Development of lacto-series ganglioside fluorescent probe using late-stage sialylation and behavior analysis with single-molecule imaging†
Received
28th March 2022
, Accepted 28th May 2022
First published on 31st May 2022
Abstract
Gangliosides are a family of sialic-acid-containing glycosphingolipids that form dynamic domains (lipid rafts) with proteins in cell plasma membranes (PMs), and are involved in various biological processes. The dynamic behavior of gangliosides can be elucidated by analyzing fluorescently-labeled molecules with a powerful technique known as single-molecule imaging. We previously developed fluorescent probes for ganglioside subfamilies such as the ganglio- and globo-series, and investigated their behavior in cell PMs. This study targeted a lacto-series ganglioside, sialyl-lactotetraosylceramide, whose behavior in PMs has not yet been investigated. We applied a recently reported method for the direct sialylation of oligosaccharyl lipid acceptors to synthesize the fluorescent ganglioside probes. The glycolipid acceptor exhibited high solubility in organic solvents owing to the installation of a large quantity of p-tert-butylbenzoyl protecting groups, which ensured direct α-sialylation at relatively low temperatures. Biophysical evaluation of the synthesized probe determined that it behaved as a raft molecule in cell PMs. Furthermore, single-molecule imaging revealed cis interactions between the lacto-series ganglioside and a major raft molecule (GPI-anchored protein CD59). Moreover, the fluorescent non-sialylated (asialyl) lactotetraosylceramide behaved similarly to its sialyl counterpart.
Introduction
Glycosphingolipids containing one or more sialic acid (Neu) residue(s) in their glycan structure are known as gangliosides. There are more than 100 different gangliosides, which are classified into several series (ganglio-, globo-, lacto-, and neolacto-) based on the types of sugar constituting the glycan moieties and their linkage forms. Each ganglioside has different biological functions in cell plasma membranes (PMs). These functions are closely associated with their specific interactions with proteins.1 The interactions are roughly divided into vertical interactions between other cells (trans interactions) and lateral interactions within the same PMs (cis interactions). The details of trans interactions have been elucidated by X-ray crystallography and NMR spectroscopy of ganglioside–protein complexes.2,3 However, little is known about cis interactions, because it is difficult to observe the dynamics of ganglioside transient interactions in cell PMs. A powerful solution for overcoming this lack of knowledge is single-molecule imaging of fluorescently-labeled membrane molecules. This technique enables observation of ganglioside dynamics in living cell PMs at the individual molecule level.4 In previous research, fluorescent ganglio-5–7 and globo-series8,9 ganglioside probes were chemically synthesized, which exhibited properties similar to those of natural gangliosides. We used single-molecule imaging in living cells to investigate the behavior of these probes, as well as their cis interactions with raft-associated membrane proteins, such as GPI-anchored proteins.5 Single-molecule observations of gangliosides GM3 and GM1 revealed that they frequently interact with transient dimers and stable oligomers of a GPI-anchored protein (CD59) to form lipid rafts.10 For a comprehensive understanding of the cis interactions of gangliosides, the dynamics of each ganglioside series should be analyzed by single-molecule tracking of their fluorescent probes.
In this study, we focused on lacto-series gangliosides, for which fluorescent probes have never been developed. Lacto-series gangliosides contain a core glycan structure [Galβ(1→3)GlcNAcβ(1→3)Galβ(1→4)Glc] and are known to be involved in several serious diseases, such as human gliomas, lung and digestive system cancers.11–16 Lipid rafts consisting of lacto-series gangliosides are thought to be related to these diseases in a manner similar to that of other gangliosides;17 however, the details are unknown. This was our motivation for developing fluorescent probes for lacto-series gangliosides. We targeted sialyl-lactotetraosylceramide (NeuAcLc4Cer), which is one of the simplest lacto-series gangliosides, and lactotetraosylceramide (Lc4Cer), as the asialo counterpart of NeuAcLc4Cer. Probes were designed using a fluorescent dye at the glycan terminal (Fig. 1). Fluorescent ganglioside probe 1 was efficiently synthesized via the direct sialylation of a glycolipid, a method that we recently reported.18 We evaluated their functions in cell PMs, then observed their interactions with the major GPI-anchored protein (CD59) by single-molecule tracking.
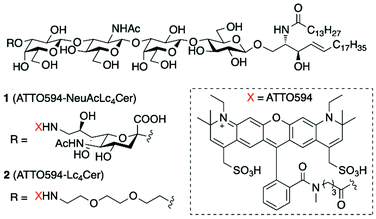 |
| Fig. 1 Structures of fluorescent glycosphingolipids NeuAcLc4Cer 1 and Lc4Cer 2. Abbreviations: sialyl-lactotetraosylceramide (NeuAcLc4Cer), lactotetraosylceramide (Lc4Cer), acetyl (Ac). | |
Results and discussion
In our previous research, we found that the behavior of ganglioside probes in cell PMs was significantly affected by the loading position and physiochemical properties of the fluorescent dyes.5 The results indicated that, to retain the raft molecule properties, the glycan terminal hydroxyl should be labeled with a hydrophilic dye. Based on this work, we designed ATTO594-NeuAcLc4Cer 1 and ATTO594-Lc4Cer 2 as target molecules (Fig. 1).
The retrosynthetic analysis of fluorescent NeuAcLc4Cer 1 is shown in Scheme 1. A chemically unstable fluorescent dye was introduced in the final step of the synthesis. The dye was incorporated via amide linkage by replacing the sialic acid C9-OH group with an amino group.
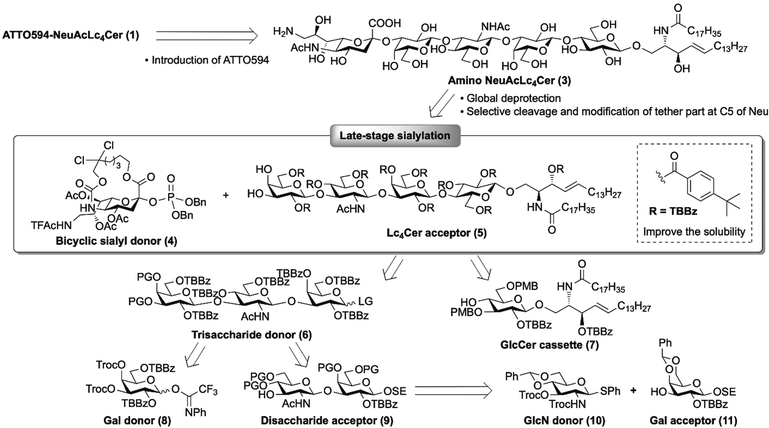 |
| Scheme 1 Retrosynthetic analysis of fluorescent NeuLc4Cer. Abbreviations: trifluoroacetyl (TFAc), benzyl (Bn), p-tert-butylbenzoyl (TBBz), protecting group (PG), leaving group (LG), 2,2,2-trichloroethoxycarbonyl (Troc), phenyl (Ph), 2-(trimethylsilyl)ethyl (SE), p-methoxybenzyl (PMB). | |
The synthesis of amino NeuAcLc4Cer 3 is challenging, because the special structural features of sialic acids make it difficult to form the Neuα(2→3)Gal linkage by α-sialylation. In glycosylation, the sialic-acid oxocarbenium intermediate is destabilized by the C1 carboxyl group and readily converts into 2,3-ene byproduct via 1,2-elimination. Furthermore, neighboring group participation is not available because of the deoxy structure at the C3 position, resulting in the preferential formation of thermodynamically stable β-glycosides in sialylations. The purification of stereoisomeric mixtures of sialic acid glycosides is problematic, particularly in the case of oligosaccharides. Hence, in conventional ganglioside synthesis, α-sialylation is initially conducted with a monosaccharide acceptor to control the stereoselectivity and isolate the α-isomer from the stereo mixtures. In our previous work, we synthesized various natural gangliosides and C9-modified fluorescent probes using N-Troc Neuα(2→3)Gal19,20 as a common unit suitable for large scale syntheses.5,21,22 However, different multistep processes are required for each synthesis, including glycan extension and lipid introduction after the Neuα(2→3)Gal linkage is constructed. This is especially true for C9-labeled probes, where additional reaction steps are required to modify the sialic acid moiety in NeuGal. Recently, we developed a fully stereoselective sialylation method using a macrobicyclic sialic acid donor23 in which the C1 and C5 positions are tethered by an alkyl chain. With this bicyclic sialyl donor, we achieved the efficient formation of C5-modified ganglioside GM3 analogs via sialylation of the glycolipid (LacCer) at a late stage of the synthesis.18 In this study, we expanded this novel approach to the synthesis of NeuAcLc4Cer probes with a larger glycan structure.
To afford amino NeuAcLc4Cer 3, we designed bicyclic sialyl donor 4, where C9-OH is replaced by the NHTFAc group, with the intention of later converting it into an NH2 group by global deprotection.8 As a coupling partner of 4, we envisioned the Lc4Cer acceptor 5 with p-tert-butylbenzoyl (TBBz) groups,24 which would improve the solubility of the glycolipids at low temperatures. Furthermore, a 3,4-diol of the non-reducing terminal Gal was designed to increase C3-OH reactivity. Following our synthetic method for glycolipid skeletons, the Lc4Cer skeleton would be constructed from trisaccharide donor 6 and GlcCer cassette 7.23,25 The protecting groups at the C3 and C4 positions of trisaccharide donor 6 were designed to be selectively removed, before it was transformed into Lc4Cer acceptor 5 after coupling with the GlcCer cassette. The C2-OH group of the non-reducing end Gal was protected by the TBBz group for β-linkage formation. The trisaccharide framework was expected to be obtained by glycosidation of Gal donor 818 and GlcNAcGal acceptor 9. Disaccharide 9 was fragmented into GlcN donor 1026 and Gal acceptor 11. The C2 amino group of GlcN donor 10 was protected with a Troc group to form a β-glycoside linkage. A similar synthetic method was designed for ATTO594-Lc4Cer 2 (see ESI†).
The disaccharide acceptor 16 was synthesized as follows (Scheme 2). Gal acceptor 11 was prepared from 1227 in two steps. First, 12 was treated with TBBzCl in CH2Cl2/pyridine to protect the hydroxyl group at the C2 position with a TBBz group and produce 13. Second, removal of the Troc group of 13 using zinc powder in AcOH/MeCN yielded Gal acceptor 11. Equimolar amounts of Gal acceptor 11 and GlcN donor 1026 were then glycosylated in CH2Cl2 at −20 °C using N-iodosuccinimide (NIS) and trifluoromethanesulfonic acid (TfOH) as glycosylation promoters to provide disaccharide 14. Then, two Troc groups in GlcN residues were cleaved using a protocol similar to that used for Gal 13. Finally, the C2-amino group of 15 was selectively acetylated by treatment with Ac2O in CH2Cl2–MeOH to afford the disaccharide acceptor 16 in 99% yield.
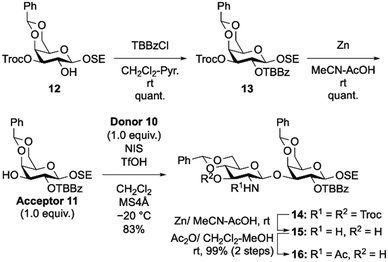 |
| Scheme 2 Synthesis of disaccharide acceptor 16. Abbreviations: pyridine (Pyr.), N-iodosuccinimide (NIS), trifluoromethanesulfonic acid (TfOH), molecular sieves (MS). | |
Next, we synthesized the Lc4Cer acceptor (Schemes 3 and 4). Disaccharide acceptor 16 and previously reported Gal donor 818 were glycosylated in the presence of trimethylsilyl trifluoromethanesulfonate (TMSOTf) in CH2Cl2 at 0 °C to generate trisaccharide framework 17 in 78% yield. Tetraol 18 was obtained by acid hydrolysis of the benzylidene groups of 17. Subsequently, TBBz groups were introduced into the free hydroxyl groups by treatment with TBBzCl and 4-dimethylaminopyridine (DMAP). After cleavage of the 2-(trimethylsilyl)ethyl group, an N-phenyltrifluoroacetimidoyl group was installed at the reducing end, resulting in trisaccharide donor 21.
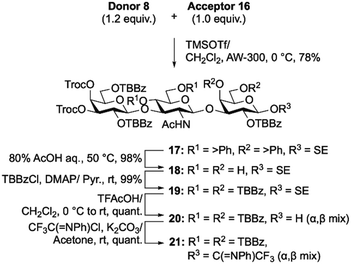 |
| Scheme 3 Synthesis of trisaccharide donor 21. Abbreviations: trimethylsilyl trifluoromethanesulfonate (TMSOTf), acid washed molecular sieves (AW), 4-dimethylaminopyridine (DMAP). | |
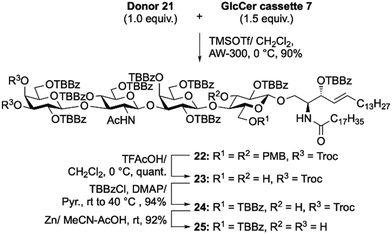 |
| Scheme 4 Synthesis of Lc4Cer acceptor 25. | |
Next, Lc4Cer skeleton 22 was afforded in excellent yield (90%) after glycosidation of trisaccharide donor 21 with GlcCer cassette 7 was promoted by TMSOTf in CH2Cl2 at 0 °C. Lc4Cer skeleton 22 was then converted into an acceptor. First, the p-methoxybenzyl groups were removed by acid hydrolysis, forming 3,6-diol 23. Subsequently, we attempted to introduce TBBz groups into the C3 and C6 hydroxyl groups of glucose in compound 23. However, the TBBz group was only inserted into the C6 hydroxyl group, which had less steric hindrance, yielding 24. Finally, the Troc groups were selectively removed by treatment with zinc powder in AcOH/MeCN to produce the Lc4Cer acceptor 25 in 92% yield. As expected, Lc4Cer acceptor 25, bearing TBBz groups, was highly soluble in CH2Cl2. We confirmed that it was completely soluble in CH2Cl2 at −70 °C.
We then focused on preparing 9-NHTFAc bicyclic sialic acid donor 4 (Scheme 5). In this study, we decreased the number of reaction steps required to go from 26 to 36, compared to the method that was previously reported.8 In the previous approach, the C1 carboxylic acid and terminal hydroxyl group of the tether moiety were macrocyclized by means of the Mitsunobu reaction. Thus, the hydroxyl groups of sialic acid were protected before macrocyclization through a multistep manipulation. In this study, we attempted simple intramolecular alkylation of the C-1 carboxylate anion with the terminal alkyl bromide moiety of the linker in the presence of free hydroxyl groups. First, we synthesized cyclization precursor 31, which carries Br at the terminal of the linker. Sialic acid derivative 26 was then transformed into compound 27via saponification of the Ac group and methyl ester. On the other hand, the tether moiety was prepared from commercially available 28 in two steps. Compound 28 was treated with methyl dichloroacetate and NaH in DMF at 0 °C to obtain 29. Subsequently, compound 29 was reacted with NaBH4 in a THF/MeOH solvent mixture to reduce the ester, giving tether moiety 30 bearing Br at the terminal. Next, N-succinimidyl carbonate was prepared from compound 30 in the presence of di(N-succinimidyl)carbonate (DSC) and triethylamine in MeCN, which was then reacted with the amino group of compound 27 to afford the cyclization precursor 31 in 83% yield (over two steps, from 26). Next, we evaluated the intramolecular cyclization reaction. After various examinations, we found that the use of K2CO3 and Drierite (CaSO4) in DMF–THF (3
:
1) provided macrobicyclic sialic acid 31 in good yield (57%).
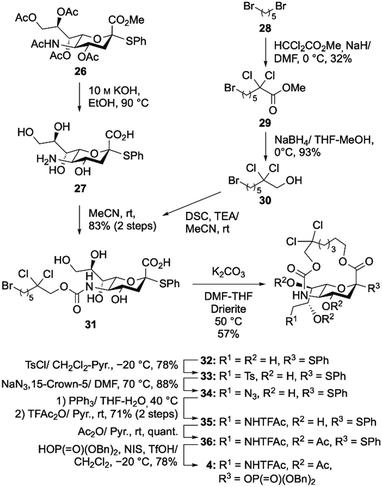 |
| Scheme 5 Synthesis of 9-NHTFAc bicyclic sialic acid donor 4. Abbreviations: di(N-succinimidyl)carbonate (DSC), triethylamine (TEA), p-toluenesulfonyl (Ts). | |
Next, selective tosylation of the C9 hydroxyl and subsequent azidation gave compound 34 in good yield. Compound 34 was treated with PPh3 in THF–H2O at 40 °C to reduce the azide group to an amino group, which was then reacted with TFAc2O to produce 9-NHTFAc bicyclic sialic acid 35. The hydroxyl groups were acetylated to form 36.8 We eliminated 3 reaction steps in the synthesis of 36 from 26. Our method required 8 steps (total yield 23%), while the previous method required 11 steps (total yield 34%). Finally, dibenzyl phosphate was introduced into the C2 position as a leaving group using NIS and TfOH in CH2Cl2 at −20 °C, resulting in donor 4.
With sialyl donor 4 and tetrasaccharyl lipid acceptor 25 successfully in hand, we examined direct sialylation to assemble the NeuLc4Cer framework (Table 1). This reaction requires low temperatures to suppress the 2,3-ene byproduct, according to our previous research.23 Therefore, the reaction was performed at the lowest possible temperature (−70 °C) to dissolve Lc4Cer acceptor 25 in CH2Cl2. It is worth noting that molecular sieves were not used, because we found that they promote aggregation of Lc4Cer acceptor 5.18 First, Lc4Cer acceptor 25 was reacted with 3.0 equiv. of donor 4 in the presence of TMSOTf in CH2Cl2 at −70 °C (entry 1). The reaction provided the NeuLc4Cer framework 37 in good yield (67%). In this reaction, Lc4Cer acceptor 25 was recovered (∼ 24%) because the donor degraded into the 2,3-ene byproduct. For entry 2, we used 4.5 equiv. of the donor to maximize the glycosylation yield, which successfully afforded NeuLc4Cer framework 37 in 82% yield. Next, C5-selective ring opening of NeuLc4Cer derivative 37 was carried out by zinc and acetic acid treatment under microwave irradiation at 40 °C.18 The C5 amino group was then sequentially reacted with Ac2O and DMAP in pyridine to deliver compound 38 in 69% yield over two steps. Next, the tether moiety and all acyl groups (TBBz, Ac, and TFAc) were removed using 1 M aq. NaOH in THF–MeOH to provide the amino derivative 3 in 99% yield. Finally, ATTO594 N-succinimidyl ester was combined with the C9-amino group in the presence of triethylamine in DMF–H2O to synthesize the target ATTO594-NeuAcLc4Cer 1 in 76% yield. These results suggest that our new synthetic approach involving late-stage sialylation of glycolipids is useful for the efficient synthesis of ganglioside analogs.
