DOI:
10.1039/D1FO02369A
(Paper)
Food Funct., 2022,
13, 76-90
Impact of following a healthy dietary pattern with co-consuming wolfberry on number and function of blood outgrowth endothelial cells from middle-aged and older adults†
Received
21st July 2021
, Accepted 19th November 2021
First published on 22nd November 2021
Abstract
Blood outgrowth endothelial cells (BOECs) have received growing attention in relation to cardiovascular disease (CVD). However, the effect of diet intervention, a primary strategy for CVD prevention, on BOECs is not reported. This study aims to investigate the effect of following a healthy dietary pattern (HDP) with or without wolfberry consumption, healthy food with potential cardiovascular benefits, on the number and function of BOECs in middle-aged and older adults. Twenty-four subjects consumed either an HDP only (n = 9) or an HDP supplemented with 15 g day−1 wolfberries (n = 15) for 16 weeks. At pre- and post-intervention, vascular health biomarkers and composite CVD risk indicators were assessed. BOECs were derived from peripheral blood mononuclear cells and their angiogenic and migration activities were measured. Isolated BOECs have typical endothelial cobblestone morphology, express von Willebrand factor and KDR. Consuming an HDP improved the BOEC colony's growth rate, which was demonstrated by significant time effects in the colony's culture time between passages 1 and 2 (P = 0.038). Both interventions increased BOECs’ tube formation capacity. Moreover, HDP intervention contributed to a time effect on BOEC migration activity (P = 0.040 for t1/2gap). Correlation analysis revealed that BOEC colony number was positively associated with blood pressure, atherogenic index, vascular age, and Framingham risk score. In conclusion, adherence to an HDP improved BOECs’ function in middle-aged and older populations, while additional wolfberry consumption did not provide an enhanced effect. Our results provide mechanistic dissection on the beneficial effects on BOECs of dietary pattern modification.
Introduction
Novel biomarkers of cardiovascular disease (CVD), the leading cause of death and disability worldwide, have recently received a surge in recognition. Endothelial progenitor cells (EPCs) are a heterogeneous subset of circulating bone marrow-derived cells with remarkable plasticity. Notably, EPCs have the ability to differentiate into mature endothelial cells, which are involved in endothelial homeostasis and vessel repair.1–4 An accumulation of evidence has demonstrated that circulating levels of EPCs could be a pathophysiological link between CVD risk factors and endothelial dysfunction, suggesting a significant role of EPCs in the progression of CVDs.3,5
EPCs originate from multiple precursors and are presented in different stages of endothelial differentiation in peripheral blood. Therefore, the precise characterization of EPCs is difficult, and currently, there is no uniform definition of an EPC.6,7 As redefined by Prater et al.,8 there are mainly four types of cells claimed to be EPCs: circulating EPCs, colony-forming unit-endothelial cells (CFU-ECs), circulating angiogenic cells (CACs) and blood outgrowth endothelial cells (BOECs). Among them, BOECs also referred to as outgrowth endothelial cells, endothelial colony-forming cells, or late-outgrowth endothelial cells, are considered the only cell type featuring the characteristics of a “true” EPC, given that these cells are self-renewing, clonogenic, and able to form capillary-like structures and integrating into functional blood vessels in vitro and in vivo, respectively.8–12 Therefore, BOECs have recently attracted considerable interest as a potential cell-based therapy for vascular regeneration, gene therapy and as a tool to study endothelial dysfunction.2,12
Non-pharmacological treatments, including dietary modification, represent the first approach for the primary prevention of CVDs.13 Recently, there has been a rapid growth in attention surrounding the effect of diet on EPCs. Several studies have investigated the effect of a healthy dietary pattern (HDP), such as the Mediterranean diet, and certain diets rich in vegetables, unsaturated fats and other food bioactive components on EPCs and found that numbers of EPCs could be altered by dietary modifications.4,5,13–20 However, studies with dietary modification mainly focused on the number of circulating EPCs with different antigenic profiles and some of them examined the number and function of CACs. Whereas data on the effect of diet modification on BOECs’ number and function are scarce.
Wolfberry, also known as Goji berry, is a fruit from the plant Lycium barbarum L. under the Solanaceous family.21 In the last decade, it has become increasingly popular in many countries due to its potential health benefits, including cardiovascular protection, which is generally explained by its chemical constituents including carotenoids, polysaccharides and polyphenols.22 Previous clinical studies confirmed that wolfberry or its polysaccharide consumption improved the blood lipid-lipoprotein profile. Besides, wolfberry polysaccharides also provide benefits to blood pressure management.23–25 Moreover, evidence suggests that wolfberry consumption lowers oxidative stress in middle-aged and older individuals, in parallel, in vitro scavenge free radical effect was also observed in wolfberry flavonoids fraction.26,27 Recently, Lee et al.28 revealed that dietary wolfberry extract presented antioxidative and anti-inflammatory effects on overweight and hypercholesterolemic subjects by controlling the mRNA levels of oxidative stress and inflammation-related genes. However, the involvement of BOECs in this benefit in conjunction with following HDP is unknown. Therefore, in this study, we aimed to investigate the effects of consuming wolfberry as part of an HDP on the number and function of BOECs derived from middle-aged and older adults.
Methods
Study design
This was a 16-week parallel, investigator blinded, randomized controlled trial. Subjects were randomized to the HDP only (HDPO) group or HDP supplemented with wolfberry (HDPW) group by a research staff who was independent of the study using STATA/MP 13 (StataCorp LP, College Station, Texas, USA). During the intervention period, all subjects followed an HDP while subjects in the HDPW group consumed wolfberry. Each subject completed a total of 2 study visits at weeks 0 and 16. At each visit, anthropometric parameters, blood pressure, brachial artery flow-mediated dilatation (FMD) and carotid intima-media thickness (IMT) were measured. Fasting blood samples and 3-day food records were also collected. Investigators involved in parameter assessment and data processing were blinded to the treatments.
Subjects
Participants were recruited from a community in Singapore during July 2018 and June 2019 with all assessments completed before April 2020. Detailed inclusion criteria were: age 50–75 years; no allergy to wolfberry; not smoking; not consuming excessive amounts of alcohol (≤21 units for men and ≤14 units for women); no significant body weight change (±5% body weight) and no vigorous exercise (defined as having more than 20 min of moderate-intensity exercise daily) in the past 3 months; not pregnant, lactating or planning pregnancy; no acute illness; not taking antihypertensive/cholesterol-lowering/type-2 diabetic medication or traditional Chinese medicine unless they have been on medication for more than 5 years before the participation; no dietary supplement consumption, which may impact the results (e.g. multivitamins and omega-3/fish oil supplements); not following healthy eating pattern diet in the past 3 months.
Participants were screened and enrolled by the main study coordinator. Forty-one eligible subjects received the intervention. Clinical and BOEC data collection and analysis were finally performed on 9 and 15 participants from the HDPO and HDPW groups, respectively, after BOEC experimental protocol optimization (Fig. S1†). The study protocol was conducted according to the Declaration of Helsinki and was approved by the National Healthcare Group's Domain Specific Review Board (NHG DSRB; Protocol 2018/0311) and registered at http://www.clinicaltrials.gov (ID: NCT03535844). All subjects provided written informed consent and received monetary compensation for participation.
Dietary intervention
Dietary counseling to follow the HDP was provided to both HDPO and HDPW groups by trained research nutritionists. HDP referred to “My Healthy Plate” designed by the Health Promotion Board, Singapore.29 According to the guidelines, for middle-aged and older adults, daily consumption of 4–6 servings of whole grains (including brown rice, whole wheat bread, wholegrain noodles and wholegrain breakfast cereal), 3 servings of meat (excluding processed food such as bacon, ham and sausage) and alternatives (including eggs, legumes, pulses, nuts, dairy and soy-based foods) with 1 serving from a calcium-rich source (including dairy-based foods, soy-based foods and fish with edible bones) and 2 servings of both fruits and vegetables (excluding starchy vegetables such as potato, sweet potato, tapioca and yam). Healthier oils such as canola, olive, soy and sunflower oils were also recommended. The serving size of each food group was defined using “My Healthy Plate”29 and World Health Organization.30 Subjects in the HDPW group additionally consumed 15 g day−1 of dried wolfberry (Ning Xia Lycium barbarum L., Eu Yan Sang Private Ltd, Singapore) by boiling or steaming as part of their meals. Wolfberry amount was determined on the basis of prior literature, which reported that consuming 14 g day−1 wolfberry for 45 days or 15 g day−1 for 28 days generated statistical improvement on lipid profile of plasma zeaxanthin levels.23,31
Anthropometric parameters measurements
At pre- and post-intervention, height, weight and waist circumference (WC) were measured by trained research staff during the fasting state. Height and weight were measured using a stadiometer (Seca, Hamburg, Germany). BMI was calculated as weight in kilograms divided by the square of height in meters. WC was measured in between the final rib and iliac crest after normal expiration ended.32 All measurements were done in duplicates.
