of the samples. Second, the crystallinity of the samples is relatively high, and there is no any other impurity peaks. Finally, the crystal structures of the samples are similar to the standard pattern of cubic NaGdF4(PDF # 27-0697), but the position of the all peaks are shifted to a small angle, due to the ionic radius of K+ being larger than that of Na+.28,35–38
 |
| Fig. 1 XRD patterns of samples, (i) KGF:0.20Eu3+; (ii) KGF:0.20Eu3+, 0.002Yb3+; (iii) KGF:0.20Eu3+, 0.002Yb3+@GQDs2 mg mol−1. | |
Table 2 Lattice parameters and volumes of samples (i–iii)a
Sample |
a = b = c/Å |
Vol./Å3 |
(i) KGF:0.20Eu3+, (ii) KGF:0.20Eu3+, 0.002Yb3+, (iii) KGF:0.20Eu3+, 0.002Yb3+@GQDs2 mg mol−1. |
(i) |
5.748 |
189.93 |
(ii) |
5.740 |
189.18 |
(iii) |
5.751 |
190.23 |
PDF # 027-0697 |
5.518 |
168.01 |
In order to confirm the phase of the samples (i–iii), we not only synthesized NaGdF4:0.20Eu3+ by the same method (Fig. 7S†), but also compared with the XRD patterns of KGdF4:Ln3+ (Ln = Eu, Ce, Sm, Tb) reported by other authors.34–38 Fig. 7S† shows that XRD pattern of the as-synthesized NaGdF4:0.20Eu3+ is in agreement with those of hexagonal NaGdF4 (PDF # 27-0699) and hexagonal NaEuF4 (PDF # 49-1897), but it does not match to that of cubic NaGdF4 (PDF # 27-0697).
Some authors illustrate that the XRD patterns of KGdF4: Ln3+ (Ln = Eu, Ce, Sm, Tb)34–38 are close to those of hexagonal YGdF4 (PDF # 27-0466), cubic NaYF4 (PDF # 06-0342), cubic NaGdF4 (PDF # 27-0697), but they are not similar to that of orthorhombic KGdF4 (PDF # 33-1007). It is interesting that the XRD patterns of the samples (i–iii) are the same as those of KGdF4: Ln3+ (Ln = Eu, Ce, Sm, Tb),34–38 indicating that they are phase of KGdF4.
Fig. 2 shows the SEM image, EDS, XPS and FTIR spectra of sample (iii), KGF: 0.20Eu3+, 0.002Yb3+@GQDs2 mg mol−1. Fig. 2a (SEM) shows that the sample is cluster of irregular particles with a size of about 5–10 μm, and many small particles are adsorbed on the cluster surface. Fig. 2b (EDS) shows that the sample contains elements of K, Gd, Eu, Yb, F and C, indicating that GQDs has been successfully coated on surface of the sample. XPS results (Fig. 2c) show that the sample is composed with K, Ti, Mn, F, C and O, which C and O come from GQDs, adsorbed CO2 and H2O, respectively. FTIR spectra (Fig. 2d) show that sample (iii) and GQDs have the same characteristic FTIR absorption peak (Fig. 6S†). Here, the characteristic sharp peak at 1639 and 3450 cm−1 can be attributed to C
C vibration of GQDs, and O–H vibration of H2O,39 confirming that surface of the sample has GQDs. In conclusion, results of EDS, FTIR and XPS all illustrate that GQDs has been coated on the surface of the sample (iii).
