DOI:
10.1039/D1SC00283J
(Edge Article)
Chem. Sci., 2021,
12, 5253-5258
Nickel-catalyzed asymmetric reductive arylation of α-chlorosulfones with aryl halides†
Received
15th January 2021
, Accepted 18th February 2021
First published on 19th February 2021
Abstract
We report an asymmetric Ni-catalyzed reductive cross-coupling of aryl/heteroaryl halides with racemic α-chlorosulfones to afford enantioenriched sulfones. The reaction tolerates a variety of functional groups under mild reaction conditions, which complements the current methods. The utility of this work was demonstrated by facile late-stage functionalization of commercial drugs.
Introduction
Sulfones are not only important functional units in synthetic chemistry1 but also unique compounds that have been widely studied in medicinal chemistry.2 Among them, a number of alkyl sulfones are FDA-approved drugs (e.g., bicalutamide for prostate cancer and apremilast for psoriatic arthritis).3 Many efforts based upon transition-metal catalyzed coupling reactions have been devoted to the functionalization of the α-positions of alkylsulfones.4–6 However, asymmetric methods are largely confined to Tsuji–Trost allylation and Michael addition reactions by virtue of formation of relatively stable α-sulfonyl carbanions.6 Fu and co-workers have reported the first enantioselective Negishi coupling of α-bromosulfonamides and -sulfones with organozinc and organozirconium reagents to furnish secondary benzylic and allylic sulfonamides and sulfones in good yields and high levels of enantioselectivities.7 Asymmetric alkylation of α-bromosulfonamides with alkenes was later found to be effective under Ni–H–alkene insertion/alkylation conditions which afforded enantioenriched α-alkyl sulfones and sulfonamides.8 In both cases, the involvement of α-sulfonyl carbon radicals is thought to be key. Although this progress significantly advances the asymmetric functionalization of α-sulfone derivatives, there are a few issues remaining to be addressed. First, asymmetric introduction of heteroaromatics to the α-carbon of sulfones remains elusive.8,9 Second, the substrate scope may be limited with the current methods due to the use of organometallic nucleophiles and terminal alkenes.7,8 We envision that a cross-electrophile coupling strategy that utilizes readily accessible aryl electrophiles and α-halosulfones may otherwise exhibit different substrate scope and functional group tolerance compared to the reported methods.10
In this work, we present a Ni-catalyzed reductive cross-coupling strategy for asymmetric arylation of α-chlorosulfones with aryl halides.11–13 The reaction offers expeditious access to enantioenriched sulfones with no need of pre-generating organozinc reagents, and displays broad substrate scope including a set of heteroaromatics with excellent functional group tolerance. To the best of our knowledge, this work represents the first asymmetric functionalization of α-sulfonyl compounds using the cross-electrophile coupling strategy that complements the reported Negishi protocol.
Results and discussion
Optimization of the reaction conditions
Our investigations began with the coupling between 1 and 2 using chiral bis(oxazoline) ligands (Table 1).14 An extensive screening of reaction parameters revealed that the use of NiBr2(dme) (10 mol%) and L1 (20 mol%), Zn as the reductant and MgCl2 as the additive in DMA delivered sulfone 3 in 73% yield and 91% ee (Table 1, entry 1). Under the latter conditions, replacing L1 with other chiral dibox ligands L2–L6 did not result in better outcomes in terms of both yields and ee's (entries 2–6). The use of Mn to replace Zn diminished the ee (entry 7). MgCl2 appeared to be crucial for this transformation, whereas MgBr2 is much less effective, and TBAB gave trace amounts of the coupling product (entries 8–9).15 Change of other parameters such as solvent and the ratio of the coupling partners was not satisfactory (Table S1†).14 The addition of the pre-coordinated L1·NiBr2 complex (10 mol%) as the precatalyst to replace NiBr2(dme) (10 mol%) and L1 (20 mol%) led to slightly higher yield and the same ee (entry 10).