Classical CVD risk factors and CVD risk assessment
Systolic and diastolic blood pressures (SBP and DBP) were measured using an upper arm blood pressure monitor (BP7100, Omron Healthcare Inc., Illinois, USA) in a rested sitting position. Fasting-state blood samples were collected by a trained phlebotomist via intravenous cannulation. The levels of triglyceride, total cholesterol (TC), low-density lipoprotein cholesterol (LDL-C) and high-density lipoprotein cholesterol (HDL-C) in serum were analyzed by Quest Laboratories Pte., Ltd (Singapore). The subject's atherogenic index (AI) was calculated as (TC − [HDL-C])/(HDL-C).33 The Framingham risk score and vascular age of subjects were calculated in accordance with the Framingham heart study, 10-year CVD risk prediction on the basis of lipid equation.34
Vascular health-related outcomes
Endothelial function was assessed by measuring the FMD of the brachial artery as described by Mak et al.35 using high-resolution ultrasound with a 10 MHz linear array transducer. Carotid IMT was evaluated by B-mode ultrasonography of the common carotid artery following the guidelines published by the American Society of Echocardiography.36 All FMD and IMT measurements were performed by sonographers who were specifically trained and blinded to the treatment group using a Prosound Alpha 10 ultrasound system (Hitachi-Aloka Medical Ltd, Tokyo, Japan). Levels of angiopoietin-1 (ANG1), angiopoietin-2 (ANG2) and von Willebrand factor (VWF) in EDTA-preserved plasma were analyzed using commercial human ELISA kits (Abcam Singapore Pte., Ltd, Singapore) following the manufacturer's instructions.
Intervention compliance assessment
Dietary compliance was regularly checked using meal photographs and frequent online and in-person contact. The intakes of the food groups (whole grains, meat and alternatives, fruits and vegetables) were assessed based on a 3-day food record. Daily protein, carbohydrates and fat intakes were assessed using the diet plan 7 (Forestfield Software Ltd, West Sussex, UK) with USDA Food Composition Databases,37 Singapore Health Promotion Board nutrient composition database38 and nutritional information panels for commercial food products. The compliance of wolfberry consumption was also monitored by checking plasma carotenoid concentrations. Briefly, carotenoids were extracted from EDTA-preserved plasma and analyzed using a Waters Alliance e2695 HPLC-PDA system (Waters, Massachusetts, USA) equipped with a reverse-phase column (Carotenoid C30, YMC Co. Ltd, Kyoto, Japan).39 Carotenoid standards (β-carotene, α-carotene, lycopene, β-cryptoxanthin, lutein and zeaxanthin) were purchased from Sigma Aldrich (Sigma-Aldrich, Missouri, USA) to create standard curves.
BOECs isolation and culture
BOECs were isolated and cultured according to Martin-Ramirez et al.12 and Wang et al.40 with minor modifications. Twenty-seven milliliters of venous blood from each subject were collected in EDTA tubes, maintained at room temperature and processed within 2 h. Blood was diluted 1
:
1 with Ca2+- and Mg2+-free PBS (Lonza Bioscience Singapore Pte., Ltd, Singapore). Buffy coat mononuclear cells (MNCs) were isolated by gradient centrifugation over Ficoll-Paque Plus (GE Healthcare Bio-Sciences AB, Uppsala, Sweden) at 1000g for 20 min at room temperature without break. Cell pellets were resuspended in the EGM-2 medium (Lonza, Singapore) supplemented with 20% FBS (GE Healthcare, Sweden) and an EGM-2 BulletKit (Lonza, Singapore) after washing with PBS and then with media. The CD34+/KDR+ percentage of MNCs was examined using flow cytometry. MNCs were seeded onto 24-well tissue culture plates precoated with rat tail collagen type I (Life Technologies Corporation, Nebraska, USA) and cultured in a 37 °C, 5% CO2 incubator (MCO-20AIC, Sanyo Electric Biomedical Co., Ltd, Osaka, Japan) with humidified atmosphere. The culture medium was refreshed three times per week by replacing 1 mL medium per well for the first week and 0.5 mL medium per well for the following 3 weeks. The first medium replacement was executed 48 h after seeding.
Discrete colonies of cells with cobblestone morphology were checked and enumerated at least three times per week from day 7 using an EVOS XL Core Cell Imaging System (Life Technologies, USA). For subculturing, cells were detached using 0.25% trypsin-EDTA solution for 5 min.12 Colony growth time from appearance to the first and second passages were recorded. Colony cells from the same sample were pooled for further expansion from passage 2. After being detached by trypsinization, phenotyping and functional evaluations were performed at passages 3–8.40,41
Flow cytometric analysis
The expression of KDR and CD34 in MNCs and BOECs was determined by flow cytometry (CytoFLEX LX, Beckman Coulter, Indiana, USA). 1 × 106 MNCs or 0.5–1 × 106 BOECs were washed once with PBS containing 2% FBS and 15 mM HEPES buffer (Lonza, Singapore). Cells were then incubated with the primary antibody for 15 min at room temperature in the dark. We used primary mouse monoclonal antibodies against human CD34 conjugated to allophycocyanin (Invitrogen, Thermo Fisher Scientific, Massachusetts, USA) and human KDR conjugated to phycoerythrin (BD Biosciences, California, USA). Unstained samples and samples incubated with fluorescence minus one control were run in parallel. Data were analyzed using the FlowJo V10 software (FlowJo LLC, Oregon, USA). Experiments were performed in duplicate for each sample.
Confocal immunofluorescence microscopy
The expression of VWF of BOECs was visualized using monoclonal antibody rabbit antihuman VWF conjugated to Alexa 488 (Abcam, Singapore). BOECs were seeded to pre-coated μ-Slide 8 well glass-bottom dishes (ibidi GmbH, Munich, Germany) with 0.5 × 105 cells per well. Cells were fixed with methanol (pre-chilled at −20 °C) for 5 min at room temperature. After rinsing with ice-cold PBS, cells were then permeabilized with PBS containing 0.1% Triton X-100 for 10 min at room temperature. After 30 min blocking with 1% bovine serum albumin (Sigma-Aldrich, USA) in PBST (PBS containing 0.1% Tween 20), cells were incubated with 1
:
100 diluted VWF antibody in the dark for 1 h at room temperature. For visualization of nuclei, cells were incubated with 5 μg mL−1 4′,6-diamidino-2-phenylindole (Invitrogen, USA) for 10 min. Images were acquired using a laser confocal microscope system with a 40× objective (Eclipse Ti-E inverted microscope, Nikon Instruments Inc., Tokyo, Japan).
Matrigel assay and microscopic imaging
The angiogenic properties of BOECs were assessed using a tube formation assay on Matrigel assay as previously described,40,42 with some modifications. Growth factor reduced basement membrane matrix (Corning Inc., New York, USA) was thawed overnight on ice. Pre-cooled 96-well plates were loaded with 50 μL per well Matrigel using pre-cooled pipette tips. The plate was incubated at 37 °C for 30 min to allow the Matrigel to solidify. In the meantime, BOECs were resuspended in a complete EGM-2 medium and then 100 μL cell suspension (containing 1 × 104 cells) was seeded to each well. The plate was incubated at 37 °C and 5% CO2 for 6 h based on our time optimization result (Fig. S2†). Images were captured using a digital sight camera under the Eclipse TS100 inverted microscope (Nikon, Japan). Characterization of the capillary-like structures was performed using the Angiogenesis Analyzer plugin for ImageJ (National Institutes of Health, Maryland, USA).43 Experiments were performed in triplicate for each sample.
Wound-healing assay
The migration ability of BOECs was examined by a wound-healing assay according to the methods described by Groeneveld et al.41 and Jonkman et al.44 with some modifications. BOECs were plated into a 6-well plate coated with collagen in a complete EGM-2 medium (4 × 105 cells per well) and cultured overnight until a confluent monolayer was formed. A gap was introduced by scratching the bottom of the well with a pipette tip. After washing gently twice with PBS to remove debris, 2 mL serum starving medium (EGM-2 medium without 20% FBS) with an additional 2 ng mL−1 actinomycin D (Thermo, USA) was added to each well to reduce bias related to proliferative effects. The culture plate was placed in a Zeiss AxioObserver Z1 microscope (Carl Zeiss AG, Jena, Germany) equipped with an environmental chamber for the control of temperature, CO2 concentration and humidity. Images were automatically acquired and recorded at 20 min intervals for 6–7 h using a phase-contrast objective. Experiments were performed in triplicate, with an average of 9 wound views were observed, for each sample.
The image of the wound area was analyzed using ImageJ. To improve the accuracy of automatic gap detection, Adobe Photoshop CC 2019 (Adobe Systems, California, USA) was used to outline and highlight the wound area. The cell sheet migration rate (vmigration, μm min−1) and the time it takes for the gap to close to half the original area (t1/2gap, min) were calculated from the following equations:44
The initial gap area (Sinitial, μm2) and length of the wound (l, μm) were measured using ImageJ. The slope was obtained by plotting wound area versus time and fitted by a line to the data by using Microsoft Excel 2010 (Microsoft, Washington, USA).
Individual cell migration analysis was performed using the Manual Tracking plugin for ImageJ. Thirty individual cells were tracked for each sample to calculate mean individual cell migration velocity (μm min−1) and average directionality. The directionality was calculated as previously described:41
The accumulated distance (daccumulated, μm) was obtained by manual tracking. The Euclidean distance (dEuclidean, μm; the shortest linear distance travelled from start to endpoint of a tracked cell) was measured using the straight lines tool in ImageJ.
Power calculation and statistical analysis
Although changes in BOEC-related markers, including BOEC colony number, outgrowth efficiency and function were primary outcomes, they were not used for power calculation due to the absence of preliminary data. Retrospectively, a power analysis was calculated based on previous research, which reported that the number of circulating EPCs (CD34+ cells) increased after following a Mediterranean dietary pattern for 12 weeks (570.46 ± 153.8 to 2246.77 ± 301.5 cells/×106 peripheral blood cells, mean ± SEM).45 Presuming the current study yield similar results, with a two-tailed significance level of 0.05 and Cohen's effect size of 1.50, a sample size of ≥9 subjects per group will provide ≥80% power to confirm a statistical difference.