Table 1 Examination of direct sialylation
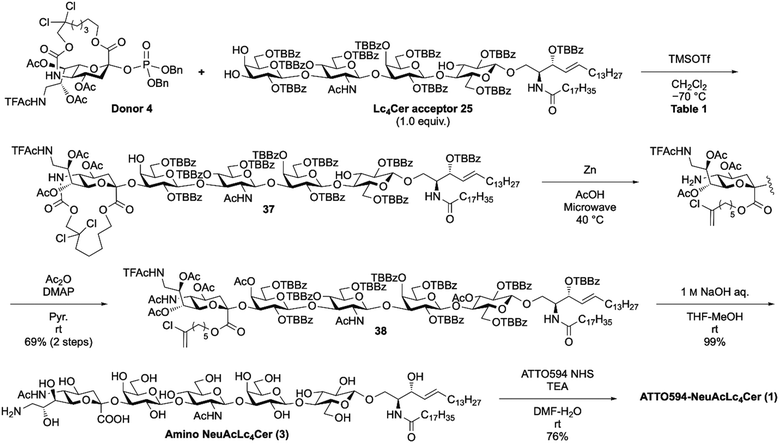
|
Entry |
Donor 4 (equiv.) |
Unreacted acceptor 25 |
Yield |
1 |
3.0 |
ca. 24% |
67% |
2 |
4.5 |
13% |
82% |
The synthesized ATTO594-NeuAcLc4Cer 1 and ATTO594-Lc4Cer 2 probes were then subjected to two different functional tests to examine their raft-philic properties in cell PMs (Fig. 2 and 3). First, we evaluated the partitioning of probes into detergent-resistant membrane (DRM) fractions. Raft molecules are not solubilized by treatment with cold non-ionic detergent, which means that they are partitioned into the DRM fraction.28 This phenomenon occurs because they are retained in the cell PMs by their lipid interactions. Fluorescent probes were incorporated into T24-cell PMs and treated with a cold detergent, 1% Triton X-100. The DRM fractions before and after treatment were observed by epifluorescence microscopy (Fig. 2). The results showed that both probes partitioned into the DRM fraction, thereby exhibiting raft molecule properties.
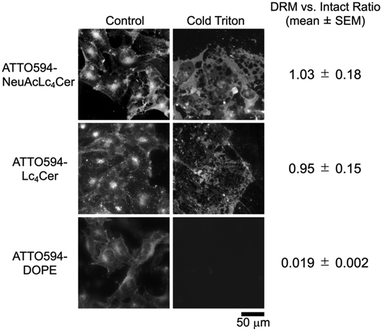 |
| Fig. 2 Detergent insolubility of ATTO594-NeuAcLc4Cer and ATTO594-Lc4Cer. Fluorescent images of ATTO594-NeuAcLc4Cer and ATTO594-Lc4Cer in T24 cell plasma membranes (PMs) before (left) and after (right) 1% cold Triton X-100 treatment. The ratio of fluorescence intensity of probes in detergent-resistant membrane (DRM) to intact cell PMs is presented at right. Number of observed cells before and after 1% cold Triton X-100 treatment were 12 and 11 for ATTO594-NeuAcLc4Cer, 16 and 12 for ATTO594-Lc4Cer, and 15 and 12 for ATTO594-DOPE. | |
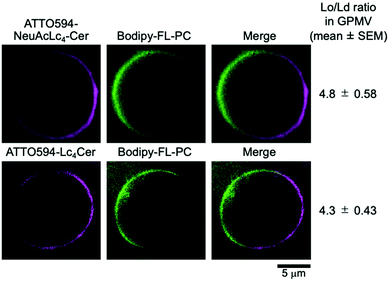 |
| Fig. 3 Partitioning of ATTO594-NeuAcLc4Cer. ATTO594-Lc4Cer and Bodipy-FL-PC into liquid-ordered (Lo)- and liquid-disordered (Ld)-like domains in giant plasma membrane vesicles (GPMVs) of RBL-2H3 cells at 10 °C. Representative still fluorescence images from simultaneous dual-color video sequence. ATTO594–NeuLc4AcCer and ATTO594-Lc4Cer are shown in magenta, and Bodipy-FL-PC, a Ld-like domain marker, is shown in green. The partitioning ratios are shown at right. Numbers of examined GPMVs were 16 for ATTO594-NeuAcLc4Cer and 13 for ATTO594-Lc4Cer. | |
Next, we tested the partitioning of the probes into liquid-ordered (Lo)-like/liquid-disordered (Ld)-like phases in giant plasma membrane vesicles (GPMVs), which contain all the lipid and protein components of native PMs, except for the actin-based membrane skeleton.29 Bodipy-FL-PC was used to mark the Ld-like domains.30 Both ATTO594-NeuAcLc4Cer 1 and ATTO594-Lc4Cer 2 were partitioned into the Lo-like phase, indicating that they retained their raft-philic properties (Fig. 3).
Finally, following a previously reported method,5 we performed simultaneous dual-color (two-molecular species) single-molecule tracking of the cell PMs (Fig. 4a–c). In steady-state cell PMs, the major GPI-anchored protein CD59 formed transient homodimers with a mean lifetime of approximately 160 ms, stabilized by lipid–lipid interactions.31,32 The non-raft-philic phospholipid, ATTO594-DOPE, was colocalized with CD59 monomers and homodimers for approximately 40 ms, while ATTO594-NeuAcLc4Cer 1 and ATTO594-Lc4Cer 2 were colocalized with CD59 homodimers for approximately 84 ms and 79 ms, respectively. Interestingly, the colocalization lifetimes of 1 and 2 with CD59 monomers were shorter (by approximately 50 ms) than those with CD59 homodimers. These lifetimes are similar to those of GM3 and GM1, which were previously reported.5 Therefore, we concluded that single-molecule tracking using fluorescent probes of lacto-series glycosphingolipids successfully detected dynamic interactions with raft molecules.
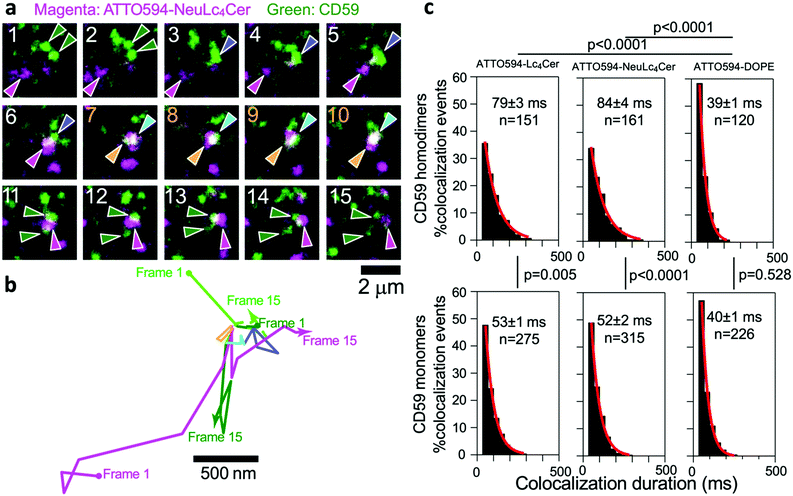 |
| Fig. 4 Single molecules of ATTO594-NeuAcLc4Cer and ATTO594-Lc4Cer were frequently and transiently recruited to CD59 monomers and homodimers in steady-state cells in the time scale of 12–45 ms. (a) Typical superimposed video-frame sequences of a ATTO594-NeuAcLc4Cer molecule (magenta spots) and two CD59 molecules (green spots) in the CHO-K1 cell PM. Two separate CD59 molecules (green arrowheads) formed a dimer (blue arrowhead) at frame 3 and diffused together (distance ≤ 240 nm) for the period of seven video frames (between frame 4 and frame 10), and the CD59 dimer (cyan arrowhead) became colocalized with a ATTO594-NeuAcLc4Cer molecule (orange arrowhead) for the period of four video frames (between frame 7 and frame 10). (b) Trajectories of the molecules shown in (a). Colocalization within 240 nm is shown in the orange (ATTO594-NeuAcLc4Cer) and cyan (CD59 transient dimer) trajectories. (c) The duration distributions of CD59 homodimers and monomers colocalized with single molecules of ATTO594-NeuAcLc4Cer, Lc4Cer and DOPE. Each time we detected colocalization, we measured its duration and obtained the distribution of colocalization durations. Single-exponential fitting of each histogram after correction with photobleaching lifetime gave the colocalization lifetime, which is shown in each box. P-Values were estimated by the log-rank test. | |
Conclusions
We developed fluorescent probes for lacto-series glycosphingolipids, and used single-molecule tracking to study their behavior in cell PMs for the first time. The fluorescent ganglioside NeuAcLc4Cer was synthesized via late-stage, fully α-selective sialylation18 of a glycolipid using a bicyclic sialyl donor.23 We improved the preparation method for this donor in terms of the macrocyclization reaction and total number of reaction steps. Next, we developed a highly soluble Lc4Cer acceptor bearing TBBz groups. The late-stage sialylation of the Lc4Cer acceptor provided a NeuLc4Cer skeleton in high yield with perfect stereoselectivity, which was then converted into the targeted NeuAcLc4Cer probe in only a few steps (global deprotection and introduction of a fluorescent dye). DRM and Lo/Ld partition tests determined the synthesized ATTO594-NeuAcLc4Cer and ATTO594-Lc4Cer functioned as raft molecules in cell PMs. Furthermore, single-molecule imaging revealed that these lacto-series glycosphingolipids were significantly colocalized with one of the major raft molecules (GPI-anchored protein CD59) (colocalization lifetime: CD59 homodimer > monomer). Thus, these probes are expected to be useful for studying the cis interactions of lacto-series glycosphingolipids and elucidating their associated lipid rafts.
Experimental section
General methods
Chemicals.
All chemicals were purchased from commercial suppliers and used without further purification. Molecular sieves were purchased from FUJIFILM Wako Pure Chemical Corporation (Osaka, Japan) and predried at 300 °C for 2 h in a muffle furnace and then dried in a flask at 300 °C for 2 h in vacuo prior to use. Drierite was purchased from FUJIFILM Wako Pure Chemical Corporation (Osaka, Japan) and predried at 230 °C for 2 h in a muffle furnace and then exposed to high vacuum. Dry solvents for reaction media (CH2Cl2, toluene, THF, MeCN, DMF, MeOH and pyridine) were purchased from Kanto Chemical Co. Inc. (Tokyo, Japan) and used without purification. Zn nano powder (<50 nm particle size) were purchased from Sigma-Aldrich. ATTO594 N-succinimidyl ester was purchased from ATTO-Tec.
TLC analysis.
TLC analyses were performed on Merck TLC plates (silica gel 60F254 on glass plate). Compound detection was either by exposure to UV light (253.6 nm) or by soaking in H2SO4 solution (10% in EtOH) or phosphomolybdic acid solution (20% in EtOH) followed by heating.
Chromatographic purification.
Silica gel column chromatography separations were performed with a flash column chromatography system. Silica gel (80 mesh and 300 mesh; Fuji Silysia Co. (Aichi, Japan)) was used for flash column chromatography. The quantity of silica gel was typically 100 to 200 times the weight of the crude sample. Sephadex LH-20 purchased from Cytiva (Marlborough, USA) was used for size-exclusion chromatography. Solvent systems for chromatography are specified as v/v ratios.
Structural analysis and acquisition of physical data.
1H and 13C NMR spectra were recorded on Avance III 500 and Avance III 800 spectrometers (Bruker, Billerica, MA, USA). Chemical shifts are expressed in ppm (δ) relative to Me4Si signal (0.00 ppm), MeCN in CD3CN (1.96 ppm) or MeNO2 in CD3NO2 (4.34 ppm). Chemical shifts in the 13C NMR spectra are expressed in ppm (δ) relative to the Me4Si signal (0.00 ppm), CDCl3 (77.36 ppm), CD3OD (49.86 ppm), CD3CN (1.79 ppm) or CD3NO2 (62.9 ppm). The sugar units are numbered using letters from a to e; Glc (a), reducing terminal Gal (b), GlcN (c), non-reducing terminal Gal (d) and Neu (e) (See the ESI†). Structural assignments were made with additional information from 2D NMR (1H–1H COSY, HMBC, and HMQC).
High-resolution mass spectrometry (ESI-TOF MS) data were obtained with a mass spectrometer (micrOTOF, Bruker). Optical rotations were measured with a high-sensitivity polarimeter (SEPA-300 and SEPA-500, Horiba (Kyoto, Japan)). [α]D values are given in 10−1 deg cm2 g−1.
General procedure for chemical reaction.
All operations were carried out in a fume hood. All reactions were carried out under a positive pressure of argon unless otherwise noted. Evaporations and concentrations were carried out in vacuo. Microwave assisted reactions were carried out using an Initiator+ Eight microwave synthesizer (Biotage Japan, Tokyo, Japan) in sealed reaction vials (0.5–2 mL or 2–5 mL vial) under magnetic stirring (600 rpm) at 40 °C without pressure.
Pretreatment for glycosidation reaction (except sialylation).
A glycosyl donor and a glycosyl acceptor were mixed in a pear-shaped flask, and then residual water was azeotropically removed with dry toluene. The mixture was exposed to high vacuum for 3 h. Molecular sieves (100 mg was used for 1 mL of reaction solvent) were predried at 300 °C for 2 h in a muffle furnace. The predried molecular sieves were added to a two-necked, round-bottomed flask, and the flask was heated at 300 °C for 2 h in vacuo.
General procedure for glycosidation (except sialylation).
In a pear-shaped flask, a glycosyl donor and a glycosyl acceptor were dissolved in the reaction solvent, and the mixture was then transferred to a two-necked flask containing predried molecular sieves via cannula. After stirring for 1 h at −20–0 °C, promoters were added to the mixture at the same temperature. The progress of the reaction was monitored by TLC analysis and/or mass spectrometry (MALDI-TOF performed with Autoflex, Bruker; matrix: CHCA). Then, the reaction mixture was quenched, filtered, subjected to an aqueous work up and then concentrated. The resulting residue was purified by size-exclusion chromatography or silica gel column chromatography. Isolated yields of the coupled products are reported.
General procedure for sialylation.
In a two-necked round-bottomed flask, a sialyl donor and glycosyl acceptor were dissolved in the reaction solvent. Promoters were added to the mixture at the −70 °C. The progress of the reaction was monitored by TLC analysis and/or mass spectrometry (MALDI-TOF performed with Autoflex, Bruker; matrix: CHCA). Then, the reaction mixture was quenched, subjected to an aqueous work up and then concentrated. The resulting residue was purified by size-exclusion chromatography and silica gel column chromatography. Isolated yields of the coupled products are reported.
Synthetic procedure
ATTO594-sialyl-lactotetraosylceramide (1).
Compound 3 (2.2 mg, 1.4 μmol) and ATTO594 N-succinimidyl ester (2.1 mg, 2.3 μmol) were dissolved in DMF/H2O (330 μL/33 μL). To the solution, triethylamine (4.0 μL, 29 μmol) was added at room temperature. After stirring for 18 h at room temperature as the reaction was monitored by TLC (CHCl3/MeOH/H2O/AcOH = 5
:
3
:
0.5
:
0.05). The resulting residue was purified by gel filtration column chromatography on Sephadex LH-20 using CHCl3/MeOH (1
:
1) as the eluent and preparative thin layer chromatography (PTLC) (CHCl3/MeOH/H2O 5
:
3
:
0.5) to give 1 (2.5 mg, 76%): 1H NMR (800 MHz, CD3OD) δ 7.73–7.35 (m, 6 H, ArATTO594), 6.80–6.79 (m, 2 H, ArATTO594), 5.92–5.88 (m, 2 H, 2 CHATTO594), 5.68 (m, 1 H, H-5Cer), 5.44 (dd, 1 H, J3,4 = 7.7 Hz, J4.5 = 15.2 Hz, H-4Cer), 4.83–3.21 (m, 49 H, H-1a, H-2a, H-3a, H-4a, H-5a, H-6aa, H-6ba, H-1b, H-2b, H-3b, H-4b, H-5b, H-6ab, H-6bb, H-1c, H-2c, H-3c, H-4c, H-5c, H-6ac, H-6bc, H-1d, H-2d, H-3d, H-4d, H-5d, H-6ad, H-6bd, H-4e, H-5e, H-6e, H-7e, H-8e, H-9ae, H-9be, H-1aCer, H-1bCer, H-2Cer, H-3Cer, 2 CH2SO3ATTO594, 3 NCH2ATTO594), 2.86 (br d, 1 H, H-3eqe), 2.72/2.66 (s, 3 H, NCH3ATTO594), 2.18–2.16 (m, 2 H, COCH2Cer), 2.03–1.97 (m, 8 H, H-6aCer, H-6bCer, 2 Ac), 1.71–1.21 (m, 75 H, H-3axe, 26 CH2Cer, 4 CH3ATTO594, 2 NCH2CH3ATTO594, NCH2CH2ATTO594, NCH2CH2CH2ATTO594), 0.91–0.86 (m, 6 H, 2 CH3Cer); 13C NMR (200 MHz, CD3OD) δ 176.0, 175.3, 174.9, 174.8, 171.2, 161.5, 159.1, 155.0, 154.2, 138.4, 137.6, 135.0, 132.2, 131.4, 130.7, 124.2, 124.2, 122.9, 122.8, 115.0, 105.1, 104.5, 103.9, 101.1, 97.1, 97.1, 83.2, 80.5, 77.6, 77.5, 77.1, 76.7, 76.5, 76.2, 74.8, 74.8, 73.0, 71.8, 71.7, 70.7, 70.6, 70.3, 70.0, 69.6, 69.4, 68.9, 64.3, 62.7, 62.5, 61.7, 56.2, 54.7, 54.0, 53.9, 53.9, 49.8, 49.5, 49.4, 49.3, 49.2, 47.7, 42.2, 41.4, 38.8, 37.4, 33.9, 33.5, 33.1, 33.1, 30.9, 30.9, 30.8, 30.8, 30.8, 30.7, 30.7, 30.6, 30.5, 30.5, 30.4, 29.5, 29.5, 29.3, 27.2, 24.2, 23.9, 23.7, 23.5, 22.7, 14.5, 14.4, 13.8, 13.8, 13.7; HRMS (ESI) m/z: found [M–2H]2− 1165.0690, C114H177N7O39S2 calcd for [M–2H]2− 1165.0689.
(5-Acetoamido-9-amino-3,5,9-trideoxy-D-glycero-α-D-galacto-2-nonulopyranosylonic acid)-(2→3)-β-D-galactopyranosyl-(1→3)-(2-acetamido-2-deoxy-β-D-glucopyranosyl)-(1→3)-β-D-galactopyranosyl-(1→4)-β-D-glucopyranosyl-(1→1)-(2S,3R,4E)-2-octadecanamido-4-octadecene-1,3-diol (3).