Table 1 Optimization for the formation of 3a
Reactions are conducted under N2 on a 0.15 mmol scale for 48 h; NMR yield is obtained using 2,5-dimethyl furan as the internal standard, ee is determined by chiral HPLC analysis, DMF = N,N-dimethyl formamide.
|
|
Entry |
Deviation from the standard conditions |
Yield% (ee%) |
1 |
No changes |
73 (91) |
2 |
L2 (20%) instead of L1 |
71 (90) |
3 |
L3 (20%) instead of L1 |
28 (88) |
4 |
L4 (20%) instead of L1 |
34 (40) |
5 |
L5 (20%) instead of L1 |
65 (88) |
6 |
L6 (20%) instead of L1 |
31 (77) |
7 |
Mn instead of Zn |
74 (88) |
8 |
TBAB instead of MgCl2 |
Trace (NA) |
9 |
MgBr2 instead of MgCl2 |
63 (55) |
10 |
L1·NiBr2 (10%) |
76 (91) |
We tentatively speculate that L1 adopting a rigid cyclopropyl linker features a larger bond angle of the C(oxazoline)–C(methylene)–C(oxazoline) bond than those in L2–L4 (entries 1–4), which appears to have a crucial effect on the enantioselectivity. On the other hand, by comparing the results arising from L1, L2 and L5 (entries 1, 2 and 5), it is reasonable to conclude that the rigid tricyclic fused rings in L1 and L2 also fulfill an optimal steric and rigidity requirement for enantioselective control.
Substrate scope
The optimized conditions (Table 1, entry 10) proved to be effective for the preparation of a wide range of chiral α,α-disubstituted sulfones 4–32 (Fig. 1). A variety of aryl bromides bearing electron-withdrawing groups underwent smooth cross-coupling with 1 to furnish the α,α-disubstituted sulfones 4–20 in moderate to good yields and high levels of enantioselectivities (Fig. 1). The functional groups included aryl esters (3, 16–20), electron-poor acetones (4, 7, 14–15), aldehyde (9), amides (10–11), phosphonate (12), ethers (14, 17), sulfone (6), sulphonamide (13), and aryl chloride (18). For electron-neutral and -rich arenes, aryliodides were found to effectively couple with 1, furnishing 21–32 in good yields and high ees. The competent functional groups included boronates (26), ethers (24, 31) and ester (29).
