DOI:
10.1039/D1SC02896K
(Edge Article)
Chem. Sci., 2021,
12, 11316-11321
Decarboxylative 1,4-carbocyanation of 1,3-enynes to access tetra-substituted allenes via copper/photoredox dual catalysis†
Received
28th May 2021
, Accepted 20th July 2021
First published on 21st July 2021
Abstract
We disclose herein the first example of merging photoredox catalysis and copper catalysis for radical 1,4-carbocyanations of 1,3-enynes. Alkyl N-hydroxyphthalimide esters are utilized as radical precursors, and the reported mild and redox-neutral protocol has broad substrate scope and remarkable functional group tolerance. This strategy allows for the synthesis of diverse multi-substituted allenes with high chemo- and regio-selectivities, also permitting late stage allenylation of natural products and drug molecules.
Introduction
Owing to their unique structural characteristics and properties, allenes represent an important structural unit that is commonly present in a wide range of natural products and bioactive molecules, and they are also versatile building blocks in organic synthesis.1 Classical synthetic methods for allene preparation predominantly rely on functionalized alkynes.2 In recent years, 1,3-enynes have drawn much attention and perceived as desirable precursors for the efficient synthesis of multi-substituted allenes; both organo- and transition metal-catalyzed 1,4-difunctionalizations of 1,3-enynes were successfully developed.3–6 Despite rapid advance of this research field, some problems persist, e.g. the necessity of using activated 1,3-enynes, the requirement of sensitive organometallic reagents, and the difficulty to access tetrasubstituted allenes, among others. As part of our long term interest in utilizing allenes in organic synthesis, we were intrigued to tackle these synthetic challenges.
Visible light photoredox and transition metal dual catalysis has recently emerged as a powerful synthetic strategy, offering a new paradigm for the effective construction of molecular architectures under mild conditions, often in a unique reaction pattern.7 In this context, 1,2-difunctionalizations of alkenes and alkynes via metallaphotoredox catalysis have recently attracted extensive research efforts because of simultaneous functionalizations at two sequential chemical bonding sites in one synthetic step.8–10 Despite the wide utilization of conventional alkenes or alkynes, activation of 1,3-enynes via metallaphotoredox catalysis still remains less explored to date.11 When the functionalizations of 1,3-enynes are concerned, there are a number of key challenges to be addressed. The allenyl radicals generated are short-lived,12 whereas allenes are excellent radical acceptors.1a,13 Controlling regioselectivity of the reaction is another challenge. When the radical addition to 1,3-enynes occurs, propargyl radicals and allenyl radicals are generated, and thus both 1,2-addition14 and 1,4-addition products may be formed.15 Very recently, the Liu group disclosed a divergent synthesis of CF3-substituted allenyl nitriles by using ligand-controlled radical 1,2- and 1,4- additions to 1,3-enynes.16 Shortly after, the Bao group reported a copper-catalyzed 1,4-difunctionalization of 1,3-enynes using diacyl peroxides as radical precursors.17 We hypothesized that merging visible light photoredox catalysis and transition metal catalysis may offer a general strategy through 1,4-difunctionalizations of 1,3-enynes to access a range of structurally diverse multi-substituted allenes. A variety of different radical precursors that can be activated under photoredox conditions may be utilized, and the employment of different transition metals in the proposed dual catalysis pathways will add in an extra dimension. Apparently, it is very challenging to gain good control of chemo- and regio-selectivities of a multi-component catalytic system in a radical process (Fig. 1).
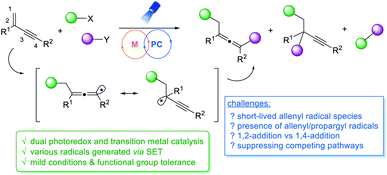 |
| Fig. 1 1,4-Difunctionalizations of 1,3-enynes via photoredox/transition metal dual catalysis. | |
To test our hypothesis, we chose N-hydroxyphthalimide (NHP) esters as a radical precursor, which are readily derived from abundant and inexpensive alkyl carboxylic acids and have been used extensively in radical decarboxylation processes.18 The addition of visible-light-induced radicals to 1,3-enynes to generate allenyl radical species is well anticipated. For the metal catalysis, we reasoned if copper(II) is utilized, it may intercept the advanced allenyl radical to form copper(III) species;19 the reductive elimination of which can lead to the creation of multi-substituted allenes. Herein, we disclose the first metallaphotoredox catalyzed radical 1,4-difunctionalization of 1,3-enynes, furnishing a wide range of tetra-substituted allenes under mild conditions.
