DOI:
10.1039/D0SC06661C
(Edge Article)
Chem. Sci., 2021,
12, 3726-3732
Facile synthesis of axially chiral styrene-type carboxylic acids via palladium-catalyzed asymmetric C–H activation†
Received
7th December 2020
, Accepted 19th January 2021
First published on 20th January 2021
Abstract
A novel method by a one-step introduction of axial chirality and sterically hindered group has been developed for facile synthesis of axially chiral styrene-type carboxylic acids. With the palladium-catalyzed C–H arylation and olefination of readily available cinnamic acid established, this transformation demonstrated excellent yield, excellent stereocontrol (up to 99% yield and 99% ee), and broad substrate scope under mild conditions. The axially chiral styrene-type carboxylic acids produced have been successfully applied to Cp*CoIII-catalyzed asymmetric C–H activation reactions, indicating their potential as chiral ligands or catalysts in asymmetric synthesis.
Introduction
Enantiopure carboxylic acids, especially natural amino acids, have been widely used as readily available chiral substances for facile syntheses of complex chiral compounds, known as the strategy of ‘chiral pool synthesis’.1 Meanwhile, chiral carboxylic acids serve not only as an important class of organocatalysts for various enantioselective transformations,2 but also as chiral ligands for transition-metal-catalyzed asymmetric chemical processes ranging from academic to industrial settings.3,4 With the enormous progress made in the past several decades in enantioselective C–H functionalization,5 chiral carboxylic acids (CCAs) have recently emerged as powerful ligands for this step- and atom-economic transformation. For instance, chiral amino-acid or cyclopropane-derived dirhodium tetracarboxylates enabled a plethora of asymmetric intra- and inter-molecular C–H functionalizations via a stereo-controlled insertion of Rh-carbene into C–H bonds.3 Although Cp*MIII (M = Co, Rh, Ir) has recently been developed as an efficient catalyst for C–H functionalization,6 the lack of extra coordination sites for extrinsic chiral ligands presented distinct difficulties for catalytic stereo-control of such transformations.7 To address this issue, CCAs have been used as chiral ligands to enable enantioselective C–H activation without the use of a synthetically difficult chiral Cp*MIII catalyst.4,7 Apart from central4b–d,f,k–n and planar CCAs,4g highly sterically demanding axial CCAs have been designed and used for efficient recognition of the prochiral C–H bonds. While a sterically hindered aryl group adjacent to the carboxyl group is required to control the enantioselectivity of C–H activation, a multiple-step synthesis to modify the binaphthyl backbone remains still a tedious task and definitely hampers their application in organic synthesis.4e,h–j
Although various synthetic methods have been well established for the synthesis of such biaryl atropisomers,8 their “diphenyl analogs”, axially chiral styrenes with the chiral axis between a substituted alkene and an aromatic ring, have long been neglected9 despite their potential in total synthesis and asymmetric synthesis as chiral catalysts or ligands.10,11a,b Besides the “central to axial chirality transfer” strategy which required the use of stoichiometric chiral intermediates,11 transition-metal or organo-catalyzed asymmetric reactions have recently been developed as efficient approaches for the construction of axially chiral styrenes.12–15 To mimic the rigidity of biaryls, alkene skeletons with the C
C double bond trapped within six-membered-rings have been used to synthesize chiral arylcyclohexenes.12 Meanwhile, a structure with the chiral axis between an open-chain alkene and an aromatic ring, which exhibited lower rotation energy, was first constructed by Tan13 and Yan14 by incorporation of sterically hindered groups into the alkynes using an organocatalytic addition strategy. Very recently, Pd-catalyzed asymmetric olefination of C–H bonds has been developed by Shi and co-workers to make axially chiral styrenes, although this protocol required the use of strongly coordinating pyridyl or other transient directing groups (TDGs).15 As part of our continuous efforts in enantioselective C–H functionalizations,16 we conceived a method in which the readily available racemic cinnamic acid derivative could be used as an efficient precursor to make axially chiral styrene-type ligands via asymmetric C–H functionalization, which thus provides an efficient and rapid way to furnish novel axially chiral acid ligands by a simple modification of racemic acids (Scheme 1).
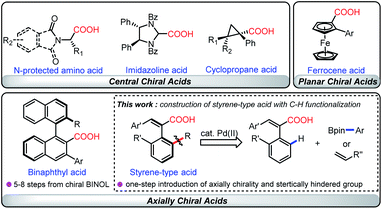 |
| Scheme 1 Chiral carboxylic acids as ligands for asymmetric C–H functionalization. | |
Herein, we describe a novel strategy for the facile synthesis of axially chiral styrene-type carboxylic acids by modification of cinnamic acid derivatives via palladium(II)-catalyzed C–H arylation or olefination.17 The carboxyl group, which serves as a key motif in the resulting chiral ligands, plays an important role as a weakly coordinating group in the one-step introduction of axial chirality and sterically hindered group in this catalytic method.18 This new transformation demonstrated excellent stereocontrol, broad scope under mild conditions, and the produced axially chiral styrene-type carboxylic acids have been successfully applied to Cp*CoIII-catalyzed asymmetric C–H activation reactions.
