Treatments of wood ash amended biochar to reduce nutrient leaching and immobilise lead, copper, zinc and cadmium in aqueous solution: column experiments†
Received
29th December 2021
, Accepted 2nd May 2022
First published on 3rd May 2022
Abstract
The pollution of aqueous environments by metals has continued to increase due to anthropogenic activities such as mining, waste disposal, industrial activities and the use of motor vehicles. Globally, vehicle numbers are predicted to increase to 2.8 billion by 2050 with aqueous pollutants associated with vehicles, such as Pb, Cu, Zn and Cd, increasing alongside vehicle numbers. With these increases, methods to minimise metal pollution are important to find; one such method is wood ash amended biochar. Whilst biochar has the potential to reduce contaminated runoff, this study explores the potential for the biochar itself to leach nutrients (PO43−, SO42− and NO3−) which are constituent parts of its biomass and potentially harmful to the ecosystem the biochar would be deployed to remediate. Treatments such as sintering wood ash to the biochar, granulating the biochar and rinsing the biochar were studied to ascertain their impact on the retention of minerals key to immobilisation, the leaching of nutrients and the immobilisation of Pb, Cu, Zn and Cd. It was demonstrated that wood ash sintered larch biochar granulated <3 mm (WASGr) retained the highest concentration of minerals associated with immobilisation, reduced leaching of nutrients to below Water Framework Directive thresholds and maintained Pb, Cu, Zn and Cd immobilisation at 97–100% once rinsed with deionised water. As a result, WASGr rinsed with deionised water, has the potential to be scaled-up and deployed to immobilise Pb, Cu, Zn and Cd from motorway runoff without a negative impact on the concentration of nutrients in the surrounding waters.
Water impact
Motorway runoff is a growing source of metal pollutants. Wood ash amended biochar can be treated to reduce its leaching of minerals and nutrients associated with metal immobilisation and eutrophication. Sintering the wood ash to the biochar, granulating the biochar and rinsing unbound minerals and nutrients prepare the biochar for deployment in aqueous environments (e.g. balancing ponds) whilst not adversely affecting metal immobilisation.
|
Introduction
The pollution of aqueous environments by heavy metals has continued to increase considerably in recent years. Anthropogenic activities have been the principle cause of this rise through activities including, but not limited to, mining, waste disposal, industrial activities and the escalating use of motor vehicles. Globally, vehicle numbers are predicted to be as high as 2.8 billion by 2050, with an anticipated increase in road length of at least 25 million km.1,2 The pollution and significant ecological effect associated with this vast and growing infrastructure extends outwards even further into the adjacent landscape in an area described as the “road-effect zone”.3 The reach of this pollution is significant in the UK, with only 6% of land escaping its impact and 25% of land being within 80 m of a road.4
Vehicular and road pollution types can include heavy metals, particulate matter, NOx, polycyclic aromatic hydrocarbons (PAHs), road salts, light and noise.4,5 Of these pollutants, heavy metals are a group considered to be of great concern as they cannot be decomposed by micro-organisms and exhibit long term toxicity for plants, animals and humans.6 Road runoff also carries pollutants such as heavy metals into rivers, tributaries and lakes leading to the pollution of ecosystems. This pollution causes significant impact on aquatic life, enters the food chain and cause human exposure to these heavy metals.7,8 Such exposure has been shown to have a detrimental impact on health such as cancer, weakened immunological responses, impairment of neurological functions and cardiovascular disease.9
The most recognised metals in roadside environments include Pb, Cu, Zn and Cd; whilst there are other metals and metalloids of importance, such as Ni, Cr and Sb the metals Pb, Cu, Zn and Cd remain among the most examined.6 The vehicular pollutants found in road runoff, notably Pb, Cu, Zn and Cd, generally occur through abrasive forces or leaks such as tyre wear, brake wear and motor oil leaks.10–12 The concentrations of these pollutants are directly linked to traffic density;13 driving style, with more aggressive speeds and breaking resulting in greater brake and tyre wear;6 manufacturer and time of component production, with compositions of tyres and brakes varying considerably;12 vehicle type, with weight, engine power and state of maintenance being of import;14,15 and road composition.16 As a result of these factors Pb, Cu, Zn and Cd contamination is pervasive in the UK. Phillips et al.4 argue that the proportion of land in the UK affected by road pollution is as high as 6.86% for Zn, 7.09% for Cu, 14.50% for Cd and 18.29% for Pb.
Alongside the increase in inorganic vehicular pollutants anthropogenic sources of contamination such as metal mines, waste disposal and industrial activities are also considered major sources of metal pollution in aqueous environments.17–19 In Wales alone, there are over 1300 metal mines affecting over 700 km of river with primary contaminants including Pb and Zn.20,21 Waste disposal, particularly landfilling, also poses a significant pressure on the environment through the leaching of heavy metals such as Cd and Pb exerting toxic effects19 and industrial effluent is known to contain large quantities of hazardous metals, such as Pb, Cu, Zn and Cd, which are contaminating water resources.
With growing anthropogenic contamination, methods to minimise pollution which are environmentally stable are key. Regulatory measures to combat the impact of pollution, such as the ban on leaded petrol, are often negated by the significant and continuing growth in the sources of contamination such as worldwide traffic and road networks.6 As a result, novel engineered techniques have a role to play in tackling this pollution. Biochar is one material that has been studied to ascertain its potential as a novel remediator that could be scaled up to deal with contaminated runoff and other anthropogenic sources of contamination.22 Biochar is defined as a porous, carbonaceous material produced by biomass pyrolysis at temperatures ranging from 350–1000 °C under limited oxygen conditions.23 During pyrolysis, highly aromatic clusters are formed responsible for biochar's high chemical stability and porosity.24 The key functions of biochar include the ability to sequester carbon, improve soil fertility and remove environmental contaminants from aqueous media.25–27 Cairns et al.28 found that the removal from aqueous media of the key vehicular inorganic contaminants, Pb, Cu, Zn and Cd, by biochar increased by an order of magnitude with the addition of wood ash. Precipitation and ion exchange were found to be the dominant immobilisation mechanisms as a result of the increased pH and addition of minerals associated with the wood ash amendment.