Results and discussion
We initiated our investigations by examining the reaction of 1,3-enynes 1a with NHP ester 2a and TMSCN using photoredox/copper dual catalytic systems (Table 1). After extensive screening (see the ESI, Tables S1 & S2†), we obtained 1,4-carbocyanation product 3a in good yield, using a catalytic system consisting of Ir(ppy)3 and Cu(CH3CN)4PF6/bpy in DMF under irradiation with blue LEDs. A solvent screening was subsequently carried out (see the ESI, Table S3†) and dimethylacetamide (DMA) was identified as the solvent of choice. In the presence of 2.5 mol% copper catalyst and 1.0 mol% photocatalyst in DMA, allene 3a was obtained in an isolated yield of 82% (entry 1). The reaction is regiospecific, as no 1,2-addition product was detected. It is noteworthy that the molar ratios of the three reactants had great influence on the chemoselectivity of the reaction. Reducing the molar equivalence of TMSCN or increasing the amount of radical precursor NHP ester led to the decreased yield of 3a, with the formation of Heck-type byproducts20 from 1a and 2a observed. (entries 2 and 3). Further experiments demonstrated that the Cu(CH3CN)4PF6/bpy system, iridium photocatalyst, and visible light are indispensable for obtaining the desired product (entries 4–7). Finally, we showed that the reaction is sensitive towards both air and moisture (entries 8 and 9).
Table 1 Optimization of reaction conditionsa

|
Entry |
Variation from the standard conditions |
Yieldb (%) |
Reaction conditions: 1a (0.2 mmol), 2a (0.2 mmol) and TMSCN (0.4 mmol) in DMA (1.0 mL), Cu(CH3CN)4PF6 (2.5 mol%), bpy (3.5 mol%), Ir(ppy)3 (1.0 mol%), at room temperature, 30 W blue LEDs, 12 h.
Determined by 1H NMR analysis of the crude product with CH2Br2 as an internal standard. Yield of the isolated product given in parentheses.
|
1 |
None |
88 (82) |
2 |
1.1 equiv. TMSCN |
65 |
3 |
1.5 equiv. 2a |
77 |
4 |
No Cu(CH3CN)4PF6 |
<5 |
5 |
No Ir(ppy)3 |
<5 |
6 |
No ligand |
<5 |
7 |
In the dark |
<5 |
8 |
In air |
<5 |
9 |
10 μL H2O was added |
46 |
With the established optimal reaction conditions, we proceeded to examine the reaction scope (Scheme 1). A wide range of 1,3-enynes could be utilized. For the C2-aryl substituent, both electron-donating and electron-withdrawing groups on the aromatic ring were well tolerated, and the corresponding tetra-substituted allenes were obtained in good yields (3a–3f). While the enyne bearing a meta-methyl phenyl substituent was found to be a good substrate (3g), the enynes containing an ortho-methyl phenyl or a naphthyl group at the C2-position led to much decreased yields (3h and 3i). The reaction was also applicable to 1,3-enynes bearing a thiophene, methyl, or cyclopropyl substituent at the 2-position, although the yields of allenes were only modest (3j, 3k, 3l). The alkynyl moiety in the 1,3-enyne structures could also be varied; linear alkyl chains of different lengths (3m, 3n), as well as the branched alkyl group (3o) and benzyl substituent (3p) were all found to be suitable. Moreover, the 1,3-enynes with a chlorine-containing alkyl chain (3q), a sterically hindered tert-butyl group (3r), or without a C4 substituent (3s) could all be utilized for the reaction, and the allene products were obtained in consistently good yields.