Results and discussion
Our study commenced with the investigation of the Pd(II)-catalyzed C–H arylation of the cinnamic acid derivative rac-1a with 4-(methoxycarbonyl)benzeneboronic acid pinacol ester 2a used as the coupling partner. After extensive examination of the reaction conditions, the desired product 3aa was obtained in 74% yield and 97% ee in the presence of 10 mol% of Pd(OAc)2, 1.5 equiv. of Ag2CO3, 0.5 equiv. of BQ, 2.0 equiv. of KHCO3, 0.2 equiv. of Boc-L-tert-leucine, and 20.0 equiv. of H2O in tAmylOH at 40 °C for 72 hours in an air atmosphere (Table 1, entry 1). A series of control experiments were then conducted, which indicated that no arylation product was detected in the absence of the palladium catalyst or base (entries 2 and 3). Meanwhile, removing the N-protecting group from Boc-L-tert-leucine completely quenched the reaction (entry 4). Furthermore, screening of oxidants showed that the combination of Ag2CO3 and BQ was crucial for this transformation, and the replacement of Ag2CO3 with Ag2O or omitting the addition of BQ resulted in a sharp decrease of yields (entries 5 and 6). Interestingly, H2O played a key role in improving both yield and enantioselectivity in this reaction system (entry 7). A careful examination of bases and solvents clearly showed that KHCO3 and tAmylOH were the optimal choices for this asymmetric C–H arylation (see the ESI† for details), while the use of KH2PO4 as the base gave 3aa in only 49% yield (entry 8) and the employment of iPrOH as the solvent decreased the yield dramatically to 34%, albeit still with excellent enantioselectivity (entry 9). Meanwhile, the replacement of Boc-L-tert-leucine with other N-protected amino acids (MPAAs) as ligands furnished only lower enantioselectivity to some extent. Not surprisingly, raising the reaction temperature to 60 °C led to a better yield but poor enantioselectivity (entry 11).
Table 1 Optimization of reaction conditionsa

|
Entry |
Change from standard conditions |
Yieldb (%) |
Eec (%) |
Standard conditions: rac-1a (0.2 mmol), 2a (2.0 equiv.), Pd(OAc)2 (0.1 equiv.), Boc-L-tert-leucine (0.2 equiv.), BQ (0.5 equiv.), Ag2CO3 (1.5 equiv.), KHCO3 (2.0 equiv.), H2O (20.0 equiv.) in tAmylOH 1.0 mL in air at 40 °C for 72 h, the crude mixture was methylated using MeI.
Isolated yields.
The ee value was determined by HPLC.
|
1 |
None |
74 |
97 |
2 |
No Pd(OAc)2 |
0 |
— |
3 |
No KHCO3 |
0 |
— |
4 |
tert-Leucine instead of Boc-L-tert-leucine |
0 |
— |
5 |
Ag2O instead of Ag2CO3 |
51 |
97 |
6 |
No BQ |
51 |
90 |
7 |
No H2O |
41 |
67 |
8 |
KH2PO4 instead of KHCO3 |
49 |
94 |
9 |
iPrOH as the solvent |
34 |
96 |
10 |
Fmoc-L-tert-leucine instead of Boc-L-tert-leucine |
60 |
92 |
11 |
Raising the temperature to 60 °C |
86 |
70 |
With the optimized conditions in hand, the scope and functional group tolerance of the aryl–aryl coupling were next investigated (Table 2). Firstly, the examination of various ortho-substituted phenylacetic acids indicated that sterically bulky substituents were required for high enantioselective control, and larger alkyl groups (3ba–3da) gave excellent enantioselectivities (94–98% ee) due to the higher rotational barriers. While the steric hindrance apparently affected the enantioselectivities, they had no influence on the reactivity, and these transformations exhibited moderate to good yield. Unsurprisingly, a strong electron-withdrawing group (–CF3) on the phenyl ring significantly reduced the reactivity and afforded the desired product in a much lower yield (22%) but still with high enantioselectivity (1f, 98% ee). At the same time, Cl- and Ph-substituents were well tolerated to afford 3ea (61%, 90% ee) and 3ga (52%, 98% ee) smoothly. Multisubstituted cinnamic acid derivatives 1i and 1j were also successfully arylated in good yields and excellent enantioselectivities (3ia, 65%, 98% ee; and 3ja 72%, 91% ee). The substituent effect on naphthalene was also investigated, which showed that methyl and phenyl were quite compatible in this transformation (3ka, 62%, 95% ee; 3la, 53%, 97% ee). Notably, a pyrene group connected to the styrene was also tolerated to provide the desired arylation product (3ma) in 41% yield and with 97% ee. The scope of different aryl-boronic acid pinacol esters has also been demonstrated in this C–H arylation system. Electron-withdrawing groups at the para-position such as cyano, trifluoromethyl, nitro, and phenyl gave the desired chiral styrenes (3ad–3ag) in moderate to good yields and with excellent enantioselectivities. The coupling of 1a with electron-rich aryl-boronic acid pinacol ester 2c afforded 3ac in a yield of 66% with slightly lower enantioselectivity (89% ee). To our delight, halogen substituents were also well tolerated in this reaction, affording 3ah and 3ai in 96% and 94% ee, respectively. Such halogenated chiral styrenes could be further transformed into various functional groups through late-stage cross-coupling reactions. Meta-substituted aryl-boronic acid pinacol esters 2j and 2k were also well compatible with the reaction conditions, furnishing the corresponding products 3aj and 3ak both in 96% ee. Multi-substituted substrates afforded the desired products (3al–3an) in slightly lower yields with similar enantioselectivities.