Normal distribution was verified with the D'Agostino–Pearson test or Kolmogorov–Smirnov test. Statistical comparison of pre- and post-intervention clinical data within groups was performed by paired t-test or Wilcoxon matched-pairs test (non-parametric). For comparisons of baseline data between groups and for BOEC data obtained from cell cultures, unpaired t-test or Mann–Whitney test (nonparametric) were conducted. Fisher's exact test was used for dichotomous variables (gender). Two-way ANOVA was used to determine the P values of time and interaction (intervention × time) effects. Spearman's coefficients correlation was calculated to assess the relationship between variables. Statistical analyses were performed using GraphPad Prism 8.0.1 (GraphPad Software Inc., California, USA) and SPSS for Windows V20 (SPSS Inc., Chicago, USA). Data were expressed as means ± SEM unless otherwise noted, and a two-sided P < 0.05 was considered statistically significant.
Results
Clinical characteristics and intervention compliance
Clinical characteristics and intervention compliance of subjects from HDPO and HDPW groups are shown in Table 1. There were no differences in the baseline characteristics of the subjects between the two groups. The changes in BMI and WC were not significant in both groups after the 16-week intervention.
Table 1 Clinical and dietary characteristics and plasma carotenoids concentrations of study subjects
Parameter |
HDPO (n = 9) |
HDPW (n = 15) |
Baseline |
Two-way ANOVA |
Pre- |
Post- |
P
|
Pre- |
Post- |
P
|
P
|
P
t
|
P
int
|
Values are presented as means ± SEM. “—”, not calculated. Statistical comparisons of pre-and post-intervention within groups were performed by paired t-test or Wilcoxon matched-pairs test. Comparison of baseline data between groups were performed by unpaired t-test or Mann–Whitney test. Fisher's exact test was used for dichotomous variables. Two-way ANOVA was used to determine the P values of time (Pt) and interaction (Pint) effects. Abbreviations: HDPO, healthy dietary pattern only; HDPW, HDP supplemented with wolfberry. |
Mean age (range, year) |
54 (50–59) |
— |
57 (50–63) |
— |
0.147 |
— |
— |
Female (n) |
7 |
— |
12 |
— |
>0.999c |
— |
— |
BMI (kg m−2) |
22.5 ± 1.1 |
22.5 ± 1.0 |
0.820 |
23.6 ± 0.9 |
23.7 ± 0.9 |
0.519 |
0.446 |
0.613 |
0.819 |
Waist circumference (cm) |
78.1 ± 2.5 |
78.5 ± 2.1 |
0.667 |
82.4 ± 2.2 |
82.2 ± 2.3 |
0.792 |
0.215 |
0.811 |
0.590 |
Diet (servings)
|
Whole grains |
0.9 ± 0.2 |
1.7 ± 0.3 |
0.035 |
0.4 ± 0.1 |
1.9 ± 0.5 |
0.001 |
0.040 |
0.003 |
0.319 |
Meats and alternatives |
2.9 ± 0.4 |
2.8 ± 0.3 |
0.637 |
2.7 ± 0.2 |
2.8 ± 0.4 |
0.873 |
0.633 |
0.853 |
0.686 |
Fruits |
2.3 ± 0.4 |
3.1 ± 0.2 |
0.049 |
2.1 ± 0.3 |
3.2 ± 0.4 |
0.015 |
0.687 |
0.004 |
0.571 |
Vegetables |
2.5 ± 0.5 |
2.7 ± 0.4 |
0.339 |
2.6 ± 0.5 |
2.8 ± 0.4 |
0.561 |
0.843 |
0.231 |
0.856 |
Fruits and vegetables |
4.8 ± 0.7 |
5.9 ± 0.5 |
0.055 |
4.7 ± 0.7 |
6.1 ± 0.6 |
0.014 |
0.949 |
0.003 |
0.709 |
Diet macronutrient (g)
|
Protein |
85.0 ± 7.1 |
92.7 ± 12.7 |
0.359 |
99.9 ± 17.1 |
83.6 ± 7.4 |
0.489 |
0.953 |
0.690 |
0.270 |
Carbohydrates |
187.1 ± 16.9 |
199.4 ± 21.2 |
0.426 |
224.9 ± 14.8 |
215.1 ± 19.0 |
0.561 |
0.109 |
0.934 |
0.465 |
Fat |
67.2 ± 6.3 |
65.5 ± 11.4 |
0.652 |
78.0 ± 9.7 |
58.7 ± 5.2 |
0.022 |
0.640 |
0.162 |
0.242 |
Plasma carotenoids (nmol L
−1
)
|
β-Carotene |
763.7 ± 167.1 |
491.8 ± 103.1 |
0.101 |
379.5 ± 44.8 |
438.3 ± 86.6 |
0.762 |
0.012 |
0.199 |
0.052 |
α-Carotene |
333.2 ± 42.2 |
252.9 ± 38.4 |
0.044 |
254.4 ± 31.8 |
262.1 ± 40.1 |
0.848 |
0.147 |
0.221 |
0.140 |
Lycopene |
432.6 ± 112.2 |
224.1 ± 62.6 |
0.008 |
331.2 ± 62.7 |
297.4 ± 60.0 |
0.542 |
0.401 |
0.020 |
0.085 |
β-Cryptoxanthin |
734.5 ± 180.6 |
481.1 ± 98.4 |
0.004 |
468.1 ± 68.0 |
414.2 ± 86.8 |
0.525 |
0.238 |
0.033 |
0.153 |
Lutein |
359.0 ± 32.7 |
347.1 ± 42.8 |
0.697 |
337.5 ± 41.9 |
368.0 ± 47.8 |
0.639 |
0.318 |
0.828 |
0.622 |
Zeaxanthin |
143.2 ± 10.2 |
141.0 ± 11.3 |
0.884 |
142.8 ± 9.6 |
226.2 ± 24.8 |
0.007 |
0.980 |
0.034 |
0.026 |
Total carotenoids |
2766.2 ± 360.0 |
1937.9 ± 270.3 |
0.022 |
1913.5 ± 205.2 |
2006.1 ± 286.7 |
0.755 |
0.037 |
0.109 |
0.048 |
Significant time effects (P < 0.05) were observed in the consumption of whole grains, fruits and total fruits and vegetables after interventions. Significant interaction effects were observed in plasma concentrations of total carotenoids (P = 0.048) and zeaxanthin (P = 0.026), which partly indicated the compliance of wolfberry intake, and only the HDPW group had a significant increase in zeaxanthin (Pre-: 142.8 ± 9.6 mmol L−1versus Post-: 226.2 ± 24.8 mmol L−1; P = 0.007).
CVD risk and vascular health
Changes in blood pressures, blood lipid-lipoprotein concentrations as well as the AI, vascular ages and Framingham risk scores are presented in Table 2. No time effects were observed in blood lipid-lipoprotein concentrations while the HDPW group had a significant increase in HDL-C concentration (1.5 ± 0.1 mmol L−1versus 1.6 ± 0.1 mmol L−1; P = 0.045). No differences were observed in other CVD risk-/vascular health-related outcomes, including blood pressures, AI, vascular age, Framingham risk scores, plasma concentrations of ANG1, ANG2 and VWF, brachial artery FMD and carotid IMT.
Table 2 CVD risk and vascular health of subjects
Parameter |
HDPO (n = 9) |
HDPW (n = 15) |
Pre- |
Post- |
P
|
Pre- |
Post- |
P
|
Values are presented as means ± SEM. Statistical comparisons of pre-and post-intervention within groups were performed by paired t-test or Wilcoxon matched-pairs test. No significant differences were observed from baseline data comparisons within groups and two-way ANOVA analyses (data not shown). Abbreviations: AI, atherogenic index; ANG1, angiopoietin-1; ANG2, angiopoietin-2; CVD, cardiovascular disease; DBP, diastolic blood pressure; FMD, flow-mediated dilatation; HDL-C, high-density lipoprotein cholesterol; HDPO, the healthy dietary pattern only; HDPW, HDP supplemented with wolfberry; IMT, intima-media thickness; LDL-C, low-density lipoprotein cholesterol; SBP, systolic blood pressure; TC, total cholesterol; VWF, von Willebrand factor. |
SBP (mmHg) |
105 ± 5 |
104 ± 4 |
0.741 |
114 ± 5 |
111 ± 4 |
0.247 |
DBP (mmHg) |
68 ± 4 |
66 ± 3 |
0.343 |
74 ± 3 |
74 ± 2 |
0.987 |
Triglyceride (mmol L−1) |
1.1 ± 0.2 |
1.2 ± 0.1 |
0.119 |
1.3 ± 0.2 |
1.1 ± 0.1 |
0.336 |
TC (mmol L−1) |
5.5 ± 0.5 |
5.4 ± 0.5 |
0.331 |
5.2 ± 0.2 |
5.4 ± 0.2 |
0.197 |
LDL-C (mmol L−1) |
3.4 ± 0.5 |
3.2 ± 0.5 |
0.188 |
3.1 ± 0.2 |
3.2 ± 0.2 |
0.257 |
HDL-C (mmol L−1) |
1.6 ± 0.1 |
1.6 ± 0.1 |
>0.999 |
1.5 ± 0.1 |
1.6 ± 0.1 |
0.045 |
AI |
2.6 ± 0.4 |
2.5 ± 0.4 |
0.432 |
2.7 ± 0.3 |
2.5 ± 0.2 |
0.171 |
Vascular age (year) |
43.9 ± 3.7 |
43.1 ± 3.2 |
0.427 |
50.4 ± 3.0 |
48.4 ± 2.2 |
0.172 |
Framingham risk score (%) |
4.5 ± 1.2 |
4.2 ± 1.2 |
0.301 |
6.1 ± 1.4 |
5.1 ± 0.9 |
0.164 |
ANG1 (ng mL−1) |
1.2 ± 0.4 |
1.8 ± 0.4 |
0.271 |
1.6 ± 0.3 |
1.8 ± 0.3 |
0.547 |
ANG2 (ng mL−1) |
1.2 ± 0.1 |
1.3 ± 0.2 |
0.373 |
1.1 ± 0.1 |
1.1 ± 0.1 |
0.136 |
VWF (IU mL−1) |
2.7 ± 0.3 |
2.3 ± 0.3 |
0.292 |
2.2 ± 0.2 |
2.1 ± 0.2 |
0.719 |
FMD (%) |
3.9 ± 1.4 |
6.0 ± 0.7 |
0.098 |
5.0 ± 0.9 |
3.7 ± 0.6 |
0.389 |
IMT (mm) |
0.7 ± 0.0 |
0.7 ± 0.0 |
0.760 |
0.7 ± 0.0 |
0.7 ± 0.0 |
0.575 |
BOEC outgrowth efficiency
BOEC colonies with cobblestone-like morphology appeared in cell culture between days 9 and 29 (median day 16) (Fig. 1a–c). Differences in the colony's proliferation potential and colony's growth rate were shown by colony numbers (Fig. 1d) and their appearance date and growth time (Fig. 1e and Fig. S3a–S3c†), respectively. Zero to 10 colonies were obtained from each sample and colonies were successfully derived from 41 out of the total 48 samples (success rate = 85.4%). No changes in colony number and MNC number (Fig. S3d†) were observed in both groups. Significant time effects were observed in colony's culture time between passages 1 and 2 (HDPO group: from 5.8 ± 0.5 day to 4.5 ± 1.1 day and HDPW group: from 5.3 ± 0.8 day to 3.6 ± 0.5 day; P = 0.038) (Fig. 1e). Discrepancies in the sample size for subsequent evaluations were attributed to the gradual loss in capacity among BOECs over subsequent cell passages.