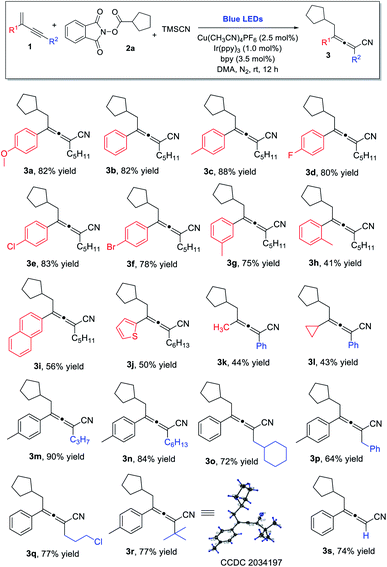 |
| Scheme 1 1,3-Enyne scope. a Reaction conditions: 1 (0.2 mmol), 2a (0.2 mmol) and TMSCN (0.4 mmol) in DMA (1.0 mL), Cu(CH3CN)4PF6 (2.5 mol%), bpy (3.5 mol%), Ir(ppy)3 (1.0 mol%), at room temperature, 30 W blue LEDs, 12 h. Yields given refer to isolated yields. | |
Alkyl carboxylic acids are readily available and inexpensive, and thus their NHP esters are desirable starting materials in chemical transformations. The employment of different NHP esters in this decarboxylative 1,4-carbocyanation of 1,3-enynes allows for the incorporation of a group of diverse alkyl moieties into the tetra-substituted allene products. The substrate scope with regard to redox-active alkyl esters is remarkably broad (Scheme 2). Simple linear alkyl groups of various chain lengths (4a–4e), as well as alkyl substituents bearing an ether (4f), a ketone (4g), an alkene (4h), or a cyclohexyl (4i) moiety at the terminal position, were all found to be compatible with the reaction conditions, leading to the formation of the corresponding allenes in good yields. Moreover, the reaction was also applicable to linear alkyls containing a terminal phenyl, furan, or thiophene (4j–4m). Notably, when benzyl NHP ester was employed, the allene product was obtained in a lower yield (4n), due to the formation of a two-component cross-coupling byproduct.21 Significantly, secondary alkyl carboxylic acid derived NHP esters were amenable to the copper/photoredox catalytic process; cyclobutyl (4o), cyclopropyl (4p), pyran (4q), piperidine (4r), as well as simply branched alkyl chains (4s and 4t) could all be readily incorporated into tetra-substituted allenes. Interestingly, when vinyl NHP ester was used, the anticipated 1,4-carbocyanation product (4u) was formed, along with a non-decarboxylative product (4u′). Lastly, we also examined tertiary alkyl NHP esters in this 1,4-difunctionalization process; remarkably, the desired allenes with highly sterically hindered alkyl groups incorporated were obtained in high yields and with excellent chemo- and regio-selectivities (4v–4zz).
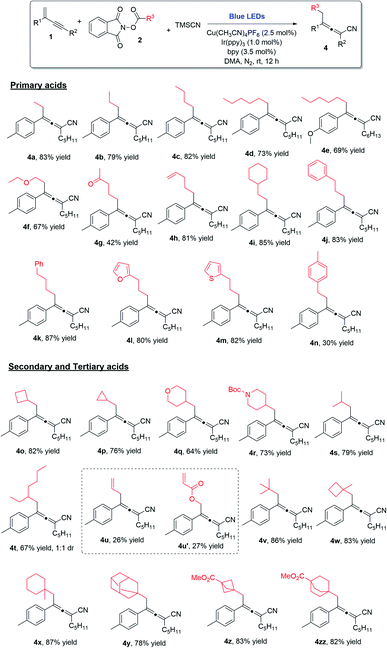 |
| Scheme 2 NHP ester scope. a Reaction conditions: 1 (0.2 mmol), 2 (0.2 mmol) and TMSCN (0.4 mmol) in DMA (1.0 mL), Cu(CH3CN)4PF6 (2.5 mol%), bpy (3.5 mol%), Ir(ppy)3 (1.0 mol%), at room temperature, 30 W blue LEDs, 12 h. Yields given refer to isolated yields. | |
This copper/photoredox dual catalytic strategy enables the synthesis of allenes at room temperature under redox-neutral conditions without requiring the utilization of external oxidizing reagents, thus providing a unique entry to access complex allene structures. We next explored late stage allenylation of natural products and drug molecules: from glycine (5a) to fatty acids, e.g. lauric acid (5b) and oleic acid (5c), and to drugs, e.g. mycophenolic acid (5d), gabapentin (5e), and chlorambucil (5f). Remarkably, all the allenylations via 1,4-difunctionalization of enynes proceeded in reasonably good yields, displaying impressive chemo- and regio-selectivities and excellent functional group compatibility (Scheme 3).