Table 2 Substrate scope of Pd catalyzed enantioselective C–H arylationa,b,c
Rac-1 (0.2 mmol), 2 (2.0 equiv.), Pd(OAc)2 (0.1 equiv.), Boc-L-tert-leucine (0.2 equiv.), BQ (0.5 equiv.), Ag2CO3 (1.5 equiv.), KHCO3 (2.0 equiv.), H2O (20.0 equiv.) in tAmylOH 1.0 mL in air at 40 °C for 72 h, the crude mixture was methylated using MeI.
Isolated yields.
The ee value was determined by HPLC.
|
|
To further expand the diversity of such axially chiral styrene-type CCAs, Pd-catalyzed C–H olefination reactions were then explored with 1a and methyl acrylate 4a as model substrates (see the ESI† for detailed optimizations). The desired olefination product 5aa was obtained in 97% yield and 97% ee with 10 mol% Pd(OAc)2 used as the catalyst and 30 mol% Boc-L-tert-leucine used as the ligand, and 2.0 equiv. of KOH as the base under 1 atm O2 in iPrOH at 30 °C for 72 h. The absolute configuration of 5aa was determined to be R by single crystal X-ray diffraction,19, which is in contrast to that of the product furnished by the TDG strategy in spite of using ligands derived from the same amino acid. To rationalize the origin of the stereoselectivity, according to previous studies, stereo-models of the transition states of two different catalysis systems are proposed in Scheme 2. In TS-1, palladium is coordinated with the MPAA ligand and the substrate in a square-planar coordination. The bulky side-chain of the amino acid points upward, which pushes the alkenyl group away from the palladium coordination plane to avoid steric repulsion.17h,20 However, in TS-2, palladium fused with cinnamaldehyde imine presents a square-planar coordination. The upward side-chain extrudes the alkenyl group downward, which leads to contrary absolute configurations.15b,21
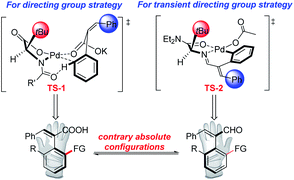 |
| Scheme 2 Transient states of two catalysed models. | |
With the optimized conditions in hand, we sought to test the olefination scope under the standard conditions (Table 3). To our delight, these transformations exhibited high efficiency, and moderate to good yields and excellent enantioselectivities were achieved in most cases. Alkyl groups (1b–1c) and chloro-(1d) on the cinnamic acid were well-tolerated, giving high enantiocontrol (5aa–5da, 90–99% ee). As expected, when a trifluoromethyl substituent was tested, inferior yield was obtained, albeit with a high enantioselectivity (5fa, 47%, 99% ee). In addition, phenyl- and MeOCH2- at the ortho position were well compatible with this transformation, and furnished the corresponding products 5ga and 5ha with high ee in moderate yield. The olefinated product 5ia was obtained in 87% yield with 98% ee when multisubstituted cinnamic acid derivative 1i was employed. Notably, substituted naphthalenes (1k, 1l) also served as suitable coupling partners with good stereocontrol as well as high yields (5ka, 79%, 89% ee; and 5la, 77%, 93% ee). To our delight, substrate 1m bearing a pyrene group gave the desired olefination product smoothly, in a yield of 54% with 99% ee. Various other acrylates were compatible with this reaction, affording corresponding products (5ab–5ah) in good yields and excellent enantioselectivities. Most remarkably, 4-methoxystyrene and 4-trifluoromethylstyrene were also suitable partners for this transformation, producing 5ai and 5aj in yields of 59% and 60%, respectively, with excellent enantioselectivities of 98% ee and 96% ee.
Table 3 Substrate scope of Pd catalyzed enantioselective C–H olefinationa,b,c
Rac-1 (0.2 mmol), 4 (3.0 equiv.), Pd(OAc)2 (0.1 equiv.), Boc-L-tert-leucine (0.3 equiv.), KOH (2.0 equiv.), H2O (10.0 equiv.) in iPrOH 2.0 mL in 1 atm O2 at 30 °C for 72 h, the crude mixture was methylated using MeI.