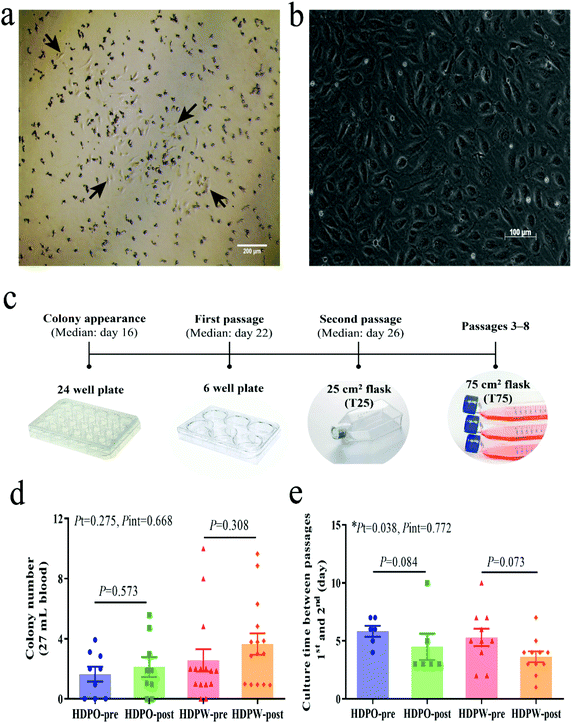 |
| Fig. 1 Isolation and enumeration of BOECs. (a) Representative images of BOEC colony upon appearance (day 15). Arrows point the cells at the colony edge. (b) Cobblestone-like morphology of BOECs under a phase-contrast microscope. (c) BOEC culture flow diagram. (d) BOECs proliferation potential is shown by colony numbers. Subject numbers in HDPO and HDPW groups were 9 and 15, respectively. (e) The growth rate of BOECs is shown by growth time. Graphs are plotted as means with SEM. Statistical comparisons of pre-and post-intervention within groups were performed by unpaired t-test or Mann–Whitney test. Two-way ANOVA was used to determine the P values of time (Pt) and interaction (Pint) effects. *Statistical significance at P < 0.05. Abbreviations: BOEC, blood outgrowth endothelial cell; HDPO, the healthy dietary pattern only; HDPW, HDP supplemented with wolfberry. | |
BOEC characterization
Immunofluorescence staining results showed that the blood-derived BOECs expressed VWF (Fig. 2a). As reported in Fig. 2b and Table S1,† BOECs uniformly and consistently expressed KDR (endothelial cell surface marker), while their expression of CD34 (progenitor cell surface marker) varied (9.0%–99.9%). The number of circulating EPCs (CD34+ and/or KDR+ cell populations) in MNCs was also examined (Fig. 2c and Table S1†) and the CD34+, KDR+ and double-positive fractions were 0.9%–67.8%, 0.7%–3.7% and 0.0%–1.6%, respectively. No changes in CD34 and KDR expression were observed in BOECs and MNCs following the dietary intervention.
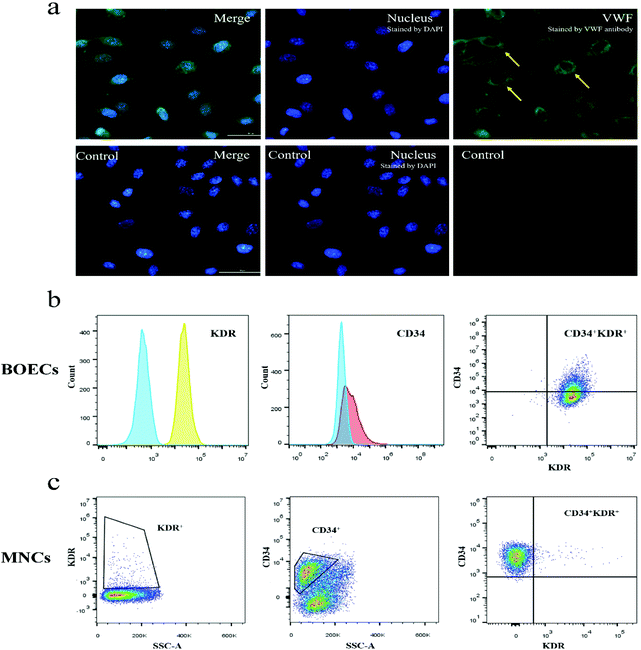 |
| Fig. 2 Phenotypic analyses of BOECs. (a) Immunofluorescence staining shows that BOECs express VWF. Yellow arrows at the upper-right panel point the VWF structures (green, stained with VWF antibody). Lower panels show BOECs only stained by DAPI (blue, as control). Scale bar, 50 μm. (b) Flow cytometric analysis of BOECs. The representative data demonstrate that BOECs were positive for endothelial cell marker (KDR) and a population of the cells positive for progenitor cell marker (CD34). Negative controls are overlaid as areas filled with blue on each histogram. At the intersection of the KDR and CD34 gates, we identified CD34+KDR+ cells in the upper-right quadrant. (c) Enumeration of circulating progenitor cells in MNCs by flow cytometry. KDR+ and CD34+ cells were gated from the MNC fraction. CD34+KDR+ cells were identified in the CD34+ gate as cells positive for KDR. Abbreviations: BOECs, blood outgrowth endothelial cells; DAPI, 4′,6-diamidino-2-phenylindole; MNCs, mononuclear cells; SSC-A, side-scatter area; VWF, von Willebrand factor. | |
Tube formation capacity of BOECs
Representative images of the capillary-like structure of BOECs are given in Fig. S4† and quantified morphological features of the structures are reported in Table 3 and Table S2.† The common parameters shown in Table 3 demonstrated that both HDPO and HDPW groups increased the overall tube formation capacity, including the number/length/area of master segments and meshes. A significant time effect (P = 0.032) was observed in the number of extremities, which were inversely correlated with mean mesh size (r = −0.466, P = 0.011) and it was more prominent in the HDPO group, which showed a significant decrease (P = 0.046).
Table 3 Characterization of capillary-like tubules of BOECs from different groups
Parameter |
HDPO |
HDPW |
Pre- (n = 5) |
Post- (n = 5) |
Change (%) |
P
|
Pre- (n = 8) |
Post- (n = 11) |
Change (%) |
P
|
The picture analysis area was 3.50 mm2. Values are presented as means ± SEM. Statistical comparison of pre-and post-intervention within groups was performed by unpaired t-test or Mann–Whitney test. No significant differences were observed at baseline data within groups (data not shown). A significant time effect was observed from the number of extremities (P = 0.032) by using two-way ANOVA. Total branching length means the sum of the length of the trees composed from segments and branches in the analyzed area. Abbreviations: BOECs, blood outgrowth endothelial cells; HDPO, the healthy dietary pattern only; HDPW, HDP supplemented with wolfberry. |
Number extremities |
77.6 ± 4.7 |
64.6 ± 2.9 |
−16.8 |
0.046 |
84.0 ± 7.4 |
73.1 ± 2.7 |
−13.0 |
0.152 |
Number master junctions |
21.0 ± 6.9 |
21.0 ± 4.5 |
−0.1 |
0.997 |
22.9 ± 2.3 |
25.6 ± 3.3 |
11.9 |
0.544 |
Number master segments |
33.7 ± 11.9 |
34.0 ± 8.2 |
0.8 |
0.985 |
38.6 ± 4.2 |
42.4 ± 5.8 |
9.9 |
0.627 |
Number meshes |
9.9 ± 4.3 |
10.1 ± 2.9 |
1.3 |
0.981 |
11.1 ± 1.5 |
12.9 ± 2.1 |
16.8 |
0.516 |
Total master segment length (mm) |
8.4 ± 2.4 |
8.9 ± 2.0 |
5.9 |
0.877 |
9.3 ± 0.9 |
10.4 ± 1.2 |
11.6 |
0.505 |
Total branching length (mm) |
14.2 ± 2.6 |
14.5 ± 2.2 |
2.1 |
0.933 |
16.0 ± 1.1 |
16.4 ± 1.4 |
2.8 |
0.813 |
Total mesh area (mm2) |
0.5 ± 0.3 |
0.6 ± 0.2 |
24.9 |
0.488 |
0.5 ± 0.1 |
0.6 ± 0.1 |
38.3 |
0.327 |
Mean mesh size (mm2) |
0.034 ± 0.009 |
0.047 ± 0.012 |
41.2 |
0.385 |
0.039 ± 0.007 |
0.045 ± 0.005 |
17.5 |
0.432 |
Cell migration activity of BOECs
Wound images at different time points in Fig. 3a represent the migration ability of BOECs. The vmigration (cell sheet migration rate) and t1/2gap (the time it takes to close half of the gap) of BOECs were 0.2–0.5 (0.3 ± 0.1, mean ± SD) μm min−1 and 111.7–354.0 (181.2 ± 58.5, mean ± SD) min, respectively (Fig. 3b). A significant time effect was observed in the t1/2gap (HDPO group: from 227.6 ± 36.9 min to 165.5 ± 10.1 min and HDPW group: from 194.7 ± 29.4 min to 161.2 ± 12.9 min; P = 0.040) after the intervention. Results of individual cell migration velocity and directionality are shown in Fig. 3c and no change was observed in either group.