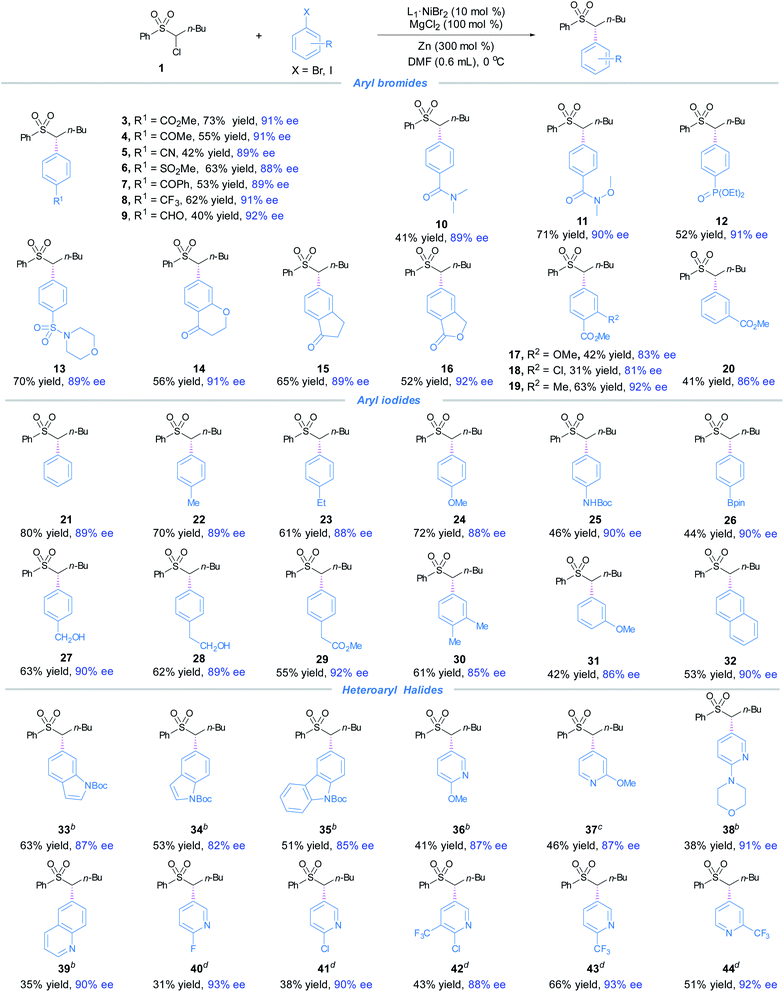 |
| Fig. 1 The scope of aryl halides. aThe standard reaction conditions; isolated yields are provided; ee is determined by chiral HPLC analysis. bMn instead of Zn, 1.5 equiv. heteroaryl iodide used. cMn instead of Zn, 1.5 equiv. heteroaryl bromide used. dMn instead of Zn, 2.0 equiv. heteroaryl bromide used, dioxane is used as the solvent, rt. | |
It was noted that amide (25) and alcohols (27–28) with acidic protons were compatible. Lastly, 2-iodonaphthalene gave the product 32 in 53% yield and 90% ee. Next, a range of heteroaryl halides were subjected to the coupling conditions (Fig. 1). By replacing Zn with Mn, the slightly modified reaction conditions effectively accommodated electron-rich indole, 9H-carbazole, quinolone and electron-deficient pyridine moieties to afford enantioenriched α,α-disubstituted products 33–34. By selection of suitable aryl iodides and bromides as the coupling partners, indole, 9H-carbazole and pyridine bearing electron-rich substituents afforded 33–36 and 38, whereas pyridine bearing electron-deficient groups delivered 40–44 in high ees. This asymmetric protocol also exhibited excellent chemoselectivity toward bromides/iodides, leaving (hetero)aryl chlorides untouched (18, 41–42).
Moreover, a broad scope of substituted α-chlorosulfones were investigated for the coupling with methyl 4-bromobenzoate (2) (Fig. 2). The coupling products (45–62) were produced in moderate to good yields and high enantioselectivities. Notable functional groups included vinyl and hydroxyl. The aryl groups attached to the sulfone moieties did not seem to have key effects on the enantioselectivities as evidenced in 54–60. Although α-chloro-arylsulfone electrophiles were the primary focus of our research, α-chlorosulfonamide (61) and α-chloroalkylsulfone (62) were also investigated. The yields were modest and the enantioselectivities were reasonably good.