Whilst biochar has the potential to reduce contaminated runoff, studies also need to explore the potential for the biochar itself to leach nutrients, such as phosphate (PO43−), sulphate (SO42−) and nitrate (NO3−), which are constituent parts of its biomass. The leaching of these nutrients could be harmful to the ecosystem the biochar would be deployed to remediate particularly in nutrient deficient (oligotrophic) ecosystems. The potential for biochar to leach nutrients such as N and P was recognised by Wu et al.29 who studied biochar from a feedstock of mallee wood and saw reductions in N and P proportions in the biochar as a result of exposure to water, indicating that leaching was occurring to some degree. Liang et al.30 also recognised the potential leaching of P from dairy manure biochar rich in nutrients and highlighted that this could pose a risk of surface and groundwater impairment if P release was too high. The potential loss of N and P as a result of biochars exposure to aqueous environments is also relevant to biochar amended with wood ash which is also rich with nutrients such as P, N and S. Whilst these nutrients aid immobilisation of metal contaminants and their chemical derivatives such as phosphates and nitrates are an important element in plant growth, their release in high levels is considered one of the key factors in eutrophication and poses risks to aquatic ecosystems.31,32 It is these concerns that have led to European Union (EU) directives such as the Nitrates Directive 91/676/EEC, Directive 91/271/EEC concerning urban waste-water treatment and the Water Framework Directive 2008/105/EC.
This study builds on previous work that demonstrates the effectiveness of wood ash amended biochar in the removal of Pb, Cu, Zn and Cd (ref. 28) to understand whether the leaching of P, N and S in the form of phosphates, nitrates and sulphates is apparent, and if so what treatment options are available to mitigate leaching this without reducing the immobilisation of Pb, Cu, Zn and Cd.
Methods
1. Biochar production and wood ash amendment
European larch (Larix decidua Mill.) wood chips were pyrolyzed in a Pyrocal BigChar-1000 pyrolysis-gasification kiln at a temperature of 485–530 °C, with a retention time of ∼90 s.28 Wood ash, purchased from NPK Ltd, was added to the biochar after pyrolysis via two alternative methods. For the first method, it was mixed with the freshly pyrolyzed, hot biochar at a ratio of 1
:
1 for 15 minutes in a cement mixer in order to sinter the materials (WAS). The sintering of the wood ash to the biochar was to promote particle bonding between the wood ash and the biochar to create a more stable matrix than when mixed cold. This was also designed to retain alkali and alkaline earth metals, P, N and S all of which are either relevant to contaminant immobilisation or nutrients that could be harmful to the wider ecosystem. For the second method the wood ash was mixed at a ratio of 1
:
1 with biochar that had been allowed to cool to ambient temperature for 15 minutes (WA). Wood ash was chosen as an amendment due to its high mineral and nutrient fraction, including Ca, K, Mg, S, P, N and Si (ESI 1†) and pH buffering capacity.27 Half of WAS was granulated to <3 mm with a Tria G1 granulator (WASGr) and half remained ungranulated (WAS). Similarly, half of WA was granulated to <3 mm (WAGr) and half remained ungranulated (WA).
2. Biochar characterisation
Total metal concentrations were determined using microwave assisted digestion. Approximately 20 mg of each sample of biochar were digested in the presence of 6 mL HNO3, 2 mL HCl and 0.5 mL HF. The samples were digested using a microwave (Speedwave4, Berghof Products + Instruments GmbH, Germany), ramped up to 220 °C for 30 minutes and then held at 220 °C for 30 minutes before cooling in a water bath. 200 mg of H3BO3 was then added as a complexation agent alongside 2 mL of H2O2 and held at 160 °C for 30 minutes. Deionised water was then added to bring the samples up to a volume of 50 mL. An aliquot of the sample was filtered through a 0.45 μm cellulose acetate filter. Metal concentrations were measured in triplicate using inductively coupled plasma-optical emission spectroscopy (ICP-OES 5110, Agilent Technologies Inc., USA).
Total carbon, hydrogen, nitrogen and sulphur contents were determined in triplicate by elemental analyses (Vario MACRO CHNS elemental analyzer, Elementar Analysensysteme GmbH, Germany). Furnace temperatures were ramped up by 2 °C per minute and then a dwell time of 750 °C was maintained for 6 hours. Ash content was determined by heating the biochars in a muffle furnace. Total oxygen was calculated from C, H, N, S and ash content via mass balance.23
3. Biochar rinsing
Each biochar treatment was rinsed with deionised water to quantify the concentration of PO43−, SO42− and nitrate NO3− leached from the biochar. Using a sieve, sand was washed with 1% HCl, rinsed with deionised water and dried. Each treatment (5 g of WA, WAGr, WAS and WASGr) was added to an open-ended polypropylene column with an internal diameter of 31 mm, a wall thickness of 1 mm and a length of 500 mm. The acid washed sand (8 g; 1% HCl) was placed at the top and bottom of each column to help distribute the flow of deionised water and prevent loss of biochar. An 80 μm nylon mesh was used to stabilise the column content and further prevent loss of biochar. Each treatment was rinsed by pumping deionised water downward through the column at flow rates of ∼5.5 mL min−1 with a peristaltic pump (Baoding peristaltic pump model YZ1515X). Each treatment was rinsed with either 200 mL (WA200, WAGr200, WAS200, WASGr200) or 800 mL of deionised water (WA800, WAGr800, WAS800, WASGr800); 800 mL equating to approximately one year of precipitation in England and Wales.33 Each treatment was performed in triplicate and the eluates from the columns were captured at 25 mL intervals in a 50 mL Falcon tube.