Isolated yields.
The ee value was determined by HPLC.
84 h.
108 h.
132 h.
|
|
To gain insights into the utility of the reaction, gram-scale synthesis and transformations were conducted (Scheme 3). First, the reaction of rac-1a and 2b on a 5 mmol scale was performed, and the desired CCA 1 was isolated with retentive enantioselectivity (77%, 94% ee, 1.34 g). The 5 mmol scale reaction of rac-1a and 4a gave 5aa in a yield of 95% and 95% ee. Subsequently, CCA 1 was reduced to form alcohol 6 under mild conditions with a mild loss in enantioselectivity. The olefination product 5aa could undergo a van Leusen reaction and afford the hetero-aryl compound 7 in 77% yield and 93% ee.
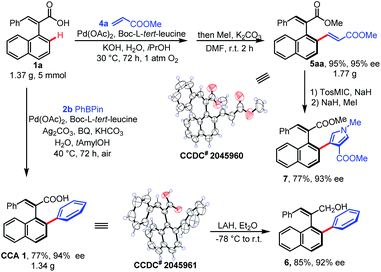 |
| Scheme 3 Gram scale reactions and transformations of CCA 1 and 5aa. | |
In order to demonstrate the application prospect of our methodology, both olefinated and arylated axially chiral styrene-type carboxylic acids (CCA 1–CCA 6) were treated as chiral ligands in a CoIII-catalyzed asymmetric synthesis, as shown in Scheme 4. In 2019, Matsunaga and co-workers demonstrated that the combination of an achiral CoIII complex and CCA promotes asymmetric C(sp3)-H amidation reactions of thioamides 8 with dioxazolones 9.4f,g A series of newly developed axially chiral styrene-type CCAs were employed (Scheme 4a), and the desired product 10 was obtained in moderate to good yields. When CCA 6 was employed, a better enantioselectivity (17
:
83 er) was obtained. Meanwhile, enantioselective 1,4-addition reaction of indole 11 with maleimide 12 which proceeds via a C2-selective C–H activation was also conducted.4i The product 13 was obtained in 49% yield with 77
:
23 er, when CCA 4 was employed (Scheme 4b). Although the enantioselectivity of the aforementioned transformations needs to be improved, these results demonstrate that the chiral styrene-type CCA skeleton is promising in developing a new class of chiral ligands and may find more applications in catalytic asymmetric transformations.
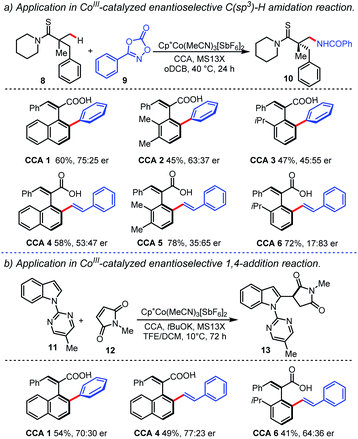 |
| Scheme 4 Application of axially chiral styrene-type carboxylic acids as ligands in CoIII-catalyzed enantioselective C–H activation reactions. | |
Conclusions
In conclusion, we have developed palladium-catalyzed C–H arylation or olefination of cinnamic acid derivatives for facile synthesis of axially chiral styrene-type carboxylic acids through a one-step incorporation of axial chirality and sterically hindered group. This method demonstrated broad scope, good yields, excellent enantioselective control, and mild conditions. The axially chiral styrene-type carboxylic acids produced have been successfully applied to Cp*CoIII-catalyzed asymmetric C–H activation reactions as potential chiral ligands. This strategy offered a valuable solution for rapid and efficient construction of novel axially chiral styrenes, which may serve as a class of novel chiral ligands or catalysts in asymmetric synthesis. Further exploration of asymmetric C–H activation to enable axially chiral motifs is underway in our laboratory.
Conflicts of interest
The authors declare no conflict of interest.
Acknowledgements
We gratefully acknowledge the Strategic Priority Research Program of the Chinese Academy of Sciences (Grant No. XDB20000000) and the National Science Foundation of China (21971228, 21772187, 21602213) for financial support. We thank the National Demonstration Center for Experimental Chemistry Education (University of Science and Technology of China) for help with HRMS analysis.