To a solution of 38 (12.9 mg, 3.6 μmol) in THF/MeOH (360 μL/360 μL) was added 1 M aq NaOH (72 μL, 72 μmol) at room temperature. After stirring for 3.5 days at room temperature as the reaction was monitored by TLC (CHCl3/MeOH/H2O/AcOH = 5
:
3
:
0.5
:
0.05), the reaction mixture was co-evaporated with EtOH. The resulting residue was purified by flash column chromatography on silica gel using CHCl3/MeOH/H2O (5
:
3
:
0.5) as the eluent to give 3 (5.5 mg, 99%): [α]D −15.2 (c 0.6, MeOH); 1H NMR (500 MHz, CDCl3/CD3OD = 1/2) δ 5.69 (td, 1 H, J5,6a = J5,6b = 7.2 Hz, J4,5 = 14.7 Hz, H-5Cer), 5.45 (dd, 1 H, J3,4 = 7.7 Hz, H-4Cer), 4.82–3.30 (m, 38 H, H-1a, H-2a, H-3a, H-4a, H-5a, H-6aa, H-6ba, H-1b, H-2b, H-3b, H-4b, H-5b, H-6ab, H-6bb, H-1c, H-2c, H-3c, H-4c, H-5c, H-6ac, H-6bc, H-1d, H-2d, H-3d, H-4d, H-5d, H-6ad, H-6bd, H-4e, H-5e, H-6e, H-7e, H-8e, H-9ae, H-1aCer, H-1bCer, H-2Cer, H-3Cer), 2.92 (dd, 1 H, J8,9b = 9.1 Hz, Jgem = 12.1 Hz, H-9be), 2.86 (dd, 1 H, J3eq,4 = 4.2 Hz, Jgem = 12.4 Hz, H-3eqe), 2.19–2.15 (m, 2 H, COCH2Cer), 2.03–2.01 (m, 8 H, H-6aCer, H-6bCer, 2 Ac), 1.76 (t, 1 H, J3ax,4 = 12.4 Hz, H-3axe), 1.59–1.57 (m, 2 H, COCH2CH2Cer), 1.39–1.28 (m, 50 H, 25 CH2Cer), 0.91–0.88 (m, 6 H, 2 CH3Cer); 13C NMR (200 MHz, CDCl3/CD3OD = 1/2) δ 175.5, 175.2, 174.5, 174.2, 135.0, 130.5, 129.5, 104.6, 104.5, 103.8, 102.8, 100.8, 83.4, 83.2, 80.4, 77.3, 76.8, 76.7, 76.1, 75.8, 75.5, 74.2, 72.6, 71.8, 71.0, 70.8, 69.7, 69.6, 69.5, 69.1, 68.9, 68.6, 68.5, 68.5, 68.3, 63.9, 62.3, 62.0, 61.9, 61.4, 56.3, 54.1, 53.3, 49.9, 49.5, 49.4, 49.3, 43.7, 41.4, 39.6, 37.1, 33.1, 32.6, 32.6, 30.7, 30.4, 30.4, 30.4, 30.4, 30.3, 30.3, 30.3, 30.2, 30.1, 30.1, 30.0, 30.0, 29.7. 28.6, 26.7, 24.5, 23.6, 23.4, 23.3, 22.6, 22.0, 21.2, 14.4, 11.3; HRMS (ESI) m/z: found [M–H]− 1543.8856, C73H132N4O30 calcd for [M–H]− 1543.8854.
4,7,8-Tri-O-acetyl-5-(2′,2′-dichloro-7′-hydroxy)heptoxycarbamoyl-3,5,9-trideoxy-9-trifluoroacetamido-D-glycero-α-D-galacto-2-nonulopyranosylono-1,7′-lactone 2-dibenzylphosphate (4).
NIS (28.6 mg, 127 μmol) was added to a solution of 36 (50.0 mg, 63.3 μmol) in CH2Cl2 (1.3 mL) at room temperature. TfOH (0.6 μL, 6 μmol) was added to the mixture at −20 °C. The reaction mixture was stirred for 70 min at −20 °C. As the reaction was monitored by TLC (n-hexane/EtOAc = 1
:
1), the reaction mixture was quenched with triethylamine. The mixture was diluted with CHCl3 and washed with satd aq Na2S2O3 and brine, dried over Na2SO4, and concentrated. The resulting residue was purified by column chromatography on silica gel, using n-hexane/EtOAc (2
:
1) to n-hexane/acetone (3
:
1) as the eluent, to give 4 (47.2 mg, 78%): [α]D +18.5 (c 1.0, CHCl3); 1H NMR (500 MHz, CD3NO2, 60 °C) δ 7.65 (br s, 1 H, NH-9), 7.46–7.38 (m, 10 H, Ar), 5.55 (m, 2 H, NH-5, H-4), 5.45 (dd, 1 H, J6,7 = 1.3 Hz, J7,8 = 5.8 Hz, H-7), 5.24 (td, 1 H, J8,9a = 3.2 Hz, J8,9b = 5.5 Hz, H-8), 5.21–5.13 (m, 4 H, 2 CH2Ph), 4.77 (br s, 1 H, H-6), 4.68–4.58 (m, 3 H, CO2CH2, NH(CO)OCH2), 4.25 (m, 1 H, CO2CH2), 3.95 (m, 1 H, JNH,9a = 6.3 Hz, Jgem = 14.8 Hz, H-9a), 3.57 (m, 1 H, JNH,9b = 5.8 Hz, H-9b), 3.15 (td, 1 H, JNH,5 = 6.9 Hz, J4,5 = J5,6 = 10.0 Hz, H-5), 3.03 (dd, 1 H, J3eq,4 = 5.2 Hz, Jgem = 13.1 Hz, H-3eq), 2.50 (br s, 1 H, CCl2CH2CH2), 2.33 (m, 1 H, CCl2CH2CH2), 2.22 (t, 1 H, J3ax,4 = 12.1 Hz, H-3ax), 2.17 (s, 3 H, Ac), 2.07 (s, 3 H, Ac), 2.07 (s, 3 H, Ac), 1.95–1.92 (m, 2 H, CH2), 1.77–1.63 (m, 4 H, 2 CH2); 13C NMR (125 MHz, CD3NO2, 85 °C) δ 172.9, 172.0, 171.8, 168.3, 159.2 (q, 2JC,F = 36.5 Hz, C(O)CF3), 155.9, 137.9, 137.8, 137.8, 118.0 (q, 1JC,F = 285.2 Hz, C(O)CF3), 101.2, 101.2, 91.7, 79.4, 74.4, 73.3, 71.8, 71.6, 71.6, 71.1, 68.9, 67.9, 63.4, 62.4, 54.6, 46.9, 41.3, 41.2, 39.4, 28.5, 26.6, 24.5, 21.4, 21.1, 21.1, 14.9; HRMS (ESI) m/z: found [M+Na]+ 979.1809, C39H46Cl2F3N2O16P calcd for [M+Na]+ 979.1806.
2-(Trimethylsilyl)ethyl 4,6-O-benzylidene-2-O-p-tert-butylbenzoyl-β-D-galactopyranoside (11).
To a solution of 13 (76.0 mg, 108 μmol) in MeCN/AcOH (1.9 mL/108 μL) was added Zn nanopowder (424 mg, 6.48 mmol) at room temperature. After stirring for 25 min at room temperature as the reaction was monitored by TLC (n-hexane/EtOAc = 3
:
2), the reaction mixture was filtered through a pad of Celite, and the pad was washed with EtOAc. The combined filtrate and washings were diluted with EtOAc, and washed with satd aq NaHCO3 and brine, dried over Na2SO4, and concentrated, to give 11 (58.7 mg, quant): [α]D +11.7 (c 1.0, CHCl3); 1H NMR (500 MHz, CDCl3) δ 8.00–7.97 (m, 2 H, Ar), 7.55–7.53 (m, 2 H, Ar), 7.46–7.44 (m, 2 H, Ar), 7.41–7.37 (m, 3 H, Ar), 5.58 (s, 1 H, >CHPh), 5.34 (dd, 1 H, J1,2 = 8.0 Hz, J2,3 = 10.0 Hz, H-2), 4.62 (d, 1 H, H-1), 4.39 (dd, 1 H, J5,6a = 1.3 Hz, Jgem = 12.4 Hz, H-6a), 4.26 (d, 1 H, J3,4 = 3.3 Hz, H-4), 4.11 (dd, 1 H, J5,6b = 1.7 Hz, H-6b), 4.03 (m, 1 H, SiCH2CH2), 3.88 (td, 1 H, J3,OH = 10.4 Hz, H-3), 3.61–3.54 (m, 2 H, SiCH2CH2, H-5), 2.60 (d, 1 H, OH-3), 1.34 (s, 9 H, t-Bu), 0.97–0.85 (m, 2 H, SiCH2CH2), −0.06 (s, 9 H, SiMe3); 13C NMR (125 MHz, CDCl3) δ 166.3, 156.7, 137.4, 129.7, 129.3, 128.2, 127.3, 126.5, 125.2, 101.5, 100.4, 75.8, 72.8, 72.1, 69.1, 67.0, 66.6, 35.1, 31.1, 18.0, −1.5; HRMS (ESI) m/z: found [M+Na]+ 551.2436, C29H40O7Si calcd for [M+Na]+ 551.2436.
2-(Trimethylsilyl)ethyl 4,6-O-benzylidene-2-O-p-tert-butylbenzoyl-3-O-(2,2,2-trichloroethoxycarbonyl)-β-D-galactopyranoside (13).
To a solution of 12 (2.99 g, 5.50 mmol) in CH2Cl2/pyridine (36.7 mL/18.3 mL) was added p-tert-butylbenzoyl chloride (1.99 mL, 11.0 mmol) at 0 °C. After stirring for 24 h at room temperature, as the reaction was monitored by TLC (n-hexane/EtOAc = 3
:
1, developed twice), MeOH was added to the reaction mixture at 0 °C, and the solution was co-evaporated with toluene. The mixture was diluted with CHCl3 and washed with 2 M HCl, H2O, satd aq NaHCO3 and brine, dried over Na2SO4, and concentrated. The residue was purified by column chromatography on silica gel, using n-hexane/EtOAc (4
:
1) as the eluent, to give 13 (3.99 g, quant): [α]D +42.2 (c 2.2, CHCl3); 1H NMR (500 MHz, CDCl3) δ 7.95–7.93 (m, 2 H, Ar), 7.56–7.53 (m, 2 H, Ar), 7.45–7.42 (m, 2 H, Ar), 7.39–7.35 (m, 3 H, Ar), 5.67 (dd, 1 H, J1,2 = 7.9 Hz, J2,3 = 10.4 Hz, H-2), 5.56 (s, 1 H, >CHPh), 5.13 (dd, 1 H, J3,4 = 3.6 Hz, H-3), 4.71 (d, 1 H, H-1), 4.68 (d, 1 H, Jgem = 11.9 Hz, CH2CCl3), 4.61 (d, 1 H, CH2CCl3), 4.49 (d, 1 H, H-4), 4.40 (dd, 1 H, J5,6a = 1.4 Hz, Jgem = 12.3 Hz, H-6a), 4.12 (dd, 1 H, J5,6b = 1.7 Hz, H-6b), 4.03 (m, 1 H, SiCH2CH2), 3.60–3.55 (m, 2 H, SiCH2CH2, H-5), 1.34 (s, 9 H, t-Bu), 0.94–0.84 (m, 2 H, SiCH2CH2), −0.08 (s, 9 H, SiMe3); 13C NMR (125 MHz, CDCl3) δ 164.8, 156.8, 153.7, 137.3, 129.7, 129.1, 128.1, 127.0, 126.4, 125.5, 125.3, 101.1, 100.6, 94.0, 73.4, 69.1, 68.9, 67.0, 66.2, 35.1, 31.1, 18.0, −1.5, −1.7; HRMS (ESI) m/z: found [M+Na]+ 725.1480, C32H41Cl3O9Si calcd for [M+Na]+ 725.1478.
2-(Trimethylsilyl)ethyl [4,6-O-benzylidene-2-deoxy-2-(2,2,2-trichloroethoxycarbamoyl)-3-O-(2,2,2-trichloroethoxycarbonyl)-β-D-glucopyranosyl]-(1→3)-4,6-O-benzylidene-2-O-p-tert-butylbenzoyl-β-D-galactopyranoside (14).
Molecular sieves 4 Å (432 mg) and NIS (36.4 mg, 162 μmol) were added to a solution of donor 10 (76.7 mg, 108 μmol) and acceptor 11 (57.1 mg, 108 μmol) in CH2Cl2 (0.9 mL) at room temperature. After stirring for 1 h at −20 °C, TfOH (0.9 μL, 0.01 mmol) was added to the mixture at −20 °C. The reaction mixture was stirred for 1 h at −20 °C as the reaction was monitored by TLC (n-hexane/EtOAc = 2
:
1). The reaction mixture was quenched with triethylamine and filtered through a pad of Celite, and the pad was washed with CHCl3. The combined filtrate and washings were washed with satd aq Na2S2O3 and brine, dried over Na2SO4, and concentrated. The resulting residue was purified by column chromatography on silica gel, using toluene/EtOAc (15
:
1 to 10
:
1) as the eluent, to give 14 (101 mg, 83%): [α]D +2.2 (c 1.6, CHCl3); 1H NMR (500 MHz, CD3CN) δ 7.95 (d, 2 H, Ar), 7.55 (d, 2 H, Ar), 7.51–7.49 (m, 2 H, Ar), 7.46–7.33 (m, 8 H, Ar), 5.89 (d, 1 H, JNH,2 = 9.2 Hz, NH), 5.57 (s, 1 H, >CHPh), 5.56 (s, 1 H, >CHPh), 5.20 (dd, 1 H, J1,2 = 8.1 Hz, J2,3 = 10.2 Hz, H-2b), 5.15 (t, 1 H, J2,3 = J3,4 = 9.9 Hz, H-3c), 4.93 (d, 1 H, J1,2 = 8.4 Hz, H-1c), 4.78 (d, 1 H, Jgem = 12.2 Hz, CH2CCl3), 4.61–4.57 (m, 2 H, H-1b, CH2CCl3), 4.48 (d, 1 H, J3,4 = 3.4 Hz, H-4b), 4.34 (dd, 1 H, J5,6a = 5.0 Hz, Jgem = 10.4 Hz, H-6ac), 4.23–4.17 (m, 2 H, H-6ab, CH2CCl3), 4.10 (dd, 1 H, J5,6b = 1.6 Hz, Jgem = 12.4 Hz, H-6bb), 4.04 (dd, 1 H, H-3b), 3.91 (m, 1 H, SiCH2CH2), 3.83–3.77 (m, 2 H, H-4c, H-6bc), 3.68 (d, 1 H, Jgem = 12.4 Hz, CH2CCl3), 3.60–3.49 (m, 4 H, H-5b, H-2c, H-5c, SiCH2CH2), 1.33 (s, 9 H, t-Bu), 0.81–0.67 (m, 2 H, SiCH2CH2), −0.14 (s, 9 H, SiMe3); 13C NMR (125 MHz, CDCl3) δ 165.5, 157.2, 154.0, 153.8, 137.9, 137.0, 130.1, 129.7, 129.5, 128.7, 128.5, 127.6, 126.8, 126.5, 125.8, 101.7, 101.6, 101.4, 100.6, 95.8, 94.7, 79.0, 78.5, 76.9, 75.5, 74.2, 70.2, 69.3, 68.7, 67.3, 66.8, 66.2, 57.3, 35.5, 31.5, 30.0, 18.4, −1.0, −1.1, −1.2, −1.4; HRMS (ESI) m/z: found [M+Na]+ 1148.1517, C48H57Cl6NO15Si calcd for [M+Na]+ 1148.1521.
2-(Trimethylsilyl)ethyl (2-acetamido-4,6-O-benzylidene-2-deoxy-β-D-glucopyranosyl)-(1→3)-4,6-O-benzylidene-2-O-p-tert-butylbenzoyl-β-D-galactopyranoside (16).
To a solution of 14 (707 mg, 626 μmol) in MeCN/AcOH (20.1 mL/5.0 mL) was added Zn nanopowder (4.09 g, 62.6 mmol) at room temperature. After stirring for 40 min at room temperature as the reaction was monitored by TLC (CHCl3/MeOH = 20
:
1), the reaction mixture was filtered through a pad of Celite, and the pad was washed with THF and EtOAc. The combined filtrate and washings were diluted with EtOAc, and washed with satd aq NaHCO3 and brine, dried over Na2SO4, and concentrated. The residue was exposed to high vacuum for 3 h. Next, to a solution of the resulting residue (15) in CH2Cl2/MeOH (16.7 mL/8.4 mL) was added Ac2O (118 μL, 1.25 mmol) at room temperature. After stirring for 80 min at room temperature, as the reaction was monitored by TLC (CHCl3/MeOH = 20
:
1), the reaction mixture was quenched with EtOH and concentrated. The residue was purified by flash column chromatography on silica gel, using CHCl3/MeOH (70
:
1) as the eluent, to give 16 (509 mg, 99%, two steps): [α]D −39.1 (c 1.0, CHCl3); 1H NMR (500 MHz, CDCl3) δ 7.97 (d, 2 H, Ar), 7.57 (dd, 2 H, Ar), 7.50 (d, 2 H, Ar), 7.46 (dd, 2 H, Ar), 7.42–7.37 (m, 3 H, Ar), 7.34–7.31 (m, 3 H, Ar), 5.99 (d, 1 H, JNH,2 = 3.1 Hz, NH), 5.62 (br s, 1 H, OH-3), 5.59–5.53 (m, 3 H, H-2b, 2 >CHPh), 4.65 (d, 1 H, J1,2 = 7.9 Hz, H-1c), 4.60 (d, 1 H, J1,2 = 8.2 Hz, H-1b), 4.37 (dd, 1 H, J5,6a = 1.1 Hz, Jgem = 12.3 Hz, H-6ab), 4.32 (dd, 1 H, J5,6b = 5.0 Hz, Jgem = 10.4 Hz, H-6ac), 4.28 (d, 1 H, J3,4 = 3.5 Hz, H-4b), 4.11 (dd, 1 H, J5,6b = 1.2 Hz, H-6bb), 4.07–3.99 (m, 2 H, H-3b, SiCH2CH2), 3.83–3.75 (m, 2 H, H-3c, H-6bc), 3.59–3.54 (m, 3 H, H-5b, H-4c, SiCH2CH2), 3.43–3.37 (m, 2 H, H-2c, H-5c), 1.83 (s, 3 H, Ac), 1.36 (s, 9 H, t-Bu), 0.92–0.80 (m, 2 H, SiCH2CH2), −0.09 (s, 9 H, SiMe3); 13C NMR (125 MHz, CDCl3) δ 173.0, 166.1, 157.5, 137.7, 136.9, 129.7, 129.0, 128.1, 128.1, 126.6, 126.5, 126.2, 125.7, 101.7, 101.3, 101.0, 100.4, 81.4, 77.4, 76.2, 71.6, 71.1, 68.8, 68.5, 66.9, 66.5, 66.4, 59.7, 35.1, 31.0, 22.7, 18.0, −1.4, −1.6, −1.8; HRMS (ESI) m/z: found [M+Na]+ 842.3542, C44H57NO12Si calcd for [M+Na]+ 842.3542.
2-(Trimethylsilyl)ethyl [2,6-di-O-p-tert-butylbenzoyl-3,4-di-O-(2,2,2-trichloroethoxycarbonyl)-β-D-galactopyranosyl]-(1→3)-(2-acetamido-4,6-O-benzylidene-2-deoxy-β-D-glucopyranosyl)-(1→3)-4,6-O-benzylidene-2-O-p-tert-butylbenzoyl-β-D-galactopyranoside (17).