 |
| Fig. 2 SEM image, EDS, XPS and FTIR spectra of sample (iii), KGF:0.20Eu3+, 0.002Yb3+@GQDs2 mg mol−1: (a) SEM, (b) EDS, (c) XPS, (d) FTIR. | |
3.2 Luminescence properties
Fig. 3 shows luminescent properties of the three samples at room temperature. As shown in Fig. 3a, the strongest excitation peaks of the samples are at about 393 nm. Co-doping of Yb3+ and coating of GQDs significantly enhances and widens the peaks, and the intensity order of the peaks is (iii) > (ii) > (i). Fig. 3b shows PL spectra of the samples, which the emission intensities are also significantly enhanced by Yb3+ co-doping and GQDs coating, but the position of the main emission peaks has not changed: (a) emission peak intensity of the sample (ii) is 1.40 times that of the sample (i); (b) emission peak intensity of sample (iii) is approximately 1.20 times that of the sample (ii) and approximately 1.68 times that of the sample (i). (c) It can be seen from Fig. 4Sb,† GQDs should have an emission peak at about 460 nm under excitation of 393 nm. However, in Fig. 3b, no emission peak of GQDs appears at about 460 nm, indicating that GQDs transfers energy to Eu3+ after absorbing excitation light. Decay curves of the samples (i) and (iii) are shown in Fig. 3c, which can be fitted with linear functions (here, the nonlinear equation of
is transformed into the linear equation of Ln(y − y0) = A1 − x/τ). Lifetimes of the samples (i–iii) obtained from the curves are 5.22, 5.90 and 6.28 ms, respectively. Fig. 3d shows chromaticity diagrams of the samples (i–iii), and corresponding CIE chromaticity coordinates are as follows: (i) (0.6156, 0.3839); (ii) (0.6150, 0.3845); (iii) (0.6147, 0.3848). Fig. 3d further shows that the three points have overlapped as one point, indicating the emissions of the samples have almost the same red color.
 |
| Fig. 3 Luminescent properties of three samples, (i) KGF:0.20Eu3+; (ii) KGF:0.20Eu3+, 0.002Yb3+; (iii) KGF:0.20Eu3+, 0.002Yb3+@GQDs2 mg mol−1: (a) PLE spectra, (b) PL spectra, (c) decay curves, (d) CIE chromaticity diagrams. | |
3.3 Luminescent properties at different concentrations of Eu3+, Yb3+ and GQDs
Fig. 4a shows PL spectra of KGF:xEu3+. All KGF:xEu3+ samples show similar PL spectra with different relative intensities. Three strong peaks observed at 591, 611, 650 and 700 nm are attributed to the 5D0 → 7F1, 5D0 → 7F2, 5D0 → 7F3 and 5D0 → 7F4 transitions of Eu3+ ion, respectively.9,11 In addition, the weak peaks at 533, 552, 578, and 625 nm (shoulder peak) belong to the 5D1–7F1, 5D1–7F2, 5D1–7F3 and 5D1–7F4, respectively.9 Curve of the emission intensity at 592 nm changes with different x is a parabola, achieves its summit when x is about 0.20.
 |
| Fig. 4 Emission spectra of three samples: (a) PL spectra of KGF:xEu3+; (b) PL spectra of KGF:0.20Eu3+,yYb3+; (c) PL spectra of KGF:0.20Eu3+,0.002Yb3+@GQDsz(z=0–4). | |
Fig. 4b shows the emission spectra (PL) of KGF:0.20Eu3+, yYb3+ samples. The dependent curve of emission intensity at 592 nm on different y is also a parabola, and it has a maximum when x is about 0.002.
Based on the result of Fig. 4b, the optimal double-doped sample (KGF:0.20Eu3+, 0.002Yb3+) was further coated with GQDs. The emission spectra (PL) of KGF:0.20Eu3+,0.002Yb3+@GQDsz samples are shown in Fig. 4c. Fig. 4c shows that the PL intensity curve with different z is a parabola with a maximum value. The optimal concentration of GDQs for KGF:0.20Eu3+,0.002Yb3+@GDQsz phosphors is 2 mg mol−1. The results show that the obvious luminescent enhancement can be obtained by coating a small amount of GQDs.