4. Eluate analysis
The eluate pH, eluate electrical conductivity (EC) and leaching of nutrients (PO43−, SO42−and NO3−) were studied for all rinsed biochars. The eluate from the rinsed biochars was pH tested in triplicate using a calibrated Voltcraft pH meter and the EC was measured in triplicate using a calibrated Whatman CDM 400 conductivity meter. Each eluate was then analysed in triplicate for phosphate (PO43−), sulphate (SO42−) and nitrate (NO3−) using an ion chromatograph (IC 930 Compact Flex, Metrohm, Switzerland).
5. Lead, copper, zinc and cadmium immobilisation
The immobilisation of Pb, Cu, Zn and Cd was studied for each of the unrinsed biochars and the biochars rinsed with deionised water. Immobilisation batch experiments were carried out using Pb, Cu, Zn and Cd at a concentration of 10 mg L−1 for each metal. This concentration was chosen to expose the biochars to levels beyond those expected in road runoff which have been reported to be as high as 88 μg L−1, 146 μg L−1, 1544 μg L−1 and 4.2 μg L−1 for Pb, Cu, Zn and Cd respectively.34–38 The solution was prepared as described previously by Cairns et al.28 Analytical-grade chemical reagents were used to prepare a stock solution (1000 mg L−1) of Pb(NO3)2, Cu(NO3)2·xH2O, Zn(NO3)2·6H2O and Cd(NO3)2·4H2O. The pH was adjusted to ∼4.7 by dropwise addition of concentrated HNO3 to equalise the pH between solutions to represent the closest to runoff conditions before precipitation occurred for those metals.
All rinsed and unrinsed biochars were oven dried at 105 °C for 24 h. Biochar (0.2 g) was then added to 25 mL of the spiked aqueous solution in 50 mL polyethylene Falcon tubes and agitated for 48 h at 280 rpm on a Unitwist 400 Orbital Shaker to reach equilibrium. The solution was subsequently separated from the sorbent using an MSE Centaur 2 centrifuge at 3000 rpm for 15 minutes.39 The supernatant was removed and immediately acidified to <pH 2 with 1 mL of 70% HNO3 before being filtered with a 0.45 μm PTFE syringe filter for elemental analysis. Two types of control experiments were included: biochar without contaminants, as well as contaminants without biochar. All experiments were performed in triplicate using a batch sorption equilibrium method.40 Pb, Cu, Zn and Cd concentrations of the acidified supernatants were measured using microwave plasma atomic emission spectroscopy (MP-AES 4200, Agilent). Sorbent loading (q) was calculated from the difference between initial metal concentration and final metal concentrations in the aqueous phase:
where
ci is the initial concentration of metals in solution,
caq is the final equilibrium concentration of metals in solution,
V is the volume of solution and
W is the weight of the biochar.
Results and discussion
Wood ash amended biochar has been demonstrated to remove the key motorway runoff contaminants Pb, Cu, Zn and Cd from aqueous solution.27,28 These previous studies have shown that immobilisation of these metals is as a result of precipitation due to increased pH caused by the increase of alkali and alkaline earth metals associated with the wood ash; ion exchange, due to the increase in carboxyl oxygenated functional groups and resultant exchange of heavy metals with the alkali and alkaline earth metals held by these functional groups.28 However, in a field setting the components of wood ash that cause immobilisation could have the potential to be leached from the biochar adding phosphate, nitrate and sulphate into the wider system resulting in potential environmental impacts such as eutrophication.41–43
Different treatments, including mixing wood ash hot with to larch biochar (sintering), mixing wood ash cold with biochar and granulating biochar, were studied in this work. The treatments were assessed to ascertain their effect on the elemental composition of the biochars; the leaching of phosphate, nitrate and sulphate; and the immobilisation of Pb2+, Cu2+, Zn2+ and Cd2+.
1. Biochar properties: unrinsed and rinsed with deionised water
Unrinsed larch biochar mixed cold with wood ash (WA) and larch biochar sintered with wood ash (WAS) contained similar concentrations (mg g−1) of alkali and alkaline earth metals overall. WA had a higher concentration of K and Na whilst WAS had higher concentration of Ca with Mg concentrations being comparable between the biochars (Fig. 1). Although concentrations of alkali and alkaline earth metals were similar between the unrinsed biochars, once they were rinsed with deionised water WAS retained a greater concentration of Ca, K, Mg and Na than WA. Furthermore, once WAS was rinsed with deionised water the concentration for each of the alkali and alkaline earth metals increased (Fig. 1). This indicates that these minerals are not only retained better when the wood ash amendment is sintered to the biochar than when it is cold mixed, but also that they are retained better than other constituent parts of the biochar.