Notes and references
- For selected reviews, see:
(a) G. Casiraghi and F. Zanardi, Chem. Rev., 1995, 95, 1677 CrossRef CAS;
(b) P. Singh, K. Samanta, S. K. Das and G. Panda, Org. Biomol. Chem., 2014, 12, 6297 RSC;
(c) S.-M. Paek, M. Jeong, J. Jo, Y. M. Heo, Y. T. Han and H. Yun, Molecules, 2016, 21, 951 CrossRef PubMed;
(d) J. Moschner, V. Stulberg, R. Fernandes, S. Huhmann, J. Leppkes and B. Koksch, Chem. Rev., 2019, 119, 10718 CrossRef CAS PubMed;
(e) N. Kratena, T. Gökler, L. Maltrovsky, E. Oburger and C. Stanetty, Chem.–Eur. J., 2021, 27, 577 CrossRef CAS PubMed;
(f) S. L. K. Manda, S. Tripathi, A. Ghoshal, M. D. Ambule, A. K. Sirvastava and G. Panda, Chem.–Eur. J., 2020, 26, 5131 CrossRef PubMed.
- For selected reviews, see:
(a) Ed. T. Hashimoto and K. Maruoka, Asymmetric Organocatalysis 2: Brønsted AcidCatalysts Other than Phosphoric Acids, Georg Thieme Verlag, Stuttgart, New York, 2012, vol. 2, pp. 279–296 Search PubMed;
(b) T. Akiyama and K. Mori, Chem. Rev., 2015, 115, 9277 CrossRef CAS PubMed;
(c) C. Min and D. Seidel, Chem. Soc. Rev., 2017, 46, 5889 RSC; for selected examples, see:
(d) T. Hashimoto and K. Maruoka, J. Am. Chem. Soc., 2007, 129, 10054 CrossRef CAS PubMed;
(e) T. Hashimoto, N. Uchiyama and K. Maruoka, J. Am. Chem. Soc., 2008, 130, 14380 CrossRef CAS PubMed;
(f) T. Hashimoto, M. Hirose and K. Maruoka, J. Am. Chem. Soc., 2008, 130, 7556 CrossRef CAS PubMed;
(g) T. Hashimoto, H. Kimura, H. Nakatsu and K. Maruoka, J. Org. Chem., 2011, 76, 6030 CrossRef CAS PubMed;
(h) T. Hashimoto, Y. Takiguchi and K. Maruoka, J. Am. Chem. Soc., 2013, 135, 11473 CrossRef CAS PubMed.
- For selected reviews, see:
(a) H. M. L. Davies and R. E. J. Beckwith, Chem. Rev., 2003, 103, 2861 CrossRef CAS PubMed;
(b) H. M. L. Davies, Angew. Chem., Int. Ed., 2006, 45, 6422 CrossRef CAS PubMed;
(c) H. M. L. Davies and J. R. Manning, Nature, 2008, 451, 417 CrossRef CAS PubMed;
(d) H. M. L. Davies and J. R. Denton, Chem. Soc. Rev., 2009, 38, 3061 RSC;
(e) M. P. Doyle, R. Duffy, M. Ratnikov and L. Zhou, Chem. Rev., 2010, 110, 704 CrossRef CAS PubMed;
(f) H. M. L. Davies and D. Morton, Chem. Soc. Rev., 2011, 40, 1857 RSC;
(g) H. M. L. Davies and K. Liao, Nat. Rev. Chem., 2019, 3, 347 CrossRef CAS PubMed; for selected examples, see:
(h) K. Liao, S. Negretti, D. G. Musaev, J. Bacsa and H. M. L. Davies, Nature, 2016, 533, 230 CrossRef CAS PubMed;
(i) K. Liao, T. C. Pickel, V. Boyarskikh, J. Bacsa, D. G. Musaev and H. M. L. Davies, Nature, 2017, 551, 609 CrossRef CAS PubMed;
(j) J. Fu, Z. Ren, J. Bacsa, D. G. Musaev and H. M. L. Davies, Nature, 2018, 564, 395 CrossRef CAS PubMed;
(k) Z. J. Garlets, J. N. Sanders, H. Malik, C. Gampe, K. N. Houk and H. M. L. Davies, Nat. Catal., 2020, 3, 351 CrossRef CAS.