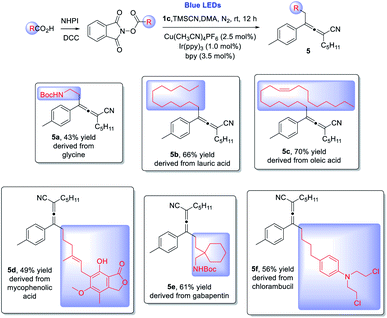 |
| Scheme 3 Late-stage functionalization of natural products and drugs. a Reaction conditions: 1c (0.2 mmol), 2 (0.2 mmol) and TMSCN (0.4 mmol) in DMA (1.0 mL), Cu(CH3CN)4PF6 (2.5 mol%), bpy (3.5 mol%), Ir(ppy)3 (1.0 mol%), at room temperature, 30 W blue LEDs, 12 h. Yields given refer to isolated yields. | |
Synthetic manipulations of the 1,4-carbocyanation allene products were demonstrated (Scheme 4). The reaction could be scaled up smoothly, virtually without compromising the chemical yield. The α-allenyl amine 6, an important skeleton found in a wide range of bioactive compounds,1 was readily prepared in 84% yield, through the reduction of allenenitrile 3c. Alternatively, when 3c was subjected to hydrolysis, allenamide 7 was obtained, and the subsequent cyclization of which led to highly functionalized lactam 8.22
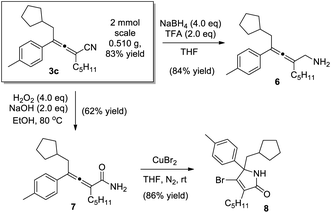 |
| Scheme 4 Synthetic manipulations of the allene product. | |
To gain mechanistic insights into the reaction, we conducted a few control experiments (Scheme 5). When one molar equivalence of the radical scavenger TEMPO was added, the decarboxylative 1,4-carbocyanation was completely inhibited, and the TEMPO-captured 9 was detected, confirming the presence of an alkyl radical species during the reaction (eqn (a)). Several radical clock studies were also performed. The reaction of NHP ester derived from cyclopropylacetic acid produced exclusively the ring-opening product 4h in 78% yield, strongly supporting that the reaction is a radical process. When substrates 11 and 13 were treated with NHP ester 2a, the corresponding allenes were obtained in moderate yields. No ring-opening product from 11 or ring-closing product from 13 was detected, suggesting that the coupling of the allenyl radical with the Cu(II) complex proceeds much faster.17b
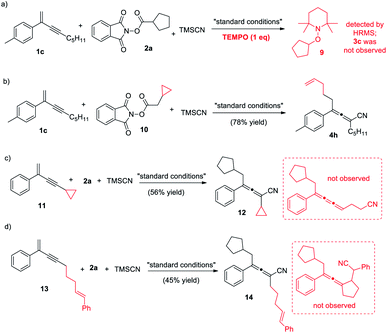 |
| Scheme 5 Control experiments. | |
On the basis of the above results as well as related literature reports,16,17 a plausible mechanism is proposed (Fig. 2). Excitation of photocatalyst Ir(ppy)3 leads to the active state (A) that undergoes single electron transfer with the NHP ester to produce the corresponding ester radical anion (C). The alkyl radical D is formed upon the extrusion of CO2. The subsequent addition of the alkyl radical to 1,3-enyne generates the propargyl radical, and the resonance form of which is an allenyl radical. The allenyl radical plays a key role in intercepting the copper(II) complex and creates a copper(III) species (E), which undergoes reductive elimination readily to furnish a tetra-substituted allene product, regenerating the copper catalyst at the same time. In this reaction cycle, the iridium photocatalyst is crucial in decarboxylative creation of the alkyl radical, as well as in facilitating the formation of copper(II) species.
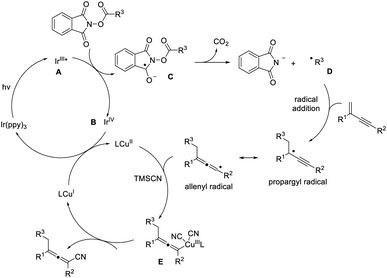 |
| Fig. 2 Proposed catalytic cycle. | |
Conclusions
In summary, we developed the first copper/photoredox dual catalysis enabled decarboxylative 1,4-carbocyanation of 1,3-enynes, for the synthesis of tetra-substituted allenes. The reaction is conducted under mild reaction conditions, displaying excellent chemoselectivity and regioselectivity, as well as remarkable functional group compatibility. The scope of the reaction is very broad, applicable to a wide range of primary, secondary, and tertiary NHP esters. Furthermore, the late-stage allenylation of natural products and drugs has also been demonstrated. We believe our reported method will have great conceptual implications, and may lead to the discovery of a range of general and versatile multi-functionalizations of 1,3-enynes and related compounds. We are currently working in these directions, and our findings will be reported in due course.
Data availability
All experimental procedures, characterization, copies of NMR spectra for all new compounds related to this article can be found in the ESI.†
Author contributions
Y. C. designed and performed the experiments, prepared the experimental part and the first draft of the manuscript. J. W. synthesized the NHP esters. Y. L. supervised the work, finalized the manuscript and coordinated the overall project.
Conflicts of interest
There are no conflicts to declare.
Acknowledgements
Y. L. thanks the Singapore National Research Foundation, Prime Minister's Office for the NRF Investigatorship Award (R-143-000-A15-281). Financial support from the National University of Singapore (C-141-000-092-001) is also gratefully acknowledged.