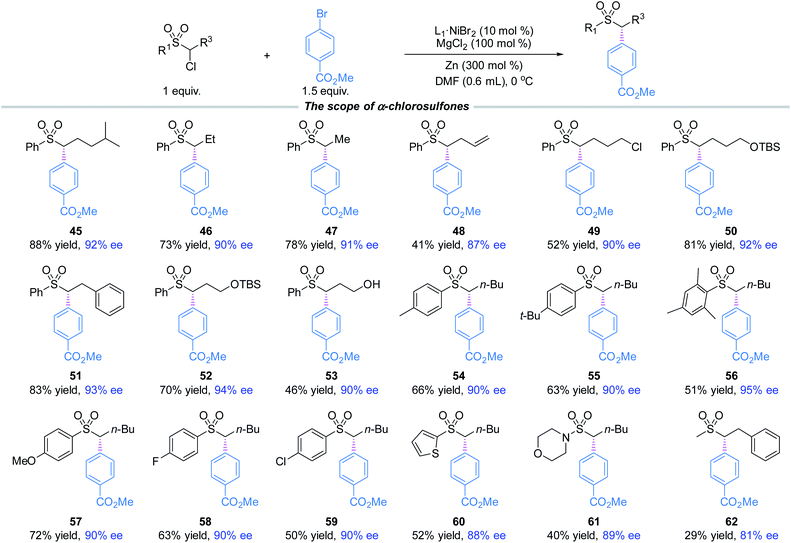 |
| Fig. 2 The standard reaction conditions; isolated yields are provided; ee is determined by chiral HPLC analysis. | |
The applicability of this asymmetric α-sulfonyl arylation method was showcased by late-stage diversification of a number of commercial drugs. To our delight, the coupling of α-chlorosulfone 1 with bromo/iodo-substituted indomethacin, aniracetam, fenofibrate, procacine and clofibrate derivatives delivered the enantioenriched products 63–67 in high ees. Next, we examined the arylation of celecoxib-derived α-chlorosulfone; compounds 68 and 69 were obtained in modest yields and high enantioselectivities (Fig. 3).16
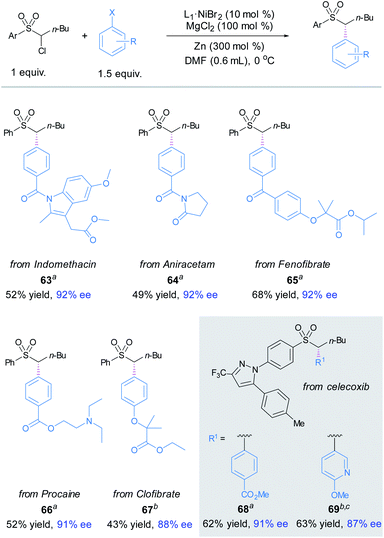 |
| Fig. 3 Late-stage functionalization. The standard reaction conditions; isolated yields are provided; ee is determined by chiral HPLC analysis. aX = Br. bX = I. cMn instead of Zn. | |
We have also examined the coupling of α-chlorosulfone 1 with 4-bromoanisole, and 1 with methyl 4-iodobenzoate. Both reactions gave the products in low yields (∼15%). The mass balance for the reaction with less reactive 4-bromoanisole includes hydro-dechlorination of 1 and a large amount of recovered 4-bromoanisole. In the case of methyl 4-iodobenzoate, we recovered substantial amounts of 1 and the aryl iodide. The first case (with 4-bromoanisole) can be easily attributed to the low reactivity of aryl bromide deactivated by the electron-donating group (MeO), leading to mismatched reactivity of the coupling partners with the Ni catalyst. The inhibition of the reaction when using more reactive methyl 4-iodobenzoate is surprising. We reason that a different competing path may operate, which prevents recycling of the Ni catalyst for the coupling process. Such a phenomenon is often seen in our previous studies, e.g., recovery of aryl–I from the aryl–Ni(II)–I complex.17 Thus, we speculate that the use of electron-poor aryl bromides and electron-neutral and -rich aryl iodides ensures matched reactivities of the coupling partners with the Ni catalyst.
Mechanistic consideration
To understand the possible mechanism of this transformation, several experiments were conducted. A cyclopropyl-containing substrate 70 was prepared and subjected to the standard reaction conditions. We did not detect the corresponding cyclopropane-retaining product 71. Rather, only the ring-opening coupling product 72 was obtained in 23% yield (Fig. 4), which indicated the involvement of a radical ring-opening process.12c,d,15 The radical nature of the coupling event was further verified by the inhibition of the reaction of 1 and 2 with externally added TEMPO (1 equivalent), the yield of 3 dropped to 5% with 90% ee; no TEMPO-trapping adducts were detected.