-
(a) D. Gwon, S. Park and S. Chang, Tetrahedron, 2015, 71, 4504 CrossRef CAS;
(b) Y.-S. Jang, M. Dieckmann and N. Cramer, Angew. Chem., Int. Ed., 2017, 56, 15088 CrossRef CAS PubMed;
(c) Y.-S. Jang, Ł. Woźniak, J. Pedroni and N. Cramer, Angew. Chem., Int. Ed., 2018, 57, 12901 CrossRef CAS PubMed;
(d) M. Brauns and N. Cramer, Angew. Chem., Int. Ed., 2019, 58, 8902 CrossRef CAS PubMed;
(e) L. Lin, S. Fukagawa, D. Sekine, E. Tomita, T. Yoshino and S. Matsunaga, Angew. Chem., Int. Ed., 2018, 57, 12048 CrossRef CAS PubMed;
(f) S. Fukagawa, Y. Kato, R. Tanaka, M. Kojima, T. Yoshino and S. Matsunaga, Angew. Chem., Int. Ed., 2019, 58, 1153 CrossRef CAS PubMed;
(g) D. Sekine, K. Ikeda, S. Fukagawa, M. Kojima, T. Yoshino and S. Matsunaga, Organometallics, 2019, 38, 3921 CrossRef CAS;
(h) S. Fukagawa, M. Kojima, T. Yoshino and S. Matsunaga, Angew. Chem., Int. Ed., 2019, 58, 18154 CrossRef CAS PubMed;
(i) T. Kurihara, M. Kojima, T. Yoshino and S. Matsunaga, Asian J. Org. Chem., 2020, 9, 368 CrossRef CAS;
(j) L.-T. Huang, S. Fukagawa, M. Kojima, T. Yoshino and S. Matsunaga, Org. Lett., 2020, 22, 8256 CrossRef CAS PubMed;
(k) F. Pesciaioli, U. Dhawa, J. C. A. Oliveira, R. Yin, M. John and L. Ackermann, Angew. Chem., Int. Ed., 2018, 57, 15425 CrossRef CAS PubMed;
(l) Y.-H. Liu, P.-X. Li, Q.-J. Yao, Z.-Z. Zhang, D.-Y. Huang, M. D. Le, H. Song, L. Liu and B.-F. Shi, Org. Lett., 2019, 21, 189 Search PubMed;
(m) L. Liu, H. Song, Y.-H. Liu, L.-S. Wu and B.-F. Shi, ACS Catal., 2020, 10, 7117 CrossRef CAS;
(n) Q. Wang, W.-W. Zhang, H. Song, J. Wang, C. Zheng, Q. Gu and S.-L. You, J. Am. Chem. Soc., 2020, 142, 15678 CrossRef CAS PubMed.
-
(a) J. He, M. Wasa, K. S. L. Chan, Q. Shao and J.-Q. Yu, Chem. Rev., 2017, 117, 8754 CrossRef CAS PubMed;
(b) C. G. Newton, S.-G. Wang, C. C. Oliveira and N. Cramer, Chem. Rev., 2017, 117, 8908 CrossRef CAS PubMed;
(c) T. G. Saint-Denis, R.-Y. Zhu, G. Chen, Q.-F. Wu and J.-Q. Yu, Science, 2018, 359, eaao4798 CrossRef PubMed;
(d) Ł. Woźniak and N. Cramer, Trends Chem., 2019, 1, 471 CrossRef;
(e) J. Loup, U. Dhawa, F. Pesciaioli, J. Wencel-Delord and L. Ackermann, Angew. Chem., Int. Ed., 2019, 58, 12803 CrossRef CAS;
(f) G. Liao, T. Zhang, Z.-K. Lin and B.-F. Shi, Angew. Chem., Int. Ed., 2020, 59, 19773 CrossRef CAS PubMed.
- For selected reviews, see:
(a) T. Satoh and M. Miura, Chem.–Eur. J., 2010, 16, 11212 CrossRef CAS PubMed;
(b) N. Kuhl, N. Schröder and F. Glorius, Adv. Synth. Catal., 2014, 356, 1443 CrossRef CAS;
(c) G. Song and X. Li, Acc. Chem. Res., 2015, 48, 1007 CrossRef CAS PubMed;
(d) T. Yoshino and S. Matsunaga, Adv. Synth. Catal., 2017, 359, 1245 CrossRef CAS;
(e) T. Piou and T. Rovis, Acc. Chem. Res., 2018, 51, 170 CrossRef CAS PubMed;
(f) J. Park and S. Chang, Chem.–Asian J., 2018, 13, 1089 CrossRef CAS PubMed;
(g) A. Peneau, C. Guillou and L. Chabaud, Eur. J. Org. Chem., 2018, 2018, 5777 CrossRef CAS; for pioneering works, see:
(h) B. Ye and N. Cramer, Science, 2012, 338, 504 CrossRef CAS PubMed;
(i) T. K. Hyster, L. Knörr, T. R. Ward and T. Rovis, Science, 2012, 338, 500 CrossRef CAS PubMed.
-
(a) T. Yoshino and S. Matsunaga, Synlett, 2019, 30, 1384 CrossRef CAS;
(b) T. Yoshino, S. Satake and S. Matsunaga, Chem.–Eur. J., 2020, 26, 7346 CrossRef CAS PubMed.