Molecular sieves AW-300 (1.00 g) were added to a solution of donor 8 (324 mg, 317 μmol) and acceptor 16 (217 mg, 264 μmol) in CH2Cl2 (10.6 mL) at room temperature. After stirring for 1 h at 0 °C, TMSOTf (4.8 μL, 26 μmol) was added to the mixture at 0 °C. The reaction mixture was stirred for 78 h at 0 °C as the reaction was monitored by TLC (CHCl3/MeOH = 20
:
1, n-hexane/EtOAc = 3
:
1). The reaction mixture was quenched with satd aq NaHCO3 and filtered through a pad of Celite, and the pad was washed with CHCl3. The combined filtrate and washings were washed with NaHCO3 and brine, dried over Na2SO4, and concentrated. The resulting residue was purified by column chromatography on silica gel, using n-hexane/EtOAc (10
:
1 to 3
:
1) as the eluent, to give 17 (340 mg, 78%): [α]D −12.2 (c 1.3, CHCl3); 1H NMR (500 MHz, CDCl3) δ 7.87 (d, 2 H, Ar), 7.83–7.80 (m, 4 H, Ar), 7.55 (dd, 2 H, Ar), 7.49–7.47 (m, 2 H, Ar), 7.44–7.31 (m, 12 H, Ar), 5.52 (s, 1 H, >CHPh), 5.51 (s, 1 H, >CHPh), 5.50–5.44 (m, 3 H, H-2b, H-2d, NH), 5.37 (d, 1 H, J3,4 = 2.8 Hz, H-4d), 5.25 (d, 1 H, J1,2 = 8.1 Hz, H-1c), 5.07 (d, 1 H, J2,3 = 10.4 Hz, H-3d), 4.72 (t, 1 H, J2,3 = J3,4 = 9.2 Hz, H-3c), 4.72 (d, 1 H, Jgem = 11.9 Hz, CH2CCl3), 4.69 (d, 1 H, CH2CCl3), 4.67 (d, 1 H, J1,2 = 8.2 Hz, H-1d), 4.64 (d, 1 H, Jgem = 11.8 Hz, CH2CCl3), 4.51 (d, 1 H, J1,2 = 7.9 Hz, H-1b), 4.50 (d, 1 H, CH2CCl3), 4.34 (d, 1 H, Jgem = 11.2 Hz, H-6ab), 4.28 (dd, 1 H, J5,6a = 4.9 Hz, Jgem = 10.4 Hz, H-6ac), 4.26 (d, 1 H, J3,4 = 3.6 Hz, H-4b), 4.23–4.19 (m, 2 H, H-6ad, H-6bd), 4.08 (d, 1 H, H-6bb), 3.96–3.89 (m, 2 H, H-3b, SiCH2CH2), 3.75 (t, 1 H, Jgem = J5,6b = 10.2 Hz, H-6bc), 3.70 (m, 1 H, H-5d), 3.65 (t, 1 H, J4,5 = 9.1 Hz, H-4c), 3.54 (m, 1 H, H-5c), 3.51–3.45 (m, 2 H, H-5b, SiCH2CH2), 2.89 (m, 1 H, H-2c), 1.33 (s, 9 H, t-Bu), 1.31 (s, 9 H, t-Bu), 1.30 (s, 9 H, t-Bu), 0.82–0.74 (m, 2 H, SiCH2CH2), 0.62 (s, 3 H, Ac), −0.13 (s, 9 H, SiMe3); 13C NMR (125 MHz, CDCl3) δ 171.0, 165.4, 165.1, 164.5, 157.4, 157.0, 156.6, 154.0. 153.2, 137.8, 137.1, 129.7, 129.6, 129.3, 129.0, 128.4, 128.1, 127.2, 126.5, 126.4, 126.1, 125.5, 125.4, 125.2, 101.6, 101.2, 101.0, 100.8, 100.2, 94.2, 93.7, 80.5, 78.1, 75.6, 71.7, 70.4, 70.0, 69.4, 68.9, 68.7, 66.7, 66.5, 65.5, 60.4, 58.7, 35.1, 35.0, 31.1, 31.1, 31.0, 29.7, 21.8, 18.0, −1.6; HRMS (ESI) m/z: found [M+Na]+ 1672.3931, C78H93Cl6NO23Si calcd for [M+Na]+ 1672.3931.
2-(Trimethylsilyl)ethyl [2,6-di-O-p-tert-butylbenzoyl-3,4-di-O-(2,2,2-trichloroethoxycarbonyl)-β-D-galactopyranosyl]-(1→3)-(2-acetamido-2-deoxy-β-D-glucopyranosyl)-(1→3)-2-O-p-tert-butylbenzoyl-β-D-galactopyranoside (18).
Compound 17 (53.4 mg, 32.3 μmol) was dissolved in AcOH/H2O (1.0 mL/260 μL) at room temperature. After stirring for 32 h at 50 °C, as the reaction was monitored by TLC (CHCl3/MeOH = 20
:
1), the solution was co-evaporated with toluene. The mixture was diluted with CHCl3 and washed with satd aq NaHCO3 and brine, dried over Na2SO4, and concentrated. The residue was purified by column chromatography on silica gel, using CHCl3/MeOH (100
:
1 to 20
:
1) as the eluent, to give 18 (46.9 mg, 98%): [α]D +15.5 (c 0.9, CHCl3); 1H NMR (500 MHz, CD3CN) δ 7.98 (dd, 2 H, Ar), 7.86–7.82 (m, 4 H, Ar), 7.55 (dd, 2 H, Ar), 7.48–7.46 (m, 4 H, Ar), 5.87 (d, 1 H, JNH,2 = 8.3 Hz, NH), 5.51 (d, 1 H, J3,4 = 2.2 Hz, H-4d), 5.35–5.29 (m, 2 H, H-2d, H-3d), 5.08 (dd, 1 H, J1,2 = 8.1 Hz, J2,3 = 9.8 Hz, H-2b), 4.89 (d, 1 H, Jgem = 12.2 Hz, CH2CCl3), 4.87 (d, 1 H, J1,2 = 7.6 Hz, H-1d), 4.78 (d, 1 H, CH2CCl3), 4.69–4.67 (m, 2 H, CH2CCl3, H-1c), 4.59 (d, 1 H, Jgem = 12.1 Hz, CH2CCl3), 4.50 (dd, 1 H, J5,6a = 5.2 Hz, Jgem = 11.6 Hz, H-6ad), 4.47 (d, 1 H, J1,2 = 8.1 Hz, H-1b), 4.43 (dd, 1 H, J5,6b = 7.4 Hz, H-6bd), 4.34 (m, 1 H, H-5d), 4.11 (t, 1 H, J3,4 = J4,OH = 2.7 Hz, H-4b), 3.92 (s, 1 H, OH-4c), 3.89–3.84 (m, 2 H, H-3c, SiCH2CH2), 3.80 (dd, 1 H, H-3b), 3.75–3.65 (m, 3 H, H-6ab, H-6bb, H-6ac), 3.59–3.46 (m, 3 H, H-5b, H-6bc, SiCH2CH2), 3.39 (m, 1 H, H-4c), 3.25 (m, 1 H, H-2c), 3.19 (m, 1 H, H-5c), 3.10 (br s, 1 H, OH-4b), 2.83 (br t, 1 H, OH-6b), 2.67 (t, 1 H, J6a,OH = J6b,OH = 5.9 Hz, OH-6c), 1.35 (s, 9 H, t-Bu), 1.33 (s, 9 H, t-Bu), 1.32 (s, 9 H, t-Bu), 0.81–0.64 (m, 5 H, Ac, SiCH2CH2), −0.14 (s, 9 H, SiMe3); 13C NMR (125 MHz, CDCl3) δ 171.3, 165.9, 165.4, 164.6, 157.6, 157.2, 156.7, 153.9, 153.1, 129.9, 129.8, 127.3, 126.2, 125.4, 125.1, 101.4, 100.9, 100.0, 94.1, 93.7, 81.2, 77.6, 75.4, 75.2, 74.0, 72.1, 71.4, 70.9, 70.1, 69.4, 68.7, 67.2, 62.5, 61.9, 61.4, 45.4, 35.1, 35.0, 31.1, 29.7, 27.7, 22.7, 21.9, 21.5, 18.0, −1.6; HRMS (ESI) m/z: found [M+Na]+ 1496.3302, C64H85Cl6NO23Si calcd for [M+Na]+ 1496.3305.
2-(Trimethylsilyl)ethyl [2,6-di-O-p-tert-butylbenzoyl-3,4-di-O-(2,2,2-trichloroethoxycarbonyl)-β-D-galactopyranosyl]-(1→3)-(2-acetamido-4,6-di-O-p-tert-butylbenzoyl-2-deoxy-β-D-glucopyranosyl)-(1→3)-2,4,6-tri-O-p-tert-butylbenzoyl-β-D-galactopyranoside (19).
To a solution of 18 (274 mg, 185 μmol) in pyridine (7.4 mL) were added p-tert-butylbenzoyl chloride (269 μL, 1.48 mmol) and DMAP (2.3 mg, 19 μmol) at 0 °C. After stirring for 70 min at room temperature, as the reaction was monitored by TLC (toluene/EtOAc = 4
:
1), H2O was added to the reaction mixture at 0 °C, and the solution was co-evaporated with toluene. The mixture was diluted with CHCl3 and washed with 2 M HCl, H2O, satd aq NaHCO3 and brine, dried over Na2SO4, and concentrated. The residue was purified by column chromatography on silica gel, using toluene/EtOAc (19
:
1 to 16
:
1) as the eluent, to give 19 (390 mg, 99%): [α]D +15.1 (c 1.0, CHCl3); 1H NMR (500 MHz, CD3CN) δ 7.94–7.88 (m, 8 H, Ar), 7.86–7.84 (m, 4 H, Ar), 7.79–7.77 (m, 2 H, Ar), 7.54–7.51 (m, 4 H, Ar), 7.49–7.40 (m, 10 H, Ar), 5.97 (d, 1 H, JNH,2 = 8.1 Hz, NH), 5.51 (d, 1 H, J3,4 = 3.4 Hz, H-4b), 5.35 (dd, 1 H, J1,2 = 8.1 Hz, J2,3 = 10.1 Hz, H-2b), 5.24 (d, 1 H, J3,4 = 3.5 Hz, H-4d), 5.17 (dd, 1 H, J2,3 = 10.4 Hz, H-3d), 5.06–5.01 (m, 2 H, H-4c, H-2d), 4.99 (d, 1 H, J1,2 = 8.3 Hz, H-1c), 4.77 (d, 1 H, Jgem = 12.3 Hz, CH2CCl3), 4.71 (d, 1 H, CH2CCl3), 4.67 (d, 1 H, J1,2 = 7.9 Hz, H-1d), 4.66 (d, 1 H, H-1b), 4.62 (d, 1 H, Jgem = 12.2 Hz, CH2CCl3), 4.52 (d, 1 H, CH2CCl3), 4.43–4.24 (m, 5 H, H-3c, H-6ac, H-6bc, H-6ad, H-6bd), 4.19 (dd, 1 H, H-3b), 4.02 (dd, 1 H, J5,6a = 5.7 Hz, J5,6b = 6.9 Hz, H-5d), 3.93 (td, 1 H, J5,6a = J5,6b = 3.5 Hz, J4,5 = 10.0 Hz, H-5c), 3.88–3.82 (m, 2 H, H-5b, SiCH2CH2), 3.62 (dd, 1 H, J5,6a = 6.2 Hz, Jgem = 11.2 Hz, H-6ab), 3.54 (m, 1 H, SiCH2CH2), 3.42 (dd, 1 H, J5,6b = 7.5 Hz, H-6bb), 3.08 (m, 1 H, H-2c), 1.33 (s, 9 H, t-Bu), 1.33 (s, 9 H, t-Bu), 1.32 (s, 9 H, t-Bu), 1.31 (s, 9 H, t-Bu), 1.29 (s, 9 H, t-Bu), 1.28 (s, 9 H, t-Bu), 1.23 (s, 9 H, t-Bu), 0.99 (s, 3 H, Ac), 0.84–0.68 (m, 2 H, SiCH2CH2), −0.16 (s, 9 H, SiMe3); 13C NMR (125 MHz, CD3CN) δ 170.7, 166.7, 166.7, 166.2, 166.0, 165.8, 165.8, 165.8, 158.2, 157.9, 157.9, 157.8, 157.8, 157.7, 154.5, 153.6, 138.9, 130.7, 130.6, 130.4, 130.4, 130.3, 130.3, 129.9, 129.2, 128.4, 128.3, 128.3, 128.2, 127.8, 127.5, 126.6, 126.6, 126.5, 126.5, 126.4, 126.3, 126.3, 101.6, 101.4, 95.4, 94.9, 79.3, 78.7, 77.6, 77.5, 76.5, 73.3, 72.6, 72.4, 71.8, 71.6, 70.6, 70.5, 70.4, 67.8, 63.7, 63.5, 61.2, 57.4, 35.8, 35.8, 35.7, 35.7, 31.4, 31.4, 31.3, 31.3, 31.3, 22.6, 21.5, 18.7, −1.5; HRMS (ESI) m/z: found [M+Na]+ 2136.6858, C108H133Cl6NO27Si calcd for [M+Na]+ 2136.6858.
[2,6-Di-O-p-tert-butylbenzoyl-3,4-di-O-(2,2,2-trichloroethoxycarbonyl)-β-D-galactopyranosyl]-(1→3)-(2-acetamido-4,6-di-O-p-tert-butylbenzoyl-2-deoxy-β-D-glucopyranosyl)-(1→3)-2,4,6-tri-O-p-tert-butylbenzoyl-D-galactopyranose (20).
To a solution of 19 (316 mg, 149 μmol) in CH2Cl2 (6.6 mL) was added TFAcOH (3.3 mL) at 0 °C. After stirring for 4.5 h at room temperature, as the reaction was monitored by TLC (CHCl3/MeOH = 50
:
1), satd aq NaHCO3 was added to the reaction mixture at 0 °C. The mixture was diluted with CHCl3 and washed with satd aq NaHCO3 and brine, dried over Na2SO4, and concentrated. The residue was purified by column chromatography on silica gel, using n-hexane/EtOAc (4
:
1 to 2
:
1) as the eluent, to give 20 (311 mg, quant): α-isomer: 1H NMR (500 MHz, CD3CN) δ 7.96–7.85 (m, 12 H, Ar), 7.76–7.74 (m, 2 H, Ar), 7.54–7.50 (m, 4 H, Ar), 7.45–7.37 (m, 10 H, Ar), 6.00 (d, 1 H, JNH,2 = 8.3 Hz, NH), 5.95 (d, 1 H, J3,4 = 3.2 Hz, H-4b), 5.46 (t, 1 H, J1,2 = J1,OH = 3.7 Hz, H-1b), 5.41 (dd, 1 H, J2,3 = 10.4 Hz, H-2b), 5.25 (d, 1 H, J3,4 = 3.5 Hz, H-4d), 5.17 (dd, 1 H, J2,3 = 10.4 Hz, H-3d), 5.06–5.00 (m, 3 H, H-1c, H-4c, H-2d), 4.91 (d, 1 H, OH-1), 4.77 (d, 1 H, Jgem = 12.2 Hz, CH2CCl3), 4.72 (d, 1 H, J1,2 = 7.6 Hz, H-1d), 4.71 (d, 1 H, CH2CCl3), 4.62 (d, 1 H, Jgem = 12.2 Hz, CH2CCl3), 4.56 (t, 1 H, J5,6a = J5,6b = 6.2 Hz, H-5d), 4.52–4.49 (m, 2 H, H-3b, CH2CCl3), 4.46–4.36 (m, 3 H, H-3c, H-6ac, H-6ad), 4.32–4.20 (m, 2 H, H-6bc, H-6bd), 3.99 (m, 1 H, H-5c), 3.90 (t, 1 H, J5,6a = J5,6b = 7.1 Hz, H-5b), 3.61 (dd, 1 H, Jgem = 11.3 Hz, H-6ab), 3.46 (dd, 1 H, H-6bb), 3.16 (m, 1 H, H-2c), 1.32 (s, 9 H, t-Bu), 1.31 (s, 9 H, t-Bu), 1.28 (m, 27 H, 3 t-Bu), 1.26 (s, 9 H, t-Bu), 1.23 (s, 9 H, t-Bu), 0.95 (s, 3 H, Ac); 13C NMR (125 MHz, CD3CN) δ 171.6, 170.8, 170.6, 166.7, 166.7, 166.4, 166.2, 166.2, 166.0, 166.0, 165.9, 165.8, 158.2, 158.2, 158.1, 158.0, 157.9, 157.8, 157.8, 157.7, 157.6, 154.5, 153.6, 130.7, 130.6, 130.5, 130.4, 130.4, 130.3, 128.3, 128.3, 128.2, 128.1, 128.1, 128.1, 127.9, 127.8, 127.5, 126.7, 126.6, 126.6, 126.5, 126.3, 126.3, 101.9, 101.6, 101.4, 96.4, 95.4, 94.8, 91.3, 79.1, 79.1, 79.0, 78.7, 77.6, 77.5, 76.5, 75.3, 73.3, 73.3, 72.7, 72.6, 72.4, 71.7, 71.2, 70.7, 70.6, 70.4, 67.9, 63.8, 63.6, 61.3, 61.2, 61.0, 57.2, 35.8, 35.8, 35.7, 35.7, 35.7, 35.4, 31.3, 31.3, 22.6, 21.2, 14.5; HRMS (ESI) m/z: found [M+Na]+ 2036.6148, C103H121Cl6NO27 calcd for [M+Na]+ 2036.6148.
[2,6-Di-O-p-tert-butylbenzoyl-3,4-di-O-(2,2,2-trichloroethoxycarbonyl)-β-D-galactopyranosyl]-(1→3)-(2-acetamido-4,6-di-O-p-tert-butylbenzoyl-2-deoxy-β-D-glucopyranosyl)-(1→3)-2,4,6-tri-O-p-tert-butylbenzoyl-D-galactopyranosyl 2,2,2-trifluoro-N-phenylacetimidate (21).