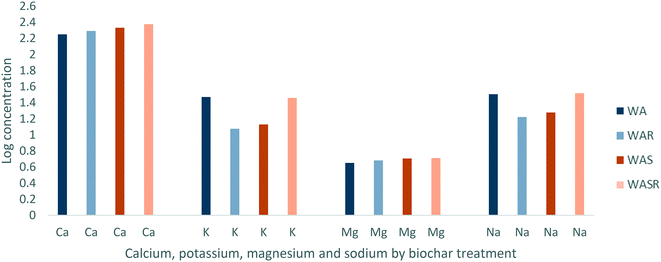 |
| Fig. 1 Log concentration of calcium, potassium, magnesium and sodium for larch biochar mixed cold with wood ash (WA), larch biochar mixed cold with wood ash and rinsed with deionised water (WAR), larch biochar sintered with wood ash (WAS) and larch biochar sintered with wood ash and rinsed with deionised water (WASR). | |
Alkali and alkaline earths are key in the immobilisation of Pb, Cu, Zn and Cd and as such the greater retention of Ca, K, Mg and Na by WAS is important to ongoing immobilisation. This importance is due to the role of alkali and alkaline earth metals in increasing aqueous pH, which is directly related to immobilisation through precipitation.28 This increase in pH will also drive the increase of negative charge of the functional groups because the anionic forms will dominate when pH > pKa allowing alkali and alkaline earth metals to be exchanged more easily.44 The retention of alkali and alkaline earth metals alongside the retention of the oxygenated functional groups that hold these metals as part of their structure are fundamental to ion exchange (ESI 4†). During deployment in the environment, the longer WAS can retain Ca, K, Mg and Na when rinsed the longer the immobilisation mechanisms associated with these elements have the potential to continue. During thermal process such as sintering, heat will drive the decomposition process (e.g., decomposition of mineral phases, charring or biomass) which changes the solid and especially the surface which is key for nutrient or pollutant retention. At the same time, any volatile material will be lost from the outer surface of the solid by evaporation. For the materials under study here, the volatile matter will be volatile organic carbon, CO2 and water. This means that the hot, as-sintered solids will be decarboxylated and dehydrated which will increase interparticle bonding because the respective surfaces are effectively unsaturated. The surfaces will also be free of any surface adsorbed material to increase the sorption of ions from solution. However, as the material is allowed to cool, it will inevitably adsorb CO2 and water from the ambient air to create a more saturated (carboxylated and hydrated) surface and hence the uptake of solutes decreases. The increased retention as a result of the higher temperature of sintering the wood ash to the biochar promoting particle bonding and creating a more stable matrix than mixing the wood ash cold has previously been observed.45 In addition, X-ray diffraction shows the presence of carbonate (calcite, kalicinite) and phosphate (hydroxyapatite) in the wood ash (ESI 2†). Hence, these are entirely compatible with the uptake of carbonate and phosphate anions (and charge balancing cations) from solution.
Unrinsed WAS had a higher concentration of P and S than WA with the concentration of N being similar between the biochars (Fig. 2). Once rinsed with deionised water the concentration of P increased for both biochars, with P in WA increasing by 15.9% and P in WAS increasing by 17.4% (Fig. 2). However, S decreased by 55% and 59% for WA and WAS respectively once rinsed with deionised water. This demonstrates that P is retained better when the wood ash amendment is sintered to the biochar, however P and N are well retained by both treatments in comparison to other elements. S is susceptible to significant loss due to rinsing with deionised water.
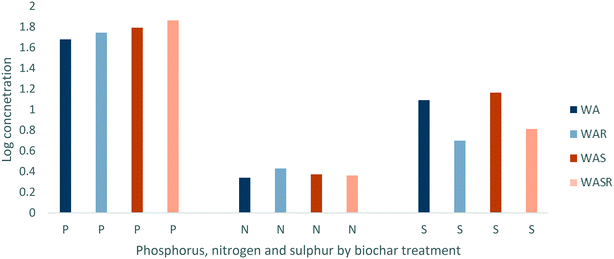 |
| Fig. 2 Log concentration of phosphorus, nitrogen and sulphur for larch biochar mixed cold with wood ash (WA), larch biochar mixed cold with wood ash and rinsed with deionised water (WAR), larch biochar sintered with wood ash (WAS) and larch biochar sintered with wood ash and rinsed with deionised water (WASR). | |
Co-precipitation is facilitated by minerals such as P and S.28,46,47 Of these elements P, and its most prevalent naturally occurring its chemical derivative phosphate, are particularly associated with the immobilisation of Pb, Cu, Zn and Cd through co-precipitation.28 With its greater initial concentrations of P and greater increases in concentrations of P once rinsed with deionised water, WAS has a greater potential to immobilise Pb, Cu, Zn and Cd over longer periods than WA. As well as the importance of P, N and S for immobilisation, they are also constituent elements of nutrients of concern such as phosphates, nitrates and sulphates. The retention of these elements is important not just to the continued immobilisation of contaminants but also to the prevention of leaching nutrients into the aqueous system due to their potentially harmful effects.32,48
2. Eluate analysis
The initial concentration (mg L−1) of eluate phosphate for all treatment types was above the Water Framework Directive (WFD) threshold for groundwater and/or drinking water.49 However, during rinsing with deionised water eluate concentrations of phosphate immediately showed a pattern of steep decline followed by a flattening of the curve to a consistent concentration (Fig. 3A). As a result, it is apparent that rinsing is necessary for all treatment types to remove unbound phosphate that is susceptible to leaching. The eluate of larch biochar sintered with wood ash and granulated to <3 mm (WASGr) and larch biochar mixed cold with wood ash and granulated to <3 mm (WAGr) were the only treatments that reduced phosphates measured to below detection limits when rinsed with deionised water. Due to this reduction in phosphate concentrations these are the only treatments that should be considered as candidates for upscaling for use in the field.
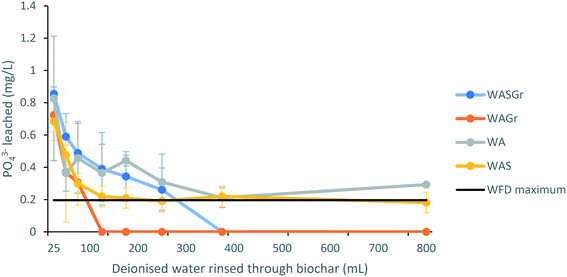 |
| Fig. 3 Concentration of phosphate leached from wood ash mixed cold with larch biochar (WA), wood ash mixed cold and granulated to <3 mm (WAGr), wood ash sintered to larch biochar (WAS) and wood ash sintered to larch biochar and granulated to <3 mm (WASGr). Concentrations are compared to WFD phosphate thresholds. | |
Sulphates initially leached from the biochar treatments at concentrations above 200 mg L−1 for each treatment type (Fig. 4A). This reflects the mg g−1 loss of S demonstrated by the analysis of the biochar properties. The initially high concentrations of sulphate in the eluates reduce within 100 mL of rinsing with deionised water to concentrations below the WFD thresholds.49 Once more, this demonstrates that rinsing with deionised water of all treatments would be necessary prior to deployment in a field situation.