Notes and references
-
(a)
N. Krause and A. S. K. Hashimi, Modern Allene Chemistry, Wiley-VCH, Weinheim, 2004 CrossRef
;
(b) A. Hoffmann-Röder and N. Krause, Angew. Chem., Int. Ed., 2004, 43, 1196 CrossRef PubMed
;
(c) S. Ma, Chem. Rev., 2005, 105, 2829 CrossRef PubMed
;
(d) S. Ma, Acc. Chem. Res., 2009, 42, 1679 CrossRef CAS PubMed
;
(e) C. Aubert, L. Fensterbank, P. Garcia, M. Malacria and A. Simonneau, Chem. Rev., 2011, 111, 1954 CrossRef CAS PubMed
;
(f) S. Yu and S. Ma, Angew. Chem., Int. Ed., 2012, 51, 3074 CrossRef CAS PubMed
;
(g) J. Ye and S. Ma, Acc. Chem. Res., 2014, 47, 989 CrossRef CAS PubMed
.
- For selected reviews on the synthesis of allenes, see:
(a) K. M. Brummond and J. E. DeForrest, Synthesis, 2007, 6, 795 CrossRef
;
(b) J. Ye and S. Ma, Org. Chem. Front., 2014, 1, 1210 RSC
;
(c) S. Yu and S. Ma, Chem. Commun., 2011, 47, 5384 RSC
;
(d) W.-D. Chu, Y. Zhang and J. Wang, Catal. Sci. Technol., 2017, 7, 4570 RSC
;
(e) X. Huang and S. Ma, Acc. Chem. Res., 2019, 52, 1301 CAS
.
- For recent reviews, see:
(a) L. Fu, S. Greßies, P. Chen and G. Liu, Chin. J. Chem., 2020, 38, 91 CrossRef CAS
;
(b) Q. Dherbassy, S. Manna, F. J. T. Talbot, W. Prasitwatcharakorn, G. J. P. Perry and D. J. Procter, Chem. Sci., 2020, 11, 11380 RSC
.
- For selected examples based on organocatalysis:
(a) W. Zhang, H. Xu, H. Xu and W. Tang, J. Am. Chem. Soc., 2009, 131, 3832 CrossRef CAS PubMed
;
(b) W. Zhang, S. Zheng, N. Liu, J. B. Werness, L. A. Guzei and W. Tang, J. Am. Chem. Soc., 2010, 132, 3664 CrossRef CAS PubMed
;
(c) H. Qian, X. Yu, J. Zhang and J. Sun, J. Am. Chem. Soc., 2013, 135, 18020 CrossRef CAS PubMed
;
(d) H.-Y. Wang, W. Zhang, C. M. Schienebeck, S. R. Bennett and W. Tang, Org. Chem. Front., 2014, 1, 386 RSC
;
(e) P. H. Poulsen, Y. Li, V. H. Lauridsen, D. K. B. Jørgensen, T. A. Palazzo, M. Meazza and K. A. Jørgensen, Angew. Chem., Int. Ed., 2018, 57, 10661 CrossRef CAS PubMed
.
- For selected examples based on organometallic reagents:
(a) H. Todo, J. Terao, H. Watanabe, H. Kuniyasu and N. Kambe, Chem. Commun., 2008, 1332 RSC
;
(b) J. Zhao, Y. Liu, Q. He, Y. Li and S. Ma, Chem. - Eur. J., 2009, 15, 11361 CrossRef CAS PubMed
;
(c) Y. Tomida, A. Nagaki and J. Yoshida, J. Am. Chem. Soc., 2011, 133, 3744 CrossRef CAS PubMed
;
(d) Y. Mori, G. Onodera and M. Kimura, Chem. Lett., 2014, 43, 97 CrossRef CAS
;
(e) B. Wang, Y. Li, J. H. Pang, K. Watanabe, R. Takita and S. Chiba, Angew. Chem., Int. Ed., 2021, 60, 217 CrossRef CAS PubMed
.