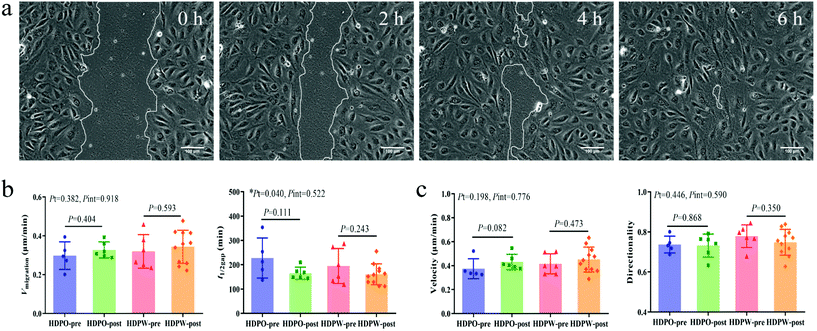 |
| Fig. 3 Cell migration activity of BOECs. (a) Images from a wound healing assay at different time points. Representative BOECs were able to migrate and close the open area after around 6 h. (b) Sheet migration activity and (c) individual cell migration activity of BOECs. Sample number in HDPO-pre, HDPO-post, HDPW-pre and HDPW-post groups were 5, 6, 6 and 11, respectively. Graphs are plotted as means with SEM. Statistical comparisons of pre- and post-intervention within groups were performed by unpaired t-test or Mann–Whitney test. Two-way ANOVA was used to determine the P values of time (Pt) and interaction (Pint) effects. *Statistically significance at P < 0.05. Abbreviations: BOEC, blood outgrowth endothelial cell; HDPO, healthy dietary pattern only; HDPW, HDP supplemented with wolfberry. | |
Correlation analysis
Correlations between CVD risk-/vascular health-related markers and circulating EPC-/BOEC-related markers were illustrated by the heatmap correlation matrices (Fig. 4). The BOEC colony number was positively associated with SBP (r = 0.393, P = 0.006), DBP (r = 0.385, P = 0.007), AI (r = 0.396, P = 0.005), vascular age (r = 0.405, P = 0.004) and Framingham CVD risk scores (r = 0.399, P = 0.005). ANG2 concentration was negatively correlated to KDR+ circulating EPCs (r = −0.342, P = 0.027) and BOECs’ tube branch numbers (r = −0.479, P = 0.009).
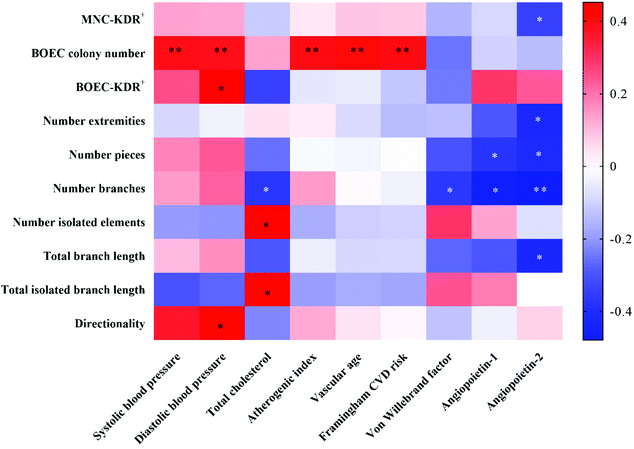 |
| Fig. 4 Heatmap of the significant correlations among clinical markers of CVD with circulating EPC- and BOEC-related markers. Spearman nonparametric correlation was conducted. The r values between variables are transformed into color scale, with stronger negative correlations are displayed in blue, while stronger positive correlations are shown in red. *, P < 0.05; **, P < 0.01. Abbreviations: BOEC, blood outgrowth endothelial cell; CVD, cardiovascular disease; EPCs, endothelial progenitor cells; MNCs, mononuclear cells. | |
Correlations among circulating EPC- and BOEC-related markers were also assessed. The time to the first BOEC colony appearance was negatively correlated with colony number (r = −0.628, P < 0.001) and cell velocity (r = −0.486, P = 0.009). Significant positive correlations were detected between BOECs’ KDR expression and cell directionality (r = 0.516, P = 0.010). No significant correlation was observed between the numbers of circulating EPCs and BOECs.
Discussion
Adherence to the HDP is recommended to combat CVDs and it has recently been shown that certain EPC types, including circulating EPCs and CACs, can be influenced by following an HDP or consuming functional food components.4,5,13,18,46 Meanwhile, previous evidence supports that regular consumption of wolfberry can also improve blood lipid-lipoprotein profile, which plays an essential role in the development of CVDs.23,47 However, there is a lack of data explaining the effect of following HDP with co-consuming wolfberry on BOECs’ number and function, especially in middle-aged and older adults who are at higher risk of CVD development.48 In the present study, we assessed the effect of consuming HDP with wolfberry on BOECs’ number and function in middle-aged and older Singaporean adults. Our results demonstrated that adherence to an HDP improved BOEC colony growth rate (Fig. 1e), angiogenic capability (Table 3) and migration behavior (Fig. 3b) without changing BOEC colony number (Fig. 1d), however, incorporating wolfberry into an HDP did not show an additional effect on BOECs’ outgrowth efficiency and function. Moreover, we found that the BOEC colony number was positively associated with CVD risk-related markers (Fig. 4).
We did not observe improvements in CVD risk- and vascular health-related markers after diet intervention, except for wolfberry consumption improved blood HDL-C concentration (Table 2). Previous evidence showed that administration of wolfberry polysaccharides to hyperlipidemic rabbits49 and type 2 diabetic patients25 or administration of wolfberry zeaxanthin to diabetic rat model50 enhanced HDL-C levels, indicating that HDL-C beneficial effect of wolfberry may be linked to bioactive compounds that are abundant in wolfberry.
Faris et al.51 summarized characterizations of BOECs. Briefly, BOECs are isolated from the MNC fraction of peripheral or umbilical cord blood with a long-term ex vivo culture under endothelial conditions. Moreover, BOECs have a typical cobblestone-like morphology as well as express typical endothelial cell markers, while they lack hematopoietic and mesenchymal stem cell antigens. Here, we successfully isolated BOECs from peripheral blood of middle-aged and older adults and the success rate (85.4%) is similar to that reported previously with success rates ranging from 72% to 85%.11,12 No changes in BOEC colony number as well as circulating EPC number were observed. Although to date, the effect of diet on BOEC colony number is unavailable, a few studies have investigated the effect of following an HDP or supplementing food bioactive components on circulating EPCs and showed inconsistent findings. Among them, no change in circulating EPCs was observed after consuming fish oil,52 green tea,53 or cranberry juice,54 however, increased trends were reported with the consumption of Mediterranean diet,4,15,45 onion peel extract5 and unsaturated fats.14,16 These mixed results might be due to different subjects’ health status and/or involvement of different types of dietary intervention. A shorter growth time indicates a faster growth rate and we found that HDP intervention improved the BOEC colony's growth rate. Paschalaki et al.2 observed that patients with chronic obstructive pulmonary disease, a disease with high CVD mortality, showed a slower BOEC growth rate. These findings suggest that the accelerated growth rate of BOEC reflect a favorable cardiovascular protective effect of HDP.
Tube formation assay and wound-healing assay were performed to measure BOECs’ function. The ability to form tubes is specific to endothelial cells55 and the duration of the assay varies from 3 to 24 hours.42 For BOECs, 4–6 hours40versus 24 hours41 have been used earlier to examine their tube formation ability and we optimized the assay duration as 6 hours since tubes had a maximal branching-time of over 6 h, after which they start to retract away from each other (Fig. S2†). Endothelial cell migration is an important process in angiogenesis and the repair of damaged blood vessels.56 Together, we found that following an HDP, the tube formation capacity of BOECs and cell sheet migration activity were improved while co-consuming wolfberry did not provide any additional improvement. Although there are currently no data explaining effects of dietary interventions on BOECs’ function, previous evidence based on human subjects, animal models or in vitro cell treatments indicated that certain food or food compounds, including red wine,57,58 whole grain,59 fish oil,60 anthocyanin61 and polyphenol62 prevented dysfunction or improved angiogenesis and/or migration function of CACs and CFU-ECs. Moreover, several studies tested the direct effect of red wine,63 n-3 polyunsaturated fatty acids16 and vitamin D64 on the function of BOECs using in vitro cell treatments and reported enhanced adhesion, migration, viability and tube formation capacity. Collectively, these findings support our result that following an HDP, which encompasses multiple food or nutrients including whole grain, polyunsaturated fat, anthocyanin and polyphenol, provided favorable effects on BOECs’ function. While no additional effect on the number or function of BOECs were observed with supplementation of 15 g day−1 of wolfberries. There are several possibilities that might account for this result: the amount of wolfberry applied in this study was determined based on its benefits in blood lipid-lipoprotein management and the intake of zeaxanthin,23,31 while there are no preliminary data to determine an optimal amount of wolfberry on BOECs. Therefore, the amount of wolfberry used may not be sufficient to detect changes in BOECs. In addition, EPCs and BOECs are very rare in peripheral circulation,65 hence, it may be difficult to detect obvious changes. Moreover, active components of HDP may compete with wolfberry or cover up its benefit. A further study investigating the BOEC benefit of wolfberry with a habitual diet is warranted to convey wolfberry's benefit with greater clarity.