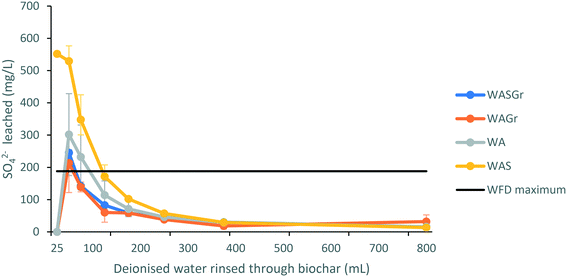 |
| Fig. 4 Concentration of sulphate leached from wood ash mixed cold with larch biochar (WA), wood ash mixed cold and granulated to <3 mm (WAGr), wood ash sintered to larch biochar (WAS) and wood ash sintered to larch biochar and granulated to <3 mm (WASGr). Concentrations are compared to WFD sulphate thresholds. | |
The concentration of nitrates in WASGr, WAGr and WA eluate are also initially above the WFD thresholds49 at over 40 mg L−1 but reduce to ∼0 mg L−1 for each treatment within 200 mL of rinsing with deionised water (Fig. 5A). This further demonstrates the need for each treatment to be rinsed before deployment in the field.
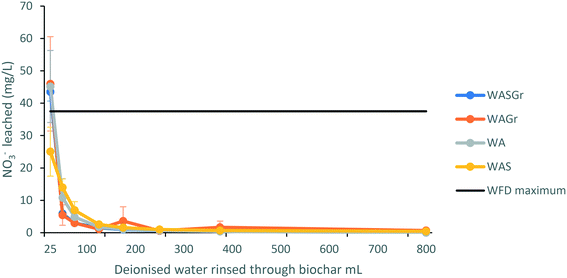 |
| Fig. 5 Concentration of nitrate leached from wood ash mixed cold with larch biochar (WA), wood ash mixed cold and granulated to <3 mm (WAGr), wood ash sintered to larch biochar (WAS) and wood ash sintered to larch biochar and granulated to <3 mm (WASGr). Concentrations are compared to WFD nitrate thresholds. | |
All of the treatments increased eluate pH for the duration of rinsing with deionised water (ESI 3†). Eluate pH did not fall below 8 for any treatment and the ΔpH for all treatments remained between 0.6 and 2.9. With pH in this range Pb, Cu, Zn and Cd are less mobile and are more likely to precipitate.50 Cairns et al.28 modelled that the distribution of divalent forms of Pb, Cu, Zn and Cd shift to hydroxyl forms at these pH levels and that when accompanied by anions such as PO43− and SO42− led to the formation of precipitates immobilising Pb, Cu, Zn and Cd (Fig. 6A–D). Surface charge is also strongly influenced by pH. Several previous studies have also shown that pH in the range of this study to be above the point of zero charge (PZC).51–53 When pH is greater than the PZC biochar is negatively charged and binds to metal cations.54 Eluate pH remaining above 8 even after rinsing with 800 mL of deionised water, or the equivalent of one year's rainfall, indicates that these treatments have the potential to immobilise Pb, Cu, Zn and Cd over longer periods as a result of precipitation and electrostatic attraction. The potential of such continued immobilisation is reinforced by the strong retention of key minerals, nutrients and oxygenated functional groups associated with ion exchange and co-precipitation (Fig. 1 and 2 and ESI 4†).
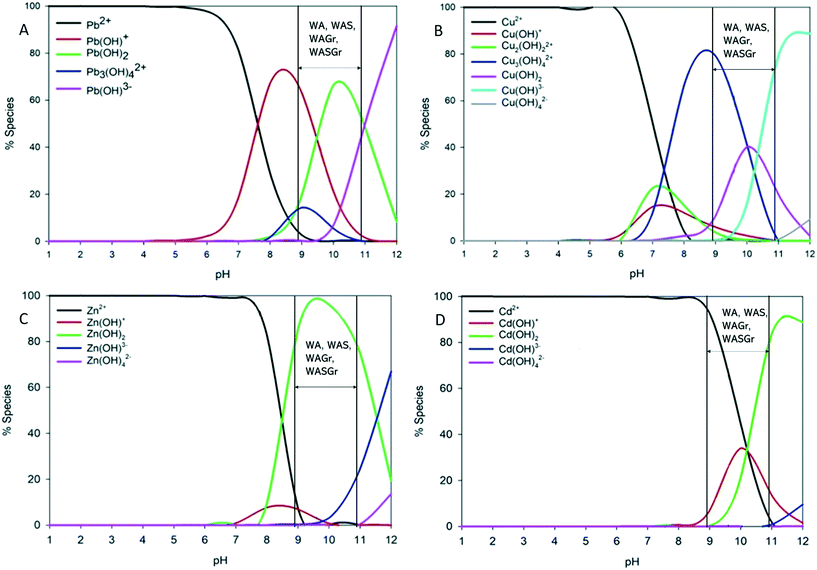 |
| Fig. 6 Speciation plots of (A) Pb, (B) Cu, (C) Zn, and (D) Cd across pH. Reference lines indicate pH range of wood ash mixed cold with larch biochar (WA), wood ash mixed cold and granulated to <3 mm (WAGr), wood ash sintered to larch biochar (WAS) and wood ash sintered to larch biochar and granulated to <3 mm (WASGr). Adapted from Cairns et al.28 | |
3. Lead, copper, zinc and cadmium immobilisation
Rinsing with deionised water did not reduce the immobilisation of Pb, Cu, Zn and Cd by any of the biochar treatments with each treatment removing between 97% and 100% of each contaminant when rinsed with the maximum 800 mL (ESI 5†). This demonstrates that when the biochar treatments are rinsed with deionised water to reduce the leaching of phosphates, sulphates and nitrates to below WFD thresholds immobilisation of Pb, Cu, Zn and Cd continues.