To a solution of 20 (86.6 mg, 42.9 μmol) in acetone (1.7 mL) were added CF3C(NPh)Cl (13.9 μL, 85.8 μmol) and K2CO3 (29.7 mg, 215 μmol) at 0 °C. After stirring for 1.5 h at room temperature, as the reaction was monitored by TLC (CHCl3/MeOH = 50
:
1), the solution was filtered through a pad of Celite, and the pad was washed with CHCl3. The combined filtrate and washings were concentrated. The residue was purified by column chromatography on silica gel, using n-hexane/EtOAc (10
:
1 to 6
:
1) as eluent, to give 21 (94.3 mg, quant, α/β = 75/25): α-isomer; [α]D +22.0 (c 1.1, CHCl3); 1H NMR (500 MHz, CD3CN) δ 7.94–7.85 (m, 12 H, Ar), 7.76–7.74 (m, 2 H, Ar), 7.55–7.54 (m, 4 H, Ar), 7.47–7.39 (m, 10 H, Ar), 7.11–7.08 (m, 2 H, Ar), 7.00 (m, 1 H, Ar), 6.58 (br s, 1 H, H-1b), 6.38 (br s, 2 H, Ar), 6.04 (d, 1 H, J3,4 = 3.1 Hz, H-4b), 6.92 (d, 1 H, JNH,2 = 8.3 Hz, NH), 5.66 (br d, 1 H, J2,3 = 8.4 Hz, H-2b), 5.23 (d, 1 H, J3,4 = 3.0 Hz, H-4d), 5.17 (dd, 1 H, J2,3 = 10.4 Hz, H-3d), 5.05–5.01 (m, 3 H, H-1c, H-4c, H-2d), 4.77 (d, 1 H, Jgem = 12.2 Hz, CH2CCl3), 4.71 (d, 1 H, CH2CCl3), 4.70 (d, 1 H, J1,2 = 7.9 Hz, H-1d), 4.62 (d, 1 H, Jgem = 12.2 Hz, CH2CCl3), 4.57–4.53 (m, 2 H, H-3b, H-6ad), 4.51 (d, 1 H, CH2CCl3), 4.45–4.35 (m, 4 H, H-3c, H-6ac, H-6bc, H-5d), 4.25 (dd, 1 H, J5,6b = 7.5 Hz, Jgem = 11.2 Hz, H-6bd), 3.98 (td, 1 H, J5,6a = 2.6 Hz, J5,6b = 4.4 Hz, J4,5 = 10.1 Hz, H-5c), 3.88 (t, 1 H, J5,6a = J5,6b = 7.0 Hz, H-5b), 3.60 (dd, 1 H, Jgem = 11.2 Hz, H-6ab), 3.44 (dd, 1 H, H-6bb), 3.15 (m, 1 H, H-2c), 1.34 (s, 9 H, t-Bu), 1.32 (s, 9 H, t-Bu), 1.30 (m, 18 H, 2 t-Bu), 1.28 (s, 9 H, t-Bu), 1.28 (s, 9 H, t-Bu), 1.23 (s, 9 H, t-Bu), 0.96 (s, 3 H, Ac); 13C NMR (125 MHz, CD3CN) δ 171.6, 170.6, 166.7, 166.6, 166.1, 166.0, 165.9, 165.8, 165.8, 158.6, 158.2, 158.1, 158.0, 158.0, 157.9, 157.6, 154.5, 153.6, 130.7, 130.4, 130.4, 130.3, 130.3, 129.6, 128.3, 128.0, 127.9, 127.8, 127.5, 127.4, 126.7, 126.6, 126.6, 126.5, 126.4, 126.3, 119.9, 118.6, 101.4, 95.4, 94.8, 79.1, 78.8, 77.6, 77.5, 76.4, 75.6, 73.3, 72.6, 71.8, 71.2, 70.5, 70.4, 68.9, 63.7, 63.6, 61.3, 60.9, 35.8, 35.8, 35.8, 35.7, 31.3, 31.3, 31.3, 31.2, 22.6, 21.1, 14.5; HRMS (ESI) m/z: found [M+Na]+ 2207.6443, C111H125Cl6F3N2O27 calcd for [M+Na]+ 2207.6445.
[2,6-Di-O-p-tert-butylbenzoyl-3,4-di-O-(2,2,2-trichloroethoxycarbonyl)-β-D-galactopyranosyl]-(1→3)-(2-acetamido-2-deoxy-4,6-di-O-p-tert-butylbenzoyl-β-D-glucopyranosyl)-(1→3)-(2,4,6-tri-O-p-tert-butylbenzoyl-β-D-galactopyranosyl)-(1→4)-(2-O-p-tert-butylbenzoyl-3,6-di-O-p-methoxybenzyl-β-D-glucopyranosyl)-(1→1)-(2S,3R,4E)-3-O-p-tert-butylbenzoyl-2-octadecanamido-4-octadecene-1,3-diol (22).
Molecular sieves AW-300 (309 mg) were added to a solution of donor 21 (169 mg, 77.2 μmol) and GlcCer cassette 7 (144 mg, 116 μmol) in CH2Cl2 (3.0 mL) at room temperature. After stirring for 1 h at 0 °C, TMSOTf (2.8 μL, 15.4 μmol) was added to the mixture at 0 °C. The reaction mixture was stirred for 1.5 h at 0 °C as the reaction was monitored by TLC (CHCl3/MeOH = 70
:
1). The reaction mixture was quenched with triethylamine and filtered through a pad of Celite, and the pad was washed with CHCl3. The combined filtrate and washings were washed with brine, dried over Na2SO4, and concentrated. The resulting residue was purified by gel filtration column chromatography on Sephadex LH-20 using CHCl3/MeOH (1
:
1) as the eluent, to give 22 (229 mg, 90%): [α]D +18.9 (c 0.8, CHCl3); 1H NMR (500 MHz, CDCl3) δ 8.01–7.75 (m, 18 H, Ar), 7.44–7.30 (m, 18 H, Ar), 7.13 (m, 2 H, Ar), 6.52 (m, 2 H, Ar), 6.81 (m, 2 H, Ar), 6.38 (m, 2 H, Ar), 5.92 (d, 1 H, J3,4 = 3.3 Hz, H-4b), 5.75 (m, 1 H, H-5Cer), 5.68 (d, 1 H, JNH,2 = 9.2 Hz, NHCer), 5.49–5.44 (m, 2 H, H-3Cer, H-2b), 5.39 (dd, 1 H, J3,4 = 7.4 Hz, J4,5 = 15.1 Hz, H-4Cer), 5.26 (dd, 1 H, J1,2 = 7.9 Hz, J2,3 = 10.5 Hz, H-2d), 5.23–5.12 (m, 5 H, H-2a, H-1c, H-4c, NHc, H-4d), 5.03 (dd, 1 H, J3,4 = 3.5 Hz, H-3d), 4.86 (t, 1 H, J2,3 = J3,4 = 9.4 Hz, H-3c), 4.82 (d, 1 H, Jgem = 11.2 Hz, PhCH2), 4.76 (d, 1 H, Jgem = 11.9 Hz, CH2CCl3), 4.69 (d, 1 H, PhCH2), 4.68 (d, 1 H, CH2CCl3), 4.63 (dd, 1 H, J5,6a = 2.6 Hz, Jgem = 12.2 Hz, H-6ac), 4.60 (d, 1 H, Jgem = 11.8 Hz, CH2CCl3), 4.57 (d, 1 H, J1,2 = 8.0 Hz, H-1b), 4.56 (d, 1 H, H-1d), 4.53 (d, 1 H, Jgem = 12.1 Hz, PhCH2), 4.43 (d, 1 H, CH2CCl3), 4.39 (dd, 1 H, J5,6b = 6.0 Hz, H-6bc), 4.35–4.28 (m, 3 H, H-1a, H-6ad, H-2Cer), 4.17 (dd, 1 H, J5,6b = 7.4 Hz, Jgem = 11.5 Hz, H-6bd), 4.09 (t, 1 H, J3,4 = J4,5 = 9.2 Hz, H-4a), 4.08 (d, 1 H, PhCH2), 4.03 (m, 1 H, H-5c), 3.97 (dd, 1 H, J1a,2 = 3.0 Hz, Jgem = 9.0 Hz, H-1aCer), 3.83–3.80 (m, 4 H, H-5d, OCH3), 3.76–3.69 (m, 3 H, H-3a, H-3b, H-6ab), 3.59 (dd, 1 H, J5,6a = 6.0 Hz, J5,6b = 8.4 Hz, H-5b), 3.54–3.52 (m, 4 H, H-6aa, OCH3), 3.44 (dd, 1 H, J1b,2 = 3.9 Hz, H-1bCer), 3.33–3.29 (m, 2 H, H-6ba, H-6bb), 3.15 (m, 1 H, H-5a), 2.71 (m, 1 H, H-2c), 1.96–1.92 (m, 2 H, H-6aCer, H-6bCer), 1.74–1.68 (m, 2 H, COCH2Cer), 1.35–1.18 (m, 133 H, 9 t-Bu, 26 CH2Cer), 0.89–0.86 (m, 6 H, 2 CH3Cer), 0.67 (s, 3 H, Ac); 13C NMR (125 MHz, CDCl3) δ 172.7, 170.9, 166.1, 166.1, 165.2, 165.0, 164.9, 164.8, 164.5, 164.4, 159.4, 158.7, 157.5, 157.3, 156.9, 156.9, 156.8, 156.6, 156.6, 156.5, 156.4, 153.9, 153.1, 136.9, 130.5, 130.0, 129.9, 129.9, 129.7, 129.6, 129.5, 129.5, 127.6, 127.2, 127.1, 127.0, 126.9, 126.8, 126.4, 126.4, 126.2, 125.5, 125.4, 125.4, 125.3, 125.3, 124.9, 113.9, 113.2, 101.3, 101.0, 100.2, 99.3, 94.3, 93.6, 79.1, 78.2, 77.6, 76.9, 76.0, 75.2, 74.9, 74.3, 73.7, 73.1, 73.0, 72.0, 71.6, 71.4, 71.1, 70.4, 70.2, 69.8, 67.5, 63.2, 62.1, 59.4, 58.9, 55.4, 55.0, 50.4, 36.4, 35.1, 35.1, 35.1, 35.1, 35.0, 32.3, 31.9, 31.1, 31.1, 31.0, 29.7, 29.7, 29.7, 29.6, 29.5, 29.5, 29.4, 29.3, 29.3, 29.0, 25.6, 22.7, 21.9, 14.1; HRMS (ESI) m/z: found [M+2Na]2+ 1664.7413, C183H240Cl6N2O38 calcd for [M+2Na]2+ 1664.7413.
[2,6-Di-O-p-tert-butylbenzoyl-3,4-di-O-(2,2,2-trichloroethoxycarbonyl)-β-D-galactopyranosyl]-(1→3)-(2-acetamido-4,6-di-O-p-tert-butylbenzoyl-2-deoxy-β-D-glucopyranosyl)-(1→3)-(2,4,6-tri-O-p-tert-butylbenzoyl-β-D-galactopyranosyl)-(1→4)-(2-O-p-tert-butylbenzoyl-β-D-glucopyranosyl)-(1→1)-(2S,3R,4E)-3-O-p-tert-butylbenzoyl-2-octadecanamido-4-octadecene-1,3-diol (23).
To a solution of 22 (36.0 mg, 10.9 μmol) in CH2Cl2 (290 μL) was added TFAcOH (150 μL) at 0 °C. After stirring for 2.5 h at 0 °C, as the reaction was monitored by TLC (n-hexane/acetone = 3
:
1), satd aq NaHCO3 was added to the reaction mixture. The mixture was diluted with CHCl3 and washed with brine, dried over Na2SO4, and concentrated. The residue was purified by column chromatography on silica gel using n-hexane/acetone (11
:
2) as the eluent and gel filtration column chromatography on Sephadex LH-20 using CHCl3/MeOH (1
:
1) as the eluent to give 23 (33.3 mg, quant): [α]D +28.4 (c 1.6, CHCl3); 1H NMR (500 MHz, CDCl3) δ 8.01–7.92 (m, 12 H, Ar), 7.88–7.86 (m, 2 H, Ar), 7.77–7.75 (m, 4 H, Ar), 7.46–7.34 (m, 18 H, Ar), 5.90 (d, 1 H, J3,4 = 3.3 Hz, H-4b), 5.87–5.81 (m, 2 H, H-5Cer, NHCer), 5.59–5.53 (m, 2 H, H-3Cer, H-2b), 5.43 (dd, 1 H, J3,4 = 8.3 Hz, J4,5 = 15.3 Hz, H-4Cer), 5.28 (d, 1 H, J1,2 = 8.1 Hz, H-1c), 5.25 (dd, J1,2 = 7.9 Hz, J2,3 = 10.5 Hz, H-2d), 5.22–5.17 (m, 2 H, H-4c, H-4d), 5.13 (d, 1 H, JNH,2 = 6.7 Hz, NHc), 5.09 (dd, 1 H, J1,2 = 8.0 Hz, J2,3 = 9.6 Hz, H-2a), 5.03 (dd, 1 H, J3,4 = 3.5 Hz, H-3d), 4.85 (t, 1 H, J2,3 = J3,4 = 9.4 Hz, H-3c), 4.76 (d, 1 H, J5,6a = 3.2 Hz, Jgem = 11.9 Hz, H-6ac), 4.75 (d, 1 H, Jgem = 11.9 Hz, CH2CCl3), 4.71–4.66 (m, 2 H, CH2CCl3, H-1b), 4.61–4.54 (m, 3 H, CH2CCl3, H-1d, H-6ad), 4.45–4.35 (m, 5 H, H-1a, OH-3a, H-6bd, H-2Cer, CH2CCl3), 4.04 (dd, 1 H, J5,6b = 9.1 Hz, H-6bc), 4.00 (dd, 1 H, J3,4 = 3.3 Hz, J2,3 = 10.2 Hz, H-3b), 3.96–3.92 (m, 3 H, H-3a, H-5c, H-5d), 3.82–3.77 (m, 3 H, H-1aCer, H-4a, H-6ab), 3.55 (dd, 1 H, J5,6a = 5.8 Hz, J5,6b = 8.8 Hz, H-5b), 3.44 (dd, 1 H, J1b,2 = 3.6 Hz, Jgem = 9.3 Hz, H-1bCer), 3.36 (dd, 1 H, Jgem = 10.1 Hz, H-6bb), 3.27 (t, 1 H, Jgem = J5,6a = 10.6 Hz, H-6aa), 3.14–3.09 (m, 2 H, H-5a, OH-6a), 2.75–2.70 (m, 2 H, H-6ba, H-2c), 1.98–1.95 (m, 4 H, H-6aCer, H-6bCer, CH2Cer), 1.50–1.41 (m, 2 H, COCH2Cer), 1.35–1.21 (m, 131 H, 9 t-Bu, 25 CH2Cer), 0.89–0.86 (m, 6 H, 2 CH3Cer), 0.64 (s, 3 H, Ac); 13C NMR (125 MHz, CDCl3) δ 172.7, 171.0, 166.1, 166.0, 165.7, 165.7, 165.1, 165.0, 164.7, 164.7, 164.4, 157.5, 157.3, 157.0, 156.9, 156.8, 156.7, 156.6, 153.9, 153.1, 138.1, 130.0, 129.9, 129.9, 129.7, 129.6, 129.5, 127.3, 127.2, 127.1, 127.0, 126.5, 126.5, 126.4, 126.2, 126.1, 125.6, 125.5, 125.5, 125.5, 125.4, 125.4, 125.4, 125.0, 102.1, 101.0, 99.8, 99.3, 94.3, 93.7, 80.6, 78.1, 77.6, 76.9, 76.9, 75.2, 74.0, 74.0, 73.6, 73.0, 72.4, 72.1, 71.3, 70.3, 70.2, 70.0, 69.8, 69.5, 62.7, 59.4, 58.9, 53.8, 50.3, 36.7, 35.2, 35.1, 35.1, 35.1, 35.0, 32.3, 31.9, 31.7, 31.1, 31.1, 31.1, 31.0, 29.8, 29.7, 29.7, 29.6, 29.5, 29.5, 29.4, 29.3, 28.9, 25.6, 22.7, 21.8, 14.1; HRMS (ESI) m/z: found [M+2Na]2+ 1544.6837, C167H224Cl6N2O36 calcd for [M+2Na]2+ 1544.6837.
[2,6-Di-O-p-tert-butylbenzoyl-3,4-di-O-(2,2,2-trichloroethoxycarbonyl)-β-D-galactopyranosyl]-(1→3)-(2-acetamido-4,6-di-O-p-tert-butylbenzoyl-2-deoxy-β-D-glucopyranosyl)-(1→3)-(2,4,6-tri-O-p-tert-butylbenzoyl-β-D-galactopyranosyl)-(1→4)-(2,6-di-O-p-tert-butylbenzoyl-β-D-glucopyranosyl)-(1→1)-(2S,3R,4E)-3-O-p-tert-butylbenzoyl-2-octadecanamido-4-octadecene-1,3-diol (24).
To a solution of 23 (40.9 mg, 13.4 μmol) in pyridine (540 μL) were added p-tert-butylbenzoyl chloride (7.3 μL, 40 μmol) and DMAP (0.3 mg, 3 μmol) at 0 °C. After stirring for 1 h at room temperature, p-tert-butylbenzoyl chloride (139 μL, 765 μmol) and DMAP (12.1 mg, 99 μmol) were added in 9 portions at 0 °C. After stirring for 3 days at 40 °C, as the reaction was monitored by TLC (toluene/acetone = 5
:
1), H2O was added to the reaction mixture at 0 °C. The mixture was diluted with CHCl3 and washed with 2 M HCl, H2O, satd aq NaHCO3 and brine, dried over Na2SO4, and concentrated. The residue was purified by column chromatography on silica gel, using toluene/EtOAc (24
:
1 to 18
:
1) as the eluent, to give 24 (40.5 mg, 94%): [α]D +19.4 (c 0.8, CHCl3); 1H NMR (500 MHz, CDCl3) δ 8.01–7.93 (m, 10 H, Ar), 7.85–7.80 (m, 4 H, Ar), 7.77–7.71 (m, 6 H, Ar), 7.48–7.46 (m, 2 H, Ar), 7.42–7.34 (m, 14 H, Ar), 7.30–7.29 (m, 2 H, Ar), 7.20–7.18 (m, 2 H, Ar), 5.92 (d, 1 H, J3,4 = 3.3 Hz, H-4b), 5.70 (td, 1 H, J5,6a = J5,6b = 7.2 Hz, J4,5 = 14.6 Hz, H-5Cer), 5.65–5.60 (m, 2 H, H-2b, NHCer), 5.43 (t, 1 H, J2,3 = J3,4 = 6.9 Hz, H-3Cer), 5.36 (dd, 1 H, H-4Cer), 5.27 (d, 1 H, J1,2 = 8.2 Hz, H-1c), 5.24 (dd, 1 H, J1,2 = 8.0 Hz, J2,3 = 10.5 Hz, H-2d), 5.20–5.12 (m, 4 H, H-2a, H-4c, NHc, H-4d), 5.01 (dd, 1 H, J3,4 = 3.5 Hz, H-3d), 4.86–4.80 (m, 2 H, H-3c, H-6ac), 4.74 (d, 1 H, Jgem = 11.9 Hz, CH2CCl3), 4.71 (d, 1 H, J1,2 = 8.1 Hz, H-1b), 4.69 (d, 1 H, J3,OH = 0.8 Hz, OH-3a), 4.67 (d, 1 H, CH2CCl3), 4.60–4.55 (m, 3 H, H-1d, H-6ad, CH2CCl3), 4.46 (d, 1 H, J1,2 = 8.0 Hz, H-1a), 4.42 (d, 1 H, Jgem = 11.8 Hz, CH2CCl3), 4.39–4.29 (m, 3 H, H-6aa, H-6bd, H-2Cer), 4.05 (dd, 1 H, J5,6b = 9.2 Hz, Jgem = 11.9 Hz, H-6bc), 4.01–3.90 (m, 6 H, H-3a, H-6ba, H-3b, H-5c, H-5d, H-1aCer), 3.78 (dd, 1 H, J5,6a = 5.1 Hz, Jgem = 11.2 Hz, H-6ab), 3.71–3.63 (m, 2 H, H-4a, H-5a), 3.55–3.52 (m, 2 H, H-5b, H-1bCer), 3.35 (dd, 1 H, J5,6b = 9.1 Hz, H-6bb), 2.72 (m, 1 H, JNH,2 = 7.5 Hz, J2,3 = 9.5 Hz, H-2c), 1.91–1.87 (m, 2 H, H-6aCer, H-6bCer), 1.77–1.68 (m, 2 H, COCH2Cer), 1.42–1.05 (m, 142 H, 10 t-Bu, 26 CH2Cer), 0.89–0.86 (m, 6 H, 2 CH3Cer), 0.59 (s, 3 H, Ac); 13C NMR (125 MHz, CDCl3) δ 172.6, 171.1, 166.2, 166.1, 165.3, 165.2, 165.1, 165.0, 164.7, 164.4, 157.5, 157.3, 157.0, 156.9, 156.8, 156.7, 156.6, 156.6, 156.3, 153.9, 153.1, 136.6, 131.0, 130.0, 129.8, 129.7, 129.6, 129.5, 129.5, 129.0, 128.9, 128.6, 128.2, 127.5, 127.1, 127.0, 126.9, 126.4, 126.4, 126.4, 126.3, 126.1, 125.7, 125.6, 125.5, 125.4, 1254, 125.3, 125.2, 124.8, 102.3, 101.0, 99.3, 94.3, 93.6, 82.3, 79.2, 78.3, 77.6, 76.9, 76.9, 75.2, 74.2, 73.3, 73.2, 72.8, 72.3, 72.3, 71.3, 70.3, 70.2, 70.1, 69.9, 69.8, 67.5, 63.2, 63.0, 61.8, 59.3, 58.8, 53.8, 50.5, 36.4, 35.1, 35.1, 35.1, 35.1, 35.0, 35.0, 34.9, 32.2, 31.9, 31.2, 31.1, 31.1, 31.0, 30.9, 29.7, 29.7, 29.7, 29.7, 29.6, 29.5, 29.5, 29.4, 29.3, 29.2, 29.0, 25.6, 22.7, 21.7, 14.1; HRMS (ESI) m/z: found [M+2Na]2+ 1624.7281, C178H236Cl6N2O37 calcd for [M+2Na]2+ 1624.7282.