- For selected examples based on transition-metal catalysis:
(a) Y. Matsumoto, M. Naito, Y. Uozumi and T. Hayashi, J. Chem. Soc., Chem. Commun., 1993, 19, 1468 RSC
;
(b) J. W. Han, N. Tokunaga and T. Hayashi, J. Am. Chem. Soc., 2001, 123, 12915 CrossRef CAS PubMed
;
(c) M. Wang, Z.-L. Liu, X. Zhang, P.-P. Tian, Y.-H. Xu and T.-P. Loh, J. Am. Chem. Soc., 2015, 137, 14830 CrossRef CAS PubMed
;
(d) Y. Huang, J. D. Pozo, S. Torker and A. H. Hoveyda, J. Am. Chem. Soc., 2018, 140, 2643 CrossRef CAS PubMed
;
(e) H. L. Sang, S. Yu and S. Ge, Org. Chem. Front., 2018, 5, 1284 RSC
;
(f) D.-W. Gao, Y. Xiao, M. Liu, Z. Liu, M. K. Karunananda, J. S. Chen and K. M. Engle, ACS Catal., 2018, 8, 3650 CrossRef CAS PubMed
;
(g) S. Yu, H. L. Sang, S.-Q. Zhang, X. Hong and S. Ge, Commun. Chem., 2018, 1, 64 CrossRef
;
(h) L. Bayeh-Romero and S. L. Buchwald, J. Am. Chem. Soc., 2019, 141, 13788 CrossRef CAS PubMed
;
(i) N. J. Adamson, H. Jeddi and S. J. Malcolmson, J. Am. Chem. Soc., 2019, 141, 8574 CrossRef CAS PubMed
;
(j) Y. Zhang, B. Yu, B. Gao, T. Zhang and H. Huang, Org. Lett., 2019, 21, 535 CrossRef CAS PubMed
;
(k) C.-Y. He, Y.-X. Tan, X. Wang, R. Ding, Y.-F. Wang, F. Wang, D. Gao, P. Tian and G.-Q. Lin, Nat. Commun., 2020, 11, 4293 CrossRef CAS PubMed
;
(l) Y. Liao, X. Yin, X. Wang, W. Yu, D. Fang, L. Hu, M. Wang and J. Liao, Angew. Chem., Int. Ed., 2020, 59, 1176 CrossRef CAS PubMed
.
- For selected reviews, see:
(a) J. Twilton, C. Le, P. Zhang, M. H. Shaw, R. W. Evans and D. W. C. MacMillan, Nat. Rev. Chem., 2017, 1, 0052 CrossRef CAS
;
(b) D. C. Fabry and M. Rueping, Acc. Chem. Res., 2016, 49, 1969 CrossRef CAS PubMed
;
(c) M. N. Hopkinson, A. Tlahuext-Aca and F. Glorius, Acc. Chem. Res., 2016, 49, 2261 CrossRef CAS PubMed
;
(d) J. A. Milligan, J. P. Phelan, S. O. Badir and G. A. Molander, Angew. Chem., Int. Ed., 2019, 58, 6152 CrossRef CAS PubMed
;
(e) A. Hossain, A. Bhattacharyya and O. Reiser, Science, 2019, 364, 450 CrossRef PubMed
;
(f) A. Lipp, S. O. Badir and G. A. Molander, Angew. Chem., Int. Ed., 2021, 60, 1714 CrossRef CAS PubMed
;
(g) K. L. Skubi, T. R. Blum and T. P. Yoon, Chem. Rev., 2016, 116, 10035 CrossRef CAS PubMed
;
(h) Y. Chen, L.-Q. Lu, D.-G. Yu, C.-J. Zhu and W.-J. Xiao, Sci. China: Chem., 2019, 62, 24 CrossRef CAS
;
(i) H.-H. Zhang, H. Chen, C. Zhu and S. Yu, Sci. China: Chem., 2020, 63, 637 CrossRef CAS
;
(j) W. Zhou, Y. Jiang, L. Chen, K. Liu and D.-G. Yu, Chin. J. Org. Chem., 2020, 40, 3697 CrossRef
;
(k) M. Zhou, P. Qin, L. Jing, J. Sun and H. Du, Chin. J. Org. Chem., 2020, 40, 598 CrossRef
.
-
(a) S. O. Badir and G. A. Molander, Chem, 2020, 6, 1327 CrossRef CAS PubMed
;
(b) C. Zhu, H. Yue, L. Chu and M. Rueping, Chem. Sci., 2020, 11, 4051 RSC
.