Correlation analysis revealed that BOEC colony number is positively associated with CVD risk-related markers including blood pressure, AI, vascular age and Framingham CVD risk scores. These results indicate a remarkable increase in BOEC numbers is an indicator of higher CVD risk. Consistently, Guven et al.10 cultured BOECs from patients with coronary artery disease and reported that the number of BOECs was found to increase in relation to disease severity. In contrast, no correlation between circulating EPCs numbers and CVD risk- and vascular health-related markers, except ANG2, were observed in this study. Although the exact role of ANG2 in angiogenesis has not yet been completely elucidated, clinical studies have reported that ANG2 disturbed the endothelial cell monolayer integrity and high plasma ANG2 have a predictive value of CVDs.66,67 A number of earlier studies have correlated EPCs with cardiovascular outcomes and although an inverse correlation was generally reported,1,8,13,14,68 positive correlations have also been reported.69–72 Overall, the relationship between EPCs and vascular health remains controversial and incompletely understood.8,10 Interestingly, we did not observe any correlation between the number of circulating EPCs and BOEC colonies. In fact, there is a lack of a standard for the identification of circulating EPCs. Although CD34, CD133 and KDR are generally used to define circulating EPCs, evidence shows that CD34, CD133 and KDR are also expressed on human hematopoietic stem cells and various subpopulations of hematopoietic progenitor cells.8 Therefore, no correlation between the number of BOEC colonies and circulating EPCs may suggest that the circulating EPCs defined by flow cytometry do not predict for BOECs. The discrepancy of correlation between BOECs and EPCs in connection with CVD risk- and vascular health-related markers in this study also supports this inference. Further investigation is required to distinguish the role of BOECs and circulating EPCs in the cardiovascular system.
This is the first randomized controlled trial that investigated the effect of following an HDP with or without adding wolfberry on BOECs’ number and function in middle-aged and older adults and all subjects reported good intervention compliance. Although previous studies assessed the effect of certain foods on circulating EPCs, CACs or CFU-ECs, notably, our study mainly focused on BOECs, the true EPCs. In addition, tube formation and migration activities, two hallmarks of endothelial cell behavior were also investigated, providing a mechanistic dissection of the beneficial effects on BOEC following the intervention. Furthermore, assessment of the associations between BOEC-related markers and CVD risk- and vascular health-related markers can provide an understanding of the role of BOECs in connection to the cardiovascular system.
Nevertheless, there are some limitations to our study. First, the number of subjects was relatively small and the sample number for BOEC characterization was nonuniform due to a gradual loss in proliferative ability over subsequent cell passages, thereby some comparisons may lack the power to detect statistical significance. Second, the ex vivo isolation and culturing of BOECs are applied to differentiate medium and manipulations, which may not reflect the real in vivo condition. Third, the intervention duration (16 weeks) was relatively long as compared to previous studies; however, it might not be sufficient to obtain significant changes in certain slow progressed markers, such as IMT. Lastly, our subjects were relatively healthy individuals and there may be a lesser chance to observe changes in BOECs after diet intervention since changes in BOECs are more observed in patients with CVDs.10,11
Conclusions
In summary, the adherence to an HDP improved BOECs’ function while incorporating 15 g day−1 of wolfberry did not provide additional benefits in middle-aged and older adults. These findings suggest the importance of dietary pattern modification to improve cardiovascular health in view of the BOEC involved mechanism. Associations between BOEC colony numbers with CVD risk-related markers also support the remarkable plasticity of BOECs as a novel biomarker for the investigation of cardiovascular-related health outcomes.
Abbreviations
AI | Atherogenic index |
ANG1 | Angiopoietin-1 |
ANG2 | Angiopoietin-2 |
BOECs | Blood outgrowth endothelial cells |
CACs | Circulating angiogenic cells |
CFU-ECs | Colony-forming unit-endothelial cells |
CVD | Cardiovascular disease |
DBP | Diastolic blood pressure |
EPCs | Endothelial progenitor cells |
FMD | Flow-mediated dilatation |
HDL-C | High-density lipoprotein cholesterol |
HDP | Healthy dietary pattern |
HDPO | Healthy dietary pattern only |
HDPW | HDP supplemented with wolfberry |
IMT | Intima-media thickness |
LDL-C | Low-density lipoprotein cholesterol |
MNCs | Mononuclear cells |
SBP | Systolic blood pressure |
TC | Total cholesterol |
VWF | von Willebrand factor |
WC | Waist circumference |
Author contributions
J. E. K. and K. K. P. designed the study; X. X. and J. W. W. set up the methods; X. X. and D. W. K. T. conducted the research and analyzed the data; S. L. N. and O. Z. helped with the experiments; X. X. wrote the manuscript. R. S. Y. F. provided important technical supports; X. X. and J. E. K. had primary responsibility for final content. All authors read and approved the final manuscript.
Conflicts of interest
There are no conflicts of interest to declare.
Acknowledgements
We thank Jing Xuan Wong, Rongrong Zhao, Matthew Ackers-Johnson and Chen Yuan Huang for their technical assistance, Clarinda Nataria Sutanto, Yuanhang Yao, Delia Pei Shan Lee, Jasmine Hui Min Low and Denise Tan for their help with the subject recruitment; Guannan Li for his statistical advice. This work was supported by the National University of Singapore [R160-000-A03-133], the Singapore Ministry of Education Academic Research Fund Tier 1 [R160-000-A26-114] and the Singapore Ministry of Health's National Medical Research Council [NMRC/OFYIRG/0081/2018].
References
- G. P. Fadini, S. V. de Kreutzenberg, A. Coracina, I. Baesso, C. Agostini, A. Tiengo and A. Avogaro, Circulating CD34+ cells, metabolic syndrome, and cardiovascular risk, Eur. Heart J., 2006, 27, 2247–2255 CrossRef CAS PubMed.
- K. E. Paschalaki, R. D. Starke, Y. Hu, N. Mercado, A. Margariti, V. G. Gorgoulis, A. M. Randi and P. J. Barnes, Dysfunction of endothelial progenitor cells from smokers and chronic obstructive pulmonary disease patients due to increased DNA damage and senescence, Stem Cells, 2013, 31, 2813–2826 CrossRef CAS PubMed.
- K. Esposito, M. Ciotola, M. I. Maiorino, F. Giugliano, R. Autorino, M. De Sio, E. Jannini, A. Lenzi and D. Giugliano, Circulating CD34+ KDR+ endothelial progenitor cells correlate with erectile function and endothelial function in overweight men, J. Sex. Med., 2009, 6, 107–114 CrossRef PubMed.
- M. I. Maiorino, G. Bellastella, M. Petrizzo, M. Gicchino, M. Caputo, D. Giugliano and K. Esposito, Effect of a Mediterranean diet on endothelial progenitor cells and carotid intima-media thickness in type 2 diabetes: Follow-up of a randomized trial, Eur. J. Prev. Cardiol., 2017, 24, 399–408 CrossRef PubMed.
- E. Y. Choi, H. Lee, J. S. Woo, H. H. Jang, S. J. Hwang, H. S. Kim, W. S. Kim, Y. S. Kim, R. Choue, Y. J. Cha, J. E. Yim and W. Kim, Effect of onion peel extract on endothelial function and endothelial progenitor cells in overweight and obese individuals, Nutrition, 2015, 31, 1131–1135 CrossRef CAS PubMed.
- P. S. Lee and K. K. Poh, Endothelial progenitor cells in cardiovascular diseases, World J. Stem Cells, 2014, 6, 355–366 CrossRef PubMed.
- D. A. Ingram, L. E. Mead, D. B. Moore, W. Woodard, A. Fenoglio and M. C. Yoder, Vessel wall-derived endothelial cells rapidly proliferate because they contain a complete hierarchy of endothelial progenitor cells, Blood, 2005, 105, 2783–2786 CrossRef CAS PubMed.
- D. N. Prater, J. Case, D. A. Ingram and M. C. Yoder, Working hypothesis to redefine endothelial progenitor cells, Leukemia, 2007, 21, 1141–1149 CrossRef CAS PubMed.
- M. C. Yoder, L. E. Mead, D. Prater, T. R. Krier, K. N. Mroueh, F. Li, R. Krasich, C. J. Temm, J. T. Prchal and D. A. Ingram, Redefining endothelial progenitor cells via clonal analysis and hematopoietic stem/progenitor cell principals, Blood, 2007, 109, 1801–1809 CrossRef CAS PubMed.
- H. Guven, R. M. Shepherd, R. G. Bach, B. J. Capoccia and D. C. Link, The number of endothelial progenitor cell colonies in the blood is increased in patients with angiographically significant coronary artery disease, J. Am. Coll. Cardiol., 2006, 48, 1579–1587 CrossRef PubMed.