-
(a) O. Baudoin, Eur. J. Org. Chem., 2005, 2005, 4223 CrossRef;
(b) G. Bringmann, A. J. Price Mortimer, P. A. Keller, M. J. Gresser, J. Garner and M. Breuning, Angew. Chem., Int. Ed., 2005, 44, 5384 CrossRef CAS PubMed;
(c) K. Tanaka, Chem.–Asian J., 2009, 4, 508 CrossRef CAS PubMed;
(d) M. C. Kozlowski, B. J. Morgan and E. C. Linton, Chem. Soc. Rev., 2009, 38, 3193 RSC;
(e) G. Bringmann, T. Gulder, T. A. M. Gulder and M. Breuning, Chem. Rev., 2011, 111, 563 CrossRef CAS PubMed;
(f) J. Wencel-Delord, A. Panossian, F. R. Leroux and F. Colobert, Chem. Soc. Rev., 2015, 44, 3418 RSC;
(g) Y.-B. Wang and B. Tan, Acc. Chem. Res., 2018, 51, 534 CrossRef CAS PubMed;
(h) A. Link and C. Sparr, Chem. Soc. Rev., 2018, 47, 3804 RSC;
(i) G. Liao, T. Zhou, Q.-J. Yao and B.-F. Shi, Chem. Commun., 2019, 55, 8514 RSC.
- For selected review, see:
(a) E. Kumarasamy, R. Raghunathan, M. P. Sibi and J. Sivaguru, Chem. Rev., 2015, 115, 11239 CrossRef CAS PubMed; for pioneering studies, see:
(b) R. W. Maxwell and R. Adams, J. Am. Chem. Soc., 1930, 52, 2959 CrossRef CAS;
(c) W. H. Mills and G. H. Dazeley, J. Chem. Soc., 1939, 460 RSC.
- For selected reviews, see:
(a) C. Defieber, H. Grützmacher and E. M. Carreira, Angew. Chem., Int. Ed., 2008, 47, 4482 CrossRef CAS PubMed;
(b) X. Feng and H. Du, Asian J. Org. Chem., 2012, 1, 204 CrossRef CAS;
(c) Y. Li and M.-H. Xu, Chem. Commun., 2014, 50, 3771 RSC;
(d) M. Nagamoto and T. Nishimura, ACS Catal., 2017, 7, 833 CrossRef CAS.
-
(a) K. Mori, K. Ohmori and K. Suzuki, Angew. Chem., Int. Ed., 2009, 48, 5633 CrossRef CAS PubMed;
(b) K. Mori, K. Ohmori and K. Suzuki, Angew. Chem., Int. Ed., 2009, 48, 5638 CrossRef CAS PubMed;
(c) T. Hattori, M. Date, K. Sakurai, N. Morohashi, H. Kosugi and S. Miyano, Tetrahedron Lett., 2001, 42, 8035 CrossRef CAS;
(d) R. W. Baker, T. W. Hambley, P. Turner and B. J. Wallace, Chem. Commun., 1996, 2571 RSC.
-
(a) J. Feng, B. Li, Y. He and Z.-H. Gu, Angew. Chem., Int. Ed., 2016, 55, 2186 CrossRef CAS PubMed;
(b) C. Pan, Z. Zhu, M. Zhang and Z.-H. Gu, Angew. Chem., Int. Ed., 2017, 56, 4777 CrossRef CAS PubMed;
(c) J. D. Jolliffe, R. J. Armstrong and M. D. Smith, Nat. Chem., 2017, 9, 558 CrossRef CAS PubMed;
(d) Q.-Y. Sun, W.-Y. Ma, K.-F. Yang, J. Cao, Z.-J. Zheng, Z. Xu, Y.-M. Cui and L.-W. Xu, Chem. Commun., 2018, 54, 10706 RSC.
- S. C. Zheng, S. Wu, Q. Zhou, L. W. Chung, L. Ye and B. Tan, Nat. Commun., 2017, 8, 15238 CrossRef PubMed.
-
(a) S. Jia, Z. Chen, N. Zhang, Y. Tan, Y. Liu, J. Deng and H. Yan, J. Am. Chem. Soc., 2018, 140, 7056 CrossRef CAS PubMed;
(b) A. Huang, L. Zhang, D. Li, Y. Liu, H. Yan and W. Li, Org. Lett., 2019, 21, 95 CrossRef CAS PubMed;
(c) Y. Tan, S. Jia, F. Hu, Y. Liu, L. Peng, D. Li and H. Yan, J. Am. Chem. Soc., 2018, 140, 7056 CrossRef PubMed;
(d) D. Li, Y. Tan, L. Peng, S. Li, N. Zhang, Y. Liu and H. Yan, Org. Lett., 2018, 20, 4959 CrossRef CAS PubMed.
-
(a) L. Jin, Q.-J. Yao, P.-P. Xie, Y. Li, B.-B. Zhan, Y.-Q. Han, X. Hong and B.-F. Shi, Chem, 2020, 6, 497 CrossRef CAS;
(b) H. Song, Y. Li, Q.-J. Yao, L. Jin, L. Liu, Y.-H. Liu and B.-F. Shi, Angew. Chem., Int. Ed., 2020, 59, 6576 CrossRef CAS PubMed.