3.4 Luminescent thermal stabilities
Luminescent thermal properties of the samples (i–iii) at different temperatures are shown in Fig. 5. Fig. 5a–c shows the integrated PL intensity curves of the three samples are strongly affected by temperature. Fig. 5d shows that the curve of the sample (i) is a nonlinear curve with monotonically decreasing, and curves of the sample (ii–iii) are parabolas, which is caused by the NTQ effect.36,37 Fig. 5d further shows that curve (iii) is higher than curve (ii), indicates that the effect of the sample (iii) is stronger than that of the sample (ii). The highest points of the two parabola curves (curves (ii) and (iii)) are 130, 170 °C, which the corresponding integrated PL intensities are 117.7 and 156.5% of that initial value at 30 °C, respectively. So, the optimal sample (KGF:0.20Eu3+, 0.002Yb3+@GQDs2 mg mol−1) has high luminescent thermal stability, due to the NTQ effect.
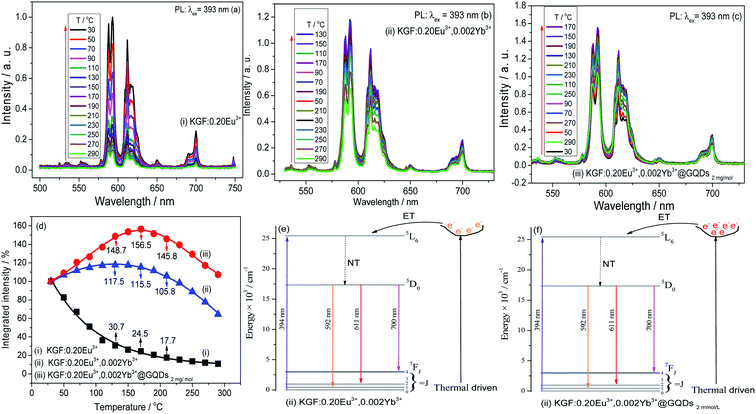 |
| Fig. 5 Luminescent properties of three samples at different temperatures, (i) KGF:0.20Eu3+; (ii) KGF:0.20Eu3+, 0.002Yb3+; (iii) KGF: 0.20Eu3+, 0.002Yb3+@GQDs2 mg mol−1: (a–c) PL spectra, (d) integrated intensity curves, (e and f) mechanism of negative thermal quenching. | |
The results of Fig. 5d show that: (a) the sample (i) has no such the effect, but the sample (ii) has, indicating that the effect is induced by co-doping of Yb3+. The effect induced by double doped ion was also observed in LuVO4:Bi3+, Eu3+ phosphor reported by Zhang et al.;38 (b) the effect is further obviously enhanced when the surface of the double doped sample is coated with GQDs. The enhancement of the effect by GQDs was also occurred in GQDs coated Mn4+ doped fluoride red emitting phosphor.40
In previous work,33 we have reported that KGdF4:Eu3+, Sm3+@GQDs also has the NTQ effect, but their co-doping sample (KGdF4:Eu3+,Sm3+) has not. The results show that Eu3+ and different rare earth ions co-doped on the same matrix (KGdF4) have different luminescent thermal properties, and only proper co-doping can obtain samples with the NTQ effect.
Mechanism of the effect has been discussed in some reports,24,41–45 and was suggested that the electrons of phosphor gain energy supply from the electron trap produced by the defect of the matrix with the increase of temperature, and then the effect is induced.
In this paper, the mechanism of the effect is further explored from the perspective of energy conservation. The intensity of the excited light is constant whether at the parabola starting point or at its apex (Fig. 5d). However, the integrated PL intensity at the apex is stronger than that at the starting point. According to the mechanism reported in the literature, the extra energy required at the apex is provided by the electrons in the electron trap. From a macro point of view, in the whole variable temperature test system, the only way to provide extra energy is the thermal energy of the heating system. Therefore, it is not difficult to find that the energy in the electron trap is converted from the thermal energy. This mechanism can be represented with Fig. 5e and f. Fig. 5e and f show that: first, after double-doping of Yb3+ (Fig. 5e), the defects suitable for forming electron traps are formed in the matrix, and then the electrons are driven by heat and trapped by the defects, and finally the electrons transfer energy to the 5L6 energy level of Eu3+ to resulting in the NTQ effect. In addition, when the double-doped sample is coated with GQDs (Fig. 5f), more defects are produced and more electrons are captured, so more energy is transferred to the 5L6 energy level, which induces a stronger the NTQ effect. The mechanism of Fig. 5e and f is suggested as mechanism of energy conversion from heat to light.