The high degree of immobilisation of Pb, Cu, Zn and Cd (97–100%) when rinsed by 800 mL of deionised water, the equivalent of one year of rainfall, demonstrates that the biochars did not reach exhaustion during this study. The treatments studied have the potential to immobilise Pb, Cu, Zn and Cd over longer periods. Such immobilisation is as a result of increased eluate pH, the retention of alkali and alkaline earths and the retention of P important to precipitation, ion exchange and co-precipitation. These mechanisms are particularly evident for treatments where the wood ash was sintered rather than mixed cold.
Conclusion
Wood ash sintered larch biochar granulated <3 mm (WASGr) and wood ash cold mixed with larch biochar granulated <3 mm (WAGr) are both viable options to immobilise Pb, Cu, Zn and Cd from motorway runoff, with WASGr having the greatest potential to immobilise these contaminants over longer periods. WAS retains Ca, K, Mg, Na and P better than WA when rinsed with deionised water. The retention of these minerals and nutrients are key to the immobilisation of Pb, Cu, Zn and Cd. The increased retention is as a result of the higher temperature of sintering the wood ash to the biochar promoting particle bonding and creating a more stable matrix than mixing the wood ash cold.45 Despite the retention of key elements such as P and N, when rinsed with deionised water the biochars all initially leach phosphates, sulphates and nitrates at concentrations (mg L−1) above the Water Framework Directive (WFD) threshold for groundwater and/or drinking water.49 However, when WASGr and WAGr were rinsed with deionised water the concentrations of phosphates, sulphates and nitrates fell below WFD thresholds. The rinsing with deionised water required to lower the concentrations of phosphates, sulphates and nitrates did not reduce the high degree of immobilisation of Pb, Cu, Zn and Cd (97–100%) demonstrated by WASGr and WAGr. As a result, WASGr rinsed with deionised water has the potential to be scaled up and deployed to immobilise Pb, Cu, Zn and Cd from motorway runoff without a negative impact on the concentration of nutrients in the surrounding waters.
Conflicts of interest
There are no conflicts to declare.
Acknowledgements
Ysgoloriaeth Sgiliau Economi Gwybodaeth (KESS) yn Gymru gyfan sgiliau lefel uwch yn fenter a arweinir gan Brifysgol Bangor ar ran y sector AU yng Nghymru. Fe'i cyllidir yn rhannol gan Gronfeydd Cymdeithasol Ewropeaidd (ESF) cydgyfeirio ar gyfer Gorllewin Cymru a'r Cymoedd. Knowledge Economy Skills Scholarships (KESS) is a pan-Wales higher level skills initiative led by Bangor University on behalf of the HE sector in Wales. It is part funded by the Welsh Government's European Social Fund (ESF) convergence programme for West Wales and the Valleys. This work is part funded by the Welsh Government's European Social Fund (ESF) convergence programme for West Wales and the Valleys. The authors would also like to extend the acknowledgements to Dr Gabriel Sigmund (University of Vienna), Sampriti Chaudhuri (University of Vienna), Richard Haine (Frog Environmental), Sion Brackenbury (TerrAffix Soil Solutions), Peter Lanfear (TerrAffix Soil Solutions) and Dr Ian Mabbett (Swansea University).
Notes and references
-
R. Van Der Ree, D. J. Smith and C. Grilo, The Ecological Effects of Linear Infrastructure and Traffic: Challenges and Opportunities of Rapid Global Growth, Handbook of Road Ecology, 2015, pp. 1–9 Search PubMed.
- W. F. Laurance, G. R. Clements, S. Sloan, C. S. O'Connell, N. D. Mueller and M. Goosem,
et al., A global strategy for road building, Nature, 2014, 513(7517), 229–232 CrossRef CAS PubMed.
- T. Richard, T. Forman and R. D. Deblinger, The ecological road-effect zone of a Massachusetts (U.S.A.) suburban highway, Conserv. Biol., 2000, 14(1), 36–46 CrossRef.
- B. B. Phillips, J. M. Bullock, J. L. Osborne and K. J. Gaston, Spatial extent of road pollution: A national analysis, Sci. Total Environ., 2021, 773, 145589, DOI:10.1016/j.scitotenv.2021.145589.
- R. Hilliges, M. Endres, A. Tiffert, E. Brenner and T. Marks, Characterization of road runoff with regard to seasonal variations, particle size distribution and the correlation of fine particles and pollutants, Water Sci. Technol., 2017, 75(5), 1169–1176 CrossRef CAS PubMed.
- M. Werkenthin, B. Kluge and G. Wessolek, Metals in European roadside soils and soil solution - A review, Environ. Pollut., 2014, 189, 98–110 CrossRef CAS PubMed.
- K. R. Reddy, T. Xie and S. Dastgheibi, Removal of heavy metals from urban stormwater runoff using different filter materials, J. Environ. Chem. Eng., 2014, 2(1), 282–292 CrossRef CAS.
- S. S. Withanachchi, G. Ghambashidze, I. Kunchulia, T. Urushadze and A. Ploeger, Water quality in surface water: A preliminary assessment of heavy metal contamination of the Mashavera river, Georgia, Int. J. Environ. Res. Public Health, 2018, 15(4), 1–25 Search PubMed.
- P. K. Rai, S. S. Lee, M. Zhang, Y. F. Tsang and K. H. Kim, Heavy metals in food crops: Health risks, fate, mechanisms, and management, Environ. Int., 2019, 125, 365–385 CrossRef CAS PubMed.
- P. Klöckner, B. Seiwert, P. Eisentraut, U. Braun, T. Reemtsma and S. Wagner, Characterization of tire and road wear particles from road runoff indicates highly dynamic particle properties, Water Res., 2020, 185, 116262 CrossRef PubMed.