- For selected examples on difunctionalization of alkenes, see:
(a) W. Sha, L. Deng, S. Ni, H. Mei, J. Han and Y. Pan, ACS Catal., 2018, 8, 7489 CrossRef CAS
;
(b) J. Chen, B.-Q. He, P.-Z. Wang, X.-Y. Yu, Q.-Q. Zhao, J.-R. Chen and W.-J. Xiao, Org. Lett., 2019, 21, 4359 CrossRef CAS PubMed
;
(c) X.-H. Ouyang, Y. Li, R.-J. Song, M. Hu, S. Luo and J.-H. Li, Sci. Adv., 2019, 5, eaav9839 CrossRef CAS PubMed
;
(d) L. Guo, H.-Y. Tu, S. Zhu and L. Chu, Org. Lett., 2019, 21, 4771 CrossRef CAS PubMed
;
(e) A. García-Domínguez, R. Mondal and C. Nevado, Angew. Chem., Int. Ed., 2019, 58, 12286 CrossRef PubMed
;
(f) M. W. Campbell, J. S. Compton, C. B. Kelly and G. A. Molander, J. Am. Chem. Soc., 2019, 141, 20069 CrossRef CAS PubMed
;
(g) R. S. Mega, V. K. Duong, A. Noble and V. K. Aggarwal, Angew. Chem., Int. Ed., 2020, 59, 4375 CrossRef CAS PubMed
;
(h) S.-Z. Sun, Y. Duan, R. S. Mega, R. J. Somerville and R. Martin, Angew. Chem., Int. Ed., 2020, 59, 4370 CrossRef CAS PubMed
;
(i) Z. Zhang and X. Hu, ACS Catal., 2020, 10, 777 CrossRef CAS
;
(j) L. Huang, C. Zhu, L. Yi, H. Yue, R. Kancherla and M. Rueping, Angew. Chem., Int. Ed., 2020, 59, 457 CrossRef CAS PubMed
;
(k) S. Zheng, Z. Chen, Y. Hu, X. Xi, Z. Liao, W. Li and W. Yuan, Angew. Chem., Int. Ed., 2020, 59, 17910 CrossRef CAS PubMed
;
(l) Z. Chen, F. Lu, F. Yuan, J. Sun, L. Du, Z. Li, M. Gao, R. Shi and A. Lei, Sci. China: Chem., 2019, 62, 1497 CrossRef CAS
;
(m) S. Xu, H. Chen, Z. Zhou and W. Kong, Angew. Chem., Int. Ed., 2021, 60, 7405 CrossRef CAS PubMed
.
- For selected examples on difunctionalization of alkynes, see:
(a) A. Tlahuext-Aca, M. N. Hopkinson, R. A. Garza-Sanchez and F. Glorius, Chem.–Eur. J., 2016, 22, 5909 CrossRef CAS PubMed
;
(b) L. Guo, F. Song, S. Zhu, H. Li and L. Chu, Nat. Commun., 2018, 9, 4543 CrossRef PubMed
;
(c) C. Zhu, H. Yue, B. Maity, I. Atodiresei, L. Cavallo and M. Rueping, Nat. Catal., 2019, 2, 678 CrossRef CAS
;
(d) H. Yue, C. Zhu, R. Kancherla, F. Liu and M. Rueping, Angew. Chem., Int. Ed., 2020, 59, 5738 CrossRef CAS PubMed
.
- H.-M. Huang, P. Bellotti, C. G. Daniliuc and F. Glorius, Angew. Chem., Int. Ed., 2021, 60, 2464 CrossRef CAS PubMed
.
-
(a) D. H. Volman, K. A. Maas and J. Wolstenholme, J. Am. Chem. Soc., 1965, 87, 3041 CrossRef CAS
;
(b) W. Adam and C. M. Ortega-Schulte, J. Org. Chem., 2003, 68, 1007 CrossRef CAS PubMed
;
(c) J. Maury, S. Jammi, F. Vibert, S. R. A. Marque, D. Siri, L. Feray and M. Bertrand, J. Org. Chem., 2012, 77, 9081 CrossRef CAS PubMed
.
- L. Liu, R. M. Ward and J. M. Schomaker, Chem. Rev., 2019, 119, 12422 CrossRef CAS PubMed
.
- For selected examples, see:
(a) T. Taniguchi, Y. Sugiura, H. Zaimoku and H. Ishibashi, Angew. Chem., Int. Ed., 2010, 49, 10154 CrossRef CAS PubMed
;
(b) H. Zhang, Y. Song, J. Zhao, J. Zhang and Q. Zhang, Angew. Chem., Int. Ed., 2014, 53, 11079 CrossRef CAS PubMed
;
(c) D.-F. Lu, C.-L. Zhu, Z.-X. Jia and H. Xu, J. Am. Chem. Soc., 2014, 136, 13186 CrossRef CAS PubMed
;
(d) R. Zhu and S. L. Buchwald, J. Am. Chem. Soc., 2015, 137, 8069 CrossRef CAS PubMed
;
(e) X. Sun, X. Li, S. Song, Y. Zhu, Y.-F. Liang and N. Jiao, J. Am. Chem. Soc., 2015, 137, 6059 CrossRef CAS PubMed
;
(f) J.-K. Cheng and T.-P. Loh, J. Am. Chem. Soc., 2015, 137, 42 CrossRef CAS PubMed
;
(g) M. R. Miner and K. A. Woerpel, Eur. J. Org. Chem., 2016, 10, 1860 CrossRef
.