3.5 Luminescent performances of prototype red light LEDs
The luminescent performances of the prototype red light LEDs are shown in Fig. 6. The LEDs were fabricated by coating mixture of the samples (i, iii) KGF:0.20Eu3+, 0.002Yb3+@GQDs2 mg mol−1 and epoxy onto a 395 nm UV chip and tested at different driving currents. Fig. 6a shows the electroluminescence spectra of the LEDs, which consist emissions of UV chip and the samples. Fig. 6b depicts CIE chromaticity diagrams of the LEDs, which are (0.5373, 0.3494) and (0.5163, 0.3392), respectively. Results of Fig. 6b indicate that the LEDs emit orange red light, which is supported by orange main emission (about 592 nm) in Fig. 3b.
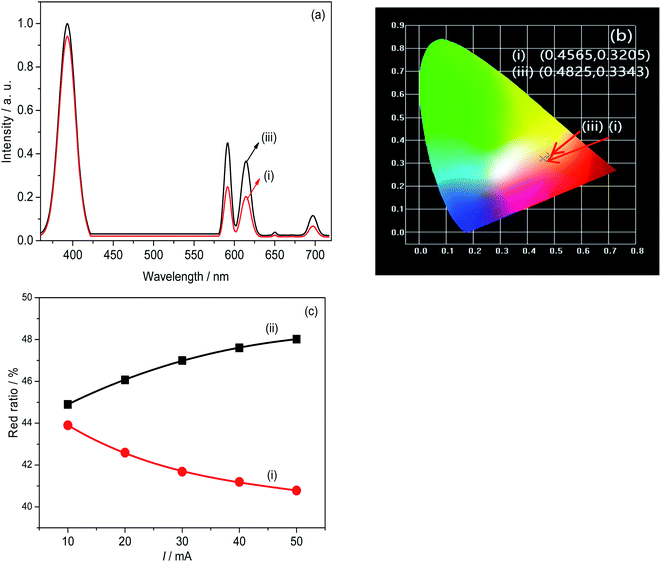 |
| Fig. 6 Luminescent performances of prototype LEDs (samples (i, iii) + 395 nm UV chip), (i) KGF:0.20Eu3+, (iii) KGF:0.20Eu3+, 0.002Yb3+@GQDs2 mg mol−1: (a) electroluminescence spectrum under a 20 mA drive current, (b) CIE chromaticity diagram, (c) red ratio under a different drive current. | |
The red color ratio of the LEDs at different driving currents is shown in Fig. 6c. Fig. 6c illustrates that the trend of the two curves is just the opposite. Curve (iii) increases with the increase of the driving current, while curve (i) decreases with the increase of the driving current. It is well known that the operating temperature of the chip increases with the increase of the driving current of the chip. It can be seen from the illustration in Fig. 5d, before 170 °C, curve (iii) monotonically rises with the increase of temperature, while curve (i) monotonically decreases with the increase of temperature. Obviously, the results of Fig. 6c are supported by results of Fig. 5d, and it can be further inferred that the operating temperature of the UV chip driven by 50 mA current does not exceed 170 °C. Furthermore, the results of Fig. 6c also indicate that the optimal sample (KGF: 0.20Eu3+, 0.002Yb3+@GQDs2 mg mol−1) has high luminescent thermal stability, which can be applied for high power WLEDs.
4. Conclusions
In summary, a series of KGdF4:Eu3+,Yb3+@GQDs red emitting phosphor have been synthesized by co-precipitation method, and their luminescent thermal properties have also been studied. More intriguingly, the NTQ effect is formed induced by the double doping of Yb3+, and the effect is further enhanced by GQDS coating. The strongest integrated PL intensities of the optimal double doped sample and the optimal GQDs coated sample are at 130 and 170 °C, which the corresponding integrated PL intensities are 117.7 and 156.5% of that initial value at 30 °C, respectively. The NTQ effect makes the optimal GQDs coated sample have good luminescent thermal stability. A mechanism of energy conversion from heat to light is suggested for the NTQ effect.