(2,6-Di-O-p-tert-butylbenzoyl-β-D-galactopyranosyl)-(1→3)-(2-acetamido-4,6-di-O-p-tert-butylbenzoyl-2-deoxy-β-D-glucopyranosyl)-(1→3)-(2,4,6-tri-O-p-tert-butylbenzoyl-β-D-galactopyranosyl)-(1→4)-(2,6-di-O-p-tert-butylbenzoyl-β-D-glucopyranosyl)-(1→1)-(2S,3R,4E)-3-O-p-tert-butylbenzoyl-2-octadecanamido-4-octadecene-1,3-diol (25).
To a solution of 24 (38.6 mg, 12.0 μmol) in MeCN/AcOH (460 μL/115 μL) was added Zn nanopowder (97.4 mg, 1.49 mmol) at room temperature. After stirring for 5.5 h at room temperature, Zn nanopowder (135 mg, 2.06 mmol) was added at room temperature. After stirring for 3 h at room temperature, as the reaction was monitored by TLC (toluene/acetone = 6
:
1), the reaction mixture was filtered through a pad of Celite, and the pad was washed with CHCl3. The combined filtrate and washings were diluted with CHCl3, and washed with satd aq NaHCO3 and brine, dried over Na2SO4, and concentrated. The residue was purified by flash column chromatography on silica gel, using toluene/acetone (16
:
1 to 10
:
1) as the eluent, to give 25 (31.4 mg, 92%): [α]D +26.3 (c 0.8, CHCl3); 1H NMR (500 MHz, CDCl3) δ 8.00–7.94 (m, 10 H, Ar), 7.85–7.80 (m, 8 H, Ar), 7.74–7.72 (m, 2 H, Ar), 7.46–7.35 (m, 16 H, Ar), 7.30–7.29 (m, 2 H, Ar), 7.21–7.20 (m, 2 H, Ar), 5.92 (d, 1 H, J3,4 = 3.2 Hz, H-4b), 5.69 (m, 1 H, J5,6a = J5,6b = 7.1 Hz, J4,5 = 14.6 Hz, H-5Cer), 5.65 (dd, 1 H, J1,2 = 8.2 Hz, J2,3 = 9.9 Hz, H-2b), 5.62 (d, 1 H, JNH,2 = 9.1 Hz, NHCer), 5.42 (t, 1 H, J2,3 = J3,4 = 6.9 Hz, H-3Cer), 5.35 (dd, 1 H, H-4Cer), 5.31 (d, 1 H, J1,2 = 8.1 Hz, H-1c), 5.25 (d, 1 H, JNH,2 = 6.7 Hz, NHc), 5.17–5.11 (m, 2 H, H-2a, H-4c), 5.01 (dd, 1 H, J1,2 = 7.9 Hz, J2,3 = 9.6 Hz, H-2d), 4.86 (br d, 1 H, H-6ac), 4.76 (t, 1 H, J2,3 = J3,4 = 9.4 Hz, H-3c), 4.73–4.72 (m, 2 H, H-1b, OH-3a), 4.57 (dd, 1 H, J5,6a = 2.1 Hz, Jgem = 12.0 Hz, H-6ad), 4.46 (d, 1 H, J1,2 = 8.0 Hz, H-1a), 4.38–4.29 (m, 4 H, H-6aa, H-1d, H-6bd, H-2Cer), 4.05–3.89 (m, 7 H, H-3a, H-6ba, H-3b, H-5c, H-6bc, H-5d, H-1aCer), 3.86 (dd, 1 H, J5,6a = 8.8 Hz, Jgem = 11.2 Hz, H-6ab), 3.70 (t, 1 H, J3,4 = J4,5 = 8.9 Hz, H-4a), 3.65 (m, 1 H, H-5a), 3.58–3.52 (m, 3 H, H-6bb, H-4d, H-1bCer), 3.48 (td, 1 H, J3,4 = 3.4 Hz, J3,OH = 9.7 Hz, H-3d), 3.21 (dd, 1 H, J5,6b = 8.5 Hz, H-5b), 2.81 (br s, 1 H, OH-4d), 2.73 (dd, 1 H, H-2c), 2.65 (d, 1 H, OH-3d), 1.91–1.87 (m, 2 H, H-6aCer, H-6bCer), 1.78–1.66 (m, 2 H, COCH2Cer), 1.34–1.17 (m, 142 H, 10 t-Bu, 26 CH2Cer), 0.89–0.86 (m, 6 H, 2 CH3Cer), 0.64 (s, 3 H, Ac); 13C NMR (125 MHz, CDCl3) δ 172.6, 170.9, 166.3, 166.2, 166.1, 165.9, 165.3, 165.2, 165.1, 165.0, 164.8, 157.3, 157.2, 156.9, 156.8, 156.7, 156.6, 156.3, 136.6, 130.0, 129.8, 129.7, 129.7, 129.6, 129.6, 129.5, 129.5, 127.5, 127.1, 127.0, 127.0, 126.9, 126.8, 126.4, 126.3, 125.7, 125.5, 125.5, 125.4, 125.4, 125.3, 125.2, 124.8, 102.3, 101.0, 100.9, 99.4, 82.3, 78.4, 77.6, 76.6, 74.2, 73.8, 73.3, 73.2, 72.8, 72.3, 72.3, 72.2, 71.7, 70.4, 70.3, 69.8, 67.8, 67.5, 63.2, 63.1, 61.8, 60.6, 58.9, 50.5, 36.4, 35.1, 35.1, 35.0, 35.0, 35.0, 32.2, 31.9, 31.2, 31.1, 31.1, 31.1, 31.0, 30.9, 29.7, 29.7, 29.7, 29.6, 29.5, 29.5, 29.4, 29.3, 29.2, 29.0, 25.6, 22.7, 21.7, 14.1; HRMS (ESI) m/z: found [M+Na]+ 2878.6585, C172H234N2O33 calcd for [M+Na]+ 2878.6586.
Methyl 7-bromo-2,2-dichloro-1-heptanoate (29).
To a solution of 1,5-dibromopentane 28 (20.0 g, 87.0 mmol) in DMF (290 mL) were slowly added NaH (14.0 g, 348 mmol) and methyl dichloroacetate (36.0 mL, 348 mmol) in 4 portions (every 10 min) at 0 °C. After stirring for 2 h at 0 °C, as the reaction was monitored by TLC (n-hexane/EtOAc = 20
:
1), satd aq NH4Cl was added to the reaction mixture at 0 °C. The mixture was diluted with EtOAc and washed with H2O, satd aq Na2S2O3 and brine, dried over Na2SO4, and concentrated. The residue was purified by column chromatography on silica gel, using n-hexane/EtOAc (1
:
0 to 50
:
1) as the eluent, to give 29 (8.29 g, 32%): 1H NMR (500 MHz, CDCl3) δ 3.83 (s, 3 H, CO2CH3), 3.35 (t, 2 H, J6,7 = Jvic = 6.5 Hz, CH2Br), 2.37 (m, 2 H, CCl2CH2CH2), 1.83 (m, 2 H, CH2CH2Br), 1.57 (m, 2 H, CH2), 1.48 (m, 2 H, CH2); 13C NMR (125 MHz, CDCl3) δ 166.6, 84.3, 54.4, 44.9, 33.3, 32.3, 27.4, 24.3; HRMS (ESI) m/z: found [M+Na]+ 312.9364, C8H13BrCl2O2 calcd for [M+Na]+ 312.9368.
7-Bromo-2,2-dichloro-1-heptanol (30).
To a solution of 29 (139 mg, 476 μmol) in THF/MeOH (8.0 mL/1.6 mL) was added NaBH4 (54 mg, 1.43 mmol) at 0 °C. After stirring for 26 h at 0 °C, as the reaction was monitored by TLC (n-hexane/EtOAc = 5
:
1), satd aq NH4Cl was added to the reaction mixture at 0 °C. The mixture was diluted with EtOAc and washed with H2O and brine, dried over Na2SO4, and concentrated. The residue was purified by column chromatography on silica gel, using n-hexane/EtOAc (1
:
0 to 50
:
1) as the eluent, to give 30 (117 mg, 93%): 1H NMR (500 MHz, CDCl3) δ 3.91 (d, 2 H, J1,OH = 8.0 Hz, CH2OH), 3.43 (t, 2 H, J6,7 = Jvic = 6.5 Hz, CH2Br), 2.31 (t, 1 H, OH), 2.25–2.22 (m, 2 H, CCl2CH2CH2), 1.92 (m, 2 H, CH2CH2Br), 1.73–1.66 (m, 2 H, CH2), 1.55–1.50 (m, 2 H, CH2); 13C NMR (125 MHz, CDCl3) δ 94.3, 72.2, 43.3, 33.5, 32.4, 27.5, 24.0; HRMS (ESI) m/z: found [M+Na]+ 284.9421, C7H13BrCl2O calcd for [M+Na]+ 284.9419.
Phenyl 5-(7-bromo-2′,2′-dichloro-1-heptyloxycarbamoyl)-3,5-dideoxy-2-thio-D-glycero-α-D-galacto-2-nonulopyranosylonic acid (31).
To a solution of 26 (316 mg, 541 μmol) in EtOH (11 mL) was added 10 M aq KOH (812 μL, 8.12 mmol) at room temperature. After stirring for 2 days under reflux as the reaction was monitored by TLC (CHCl3/MeOH/H2O = 5
:
4
:
1), the reaction mixture was neutralized with HCl (2 M solution in EtOH, 4.1 mL) to give a solution of compound 27. To a solution of 30 (641 mg, 2.43 mmol) in MeCN (11 mL) were added triethylamine (600 μL, 4.33 mmol) and di(N-succinimidyl)carbonate (622 mg, 2.43 mmol) at 0 °C. After stirring for 50 min at room temperature as the reaction was monitored by TLC (n-hexane/EtOAc = 2
:
1), the reaction mixture was added a solution of compound 27 at 0 °C. After stirring for 2 h at room temperature as the reaction was monitored by TLC (CHCl3/MeOH/H2O = 5
:
2
:
0.2), the reaction mixture was diluted with EtOAc and washed with 2 M HCl, H2O, satd aq NaHCO3 and brine, dried over Na2SO4, and concentrated. The residue was purified by column chromatography on silica gel using CHCl3/MeOH (20
:
1 to 5
:
1) as the eluent and gel filtration column chromatography on Sephadex LH-20 using CHCl3/MeOH (1
:
1) as the eluent to give 31 (291 mg, 83%, two steps): [α]D +53.9 (c 1.5, MeOH); 1H NMR (500 MHz, CD3OD) δ 7.60–7.32 (m, 5 H, Ar), 4.54 (d, 1 H, Jgem = 11.5 Hz, NH(CO)OCH2), 4.45 (d, 1 H, NH(CO)OCH2), 3.80–3.76 (m, 2 H, H-8, H-9a), 3.68 (td, 1 H, J3eq,4 = 5.0 Hz, J3ax,4 = J4,5 = 11.0 Hz, H-4), 3.61–3.55 (m, 3 H, H-5, H-7, H-9b), 3.49–3.44 (m, 3 H, H-6, CH2Br), 2.86 (dd, 1 H, Jgem = 12.5 Hz, H-3eq), 2.25–2.22 (m, 2 H, CCl2CH2CH2), 1.91–1.83 (m, 3 H, H-3ax, CH2), 1.72–1.66 (m, 2 H, CH2), 1.54–1.48 (m, 2 H, CH2); 13C NMR (125 MHz, CD3OD) δ 159.0, 158.4, 137.6, 130.8, 129.7, 91.6, 77.2, 73.5, 72.6, 70.2, 69.3, 64.5, 55.0, 49.7, 49.5, 49.3, 44.9, 42.1, 34.1, 33.6, 28.5, 26.3, 25.1; HRMS (ESI) m/z: found [M–H]− 646.0289, C23H32BrCl2NO9S calcd for [M–H]− 646.0285.
Phenyl 5-(2′,2′-dichloro-7′-hydroxyheptoxycarbamoyl)-3,5-dideoxy-2-thio-D-glycero-α-D-galacto-2-nonulopyranosidono-1,7′-lactone (32).
To a solution of 31 (2.00 g, 3.08 mmol) in DMF/THF (1.16 L/385 mL) were added Drierite (77 g) and K2CO3 (831 mg, 6.16 mmol) at room temperature. After stirring for 5.5 h at 50 °C, as the reaction was monitored by TLC (CHCl3/MeOH = 8
:
1), the reaction mixture was filtered through a pad of Celite, and the pad was washed with EtOAc. The combined filtrate and washings were neutralized with AcOH and concentrated. The resulting residue was purified by flash column chromatography on silica gel using CHCl3/MeOH (20
:
1) as the eluent, to give 32 (1.00 g, 57%): [α]D −6.7 (c 0.6, MeOH); 1H NMR (500 MHz, CD3NO2, 90 °C) δ 7.61–7.59 (m, 2 H, Ar), 7.49 (m, 1 H, Ar), 7.45–7.41 (m, 2 H, Ar), 5.50 (d, 1 H, JNH,5 = 6.1 Hz, NH), 4.77 (d, 1 H, Jgem = 12.3 Hz, NH(CO)OCH2), 4.45 (d, 1 H, NH(CO)OCH2), 4.41–4.29 (m, 2 H, H-4, CO2CH2), 4.17 (d, 1 H, J5,6 = 10.4 Hz, H-6), 4.11 (m, 1 H, CO2CH2), 3.81–3.69 (m, 4 H, H-7, H-8, H-9a, H-9b), 3.17 (td, 1 H, J4,5 = 10.3 Hz, H-5), 3.07 (d, 1 H, OH-8), 3.00 (dd, 1 H, J3eq,4 = 4.9 Hz, Jgem = 12.9 Hz, H-3eq), 2.88 (br s, 1 H, OH-4), 2.68 (d, 1 H, J7,OH = 7.5 Hz, OH-7), 2.34–2.31 (m, 3 H, OH-9, CCl2CH2CH2), 1.93–1.48 (m, 7 H, H-3ax, 3 CH2); 13C NMR (125 MHz, CD3CN, 60 °C) δ 170.5, 155.7, 137.9, 131.2, 130.2, 130.0, 91.2, 88.0, 75.8, 73.2, 73.1, 71.0, 70.9, 70.6, 66.6, 66.5, 65.8, 64.9, 56.9, 50.1, 46.6, 42.5, 27.5, 26.4, 26.3, 25.6, 23.6; HRMS (ESI) m/z: found [M+Na]+ 590.0989, C23H31Cl2NO9S calcd for [M+Na]+ 590.0989.
Phenyl 5-(2′,2′-dichloro-7′-hydroxyheptoxycarbamoyl)-3,5-dideoxy-2-thio-9-O-p-toluenesulfonyl-D-glycero-α-D-galacto-2-nonulopyranosidono-1,7′-lactone (33).
To a solution of 32 (274 mg, 481 μmol) in CH2Cl2/pyridine (9.7 mL/9.7 mL) was added p-toluenesulfonyl chloride (440 mg, 2.31 mmol) in 4 portions at −20 °C. After stirring for 2 days at −20 °C, as the reaction was monitored by TLC (CHCl3/MeOH = 8
:
1), MeOH was added to the reaction mixture at −20 °C. The mixture was co-evaporated with toluene and diluted with EtOAc and washed with 2 M HCl, H2O, satd aq NaHCO3 and brine, dried over Na2SO4, and concentrated. The residue was purified by column chromatography on silica gel, using CHCl3/MeOH (70
:
1) as the eluent, to give 33 (270 mg, 78%): [α]D +9.6 (c 0.6, MeOH); 1H NMR (500 MHz, CD3CN, 60 °C) δ 7.81–7.80 (m, 2 H, Ar), 7.51–7.49 (m, 2 H, Ar), 7.45–7.42 (m, 3 H, Ar), 7.36–7.33 (m, 2 H, Ar), 5.64 (br s, 1 H, NH), 4.67 (d, 1 H, Jgem = 12.3 Hz, NH(CO)OCH2), 4.35 (d, 1 H, NH(CO)OCH2), 4.28 (d, 1 H, Jgem = 10.4 Hz, H-9a), 4.18–4.15 (m, 2 H, H-4, CO2CH2), 4.11 (dd, 1 H, J8,9b = 6.1 Hz, H-9b), 4.01–3.98 (m, 2 H, H-6, CO2CH2), 3.84 (m, 1 H, H-8), 3.54 (t, 1 H, J7,8 = J7,OH = 8.9 Hz, H-7), 3.36 (br d, 1 H, OH-4), 3.13 (br s, 1 H, OH-8), 2.99–2.93 (m, 2 H, H-5, OH-7), 2.85 (dd, 1 H, J3eq,4 = 4.7 Hz, Jgem = 12.8 Hz, H-3eq), 2.45 (s, 3 H, CH3), 2.21 (m, 2 H, CCl2CH2CH2), 1.81–1.38 (m, 7 H, H-3ax, 3 CH2); 13C NMR (125 MHz, CD3CN, 60 °C) δ 170.5, 146.5, 137.9, 134.6, 131.3, 131.2, 130.2, 130.0, 129.0, 118.2, 91.2, 88.0, 75.4, 73.4, 71.4, 70.1, 66.5, 66.0, 56.8, 46.6, 42.5, 27.6, 25.7, 23.7, 21.8; HRMS (ESI) m/z: found [M+Na]+ 744.1073, C30H37Cl2NO11S2 calcd for [M+Na]+ 744.1077.
Phenyl 9-azido-5-(2′,2′-dichloro-7′-hydroxyheptoxycarbamoyl)-3,5,9-trideoxy-2-thio-D-glycero-α-D-galacto-2-nonulopyranosidono-1,7′-lactone (34).