-
(a) M. L. Poutsma and P. A. Ibarbia, J. Org. Chem., 1970, 35, 4038 CrossRef
;
(b) A. E. Soueni, J. M. Tedder and J. C. Walton, J. Fluorine Chem., 1981, 17, 51 CrossRef
;
(c) C. Alameda-Angulo, B. Quiclet-Sire and S. Z. Zard, Tetrahedron Lett., 2006, 47, 913 CrossRef CAS
;
(d) J. Terao, F. Bando and N. Kambe, Chem. Commun., 2009, 7336 RSC
;
(e) K.-F. Zhang, K.-J. Bian, C. Li, J. Sheng, Y. Li and X.-S. Wang, Angew. Chem., Int. Ed., 2019, 58, 5069 CrossRef CAS PubMed
;
(f) Y. Song, S. Song, X. Duan, X. Wu, F. Jiang, Y. Zhang, J. Fan, X. Huang, C. Fu and S. Ma, Chem. Commun., 2019, 55, 11774 RSC
;
(g) H. Shen, H. Xiao, L. Zhu and C. Li, Synlett, 2020, 31, 41 CrossRef CAS
;
(h) X.-Y. Dong, T.-Y. Zhan, S.-P. Jiang, X.-D. Liu, L. Ye, Z.-L. Li, Q.-S. Gu and X.-Y. Liu, Angew. Chem., Int. Ed., 2021, 60, 2160 CrossRef CAS PubMed
.
- F. Wang, D. Wang, Y. Zhou, L. Liang, R. Lu, P. Chen, Z. Lin and G. Liu, Angew. Chem., Int. Ed., 2018, 57, 7140 CrossRef CAS PubMed
.
-
(a) X. Zhu, W. Deng, M.-F. Chiou, C. Ye, W. Jian, Y. Zeng, Y. Jiao, L. Ge, Y. Li, X. Zhang and H. Bao, J. Am. Chem. Soc., 2019, 141, 548 CrossRef CAS PubMed
;
(b) C. Ye, Y. Li, X. Zhu, S. Hu, D. Yuan and H. Bao, Chem. Sci., 2019, 10, 3632 RSC
;
(c) Y. Zeng, M.-F. Chiou, X. Zhu, J. Cao, D. Lv, W. Jian, Y. Li, X. Zhang and H. Bao, J. Am. Chem. Soc., 2020, 142, 18014 CrossRef CAS PubMed
.
- For selected reviews, see:
(a) J. Xuan, Z.-G. Zhang and W.-J. Xiao, Angew. Chem., Int. Ed., 2015, 54, 15632 CrossRef CAS PubMed
;
(b) S. Murarka, Adv. Synth. Catal., 2018, 360, 1735 CrossRef CAS
.
- For selected reviews, see:
(a) F. Wang, P. Chen and G. Liu, Acc. Chem. Res., 2018, 51, 2036 CrossRef CAS PubMed
;
(b) Q.-S. Gu, Z.-L. Li and X.-Y. Liu, Acc. Chem. Res., 2020, 53, 170 CrossRef CAS PubMed
;
(c) Z.-L. Li, G.-C. Fang, Q.-S. Gu and X.-Y. Liu, Chem. Soc. Rev., 2020, 49, 32 RSC
; For selected examples, see:;
(d) W. Zhang, F. Wang, S. D. McCann, D. Wang, P. Chen, S. S. Stahl and G. Liu, Science, 2016, 353, 1014 CrossRef CAS PubMed
;
(e) J. Li, Z. Zhang, L. Wu, W. Zhang, P. Chen, Z. Lin and G. Liu, Nature, 2019, 574, 516 CrossRef CAS PubMed
;
(f) X.-Y. Dong, Y.-F. Zhang, C.-L. Ma, Q.-S. Gu, F.-L. Wang, S.-P. Jiang and X.-Y. Liu, Nat. Chem., 2019, 11, 1158 CrossRef CAS PubMed
;
(g) H.-D. Xia, Z.-L. Li, Q.-S. Gu, X.-Y. Dong, J.-H. Fang, X.-Y. Du, L.-L. Wang and X.-Y. Liu, Angew. Chem., Int. Ed., 2020, 59, 16926 CrossRef CAS PubMed
.
- B. Zhao and Z. Shi, Angew. Chem., Int. Ed., 2017, 56, 12727 CrossRef CAS PubMed
.
- D. Wang, N. Zhu, P. Chen, Z. Lin and G. Liu, J. Am. Chem. Soc., 2017, 139, 15632 CrossRef CAS PubMed
.
- Y.-F. Ao, D.-X. Wang, L. Zhao and M.-X. Wang, J. Org. Chem., 2014, 79, 3103 CrossRef CAS PubMed
.
Footnote |
† Electronic supplementary information (ESI) available. CCDC 2034197. For ESI and crystallographic data in CIF or other electronic format see DOI: 10.1039/d1sc02896k |
|
This journal is © The Royal Society of Chemistry 2021 |
Click here to see how this site uses Cookies. View our privacy policy here.