Conflicts of interest
The authors declare that they have no conflict of interest.
Author contributions
Zhigao Wu: methodology, formal analysis, investigation, writing – original draft. Chang Chen: investigation. Yaxiong Wang: investigation. Chaolian Luo: investigation. Sen Liao: conceptualization, supervision. Yingheng Huang: review & editing, visualization. Junyu Ming: review & editing, visualization.
Acknowledgements
This research is supported by the National Natural Science Foundation of China (Grant No. 21661006 and 21965004), the Natural Science Foundation of Guangxi Zhuang Autonomous Region, China (Grant No. 2019GXNSFDA245022), the Scientific Research Foundation of Guangxi University (Grant No. XDZ140116), the Innovation Project of Guangxi Graduate Education (Grant No. YCSW2020015), the Students Experimental Skills and Innovation Ability Training Fund Project of Guangxi University (No. 202010593186).
References
- P. Du, X. Y. Huang and J. S. Yu, Facile synthesis of bifunctional Eu3+-activated NaBiF4 red-emitting nanoparticles for simultaneous white light-emitting diodes and field emission displays, Chem. Eng. J., 2017, 337, 91–100 CrossRef.
- S. S. Liang, M. M. Shang, H. Z. Liang, K. Li, Y. Zhang and J. Lin, Deep red MGe4O9:Mn4+ (M = Sr, Ba) phosphors: structure, luminescence properties and application in warm white light emitting diodes, J. Mater. Chem. C, 2016, 426, 6409–6416 RSC.
- A. J. Huang, Z. W. Yang, C. Y. Yu, Z. Z. Chai, J. B. Qiu and Z. G. Song, Tunable and white light emission of a single-phased Ba2Y(BO3)2Cl: Bi3+, Eu3+ phosphor by energy transfer for ultraviolet converted white LEDs, J. Phys. Chem. C, 2017, 121, 5267–5276 CrossRef CAS.
- J. H. Zheng, S. Q. Wu, G. Chen, S. J. Dang, Y. X. Zhuang, Z. Q. Guo, Y. J. Lu, Q. J. Cheng and C. Chen, Blue-emitting Ca5(PO4)3Cl:Eu2+ phosphor for near-UV pumped light emitting diodes: Electronic structures, luminescence properties and LED fabrications, J. Alloys Compd., 2016, 663, 332–339 CrossRef CAS.
- G. Annadurai and S. Masilla Moses Kennedy, Synthesis and photoluminescence properties of Ba2CaZn2Si6O17:Eu3+ red phosphors for white LED applications, J. Lumin., 2016, 169, 690–694 CrossRef CAS.
- H. Guo, X. Y. Huang and Y. J. Zeng, Synthesis and photoluminescence properties of novel highly thermal-stable red-emitting Na3Sc2(PO4)3:Eu3+ phosphors for UV-excited white-light-emitting diodes, J. Alloys Compd., 2018, 741, 300–306 CrossRef CAS.
- Z. Jia, X. L. Zhang, X. Y. Hua, Y. Dong, H. L. Li, C. Q. Feng, Y. G. Wang and M. J. Xia, Engineering mixed polyanion red-emitting Rb2Bi(PO4)(WO4):Eu3+ phosphors with negligible thermal quenching and high quantum yield, J. Alloys Compd., 2020, 844, 155875 CrossRef CAS.
- K. Li and R. V. Deun, Eu3+/Sm3+-doped Na2BiMg2(VO4)3 from substitution of Ca2+ by Na+and Bi3+ in Ca2NaMg2(VO4)3: Color-tunable luminescence via efficient energy transfer from (VO4)3- to Eu3+/Sm3+ ions, Dyes Pigm., 2018, 155, 258–264 CrossRef CAS.