To a solution of 33 (139 mg, 192 μmol) in DMF (7.7 mL) were added NaN3 (50.0 mg, 769 μmol) and 15-crown-5 (38 μL, 192 μmol) at room temperature. After stirring for 2.5 h at 70 °C, as the reaction was monitored by TLC (CHCl3/MeOH = 7
:
1, developed twice), the mixture was diluted with EtOAc and washed with H2O and brine, dried over Na2SO4, and concentrated. The residue was purified by column chromatography on silica gel, using CHCl3/MeOH (70
:
1) as the eluent, to give 34 (99.9 mg, 88%): [α]D −6.3 (c 1.5, MeOH); 1H NMR (500 MHz, CD3NO2, 90 °C) δ 7.63–7.58 (m, 2 H, Ar), 7.52–7.49 (m, 3 H, Ar), 5.57 (d, 1 H, JNH,5 = 6.5 Hz, NH), 4.78 (d, 1 H, Jgem = 12.3 Hz, NH(CO)OCH2), 4.46 (d, 1 H, NH(CO)OCH2), 4.41–4.35 (m, 2 H, H-4, CO2CH2), 4.15 (d, 1 H, J5,6 = 10.3 Hz, H-6), 4.10 (m, 1 H, CO2CH2), 3.95 (m, 1 H, H-8), 3.72 (m, 1 H, H-7), 3.56 (dd, 1 H, J8,9a = 2.6 Hz, Jgem = 12.9 Hz, H-9a), 3.40 (dd, 1 H, J8,9b = 6.8 Hz, H-9b), 3.23 (d, 1 H, J8,OH = 4.3 Hz, 8-OH), 3.26 (td, 1 H, J4,5 = 10.4 Hz, H-5), 3.01 (dd, 1 H, J3eq,4 = 4.9 Hz, Jgem = 13.0 Hz, H-3eq), 2.98 (d, 1 H, J4,OH = 4.7 Hz, 4-OH), 2.71 (d, 1 H, J7,OH = 9.6 Hz, 7-OH), 2.32 (m, 1 H, CCl2CH2CH2), 1.90–1.49 (m, 7 H, H-3ax, 3 CH2); 13C NMR (125 MHz, CD3CN, 60 °C) δ 170.6, 155.6, 138.0, 137.7, 131.4, 130.2, 130.0, 91.2, 88.0, 79.3, 75.6, 73.0, 71.2, 70.6, 66.6, 66.1, 56.9, 54.9, 46.7, 42.5, 27.7, 25.7, 23.7; HRMS (ESI) m/z: found [M+Na]+ 615.1051, C23H30Cl2N4O8S calcd for [M+Na]+ 615.1054.
Phenyl 5-(2′,2′-dichloro-7′-hydroxyheptoxycarbamoyl)-3,5,9-trideoxy-2-thio-9-trifluoroacetamido-D-glycero-α-D-galacto-2-nonulopyranosidono-1,7′-lactone (35).
To a solution of 34 (390 mg, 657 μmol) in THF/H2O (39.4 mL/4.4 mL) was added PPh3 (190 mg, 723 μmol) at room temperature. After stirring for 36 h at 40 °C, PPh3 (138 mg, 526 μmol) and H2O (1.5 mL) were added at room temperature. After stirring for 24 h at 40 °C, as the reaction was monitored by TLC (CHCl3/MeOH = 7
:
1, CHCl3/MeOH/AcOH = 3
:
1
:
0.02), the mixture was concentrated and exposed to high vacuum for 24 h. To the resulting residue in pyridine (13.1 mL) was added (TFAc)2O (1.8 mL, 13.1 mmol) at 0 °C. After stirring for 3.5 h at room temperature, as the reaction was monitored by TLC (CHCl3/MeOH = 7
:
1, CHCl3/MeOH/AcOH = 3
:
1
:
0.02), H2O was added to the reaction mixture at room temperature. After stirring for 30 min at 40 °C, the solution was co-evaporated with toluene. The mixture was diluted with EtOAc and washed with 2 M HCl, H2O, satd aq NaHCO3 and brine, dried over Na2SO4, and concentrated. The residue was purified by column chromatography on silica gel, using CHCl3/MeOH (60
:
1) as the eluent, to give 35 (310 mg, 71%, two steps): [α]D +30.1 (c 0.7, MeOH); 1H NMR (500 MHz, CD3NO2, 90 °C) δ 7.60–7.57 (m, 2 H, Ar), 7.49 (m, 1 H, Ar), 7.44–7.40 (m, 2 H, Ar), 7.13 (br s, 1 H, NH-9), 5.53 (d, 1 H, JNH,5 = 6.6 Hz, NH-5), 4.76 (d, 1 H, Jgem = 12.3 Hz, NH(CO)OCH2), 4.45 (d, 1 H, NH(CO)OCH2), 4.43–4.36 (m, 2 H, H-4, CO2CH2), 4.16–4.08 (m, 2 H, H-6, CO2CH2), 3.88 (m, 1 H, H-8), 3.79 (m, 1 H, J8,9a = 3.5 Hz, JNH,9a = 5.9 Hz, Jgem = 13.7 Hz, H-9a), 3.66 (td, 1 H, J6,7 = 1.8 Hz, J7,8 = J7,OH = 8.8 Hz, H-7), 3.45 (m, 1 H, H-9b), 3.32 (d, 1 H, J8,OH = 4.1 Hz, OH-8), 3.18 (td, 1 H, J4,5 = J5,6 = 10.3 Hz, H-5), 3.01 (dd, 1 H, J3eq,4 = 4.9 Hz, Jgem = 13.0 Hz, H-3eq), 2.94 (d, 1 H, J4,OH = 4.5 Hz, OH-4), 2.81 (d, 1 H, J7,OH = 9.2 Hz, OH-7), 2.33–2.30 (m, 2 H, CCl2CH2CH2), 1.94–1.48 (m, 7 H, H-3ax, 3 CH2); 13C NMR (125 MHz, CD3CN, 60 °C) δ 170.7, 158.3 (q, 2JC,F = 36.1 Hz, C(O)CF3), 155.5, 138,0, 137.8, 131.3, 130.1, 130.0, 130.0, 117.5 (q, 1JC,F = 285.7 Hz, C(O)CF3), 91.2, 87.8, 79.3, 75.8, 75.6, 72.7, 72.0, 71.7, 71.3, 71.1, 70.6, 68.5, 67.5, 66.7, 66.0, 56.8, 54.1, 46.6, 44.4, 44.0, 42.5, 41.8, 31.0, 30.5, 30.2, 29.1, 27.7, 26.3, 25.7, 25.5, 23.7, 23.5, 14.5; HRMS (ESI) m/z: found [M+Na]+ 685.0976, C25H31Cl2F3N2O9S calcd for [M+Na]+ 685.0972.
Phenyl 4,7,8-tri-O-acetyl-5-(2′,2′-dichloro-7′-hydroxyheptoxycarbamoyl)-3,5,9-trideoxy-2-thio-9-trifluoroacetamido-D-glycero-α-D-galacto-2-nonulopyranosidono-1,7′-lactone (36).
To a solution of 35 (376 mg, 567 μmol) in pyridine (18.9 mL) was added Ac2O (3.8 mL, 40.2 mmol) at room temperature. After stirring for 25 h at room temperature, as the reaction was monitored by TLC (CHCl3/MeOH = 40
:
1), to the reaction mixture was added MeOH at 0 °C. The mixture was co-evaporated with toluene. The resulting residue was diluted with CHCl3 and washed with 2 M HCl, H2O, satd aq NaHCO3 and brine, dried over Na2SO4, and concentrated. The residue was purified by column chromatography on silica gel, using CHCl3/MeOH (70
:
1 to 40
:
1) as the eluent, to give 36 (450 mg, quant). Optical rotation, NMR data, and mass spectrometry data were reported.8
[4,7,8-Tri-O-acetyl-5-(2′,2′-dichloro-7′-hydroxyheptoxycarbamoyl)-3,5,9-trideoxy-9-trifluoroacetamido-D-glycero-α-D-galacto-2-nonulopyranosidono-1,7′-lactone]-(2→3)-(2,6-di-O-p-tert-butylbenzoyl-β-D-galactopyranosyl)-(1→3)-(2-acetamido-4,6-di-O-p-tert-butylbenzoyl-2-deoxy-β-D-glucopyranosyl)-(1→3)-(2,4,6-tri-O-p-tert-butylbenzoyl-β-D-galactopyranosyl)-(1→4)-(3-O-acetyl-2,6-di-O-p-tert-butylbenzoyl-β-D-glucopyranosyl)-(1→1)-(2S,3R,4E)-3-O-p-tert-butylbenzoyl-2-octadecanamido-4-octadecene-1,3-diol (37).
Sialyl donor 4 (52.9 mg, 55.3 μmol) and Lc4Cer acceptor 25 (35.1 mg, 12.3 μmol) were dissolved in CH2Cl2 (490 μL) at room temperature. TMSOTf (13.0 μL, 73.8 μmol) was added to the mixture at −70 °C. The reaction mixture was stirred for 5 h at −70 °C as the reaction was monitored by TLC (toluene/acetone = 6
:
1 developed twice, toluene/EtOAc = 2
:
1). The reaction mixture was quenched with satd aq NaHCO3, diluted with CHCl3 and washed with NaHCO3 and brine, dried over Na2SO4, and concentrated. The resulting residue was purified by column chromatography on silica gel using n-hexane/acetone (5.5
:
1) as the eluent and gel filtration column chromatography on Sephadex LH-20 using CHCl3/MeOH (1
:
1) as the eluent to give 37 (35.6 mg, 82%): [α]D +19.3 (c 0.9, CHCl3); 1H NMR (500 MHz, CD3NO2, 90 °C) δ 8.04–7.92 (m, 16 H, Ar), 7.90–7.88 (m, 2 H, Ar), 7.84–7.82 (m, 2 H, Ar), 7.61–7.49 (m, 16 H, Ar), 7.45–7.43 (m, 2 H, Ar), 7.41–7.39 (m, 2 H, Ar), 7.10 (br s, 1 H, NH-9e), 6.01 (d, 1 H, J3,4 = 2.3 Hz, H-4b), 5.76–5.74 (m, 2 H, NHCer, H-5Cer), 5.68 (t, 1 H, J1,2 = J2,3 = 8.9 Hz, H-2b), 5.59 (br s, 1 H, NHc), 5.48–5.46 (m, 2 H, H-3Cer, H-4Cer), 5.34–5.31 (m, 3 H, H-4e, H-8e, NH-5e), 5.24 (d, 1 H, J7,8 = 8.2 Hz, H-7e), 5.20 (br d, 1 H, H-1c), 5.15–5.12 (m, 2 H, H-2a, H-4c), 5.05–5.02 (m, 2 H, H-1b, H-2d), 4.78 (dd, 1 H, J5,6a = 3.0 Hz, Jgem = 11.8 Hz, H-6ad), 4.74–4.71 (m, 3 H, H-1a, H-3c, H-1d), 4.53–4.28 (m, 11 H, OH-3a, H-6aa, H-3b, H-6ac, H-3d, H-5d, H-6e, NH(CO)OCH2e, CO2CH2e, H-2Cer), 4.24–4.19 (m, 3 H, H-5a, H-6bc, H-6bd), 4.09–3.99 (m, 4 H, H-3a, H-6ab, H-5c, H-1aCer), 3.87–3.78 (m, 6 H, H-4a, H-6ba, H-6bb, H-4d, CO2CH2e, H-9ae), 3.70–3.66 (m, 2 H, H-1bCer, H-5b), 3.29 (br d, 1 H, H-9be), 3.18 (br d, 1 H, H-2c), 2.82 (m, 1 H, H-5e), 2.65 (br d, 1 H, H-3eqe), 2.58 (br d, 1 H, OH-4d), 2.36 (m, 1 H, CCl2CH2CH2e), 2.21 (m, 1 H, CCl2CH2CH2e), 2.02–1.85 (m, 11 H, 3 Ac, H-6aCer, H-6bCer), 1.68–1.25 (m, 151 H, H-3axe, 3 CH2e, COCH2Cer, 10 t-Bu, 26 CH2Cer), 1.14 (s, 3 H, Ac), 0.94–0.91 (m, 6 H, 2 CH3Cer); 13C NMR (125 MHz, CD3NO2, 90 °C) δ 172.9, 172.2, 171.6, 169.7, 168.0, 167.9, 167.6, 167.4, 167.3, 167.3, 167.1, 167.1, 167.0, 167.0, 159.2, 159.2, 159.2 (q, 2JC,F = 36.1 Hz, C(O)CF3), 159.1, 159.0, 158.9, 158.8, 156.0, 137.9, 131.5, 131.4, 131.3, 131.2, 131.2, 131.1, 131.1, 131.0, 129.7, 129.3, 129.3, 129.3, 129.2, 129.1, 128.7, 128.5, 127.3, 127.2, 127.1, 127.1, 127.0, 126.7, 117.9 (q, 1JC,F = 285.5 Hz, C(O)CF3), 104.1, 102.8, 102.7, 99.7, 91.7, 84.1, 79.8, 76.2, 75.7, 75.4, 75.2, 75.1, 74.9, 74.6, 74.2, 73.6, 72.8, 72.4, 72.0, 71.8, 70.6, 70.5, 69.3, 66.8, 65.0, 64.4, 54.6, 46.6, 38.4, 37.8, 36.5, 36.5, 36.5, 36.5, 36.4, 36.4, 33.5, 33.4, 33.3, 32.1, 32.1, 32.0, 32.0, 32.0, 32.0, 31.9, 31.9, 31.1, 31.0, 30.9, 30.9, 30.8, 30.7, 30.7, 30.7, 30.5, 30.5, 28.4, 27.1, 25.8, 24.2, 24.0, 24.0, 21.7, 21.3, 21.1, 14.7, 14.7; HRMS (ESI) m/z: found [M+2Na]2+ 1789.8840, C197H265Cl2F3N4O45 calcd for [M+2Na]2+ 1789.8842.
(2-Chloro-1-heptenyl 5-acetoamido-4,7,8-tri-O-acetyl-3,5,9-trideoxy-9-trifluoroacetamido-D-glycero-α-D-galacto-2-nonulopyranosylonate)-(2→3)-(4-O-acetyl-2,6-di-O-p-tert-butylbenzoyl-β-D-galactopyranosyl)-(1→3)-(2-acetamido-4,6-di-O-p-tert-butylbenzoyl-2-deoxy-β-D-glucopyranosyl)-(1→3)-(2,4,6-tri-O-p-tert-butylbenzoyl-β-D-galactopyranosyl)-(1→4)-(3-O-acetyl-2,6-di-O-p-tert-butylbenzoyl-β-D-glucopyranosyl)-(1→1)-(2S,3R,4E)-3-O-p-tert-butylbenzoyl-2-octadecanamido-4-octadecene-1,3-diol (38).
To a solution of 37 (28.5 mg, 8.06 μmol) in AcOH (400 μL) was added Zn nanopowder (421 mg, 6.46 mmol) at room temperature. The vial was sealed and irradiated by microwave for 10 min for three times (total 30 min) under magnetic stirring at 40 °C. Then Zn nanopowder (421 mg, 6.46 mmol) and AcOH (1.2 mL) were added in 2 portions at room temperature. The vial was sealed and irradiated by microwave for eight times (total 170 min) under magnetic stirring at 40 °C, as the reaction was monitored by TLC (toluene/EtOAc = 1
:
1). The reaction mixture was filtered through a pad of Celite, and the pad was washed with CHCl3. The combined filtrate and washings were diluted with CHCl3, and washed with satd aq NaHCO3 and brine, dried over Na2SO4, and concentrated. The residue was exposed to high vacuum for 10 min. To the resulting residue in pyridine (400 μL) were added Ac2O (5.3 μL, 56 μmol) and DMAP (1.0 mg, 8.1 μmol) at 0 °C. After stirring for 15 h at room temperature, as the reaction was monitored by TLC (toluene/EtOAc = 2
:
1), MeOH was added to the reaction mixture at 0 °C, and the solution was co-evaporated with toluene. The mixture was diluted with CHCl3 and washed with 2 M HCl, H2O, satd aq NaHCO3 and brine, dried over Na2SO4, and concentrated. The residue was purified by column chromatography on silica gel, using n-hexane/acetone (5
:
1 to 3
:
1) as the eluent, to give 38 (19.8 mg, 69%, two steps): [α]D +20.0 (c 0.7, CHCl3); 1H NMR (500 MHz, CD3CN, 60 °C) δ 7.99–7.94 (m, 4 H, Ar), 7.92–7.84 (m, 12 H, Ar), 7.82–7.77 (m, 4 H, Ar), 7.55–7.52 (m, 6 H, Ar), 7.50–7.37 (m, 14 H, Ar, NH-9e), 5.89–5.85 (m, 3 H, NHc, NH-5e, NHCer), 5.83 (d, 1 H, J3,4 = 3.2 Hz, H-4b), 5.61 (m, 1 H, H-5Cer), 5.39–5.33 (m, 4 H, H-3a, H-2b, H-3Cer, H-4Cer), 5.22 (m, 1 H, H-8e), 5.14 (d, 1 H, Jgem = 1.1 Hz, CH2
C(Cl)CH2), 5.11 (d, 1 H, CH2
C(Cl)CH2), 5.07 (dd, 1 H, J1,2 = 8.0 Hz, J2,3 = 9.7 Hz, H-2a), 5.00–4.95 (m, 3 H, H-4c, H-4d, H-7e), 4.88 (d, 1 H, J1,2 = 8.3 Hz, H-1c), 4.83 (dd, 1 H, J1,2 = 8.0 Hz, J2,3 = 10.2 Hz, H-2d), 4.76 (d, 1 H, J1,2 = 8.0 Hz, H-1b), 4.74–4.69 (m, 2 H, H-1a, H-4e), 4.61 (d, 1 H, H-1d), 4.52 (dd, 1 H, J3,4 = 3.2 Hz, H-3d), 4.45–4.41 (m, 2 H, H-6ac, H-6ad), 4.38–4.24 (m, 4 H, H-6aa, H-3c, H-6bc, H-2Cer), 4.21 (dd, 1 H, J2,3 = 10.0 Hz, H-3b), 4.16–4.02 (m, 4 H, H-6ba, H-5d, H-6bd, CO2CH2e), 3.98 (t, 1 H, J3,4 = J4,5 = 9.5 Hz, H-4a), 3.92–3.78 (m, 4 H, H-5c, H-5e, H-1aCer, CO2CH2e), 3.75–3.71 (m, 2 H, H-5a, H-6ab), 3.66 (dd, 1 H, J6,7 = 2.2 Hz, J5,6 = 10.8 Hz, H-6e), 3.60–3.47 (m, 4 H, H-5b, H-6bb, H-9ae, H-1bCer), 3.15 (m, 1 H, H-2c), 2.92 (td, 1 H, J8,9b = JNH,9b = 5.4 Hz, Jgem = 14.8 Hz, H-9be), 2.32–2.24 (m, 3 H, H-3eqe, CH2
C(Cl)CH2), 1.88–1.84 (m, 2 H, H-6aCer, H-6bCer), 1.82 (s, 3 H, Ac), 1.78 (s, 3 H, Ac), 1.76 (s, 3 H, Ac), 1.74 (s, 3 H, Ac), 1.69 (s, 3 H, Ac), 1.60–1.02 (m, 151 H, H-3axe, 10 t-Bu, 3 CH2, 27 CH2Cer), 0.91–0.87 (m, 9 H, Ac, 2 CH3Cer); 13C NMR (125 MHz, CDCl3) δ 172.5 (COCer), 172.4 (NHAce), 171.0 (Acc), 170.6 (Ac), 170.2 (Ac), 169.9 (Ac), 169.9 (Ac), 169.7 (Ac), 167.2 (C-1e), 166.0, 166.0, 165.7, 165.0, 165.0, 164.9, 164.7, 164.6, 157.7 (q, 2JC,F = 37.8 Hz, C(O)CF3), 157.3, 157.2, 157.0, 156.9, 156.7, 156.6, 156.5, 156.3, 156.2, 142.5 (CH2
C(Cl)CH2), 136.8, 129.9, 129.8, 129.6, 129.5, 129.5, 129.4, 127.5, 127.4, 127.2, 127.1, 127.0, 126.7, 126.7, 126.6, 126.4, 126.2, 125.6, 125.5, 125.5, 125.3, 125.2, 125.2, 125.1, 124.8, 115.8 (q, 1JC,F = 286.3 Hz, C(O)CF3), 112.1 (CH2
C(Cl)CH2), 100.9 (C-1a, C-1b), 100.8 (C-1d), 99.4 (C-1c), 96.8 (C-2e), 78.6, 77.6, 75.6, 75.5, 73.8, 73.1, 72.0, 71.9, 71.8, 71.5, 71.3, 70.8, 70.6, 70.3, 70.0, 69.6, 69.5, 69.3, 67.6, 67.1, 67.1, 66.6, 66.4, 63.0, 62.3, 62.2, 60.2, 58.9, 50.4, 48.7, 38.7, 38.2, 37.0, 36.5 (C-3e), 35.1, 35.1, 35.1, 35.1, 35.1, 35.0, 35.0, 34.9, 33.7, 32.2, 31.9, 31.7, 31.2, 31.1, 31.0, 31.0, 30.8, 30.3, 30.1, 30.0, 29.7, 29.7, 29.6, 29.6, 29.5, 29.4, 29.3, 29.3, 29.2, 29.0, 27.7, 26.7, 26.5, 25.5, 24.6, 23.2, 23.0, 22.7, 21.8, 21.0, 20.8, 20.7, 20.4, 14.2, 14.1; HRMS (ESI) m/z: found [M+2Na]2+ 1813.9248, C202H272ClF3N4O46 calcd for [M+2Na]2+ 1813.9247.