- Y. Ma, S. Mummullage, B. Wijesiri, P. Egodawatta, J. McGree and G. A. Ayoko,
et al., Source quantification and risk assessment as a foundation for risk management of metals in urban road deposited solids, J. Hazard. Mater., 2021, 408, 124912 CrossRef CAS PubMed.
- P. Budai and A. Clement, Refinement of national-scale heavy metal load estimations in road runoff based on field measurements, Transp. Res. D: Transp. Environ., 2011, 16(3), 244–250, DOI:10.1016/j.trd.2010.12.003.
- X. Du, Y. Zhu and Q. Han,
et al., The influence of traffic density on heavy metals distribution in urban road runoff in Beijing, China, Environ. Sci. Pollut. Res., 2019, 26, 886–895 CrossRef CAS PubMed.
- S. Wagner, T. Hüffer, P. Klöckner, M. Wehrhahn, T. Hofmann and T. Reemtsma, Tire wear particles in the aquatic environment - A review on generation, analysis, occurrence, fate and effects, Water Res., 2018, 139, 83–100 CrossRef CAS PubMed.
- F. Napier, B. D'Arcy and C. Jefferies, A review of vehicle related metals and polycyclic aromatic hydrocarbons in the UK environment, Desalination, 2008, 226(1–3), 143–150 CrossRef CAS.
- T. T. T. Duong and B. K. Lee, Determining contamination level of heavy metals in road dust from busy traffic areas with different characteristics, J. Environ. Manage., 2011, 92(3), 554–562 CrossRef CAS PubMed.
- S. J. Beane, S. D. W. Comber, J. Rieuwerts and P. Long, Abandoned metal mines and their impact on receiving waters: A case study from Southwest England, Chemosphere, 2016, 153, 294–306 CrossRef CAS PubMed.
- P. Sharma and S. Kumar, Bioremediation of heavy metals from industrial effluents by endophytes and their metabolic activity: Recent advances, Bioresour. Technol., 2021, 339, 125589 CrossRef CAS PubMed.
- M. Hussein, K. Yoneda, Z. Mohd-Zaki, A. Amir and N. Othman, Heavy metals in leachate, impacted soils and natural soils of different landfills in Malaysia: An alarming threat, Chemosphere, 2021, 267, 128874 CrossRef CAS PubMed.
-
Environment Agency Wales, Metal Mines Strategy for Wales, Cardiff, 2002, Available from: https://naturalresources.wales/media/680181/metal-mines-strategy-for-wales-2.pdf Search PubMed.
-
Coal Authority, Metal Mine Failing Waterbodies Assessment - Overview Report, Mansfield, 2020 Search PubMed.
- M. S. Hasan, M. Geza and R. Vasquez,
et al., Enhanced Heavy Metal Removal from Synthetic Stormwater Using Nanoscale Zerovalent Iron–Modified Biochar, Water, Air, Soil Pollut., 2020, 231, 220 CrossRef CAS.
-
European Biochar Foundation (EBC), Guidelines for a Sustainable Production of Biochar, European Biochar Foundation (EBC), 2016, pp. 1–22 Search PubMed.
- Y. Yang, K. Sun, L. Han, J. Jin, H. Sun and Y. Yang,
et al., Effect of minerals on the stability of biochar, Chemosphere, 2018, 204, 310–317 CrossRef CAS PubMed.
- M. I. Inyang, B. Gao, Y. Yao, Y. Xue, A. Zimmerman and A. Mosa,
et al., A review of biochar as a low-cost adsorbent for aqueous heavy metal removal, Crit. Rev. Environ. Sci. Technol., 2016, 46(4), 406–433 CrossRef CAS.
- T. Kätterer, D. Roobroeck, O. Andrén, G. Kimutai, E. Karltun and H. Kirchmann,
et al., Biochar addition persistently increased soil fertility and yields in maize-soybean rotations over 10 years in sub-humid regions of Kenya, Field Crops Res., 2019, 235, 18–26 CrossRef.
- S. Cairns, I. Robertson, G. Sigmund and A. Street-Perrott, The removal of lead, copper, zinc and cadmium from aqueous solution by biochar and amended biochars, Environ. Sci. Pollut. Res., 2020, 27(17), 21702–21715 CrossRef CAS PubMed.
- S. Cairns, S. Chaudhuri, G. Sigmund, I. Robertson, N. Hawkins and T. Dunlop,
et al., Wood ash amended biochar for the removal of lead, copper, zinc and cadmium from aqueous solution, Environ. Technol. Innovation, 2021, 101961 CrossRef CAS , Available from: https://linkinghub.elsevier.com/retrieve/pii/S235218642100609X.
- H. Wu, K. Yip, Z. Kong, C. Z. Li, D. Liu and Y. Yu,
et al., Removal and recycling of inherent inorganic nutrient species in mallee biomass and derived biochars by water leaching, Ind. Eng. Chem. Res., 2011, 50(21), 12143–12151 CrossRef CAS.
- Y. Liang, X. Cao, L. Zhao, X. Xu and W. Harris, Phosphorus Release from Dairy Manure, the Manure-Derived Biochar, and Their Amended Soil: Effects of Phosphorus Nature and Soil Property, J. Environ. Qual., 2014, 43(4), 1504–1509 CrossRef PubMed.
- X. Cui, X. Dai, K. Y. Khan, T. Li, X. Yang and Z. He, Removal of phosphate from aqueous solution using magnesium-alginate/chitosan modified biochar microspheres derived from Thalia dealbata, Bioresour. Technol., 2016, 218, 1123–1132 CrossRef CAS PubMed.
- V. H. Smith, G. D. Tilman and J. C. Nekola, Eutrophication: impacts of excess nutrient inputs on freshwater, marine, and terrestrial ecosystems, Environ. Pollut., 1999, 100, 179–196 CrossRef CAS PubMed.