- M. E. Alvarez-Ramosa, R. C. Carrillo-Torresa, R. Sánchez-Zeferinoa, U. Caldiñob and J. Alvarado-Rivera, Co-emission and energy transfer of Sm3+ and/or Eu3+ activated zincgermanate-tellurite glass as a potential tunable orange to reddish-orange phosphor, J. Non-Cryst. Solids, 2019, 521, 119462 CrossRef.
- Z. X. Fu and B. R. Liu, Hydrothermal synthesis, energy transfer and luminescence enhancement of rhombohedral LaOF: Sm3+-Eu3+ nanoparticles, Phys. B, 2019, 574, 311653 CrossRef CAS.
- X. G. Zhang, J. L. Zhang, Y. B. Chen and M. L. Gong, Energy transfer and multicolor tunable emission in single-phase Tb3+, Eu3+ co-doped Sr3La(PO4)3 phosphors, Ceram. Int., 2016, 42, 13919–13924 CrossRef CAS.
- B. Li, X. Huang, H. Guo and Y. Zeng, Energy transfer and tunable photoluminescence of LaBWO6:Tb3+, Eu3+ phosphors for near-UV white LEDs, Dyes Pigm., 2018, 150, 67–72 CrossRef CAS.
- G. T. Xiang, Y. Ma and W. Liu, Enhancement of Eu3+ red upconversion in Lu2O3 : Yb3+/Eu3+ powders under the assistance of bridging function originated from Ho3+ tridoping, Inorg. Chem., 2017, 56, 13955–13961 CrossRef CAS PubMed.
- N. Rakova, S. C. Duartea and G. S. Maciel, Evaluation of the energy transfer mechanism leading to tunable green-to-red cooperative up-conversion emission in Eu3+-Yb3+ co-doped CaF2 powders, J. Lumin., 2019, 214, 116561 CrossRef.
- S. K. Ranjan, A. K. Soni and V. K. Rai, Frequency upconversion and fluorescence intensity ratio method in Yb3+-ion-sensitized Gd2O3:Er3+-Eu3+ phosphors for display and temperature sensing, Methods Appl. Fluoresc., 2017, 5, 035004 CrossRef PubMed.
- X. X. Han, E. H. Song, W. B. Chen, Y. Y. Zhou and Q. Y. Zhang, Color-tunable upconversion luminescence and prolonged Eu3+ fluorescence lifetime in fluoride KCdF3: Yb3+,Mn2+,Eu3+ via controllable and efficient energy transfer, J. Mater. Chem. C, 2020, 8, 9836–9844 RSC.
- T. K. Pathak, A. Kumar, L. J. B. Erasmus, A. Pandey, E. Coetsee, H. C. Swart and R. E. Kroon, Highly efficient infrared to visible up-conversion emission tuning from red to white in Eu/Yb co-doped NaYF4 phosphor, Spectrochim. Acta, Part A, 2019, 207, 23–30 CrossRef CAS PubMed.
- H. F. Guo, Q. F. Shi, K. V. Ivanovskikh, L. Wang, C. Cui and P. Huang, A high color purity red-emission phosphor based on Sm3+ and Eu3+ co-doped Ba3Bi(PO4)3, Mater. Res. Bull., 2020, 126, 110836 CrossRef CAS.
- P. Ranjith, S. Sreealsa, J. Tyagi, K. Jayanthi, G. Jagannath, P. Patra, S. Ahmad, K. Annapurna, A. R. Allu and S. Das, Elucidating the structure and optimising the photoluminescence properties of Sr2Al3O6F: Eu3+ oxyfluorides for cool white-LEDs, J. Alloys Compd., 2020, 826, 154015 CrossRef CAS.
- L. Zhao, F. Y. Fan and X. Chen, Luminescence and thermal-quenching properties of silicate-based red-emitting K4CaSi3O9:Eu3+ phosphor, J. Mater. Sci.: Mater. Electron., 2018, 29, 5975–5981 CrossRef CAS.
- Z. J Zhang, L. L. Sun and B. Devakumar, Synthesis and photoluminescence properties of a new blue-light-excitable red phosphor Ca2LaTaO6:Eu3+ for white LEDs, J. Lumin., 2020, 222, 117173 CrossRef.