Cells, cell culture, cDNA construction
To investigate whether ATTO594-NeuAcLc4Cer and ATTO594-Lc4Cer are partitioned into detergent-resistant membrane (DRM) fraction, T24 cells were used as previously reported.5,7,8 T24 cells were cultured in HAM's F12 medium (Gibco), supplemented with 10% FBS. To examine partitioning of ATTO594-NeuAcLc4Cer and ATTO594-Lc4Cer into liquid-order (Lo) like and liquid-disorder (Ld) like phases in phase-separated giant plasma membrane vesicles (GUVs), RBL-2H3 cells were used as previously reported.5,7,8 RBL-2H3 cells were maintained in Eagle's minimal essential medium (Sigma; M4655), supplemented with 1 mM sodium pyruvate (Sigma; S8636), 0.1 mM MEM non-essential amino acids (Wako; 139-15651) and 15% fetal bovine serum (Sigma; F2442). For the observations of colocalization of Halo7-CD59 homodimers with single molecules of ATTO594-NeuAcLc4Cer and ATTO594-Lc4Cer, CHO-K1 cells were used. CHO-K1 cells were cultured in HAM's F21 medium (Gibco), supplemented with 10% FBS. CHO-K1 cells were transfected with the cDNA encoding Halo7-CD59 by using 4-D nucleofector (LONZA), according to manufacturer's recommendations. The cDNA sequence encoding Halo7-CD59 was placed in the Epstein-Barr virus (EBV)-based episomal vector pOsTet15T3, which carries the tetracycline-regulated expression units, the transactivator (rtTA2-M2), and the TetO sequence (a Tet-on vector). The signal sequence of CD59 was fused to the N-terminus of Halo7-CD59, and the linker sequence 5′-GAAGCGGCCGCGACTAAGCTTGCCATGGAGCAGAAACTCATCT CTGAAGAGGATCTG-3′ was inserted between Halo7 and CD59. These cells were seeded in glass-base dishes (35 mm ∅ with a glass window of 12 mm ∅, 0.15 mm-thick glass; Iwaki) and grown for a day, and then incubated with 500 ng mL−1 (final concentration) doxycycline for an additional day.31 To fluorescently label Halo7-CD59, CHO-K1 cells were incubated with 50 nM Rhodamine110 (R110) halo ligand (Promega) in HAM's F12 medium containing 10% FBS for 30 min, and washed. Under these conditions, most of the Halo7-CD59 molecules were labeled with R110 as previously reported.31
Evaluation of cold Triton X-100 insolubility.
For the epi-fluorescence imaging of ATTO594-NeuAcLc4Cer and ATTO594-Lc4Cer incorporated in cell plasma membranes (PMs), the fluorescent analogs were first dispersed in HBSS (Nissui), buffered with 2 mM PIPES (Dojindo) at pH 7.4 (P-HBSS) at a final concentration of 1.0 μM, and the dispersed solution was incubated with T24 cells on a glass-base dish (Iwaki) at 22 °C for 15 min. Then, the cells were chilled on ice-water (2.8 °C), washed three times with pre-chilled P-HBSS, and incubated in pre-chilled P-HBSS containing 1% (v/v) Triton X-100 on ice-water for 15 min.28 Then, the cells were washed three times with cold P-HBSS, washed twice with pre-chilled PBS, fixed with 4% paraformaldehyde in PBS for 90 min, and observed under a Nikon Ts-2FL epi-fluorescence microscope equipped with a 60× 1.40 NA oil objective and a high-sensitive CMOS sensor-based camera (Nikon, DS-Qi2).
Formation and microscopic observation of GPMVs containing Lo- and Ld-like phases
RBL-2H3 cells grown on a glass-base dish (Iwaki) for 2 days were washed twice with P-HBSS. For the incorporation of ATTO594-NeuAcLc4Cer and ATTO594-Lc4Cer into the PMs, first, a 2 μL of the methanol stock solution of a fluorescent ganglioside analog (1 mM) was injected into 200 μL P-HBSS, and after vigorous vortexing for 1 min, the mixture was sonicated for 5 min. Subsequently, 0.1 mL of this suspension (final analog concentration of 10 μM) was overlaid on the cells in each glass-base dish, followed by an incubation at 22 °C for 12 min. To mark the Ld-like domains, Bodipy-FL-PC was incorporated in the PMs as well.5,7,8
After washing the cells with P-HBSS three times, the membrane blebs were induced by incubating the cells in 25 mM formaldehyde and 2 mM dithiothreitol (DTT) in P-HBSS, at 37 °C for 1 h.33 During this incubation time, numerous blebs were generated and then detached from the cells to form giant plasma membrane vesicles (GPMVs). The dish was moved onto the microscope stage of a total internal reflection fluorescence (TIRF) microscope, based on an Olympus IX-83 with a 100× 1.40 NA oil objective, quietly left at 20 °C for 15 min to let the GPMVs settle on the bottom glass, and then cooled by circulating a chilled water–ethanol solution (−5 °C) so that the temperature of the top surface of the glass (facing the P-HBSS solution) became stabilized at 10 °C. Under these conditions, the majority (>90%) of the GPMVs exhibited two coexisting domains: Ld-like domains preferentially concentrating Bodipy-FL-PC (Fig. 3),5,7,8 as simultaneously observed by oblique angle illumination with 488 and 594 nm laser beams introduced by an optical fiber through the rear port in our TIRF microscope. The focus was adjusted to collect the fluorescence signal emitted from the equatorial plane of the blebs. Images were simultaneously recorded with sCMOS cameras (ORCA-Flash4.0 V2; Hamamatsu Photonics) in two emission channels (520 and 630 nm), at a rate of 30 Hz.
Single-fluorescent-molecule tracking and dual-color observation
Single-fluorescent-molecule tracking was performed using a home-built, objective-lens-type, total internal reflection fluorescence (TIRF) microscope based on an Olympus IX-83 with a 100× 1.50 NA oil objective as described previously with slight modifications.5,31 The precisions of the position determinations for single stationary fluorescent probes were estimated from the standard deviations of the determined coordinates of the probes fixed on coverslips. For the R110 and ATTO594 probes recorded at a video rate, the localization precisions of single molecules were ±18 and ±12 nm, respectively. Statistical analysis for the distributions of colocalization durations was performed using the log-rank test (statistical survival analysis). Dual-color single-molecule images were simultaneously recorded with sCMOS cameras (ORCA-Flash4.0 V2; Hamamatsu Photonics) in two emission channels (520 and 630 nm) at a video rate. The distance between the two molecules was directly measured from the coordinates (x, y-positions) of each molecule, which can be determined simultaneously and independently in each image in different colors.5,31,34,35 Even when examining pairs of different-colored molecules that are known to be truly associated, the probability of scoring the two molecules as associated is limited by the localization accuracies of each molecule and accuracies of superimposing the two images. Based on the method developed previously5,31,35 and the determined position accuracies, we found that, for truly associated molecules, the probability of scoring the two molecules as associated increases to 99% using the criterion that the molecules lie within 240 nm of each other. Therefore, we used this criterion as the definition of colocalization of the two molecules in simultaneous dual-color single-molecule observations.
Author contributions
N. K., K. G. N. S. and H. A. conceived this research and designed the experiments. M. T., Y. Y., E. Y. and A. H. performed the chemical synthesis; K. G. N. S. performed the biophysical evaluation and single-molecule imaging; M. T., N. K., E. Y., H.-N. T., A. I., H. I., K. G. N. S. and H. A. analyzed the data; M. T., N. K., K. G. N. S. and H. A. wrote the original draft of the manuscript; all authors contributed to its reviewing and editing.
Conflicts of interest
There are no conflicts to declare.
Acknowledgements
This work was supported in part by KAKENHI Grant No. JP18H0392 (H. A.), JP21H02424 (K. G. N. S.), JP20K21387 (K. G. N. S.), JP18H04671 (K. G. N. S.), JP20K15412 (N. K.), and JP22J12660 (M. T.); JSPS Core-to-Core Program Grant No. JPJSCCA20200007 (H. A.); JST CREST Grant No. JRMJCR18H2 (K. G. N. S. and H. A.); JST FOREST Grant No. JPMJFR2004 (N. K.); the Takeda Science Foundation (K. G. N. S.); the Uehara Memorial Foundation (K. G. N. S.); the Mizutani Foundation for Glycoscience (K. G. N. S.), SUNBOR Grant from the Suntory Foundation for Life Sciences (N. K.). We thank Ms Shinobu Kawaguchi and Mr Toui Kawai for a cDNA plasmid of Halo7-CD59.
References
- I. Levental, K. R. Levental and F. A. Heberle, Trends Cell Biol., 2020, 30, 341–353 CrossRef CAS PubMed.
- T. Haselhorst, F. E. Fleming, J. C. Dyason, R. D. Hartnell, X. Yu, G. Holloway, K. Santegoets, M. J. Kiefel, H. Blanchard, B. S. Coulson and M. von Itzstein, Nat. Chem. Biol., 2009, 5, 91–93 CrossRef CAS PubMed.
- J. E. Stencel-Baerenwald, K. Reiss, D. M. Reiter, T. Stehle and T. S. Dermody, Nat. Rev. Microbiol., 2014, 12, 739–749 CrossRef CAS PubMed.
- N. Hiramoto-Yamaki, K. A.-K. Tanaka, K. G.-N. Suzuki, K. M. Hirosawa, M. S.-H. Miyahara, Z. Kalay, K. Tanaka, R. S. Kasai, A. Kusumi and T. K. Fujiwara, Traffic, 2014, 15, 583–612 CrossRef CAS PubMed.
- N. Komura, K. G.-N. Suzuki, H. Ando, M. Konishi, M. Koikeda, A. Imamura, R. Chadda, T. K. Fujiwara, H. Tsuboi, R. Sheng, W. Cho, K. Furukawa, Y. Yamaguchi, H. Ishida, A. Kusumi and M. Kiso, Nat. Chem. Biol., 2016, 12, 402–410 CrossRef CAS PubMed.
- M. Koikeda, N. Komura, H. N. Tanaka, A. Imamura, H. Ishida, M. Kiso and H. Ando, J. Carbohydr. Chem., 2019, 38, 509–527 CrossRef CAS.
- M. Konishi, N. Komura, Y. Hirose, Y. Suganuma, H. N. Tanaka, A. Imamura, H. Ishida, K. G.-N. Suzuki and H. Ando, J. Org. Chem., 2020, 85, 15998–16013 CrossRef CAS PubMed.
- S. Asano, R. Pal, H. N. Tanaka, A. Imamura, H. Ishida, K. G.-N. Suzuki and H. Ando, Int. J. Mol. Sci., 2019, 20, 6187 CrossRef CAS PubMed.
- J. Sibold, K. Kettelhoit, L. Vuong, F. Liu, D. B. Werz and C. Steinem, Angew. Chem., Int. Ed., 2019, 58, 17805–17813 CrossRef CAS PubMed.
- D. Lingwood and K. Simons, Science, 2010, 327, 46–50 CrossRef CAS PubMed.
- O. Nilsson, J. E. Månsson, L. Lindholm, J. Holmgren and L. Svennerholm, FEBS Lett., 1985, 182, 398–402 CrossRef CAS PubMed.
- S. Hakomori, Annu. Rev. Immunol., 1984, 2, 103–126 CrossRef CAS PubMed.
- Y. Hamanaka, S. Hamanaka, Y. Shinagawa, T. Suzuki, F. Inagaki, M. Suzuki and A. Suzuki, FEBS Lett., 1994, 353, 48–52 CrossRef CAS PubMed.
- C. J. Wikstrand, X. He, G. N. Fuller, S. H. Bigner, P. Fredman, L. Svennerholm and D. D. Bigner, J. Neuropathol. Exp. Neurol., 1991, 50, 756–769 CrossRef CAS PubMed.
- C. J. Wikstrand, D. C. Longee, R. E. McLendon, G. N. Fuller, H. S. Friedman, P. Fredman, L. Svennerholm and D. D. Bigner, Cancer Res., 1993, 53, 120–126 CAS.
- C. J. Wikstrand, P. Fredman, R. R. McLendon, L. Svennerholm and D. D. Bigner, Mol. Chem. Neuropathol., 1994, 21, 129–138 CrossRef CAS PubMed.
- K. Kaneko, Y. Ohkawa, N. Hashimoto, Y. Ohmi, N. Kotani, K. Honke, M. Ogawa, T. Okajima, K. Furukawa and K. Furukawa, J. Biol. Chem., 2016, 291, 16630–16643 CrossRef CAS PubMed.
- M. Takahashi, J. Shirasaki, N. Komura, K. Sasaki, H. N. Tanaka, A. Imamura, H. Ishida, S. Hanashima, M. Murata and H. Ando, Org. Biomol. Chem., 2020, 18, 2902–2913 RSC.
- H. Ando, Y. Koike, H. Ishida and M. Kiso, Tetrahedron Lett., 2003, 44, 6883–6886 CrossRef CAS.
- T. Fuse, H. Ando, A. Imamura, N. Sawada, H. Ishida, M. Kiso, T. Ando, S.-C. Li and Y.-T. Li, Glycoconjugate J., 2006, 23, 329–343 CrossRef CAS PubMed.
- N. Komura, K. G. N. Suzuki, H. Ando, M. Konishi, A. Imamura, H. Ishida, A. Kusumi and M. Kiso, Methods Enzymol., 2017, 597, 239–263 CAS.
- A. M. Vibhute, N. Komura, H. N. Tanaka, A. Imamura and H. Ando, Chem. Rec., 2021, 21, 1–31 CrossRef PubMed.
- N. Komura, K. Kato, T. Udagawa, S. Asano, H.-N. Tanaka, A. Imamura, H. Ishida, M. Kiso and H. Ando, Science, 2019, 364, 677–680 CrossRef CAS PubMed.
- S. Asano, H. N. Tanaka, A. Imamura, H. Ishida and H. Ando, Org. Lett., 2019, 21, 4197–4200 CrossRef CAS PubMed.
- A. Imamura, H. Ando, H. Ishida and M. Kiso, J. Org. Chem., 2009, 74, 3009–3023 CrossRef CAS PubMed.
- S. Nakashima, H. Ando, A. Imamura, N. Yuki, H. Ishida and M. Kiso, Chem. – Eur. J., 2011, 17, 588–597 CrossRef CAS PubMed.
- T. Nohara, A. Imamura, M. Yamaguchi, K. I.-P. J. Hidari, T. Suzuki, T. Komori, H. Ando, H. Ishida and M. Kiso, Molecules, 2012, 17, 9590–9620 CrossRef CAS PubMed.
- A. K. Kenworthy, B. J. Nichols, C. L. Remmert, G. M. Hendirix, M. Kumar, J. Zimmerberg and J. Lippincott-Schwartz, J. Cell Biol., 2004, 165, 735–746 CrossRef CAS PubMed.
-
(a) D. Lingwood, J. Ries, P. Schwille and K. Simons, Proc. Natl. Acad. Sci. U. S. A., 2008, 105, 10005–10010 CrossRef CAS PubMed;
(b) T. Baumgart, A. T. Hammond, P. Sengupta, S. T. Hess, D. A. Holowka, B. A. Baird and W. W. Webb, Proc. Natl. Acad. Sci. U. S. A., 2007, 104, 3165–3170 CrossRef CAS PubMed;
(c) I. Levental, M. Grzybek and K. Simons, Proc. Natl. Acad. Sci. U. S. A., 2011, 108, 11411–11416 CrossRef CAS PubMed.
- H. J. Kaiser, D. Lingwood, I. Levental, J. L. Sampaio, L. Kalvodova, L. Rajendran and K. Simons, Proc. Natl. Acad. Sci. U. S. A., 2009, 106, 16645–16650 CrossRef CAS PubMed.
- K. G.-N. Suzuki, R. S. Kasai, K. M. Hirosawa, Y. L. Nemoto, M. Ishibashi, Y. Miwa, T. K. Fujiwara and A. Kusumi, Nat. Chem. Biol., 2012, 8, 774–783 CrossRef CAS PubMed.
- K. G.-N. Suzuki, R. S. Kasai, T. K. Fujiwara and A. Kusumi, Methods Cell Biol., 2013, 117, 373–390 CAS.
- T. Baumgart, A. T. Hammond, P. Sengupta, S. T. Hess, D. A. Holowka, B. A. Baird and W. W. Webb, Proc. Natl. Acad. Sci. U. S. A., 2007, 104, 3165–3170 CrossRef CAS PubMed.
-
(a) K. G.-N. Suzuki, T. K. Fujiwara, F. Sanematsu, R. Iino, M. Edidin and A. Kusumi, J. Cell Biol., 2007, 177, 717–730 CrossRef CAS PubMed;
(b) K. G.-N. Suzuki, T. K. Fujiwara, M. Edidin and A. Kusumi, J. Cell Biol., 2007, 177, 731–742 CrossRef CAS PubMed.
- M. Kinoshita, K. G.-N. Suzuki, N. Matsumori, M. Takada, H. Ano, K. Morigaki, M. Abe, A. Makino, T. Kobayashi, K. M. Hirosawa, T. K. Fujiwara, A. Kusumi and M. Murata, J. Cell Biol., 2017, 216, 1183–1204 CrossRef CAS PubMed.
Footnote |
† Electronic supplementary information (ESI) available: 1H and 13C NMR spectra for all new compounds and synthesis of ATTO594-Lc4Cer. See DOI: https://doi.org/10.1039/d2cb00083k |
|
This journal is © The Royal Society of Chemistry 2022 |
Click here to see how this site uses Cookies. View our privacy policy here.