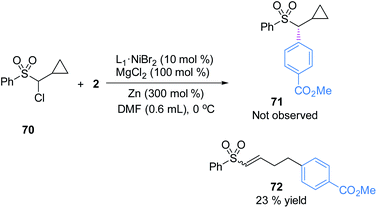 |
| Fig. 4 Radical clock. | |
In general, the coupling of an aryl electrophile with an alkyl halide follows a radical-chain mechanism, as initially proposed by Weix.18 One of the key experiments therein showed that a stable Ar–Ni(II)–X complex can initiate radical formation by reacting with an alkyl iodide to generate the coupling product. The process involves halide abstraction to generate an alkyl radical and an Ar–Ni(III)X2 intermediate. The radical then diffuses to bulk solution and finds Ar–Ni(II)–X to form Ar–Ni(III)X–alkyl, whose reductive elimination gives the product. Following this, we first conducted a catalytic reaction of 1 with methyl 4-bromobenzoate which generated racemic 3 in 65% yield along with the hydrodechlorination product 1-H in 25% yield (eqn (1)). Next, we performed the stoichiometric reaction of Ar–Ni(II)–Br (complex 73) with 1 in DMF at 0 °C, and the racemic product 3 was obtained in 23% yield associated with recovered 1 (eqn (2)). Addition of MgCl2 and/or Zn increased the coupling yield of 3. With Zn, reduction of 1 was observed. These results are in accordance with a radical-chain mechanism, in which the addition of an alkyl radical to Ar–Ni(II)X is featured.
| 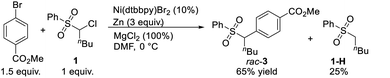 | (1) |
| 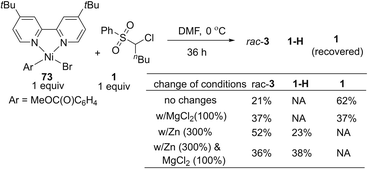 | (2) |
Thus, we propose a mechanism that the oxidative addition of an aryl halide to Ni(0) results in an Ar–Ni(II)–X complex, which intercepts an α-sulfonyl radical to give the Ar–Ni(III)X–alkyl complex, and upon reductive elimination, the product is omitted along with a Ni(I)X complex or a dimeric Ni(I) species.19 The latter species reacts with α-chlorosulfone to generate the alkyl radical and Ni(II)ClX. The radical diffuses to the bulk solution to combine with the Ar–Ni(II)X complex, whereas the Ni(II)ClX is reduced to Ni(0) by Mn, allowing the catalytic cycle to continue (Scheme 1).
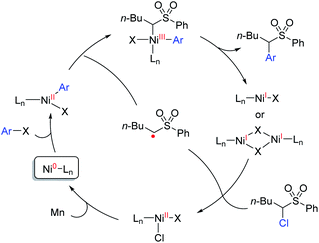 |
| Scheme 1 A working hypothesis of the reaction mechanism. | |
Conclusions
In summary, we have developed a Ni-catalyzed asymmetric reductive cross-coupling between α-chlorosulfones and aryl halides. This transformation enables the synthesis of enantioenriched α,α-disubstituted sulfones from simple organic halides under mild conditions, including easy introduction of a wide set of heteroaromatic moieties into the α-sulfonyl positions. The present method is tolerant of a variety of functional groups, affording the coupling products generally in modest to good yields and high enantioselectivities. Finally, this work provides a convenient tool for late-stage functionalization of bioactive compounds by facile introduction of chiral α-sulfonyl subunits.
Conflicts of interest
There are no conflicts to declare.
Acknowledgements
Financial support was provided by the Chinese NSF (21871173).