- S. Y. Xin, F. G. Zhou and L. Zhao, Synthesis, Luminescence Property and Thermal Quenching Investigation of Niobate Phosphors Ba3ZrNb4O15:Eu3+under Multiple Excitations, ECS J. Solid State Sci. Technol., 2018, 7, R94–R98 CrossRef CAS.
- D. Zhao, Y. L. Xue and S. R. Zhang, Non-concentration quenching, good thermal stability and high quantum efficiency of K5Y(P2O7)2:Eu3+/Tb3+ phosphors with a novel two-dimensional layer structure, J. Mater. Chem. C, 2019, 7, 14264–14274 RSC.
- G. Zhu, Z. W. Li, X. J. Wang, F. G. Zhuo, M. Gao, S. Y. Xin and Y. H. Wang, Highly Eu3+ ions doped novel red emission solid solution phosphors Ca18Li3(Bi, Eu) (PO4)14: structure design, characteristic luminescence and abnormal thermal quenching behavior investigation, Dalton Trans., 2019, 48, 1624–1632 RSC.
- W. J. Zhang, Y. Wang and X. X. Zhang, The effect of graphene oxide concentration on luminescence property of Tb3+-complexes, J. Inorg. Organomet. Polym. Mater., 2018, 28, 2596–2602 CrossRef CAS.
- Y. M. Liu, Y. L. Li and Y. T. Feng, Effects of graphene quantum dots coating on the luminescence properties of K2SiF6:Mn4+ red-emitting phosphors, J. Mater. Sci.: Mater. Electron., 2020, 31, 444–456 CrossRef CAS.
- A. Prasad, A. S. Rao and G. V. Prakash, A study on up-conversion and energy transfer kinetics of KGdF4:Yb3+/Er3+ nanophosphors, J. Mol. Struct., 2020, 1205, 127647 CrossRef CAS.
- A. Prasad, A. S. Rao, M. Gupta and G. V. Prakash, Morphological and luminescence studies on KGdF4:Yb3+/Tb3+ up-conversion nanophosphors, Mater. Chem. Phys., 2018, 219, 13–21 CrossRef CAS.
- J. P. Ma, C. Yi and C. Li, Facile synthesis and functionalization of color-tunable Ln3+-doped KGdF4 nanoparticles on a microfluidic platform, Mater. Sci. Eng., 2020, 108, 110381 CrossRef CAS PubMed.
- A. Prasad, A. S. Rao and G. V. Prakash, Up-conversion luminescence and EPR properties of KGdF4:Yb3+/Tm3+ nanophosphors, Optik, 2020, 208, 164538 CrossRef CAS.
- Y. Y. Bu and X. H. Yan, Temperature dependent photoluminescence of Eu3+-doped Ca7V4O17, J. Lumin., 2017, 190, 50–55 CrossRef CAS.
- S. D. Li, Q. Y. Meng and S. C. Lü, Study on optical temperature sensing properties of Tb3+, Eu3+ co-doped CaMoO4 phosphor, J. Lumin., 2018, 200, 103–110 CrossRef CAS.
- J.
Y. Ming, Y. X. Wang, S. K. Ling, S. Liao, Y. H. Huang and H. X. Zhang, Conversion of thermal energy to light energy and energy transfer in KGdF4: Eu3+,Tb3+ phosphors, Inorg. Chem. Commun., 2021, 127, 108549 CrossRef CAS.
- N. Duan, H. Zhang, Y. Nie, S. J. Wu, T. T. Miao, J. Chen and Z. P. Wang, Fluorescence resonance energy transfer-based aptamer biosensors for bisphenol a using lanthanide-doped KGdF4 nanoparticle, Anal. Methods, 2015, 7, 5186–5192 RSC.
- P. V. Do, V. X. Quang, L. D. Thanh, V. P. Tuyen, N. X. Ca, V. X. Hoa and H. V. Tuyen, Energy transfer and white light emission of KGdF4 polycrystalline co-doped with Tb3+/Sm3+ ions, Opt. Mater., 2019, 92, 174–180 CrossRef.