- D. Dauwe, B. Pelacho, A. Wibowo, A. S. Walravens, K. Verdonck, H. Gillijns, E. Caluwe, P. Pokreisz, N. van Gastel, G. Carmeliet, M. Depypere, F. Maes, N. Vanden Driessche, W. Droogne, J. Van Cleemput, J. Vanhaecke, F. Prosper, C. Verfaillie, A. Luttun and S. Janssens, Neovascularization Potential of Blood Outgrowth Endothelial Cells From Patients With Stable Ischemic Heart Failure Is Preserved, J. Am. Heart Assoc., 2016, 5, e002288 Search PubMed.
- J. Martin-Ramirez, M. Hofman, M. van den Biggelaar, R. P. Hebbel and J. Voorberg, Establishment of outgrowth endothelial cells from peripheral blood, Nat. Protoc., 2012, 7, 1709–1715 CrossRef CAS PubMed.
- R. Mano, A. Ishida, Y. Ohya, H. Todoriki and S. Takishita, Dietary intervention with Okinawan vegetables increased circulating endothelial progenitor cells in healthy young women, Atherosclerosis, 2009, 204, 544–548 CrossRef CAS PubMed.
- M. Weech, H. Altowaijri, J. Mayneris-Perxachs, K. Vafeiadou, J. Madden, S. Todd, K. G. Jackson, J. A. Lovegrove and P. Yaqoob, Replacement of dietary saturated fat with unsaturated fats increases numbers of circulating endothelial progenitor cells and decreases numbers of microparticles: findings from the randomized, controlled Dietary Intervention and VAScular function (DIVAS) study, Am. J. Clin. Nutr., 2018, 107, 876–882 CrossRef PubMed.
- F. Cesari, F. Sofi, R. Molino Lova, F. Vannetti, G. Pasquini, F. Cecchi, R. Marcucci, A. M. Gori, C. Macchi and Mugello Study Working G, Aging process, adherence to Mediterranean diet and nutritional status in a large cohort of nonagenarians: Effects on endothelial progenitor cells, Nutr., Metab. Cardiovasc. Dis., 2018, 28, 84–90 CrossRef CAS PubMed.
- V. Spigoni, C. Lombardi, M. Cito, A. Picconi, V. Ridolfi, R. Andreoli, N. Anelli, L. Gnudi, M. Goldoni, I. Zavaroni, R. Raddino and A. Dei Cas, N-3 PUFA increase bioavailability and function of endothelial progenitor cells, Food Funct., 2014, 5, 1881–1890 RSC.
- F. Felice, A. Francini, V. Domenici, M. Cifelli, E. Belardinelli, L. Sebastiani, C. Cantini and R. Di Stefano, Effects of Extra Virgin Olive Oil and Apples Enriched-Dark Chocolate on Endothelial Progenitor Cells in Patients with Cardiovascular Risk Factors: A Randomized Cross-Over Trial, Antioxidants, 2019, 8, 88 CrossRef CAS PubMed.
- Y. H. Chan, T. H. Lam, K. K. Lau, K. H. Yiu, C. W. Siu, S. W. Li, H. T. Chan, S. Tam, C. P. Lau and H. F. Tse, Dietary intake of phytoestrogen is associated with increased circulating endothelial progenitor cells in patients with cardiovascular disease, Eur. J. Cardiovasc. Prev. Rehabil., 2011, 18, 360–368 CrossRef PubMed.
- C. Heiss, S. Jahn, M. Taylor, W. M. Real, F. S. Angeli, M. L. Wong, N. Amabile, M. Prasad, T. Rassaf, J. I. Ottaviani, S. Mihardja, C. L. Keen, M. L. Springer, A. Boyle, W. Grossman, S. A. Glantz, H. Schroeter and Y. Yeghiazarians, Improvement of endothelial function with dietary flavanols is associated with mobilization of circulating angiogenic cells in patients with coronary artery disease, J. Am. Coll. Cardiol., 2010, 56, 218–224 CrossRef CAS PubMed.
- W. Kim, M. H. Jeong, S. H. Cho, J. H. Yun, H. J. Chae, Y. K. Ahn, M. C. Lee, X. Cheng, T. Kondo, T. Murohara and J. C. Kang, Effect of Green Tea Consumption on Endothelial Function and Circulating Endothelial Progenitor Cells in Chronic Smokers, Circ. J., 2006, 70, 1052–1057 CrossRef CAS PubMed.
- I. Bondia-Pons, O. Savolainen, R. Törrönen, J. A. Martinez, K. Poutanen and K. Hanhineva, Metabolic profiling of Goji berry extracts for discrimination of geographical origin by non-targeted liquid chromatography coupled to quadrupole time-of-flight mass spectrometry, Food Res. Int., 2014, 63, 132–138 CrossRef CAS.
- D. W. K. Toh, X. Xia, C. N. Sutanto, J. H. M. Low, K. K. Poh, J. W. Wang, R. S. Foo and J. E. Kim, Enhancing the cardiovascular protective effects of a healthy dietary pattern with wolfberry (Lycium barbarum): A randomized controlled trial, Am. J. Clin. Nutr., 2021, 114, 80–89 CrossRef PubMed.
- M. Z. de Souza Zanchet, G. M. Nardi, L. de Oliveira Souza Bratti, F. B. Filippin-Monteiro and C. Locatelli,
Lycium barbarum Reduces Abdominal Fat and Improves Lipid Profile and Antioxidant Status in Patients with Metabolic Syndrome, Oxid. Med. Cell. Longevity, 2017, 2017, 9763210 Search PubMed.
- D. H. Yu, J. M. Wu and A. J. Niu, Health-promoting effect of LBP and healthy Qigong exercise on physiological functions in old subjects, Carbohydr. Polym., 2009, 75, 312–316 CrossRef CAS.
- H. Cai, F. Liu, P. Zuo, G. Huang, Z. Song, T. Wang, H. Lu, F. Guo, C. Han and G. Sun, Practical Application of Antidiabetic Efficacy of Lycium barbarum Polysaccharide in Patients with Type 2 Diabetes, Med. Chem., 2015, 11, 383–390 CrossRef CAS PubMed.
- D. W. K. Toh, W. Y. Lee, H. Zhou, C. N. Sutanto, D. P. S. Lee, D. Tan and J. E. Kim, Wolfberry (Lycium barbarum) Consumption with a Healthy Dietary Pattern Lowers Oxidative Stress in Middle-Aged and Older Adults: A Randomized Controlled Trial, Antioxidants, 2021, 10, 567 CrossRef CAS PubMed.
- S. Wu, Y. Wang, G. Gong, F. Li, H. Ren and Y. Liu, Adsorption and desorption properties of macroporous resins for flavonoids from the extract of Chinese wolfberry (Lycium barbarum L.), Food Bioprod. Process., 2015, 93, 148–155 CrossRef CAS.
- Y. J. Lee, Y. Ahn, O. Kwon, M. Y. Lee, C. H. Lee, S. Lee, T. Park, S. W. Kwon and J. Y. Kim, Dietary Wolfberry Extract Modifies Oxidative Stress by Controlling the Expression of Inflammatory mRNAs in Overweight and Hypercholesterolemic Subjects: A Randomized, Double-Blind, Placebo-Controlled Trial, J. Agric. Food Chem., 2017, 65, 309–316 CrossRef CAS PubMed.
- Health Promotion Board My Healthy Plate. https://www.healthhub.sg/programmes/55/my-healthy-plate (accessed 21 June 2020).
-
World Health Organization, Fruit and vegetables for health: Report of the Joint FAO; 9241592818, 2005 Search PubMed.
- C. Y. Cheng, W. Y. Chung, Y. T. Szeto and I. F. F. Benzie, Fasting plasma zeaxanthin response to Fructus barbarum L. (wolfberry; Kei Tze) in a food-based human supplementation trial, Br. J. Nutr., 2005, 93, 123–130 CrossRef CAS PubMed.
-
World Health Organization, Waist circumference and waist-hip ratio: Report of a WHO expert consultation, Geneva, 8–11 December 2008; 9241501499, 2011 Search PubMed.
- X. Xia, G. Li, Y. Ding, T. Ren, J. Zheng and J. Kan, Effect of Whole Grain Qingke (Tibetan Hordeum vulgare L. Zangqing 320) on the Serum Lipid Levels and Intestinal Microbiota of Rats under High-Fat Diet, J. Agric. Food Chem., 2017, 65, 2686–2693 CrossRef CAS PubMed.
- Cardiovascular Disease (10-year risk), https://www.framinghamheartstudy.org/fhs-risk-functions/cardiovascular-disease-10-year-risk/ (accessed 21 June 2020).
- A. Mak, N. Y. Kow, H. Schwarz, L. Gong, S. H. Tay and L. H. Ling, Endothelial dysfunction in systemic lupus erythematosus - a case-control study and an updated meta-analysis and meta-regression, Sci. Rep., 2017, 7, 7320 CrossRef PubMed.
- J. H. Stein, C. E. Korcarz, R. T. Hurst, E. Lonn, C. B. Kendall, E. R. Mohler, S. S. Najjar, C. M. Rembold and W. S. Post, American Society of Echocardiography Carotid Intima-Media Thickness Task F. Use of carotid ultrasound to identify subclinical vascular disease and evaluate cardiovascular disease risk: a consensus statement from the American Society of Echocardiography Carotid Intima-Media Thickness Task Force. Endorsed by the Society for Vascular Medicine, J. Am. Soc. Echocardiogr., 2008, 21, 93–111 CrossRef PubMed ; quiz 189–190.
- U.S. Department,[:] of Agriculture. FoodData Central [Internet]. U.S. Department of Agriculture. 2019. Available from: https://fdc.nal.usda.gov/.