Notes and references
- For selected reviews, see:
(a) A. El-Awa, M. N. Noshi, X. Mollat du Jourdin and P. L. Fuchs, Chem. Rev., 2009, 109, 2315 CrossRef CAS PubMed;
(b) A.-N. R. Alba, X. Companyó and R. Rios, Chem. Soc. Rev., 2010, 39, 2018 RSC;
(c) S. Otsuka, K. Nogi and H. Yorimitsu, Top. Curr. Chem., 2018, 376, 13 CrossRef PubMed;
(d) J. Lou, Q. Wang, P. Wu, H. Wang, Y.-G. Zhou and Z. Yu, Chem. Soc. Rev., 2020, 49, 4307 RSC.
-
(a) X. Chen, S. Hussain, S. Parveen, S. Zhang, Y. Yang and C. Zhu, Curr. Med. Chem., 2012, 19, 3578 CrossRef CAS PubMed;
(b) C. Zhao, K. P. Rakesh, L. Ravidar, W.-Y. Fang and H.-L. Qin, Eur. J. Med. Chem., 2019, 162, 679 CrossRef CAS PubMed;
(c) S. S. A. Shah, G. Rivera and M. Ashfaq, Mini-Rev. Med. Chem., 2013, 13, 70 CrossRef CAS PubMed;
(d) M. Feng, B. Tang, S. Liang and X. Jiang, Curr. Top. Med. Chem., 2016, 16, 1200 CrossRef CAS PubMed.
- K. A. Scott and J. T. Njardarson, Top. Curr. Chem., 2018, 376, 5 CrossRef PubMed.
- For RSO2 radical reactions, see:
(a) Q. Lu, J. Zhang, G. Zhao, Y. Qi, H. Wang and A. Lei, J. Am. Chem. Soc., 2013, 135, 11481 CrossRef CAS PubMed;
(b) R. Zhu and S. L. Buchwald, J. Am. Chem. Soc., 2015, 137, 8069 CrossRef CAS PubMed;
(c) J. He, G. Chen, B. Zhang, Y. Li, J.-R. Chen, W.-J. Xiao, F. Liu and C. Li, Chem, 2020, 6, 1149 CrossRef CAS;
(d) X.-T. Li, L. Lv, T. Wang, Q.-S. Gu, G.-X. Xu, Z.-L. Li, L. Ye, X. Zhang, G.-J. Cheng and X.-Y. Liu, Chem, 2020, 6, 1692 CrossRef CASFor introduction of the sulfonyl group from RSO2Na nucleophiles, see:
(e) A. García-Domínguez, R. Mondal and C. Nevado, Angew. Chem., Int. Ed., 2019, 58, 12286 CrossRef PubMed;
(f) A. Khan, M. Zhang and S. Khan, Angew. Chem., Int. Ed., 2020, 59, 1340 CrossRef CAS PubMedUse of sulfones as leaving groups, see:
(g) Z. T. Arika, Y. Maekawa, M. Nambo and C. M. Crudden, J. Am. Chem. Soc., 2018, 140, 78 CrossRef PubMed;
(h) A palladium reductive coupling with a SO2 surrogate, see: Y. Meng, M. Wang and X. Jiang, Angew. Chem., Int. Ed., 2020, 59, 1346 CrossRef CAS PubMed;
(i) G. Qiu, K. Zhou, L. Gao and J. Wu, Org. Chem. Front., 2018, 5, 691 RSC.