- C. Y. Cao, H. M. Noh, B. K. Moon, B. C. Choi and J. H. Jeong, Synthesis and optical properties of Ce3+/Tb3+ doped LaF3, KGdF4, and KYF4 nanocrystals, J. Lumin., 2014, 152, 206–209 CrossRef CAS.
- D. M. Yang, G. G. Li, X. J. Kang, Z. Y. Cheng, P. A. Ma, C. Peng, H. Z. Lian, C. X. Li and J. Lin, Room temperature synthesis of hydrophilic Ln3+-doped KGdF4 (Ln = Ce, Eu, Tb, Dy) nanoparticles with controllable size: energy transfer, size-dependent and color-tunable luminescence properties, Nanoscale, 2012, 4, 3450–3459 RSC.
- C. Y. Cao, H. K. Yang, J. W. Chung, B. K. Moon, B. C. Choi, J. H. Jeong and K. H. Kim, Hydrothermal synthesis and enhanced photoluminescence of Tb3+ in Ce3+/Tb3+ doped KGdF4 nanocrystals, J. Mater. Chem., 2011, 21, 10342–10347 RSC.
- Y. C. Zhao, L. J. Huang, Y. X. Wang, J. G. Tang, Y. Wang, J. X. Liu, L. A. Belfiore and M. J. Kipper, Synthesis of graphene oxide/rare-earth complex hybrid luminescent materials via π–π stacking and their pH-dependent luminescence, J. Alloys Compd., 2016, 687, 95–103 CrossRef CAS.
- E. H. Song, J. Q. Wang, S. Ye, X. F. Jiang, M. Y. Peng and Q. Y. Zhang, Room-temperature synthesis and warm-white LED applications of Mn4+ ion doped fluoroaluminate red phosphor Na3AlF6: Mn4+, J. Mater. Chem. C, 2016, 4, 2480–2487 RSC.
- E. H. Song, J. Q. Wang, J. H. Shi, T. T. Deng, S. Ye, M. Y. Peng, J. Wang, L. Wondraczek and Q. Y. Zhang, Highly efficient and thermally stable K3Al6:Mn4+ as a red phosphor for ultra-high-performance warm white light-emitting diodes, ACS Appl. Mater. Interfaces, 2017, 9, 8805–8812 CrossRef CAS PubMed.
- Z. G. Zhang, Z. P. Zhu, Z. Y. Guo, F. W. Mo and Z. C. Wu, A zero-thermal-quenching and color-tunable phosphor LuVO4: Bi3+, Eu3+ for NUV LEDs, Dyes Pigm., 2018, 156, 67–73 CrossRef.
- Y. L. Li, X. Zhong, Y. Yu, Y. M. Liu, S. Liao, Y. H. Huang and H. X. Zhang, H2O2-free preparation of K2SiF6:Mn4+ and remarkable high luminescent thermal stability induced by coating with graphene quantum dots, Mater. Chem. Phys., 2021, 260, 124149 CrossRef CAS.
- S. Q. Fang, T. C. Lang, T. Hao, J. Y. Wang, J. Y. Yang, S. X. Cao, L. L. Ping, B. T. Liu, A. N. Yakovlev and V. I. Korepanov, Zero-thermal-quenching of Mn4+ far-red-emitting in LaAlO3 perovskite phosphor via energy compensation of electrons' traps, Chem. Eng. J., 2020, 389, 124297 CrossRef CAS.
- X. C. Wang, Z. Y. Zhao, Q. S. Wu, C. Wang, Q. Wang, Y. Y. Li and Y. H. Wang, Structure, photoluminescence and abnormal thermal quenching behavior of Eu2+-doped Na3Sc2(PO4)3: a novel blue-emitting phosphor for n-UV LEDs, J. Mater. Chem. C, 2016, 4, 8795–8801 RSC.
Footnote |
† Electronic supplementary information (ESI) available. See DOI: 10.1039/d1ra07413j |
|
This journal is © The Royal Society of Chemistry 2021 |
Click here to see how this site uses Cookies. View our privacy policy here.