-
Met Office National Climate Information Centre, Monthly, seasonal and annual total precipitation amount for England and Wales [Dataset], cited 1 December 2021, Available from: https://www.metoffice.gov.uk/pub/data/weather/uk/climate/datasets/Rainfall/ranked/England_and_Wales.txt.
- B. Crabtree, P. Dempsey, I. Johnson and M. Whitehead, The development of an ecological approach to manage the pollution risk from highway runoff, Water Sci. Technol., 2009, 59(3), 549–555 CrossRef CAS PubMed.
- H. Zhao, X. Li, X. Wang and D. Tian, Grain size distribution of road-deposited sediment and its contribution to heavy metal pollution in urban runoff in Beijing, China, J. Hazard. Mater., 2010, 183(1–3), 203–210 CrossRef CAS PubMed.
- Q. Zhang, X. Wang, P. Hou, W. Wan, Y. Ren and Z. Ouyang,
et al., The temporal changes in road stormwater runoff quality and the implications to first flush control in Chongqing, China, Environ. Monit. Assess., 2013, 185(12), 9763–9775 CrossRef CAS PubMed.
- A. A. MacKay, S. Zinke, J. Mahoney and J. T. Bushey, Roadway Runoff Water Quality from Milled and Unaltered Surfaces during Convective Storms, J. Environ. Eng., 2011, 137(12), 1165–1175 CrossRef CAS.
- M. Legret and C. Pagotto, Evaluation of pollutant loadings in the runoff waters from a major rural highway, Sci. Total Environ., 1999, 235(1–3), 143–150 CrossRef CAS PubMed.
- OECD, Guideline 106 for the testing of chemicals- Adsorption - Desorption Using a Batch Equilibrium Method, OECD Organisation for Economic Co-Operation and Development, 2000, pp. 1–44.
- OECD, Test No. 106: Adsorption -- Desorption Using a Batch Equilibrium Method. OECD Guidelines for the Testing of Chemicals, 2000, Section 1.
- T. Tulonen, L. Arvola and S. Ollila, Limnological Effects of Wood Ash Application to the Subcatchments of Boreal, Humic Lakes, J. Environ. Qual., 2002, 31(3), 946–953 CrossRef CAS PubMed.
- A. A. Bogush, J. A. Stegemann, R. Williams and I. G. Wood, Element speciation in UK biomass power plant residues based on composition, mineralogy, microstructure and leaching, Fuel, 2018, 211, 712–725 CrossRef CAS.
- S. V. Vassilev, D. Baxter, L. K. Andersen and C. G. Vassileva, An overview of the composition and application of biomass ash. Part 1. Phase-mineral and chemical composition and classification, Fuel, 2013, 105, 40–76 CrossRef CAS.
- A. Silber, I. Levkovitch and E. R. Graber, PH-dependent mineral release and surface properties of cornstraw biochar: Agronomic implications, Environ. Sci. Technol., 2010, 44(24), 9318–9323 CrossRef CAS PubMed.
-
T. Astrup, A. Muntoni, A. Polettini, R. Pomi, T. Van Gerven and A. Van Zomeren, Treatment and Reuse of Incineration Bottom Ash, in Environmental Materials and Waste: Resource Recovery and Pollution Prevention, Elsevier Inc., 2016, pp. 607–645, DOI:10.1016/B978-0-12-803837-6.00024-X.
- A. Fouladi Tajar, T. Kaghazchi and M. Soleimani, Adsorption of cadmium from aqueous solutions on sulfurized activated carbon prepared from nut shells, J. Hazard. Mater., 2009, 165(1–3), 1159–1164 CrossRef CAS PubMed.
- Y. Zarga, H. ben Boubaker, N. Ghaffour and H. Elfil, Study of calcium carbonate and sulfate co-precipitation, Chem. Eng. Sci., 2013, 96, 33–41 CrossRef CAS.
- D. Anderson, P. Glibert and J. Burkholder, Harmful Algal Blooms and Eutrophication Nutrient Sources, Composition, and Consequences, Estuaries, 2002, 25(4), 704–726 CrossRef.
- The Water Framework Directive, The Water Framework Directive (Standards and Classification) Directions (England and Wales), Water Framework Directive, 2015, vol. 40, 2, p. 66, Available from: http://www.legislation.gov.uk/uksi/2015/1623/pdfs/uksiod_20151623_en_auto.pdf%0Ahttps://www.gov.uk/guidance/chemical-standards-database.
-
M. Pourbaix, Atlas of electrochemical equilibria in aqueous solutions, Bristol, First Engl, 1966 Search PubMed.
- W. Yin, C. Zhao and J. Xu, Enhanced adsorption of Cd (II) from aqueous solution by a shrimp bran modified Typha orientalis biochar, Environ. Sci. Pollut. Res., 2019, 26(36), 37092–37100 CrossRef CAS PubMed , Available from: https://link.springer.com/article/10.1007/s11356-019-06658-x.
- P. Maneechakr and S. Mongkollertlop, Investigation on adsorption behaviors of heavy metal ions (Cd2+, Cr3+, Hg2+ and Pb2+) through low-cost/active manganese dioxide-modified magnetic biochar derived from palm kernel cake residue, J. Environ. Chem. Eng., 2020, 8(6), 104467 CrossRef CAS.
- J. H. Yuan, R. K. Xu and H. Zhang, The forms of alkalis in the biochar produced from crop residues at different temperatures, Bioresour. Technol., 2011, 102(3), 3488–3497 CrossRef CAS PubMed.
- H. Li, X. Dong, E. B. da Silva, L. M. de Oliveira, Y. Chen and L. Q. Ma, Mechanisms of metal sorption by biochars: Biochar characteristics and modifications, Chemosphere, 2017, 178, 466–478 CrossRef CAS PubMed.
|
This journal is © The Royal Society of Chemistry 2022 |