- For direct functionalization of the α-positions of alkylsulfones see:
(a) A. N. Kashin, A. V. Mitin, I. P. Beletskaya and R. Wife, Tetrahedron Lett., 2002, 43, 2539 CrossRef CAS;
(b) T. Niwa, H. Yorimitsu and K. Oshima, Tetrahedron, 2009, 65, 1971 CrossRef CAS;
(c) G. Zhou, P. C. Ting and R. G. Aslanian, Tetrahedron Lett., 2010, 51, 939 CrossRef CAS;
(d) B. Zheng, T. Jia and P. J. Walsh, Org. Lett., 2013, 15, 1690 CrossRef CAS PubMed;
(e) M. Nambo and C. M. Crudden, Angew. Chem., Int. Ed., 2014, 53, 742 CrossRef CAS PubMed;
(f) T. Knauber and J. Tucker, J. Org. Chem., 2016, 81, 5636 CrossRef CAS PubMed;
(g) R. R. Kuchukulla, Q. Tang, Y. Huang, Z. He, Li. Zhou and Q. Zeng, Eur. J. Org. Chem., 2020, 4004 CrossRef CAS;
(h) T. Vojkovsky, S. Deolka, S. Stepanova, M. C. Roy and E. Khaskin, ACS Catal., 2020, 10, 6810 CrossRef CASFor an example of arylation of α-alkyl sulfoxides, see:
(i) T. Jia, A. Bellomo, K. EL Baina, S. D. Dreher and P. J. Walsh, J. Am. Chem. Soc., 2013, 135, 3740 CrossRef CAS PubMed.
- For selected examples of asymmetric allylation, see:
(a) T. Fukuzumi, N. Shibata, M. Sugiura, H. Yasui, S. Nakamura and T. Toru, Angew. Chem., Int. Ed., 2006, 45, 4973 CrossRef CAS PubMed;
(b) J. D. Weaver, B. J. Ka, D. K. Morris, W. Thompson and J. A. Tunge, J. Am. Chem. Soc., 2010, 132, 12179 CrossRef CAS PubMed;
(c) A. Cai and W. Kleij, Angew. Chem., Int. Ed., 2019, 58, 14944 CrossRef CAS PubMed.
- J. Choi, P. Martín-Gago and G. C. Fu, J. Am. Chem. Soc., 2014, 136, 12161 CrossRef CAS PubMed.
- S.-J. He, J.-W. Wang, Y. Li, Z.-Y. Xu, X.-X. Wang, X. Lu and Y. Fu, J. Am. Chem. Soc., 2020, 142, 214 CrossRef CAS PubMed.
- The only two examples using 5-indolyl–Zn and 2-(trifluoromethyl)pyridin-4-yl bromide were reported in Fu's Negishi coupling (ref. 8) and Chu's photo-induced three-component arylation of conjugated vinyl sulfones, see: L. Guo, M. Yuan, Y. Zhang, F. Wang, S. Zhu, O. Gutierrez and L. Chu, J. Am. Chem. Soc., 2020, 142, 20390 CrossRef CAS PubMed.
- For an organocatalyzed photo-induced SET reaction of imines with α-iodosulfones involving α-sulfonyl carbon radicals, see: G. Filippini, M. Silvi and P. Melchiorre, Angew. Chem., Int. Ed., 2017, 56, 4447 CrossRef CAS PubMed.
- For selected reviews on cross-electrophile couplings, see:
(a) C. E. I. Knappke, S. Grupe, D. Gärtner, M. Corpet, C. Gosmini and A. Jacobi von Wangelin, Chem.–Eur. J., 2014, 20, 6828 CrossRef CAS PubMed;
(b) T. Moragas, A. Correa and R. Martin, Chem.–Eur. J., 2014, 20, 8242 CrossRef CAS PubMed;
(c) D. J. Weix, Acc. Chem. Res., 2015, 48, 1767 CrossRef CAS PubMed;
(d) M. Holmes, L. A. Schwartz and M. J. Krische, Chem. Rev., 2018, 118, 6026 CrossRef CAS PubMed;
(e) K. E. Poremba, S. E. Dibrell and S. E. Reisman, ACS Catal., 2020, 10, 8237 CrossRef CAS PubMed;
(f) Z. Zhang, J.-H. Ye, T. Ju, L.-L. Liao, H. Huang, Y.-Y. Gui, W.-J. Zhou and D.-G. Yu, ACS Catal., 2020, 10, 10871 CrossRef CAS;
(g) J. Liu, Y. Ye, J. L. Sessler and H. Gong, Acc. Chem. Res., 2020, 53, 1833 CrossRef CAS PubMed.