-
(a) X.-F. Cheng, Y. Li, Y.-M. Su, F. Yin, J.-Y. Wang, J. Sheng, H. U. Vora, X.-S. Wang and J.-Q. Yu, J. Am. Chem. Soc., 2013, 135, 1236 CrossRef CAS PubMed;
(b) Y.-C. Zhu, Y. Li, B.-C. Zhang, F.-X. Zhang, Y.-N. Yang and X.-S. Wang, Angew. Chem., Int. Ed., 2018, 57, 5129 CrossRef CAS PubMed;
(c) X.-F. Cheng, F. Fei, Y. Li, Y.-M. Hou, X. Zhou and X.-S. Wang, Org. Lett., 2020, 22, 6394 CrossRef CAS PubMed;
(d) Y. Li, X.-F. Cheng, F. Fei, T.-R. Wu, K.-J. Bian, X. Zhou and X.-S. Wang, Chem. Commun., 2020, 56, 11605 RSC.
- For pioneering work, see:
(a) B.-F. Shi, N. Maugel, Y.-H. Zhang and J.-Q. Yu, Angew. Chem., Int. Ed., 2008, 47, 4882 CrossRef CAS PubMed; for selected recent examples:
(b) G. Chen, W. Gong, Z. Zhuang, M. S. Andr-, Y.-Q. Chen, X. Hong, Y.-F. Yang, T. Liu, K. N. Houk and J.-Q. Yu, Science, 2016, 353, 1023 CrossRef CAS PubMed;
(c) Q. Shao, Q.-F. Wu, J. He and J.-Q. Yu, J. Am. Chem. Soc., 2018, 140, 5322 CrossRef CAS PubMed;
(d) L. Hu, P.-X. Shen, Q. Shao, K. Hong, J. X. Qiao and J.-Q. Yu, Angew. Chem., Int. Ed., 2019, 58, 2134 CrossRef CAS PubMed;
(e) E. A. Romero, G. Chen, M. Gembicky, R. Jazzar, J.-Q. Yu and G. Bertrand, J. Am. Chem. Soc., 2019, 141, 16726 CrossRef CAS PubMed; for selected reviews, see:
(f) K. M. Engle and J.-Q. Yu, J. Org. Chem., 2013, 78, 8927 CrossRef CAS PubMed;
(g) K. M. Engle, Pure Appl. Chem., 2016, 88, 119 CAS;
(h) Q. Shao, K. Wu, Z. Zhuang, S. Qian and J.-Q. Yu, Acc. Chem. Res., 2020, 53, 833 CrossRef CAS PubMed.
- Carboxyl group serves as weakly coordinating group has been well developed, for selected recent examples:
(a) L. Liu, Y.-H. Liu and B.-F. Shi, Chem. Sci., 2020, 11, 290 RSC;
(b) J. Das, P. Dolui, W. Ali, J. P. Biswas, H. B. Chandrashekar, G. Prakash and D. Maiti, Chem. Sci., 2020, 11, 9697 RSC.
- CCDC 2045960 and 2045961 (5aa and CCA1) contains the supplementary crystallographic data for this paper.†.
-
(a) B.-F. Shi, Y.-H. Zhang, J. K. Lam, D.-H. Wang and J.-Q. Yu, J. Am. Chem. Soc., 2010, 132, 460 CrossRef CAS PubMed;
(b) D. G. Musaev, A. Kaledin, B.-F. Shi and J.-Q. Yu, J. Am. Chem. Soc., 2012, 134, 1690 CrossRef CAS PubMed;
(c) G.-J. Cheng, Y.-F. Yang, P. Liu, P. Chen, T.-Y. Sun, G. Li, X. Zhang, K. N. Houk, J.-Q. Yu and Y.-D. Wu, J. Am. Chem. Soc., 2014, 136, 894 CrossRef CAS PubMed;
(d) G.-J. Cheng, P. Chen, T.-Y. Sun, X. Zhang, J.-Q. Yu and Y.-D. Wu, Chem.–Eur. J., 2015, 21, 11180 CrossRef CAS PubMed.
-
(a) F.-L. Zhang, K. Hong, T.-J. Li, H. Prak and J.-Q. Yu, Science, 2016, 351, 252 CrossRef CAS PubMed;
(b) Q.-J. Yao, S. Zhang, B.-B. Zhan and B.-F. Shi, Angew. Chem., Int. Ed., 2017, 56, 6617 CrossRef CAS PubMed;
(c) P. Gandeepan and L. Ackermann, Chem, 2018, 4, 199 CrossRef CAS;
(d) H. Park, P. Verma, K. Hong and J.-Q. Yu, Nat. Chem., 2018, 10, 755 CrossRef CAS PubMed;
(e) J. Xu, Y. Liu, J. Zhang, X. Xu and Z. Jin, Chem. Commun., 2018, 54, 689 RSC.
Footnote |
† Electronic supplementary information (ESI) available. CCDC 2045960 and 2045961. For ESI and crystallographic data in CIF or other electronic format see DOI: 10.1039/d0sc06661c |
|
This journal is © The Royal Society of Chemistry 2021 |
Click here to see how this site uses Cookies. View our privacy policy here.