- For selected examples of asymmetric reductive coupling of activated electrophiles with C(sp2)-electrophiles, see:
(a) A. H. Cherney, N. T. Kadunce and S. E. Reisman, J. Am. Chem. Soc., 2013, 135, 7442 CrossRef CAS PubMed;
(b) A. H. Cherney and S. E. Reisman, J. Am. Chem. Soc., 2014, 136, 14365 CrossRef CAS PubMed;
(c) N. T. Kadunce and S. E. Reisman, J. Am. Chem. Soc., 2015, 137, 10480 CrossRef CAS PubMed;
(d) K. E. Poremba, N. T. Kadunce, N. Suzuki, A. H. Cherney and S. E. Reisman, J. Am. Chem. Soc., 2017, 139, 5684 CrossRef CAS PubMed;
(e) J. L. Hofstra, A. H. Cherney, C. M. Ordner and S. E. Reisman, J. Am. Chem. Soc., 2018, 140, 139 CrossRef CAS PubMed;
(f) T. J. DeLano and S. E. Reisman, ACS Catal., 2019, 9, 6751 CrossRef CAS PubMed;
(g) H. Guan, Q. Zhang, P. J. Walsh and J. Mao, Angew. Chem., Int. Ed., 2020, 59, 5172 CrossRef CAS PubMed.
- For recent examples of reductive coupling of three-components, see:
(a) X. Wei, W. Shu, G.-D. Andres, E. Merino and C. Nevado, J. Am. Chem. Soc., 2020, 142, 13515 CrossRef CAS PubMed;
(b) H.-Y. Tu, F. Wang, L. Huo, Y. Li, S. Zhu, X. Zhao, H. Li, F.-L. Qing and L. Chu, J. Am. Chem. Soc., 2020, 142, 9604 CAS;
(c) Y. Jin and C. Wang, Angew. Chem., Int. Ed., 2019, 58, 6722 CrossRef CAS PubMed;
(d) K. Wang, Z. Ding, Z. Zhou and W. Kong, J. Am. Chem. Soc., 2018, 140, 12364 CrossRef CAS PubMed.
- See the ESI† for details.
- The role of MgCl2 has been discussed in our previous studies of reductive arylation of tertiary alkyl bromides, wherein MgCl2 offers chloride for the formation of Ar–Ni–Cl complexes and activates the Zn surface, see: X. Wang, G. Ma, Y. Peng, C. E. Pitsch, B. J. Moll, T. D. Ly, X. Wang and H. Gong, J. Am. Chem. Soc., 2018, 140, 14490 CrossRef CAS PubMed.
- For synthesis of sulfones from widely studied sulfonamide-containing drugs, see:
(a) P. S. Fier and K. M. Maloney, J. Am. Chem. Soc., 2019, 141, 1441 CrossRef CAS PubMed;
(b) P. S. Fier, S. Kim and K. M. Maloney, J. Am. Chem. Soc., 2019, 141, 18416 CrossRef CAS PubMed.
- X. Tao, Y. Chen, J. Guo, X. Wang and H. Gong, Chem. Sci., 2021, 12, 220 RSC.
- S. Biswas and D. J. Weix, J. Am. Chem. Soc., 2013, 135, 16192 CrossRef CAS PubMed.
- Formation of a dimeric Ni(I) is also possible according to a recent report: M. Mohadjer Beromi, G. W. Brudvig, N. Hazari, H. M. C. Lant and B. Q. Mercado, Angew. Chem., Int. Ed., 2019, 58, 6094 CrossRef CAS PubMed.
Footnote |
† Electronic supplementary information (ESI) available: General experimental information, experimental procedure for product synthesis, full characterization data, and NMR and HPLC spectra of all products related to this article. See DOI: 10.1039/d1sc00283j |
|
This journal is © The Royal Society of Chemistry 2021 |
Click here to see how this site uses Cookies. View our privacy policy here.