- Health Promotion Board Singapore. Energy & nutrient composition of food [Internet]. Health Promotion Board Singapore. 2011. Available from: https://focos.hpb.gov.sg/eservices/ENCF/.
- D. W. K. Toh, W. W. Loh, C. N. Sutanto, Y. Yao and J. E. Kim, Skin carotenoid status and plasma carotenoids: biomarkers of dietary carotenoids, fruits and vegetables for middle-aged and older Singaporean adults, Br. J. Nutr., 2021, 1–10 Search PubMed.
- J. W. Wang, E. A. Bouwens, M. C. Pintao, J. Voorberg, H. Safdar, K. M. Valentijn, H. C. de Boer, K. Mertens, P. H. Reitsma and J. Eikenboom, Analysis of the storage and secretion of von Willebrand factor in blood outgrowth endothelial cells derived from patients
with von Willebrand disease, Blood, 2013, 121, 2762–2772 CrossRef CAS PubMed.
- D. J. Groeneveld, T. van Bekkum, R. J. Dirven, J. W. Wang, J. Voorberg, P. H. Reitsma and J. Eikenboom, Angiogenic characteristics of blood outgrowth endothelial cells from patients with von Willebrand disease, J. Thromb. Haemostasis, 2015, 13, 1854–1866 CrossRef CAS PubMed.
- I. Arnaoutova and H. K. Kleinman,
In vitro angiogenesis: endothelial cell tube formation on gelled basement membrane extract, Nat. Protoc., 2010, 5, 628–635 CrossRef CAS PubMed.
- J. Schindelin, I. Arganda-Carreras, E. Frise, V. Kaynig, M. Longair, T. Pietzsch, S. Preibisch, C. Rueden, S. Saalfeld, B. Schmid, J.-Y. Tinevez, D. J. White, V. Hartenstein, K. Eliceiri, P. Tomancak and A. Cardona, Fiji: an open-source platform for biological-image analysis, Nat. Methods, 2012, 9, 676–682 CrossRef CAS PubMed.
- J. E. Jonkman, J. A. Cathcart, F. Xu, M. E. Bartolini, J. E. Amon, K. M. Stevens and P. Colarusso, An introduction to the wound healing assay using live-cell microscopy, Cell Adhes. Migr., 2014, 8, 440–451 CrossRef PubMed.
- J. M. Fernandez, D. Rosado-Alvarez, M. E. Da Silva Grigoletto, O. A. Rangel-Zuniga, L. L. Landaeta-Diaz, J. Caballero-Villarraso, J. Lopez-Miranda, F. Perez-Jimenez and F. Fuentes-Jimenez, Moderate-to-high-intensity training and a hypocaloric Mediterranean diet enhance endothelial progenitor cells and fitness in subjects with the metabolic syndrome, Clin. Sci., 2012, 123, 361–373 CrossRef CAS PubMed.
- N. A. Mikirova, J. A. Jackson, R. Hunninghake, J. Kenyon, K. W. H. Chan, C. A. Swindlehurst, B. Minev, A. N. Patel, M. P. Murphy, L. Smith, F. Ramos, T. E. Ichim and N. H. Riordan, Nutraceutical augmentation of circulating endothelial progenitor cells and hematopoietic stem cells in human subjects, J. Transl. Med., 2010, 8, 34–34 CrossRef PubMed.
- D. W. K. Toh, X. Xia, C. N. Sutanto, J. H. M. Low, K. K. Poh, J. W. Wang, R. S. Foo and J. E. Kim, Enhancing the cardiovascular protective effects of a healthy dietary pattern with wolfberry (Lycium barbarum): A randomized controlled trial, Am. J. Clin. Nutr., 2021, 114, 80–89 CrossRef PubMed.
- J. L. Rodgers, J. Jones, S. I. Bolleddu, S. Vanthenapalli, L. E. Rodgers, K. Shah, K. Karia and S. K. Panguluri, Cardiovascular Risks Associated with Gender and Aging, J. Cardiovasc. Dev. Dis., 2019, 6, 19 CrossRef CAS PubMed.
- Q. Luo, Y. Cai, J. Yan, M. Sun and H. Corke, Hypoglycemic and hypolipidemic effects and antioxidant activity of fruit extracts from Lycium barbarum, Life Sci., 2004, 76, 137–149 CrossRef CAS PubMed.
- L. Kou, M. Du, C. Zhang, Z. Dai, X. Li and B. Zhang, The Hypoglycemic, Hypolipidemic, and Anti-Diabetic Nephritic Activities of Zeaxanthin in Diet-Streptozotocin-Induced Diabetic Sprague Dawley Rats, Appl. Biochem. Biotechnol., 2017, 182, 944–955 CrossRef CAS PubMed.
- P. Faris, S. Negri, A. Perna, V. Rosti, G. Guerra and F. Moccia, Therapeutic Potential of Endothelial Colony-Forming Cells in Ischemic Disease: Strategies to Improve their Regenerative Efficacy, Int. J. Mol. Sci., 2020, 21, 7406 CrossRef CAS PubMed.
- C. Y. Wong, K. H. Yiu, S. W. Li, S. Lee, S. Tam, C. P. Lau and H. F. Tse, Fish-oil supplement has neutral effects on vascular and metabolic function but improves renal function in patients with Type 2 diabetes mellitus, Diabetic Med., 2010, 27, 54–60 CrossRef CAS PubMed.
- C. S. Park, W. Kim, J. S. Woo, S. J. Ha, W. Y. Kang, S. H. Hwang, Y. W. Park, Y. S. Kim, Y. K. Ahn, M. H. Jeong and W. Kim, Green tea consumption improves endothelial function but not circulating endothelial progenitor cells in patients with chronic renal failure, Int. J. Cardiol., 2010, 145, 261–262 CrossRef PubMed.
- A. J. Flammer, E. A. Martin, M. Gossl, R. J. Widmer, R. J. Lennon, J. A. Sexton, D. Loeffler, S. Khosla, L. O. Lerman and A. Lerman, Polyphenol-rich cranberry juice has a neutral effect on endothelial function but decreases the fraction of osteocalcin-expressing endothelial progenitor cells, Eur. J. Nutr., 2013, 52, 289–296 CrossRef CAS PubMed.
- I. Arnaoutova, J. George, H. K. Kleinman and G. Benton, The endothelial cell tube formation assay on basement membrane turns 20: state of the science and the art, Angiogenesis, 2009, 12, 267–274 CrossRef PubMed.
- A. D. van der Meer, K. Vermeul, A. A. Poot, J. Feijen and I. Vermes, A microfluidic wound-healing assay for quantifying endothelial cell migration, Am. J. Physiol.: Heart Circ. Physiol., 2010, 298, H719–H725 CrossRef CAS PubMed.
- P. H. Huang, Y. H. Chen, H. Y. Tsai, J. S. Chen, T. C. Wu, F. Y. Lin, M. Sata, J. W. Chen and S. J. Lin, Intake of red wine increases the number and functional capacity of circulating endothelial progenitor cells by enhancing nitric oxide bioavailability, Arterioscler., Thromb., Vasc. Biol., 2010, 30, 869–877 CrossRef CAS PubMed.
- S. Hamed, J. Alshiek, A. Aharon, B. Brenner and A. Roguin, Red wine consumption improves in vitro migration of endothelial progenitor cells in young, healthy individuals, Am. J. Clin. Nutr., 2010, 92, 161–169 CrossRef CAS PubMed.
- L. Giusti, M. Gabriele, G. Penno, M. Garofolo, V. Longo, S. Del Prato, D. Lucchesi and L. Pucci, A Fermented Whole Grain Prevents Lipopolysaccharides-Induced Dysfunction in Human Endothelial Progenitor Cells, Oxid. Med. Cell. Longevity, 2017, 2017, 1026268 Search PubMed.
- J. Turgeon, S. Dussault, F. Maingrette, J. Groleau, P. Haddad, G. Perez and A. Rivard, Fish oil-enriched diet protects against ischemia by improving angiogenesis, endothelial progenitor cell function and postnatal neovascularization, Atherosclerosis, 2013, 229, 295–303 CrossRef CAS PubMed.
- Y. Zhang, X. Wang, Y. Wang, Y. Liu and M. Xia, Supplementation of cyanidin-3-O-beta-glucoside promotes endothelial repair and prevents enhanced atherogenesis in diabetic apolipoprotein E-deficient mice, J. Nutr., 2013, 143, 1248–1253 CrossRef CAS PubMed.
- H. Huang, C. Y. Jin, X. K. Bi, Y. B. Zhao, S. J. Xu, M. H. Wang, L. Yu, Y. X. Sun and D. Hu, Green Tea Polyphenol Epigallocatechin-3-Gallate Promotes Reendothelialization in Carotid Artery of Diabetic Rabbits by Reactivating Akt/eNOS Pathway, Front. Pharmacol., 2018, 9, 1305 CrossRef CAS PubMed.
- P. H. Huang, H. Y. Tsai, C. H. Wang, Y. H. Chen, J. S. Chen, F. Y. Lin, C. P. Lin, T. C. Wu, M. Sata, J. W. Chen and S. J. Lin, Moderate intake of red wine improves ischemia-induced neovascularization in diabetic mice–roles of endothelial progenitor cells and nitric oxide, Atherosclerosis, 2010, 212, 426–435 CrossRef CAS PubMed.
- J. Gui, A. Rohrbach, K. Borns, P. Hillemanns, L. Feng, C. A. Hubel and F. von Versen-Hoynck, Vitamin D rescues dysfunction of fetal endothelial colony forming cells from individuals with gestational diabetes, Placenta, 2015, 36, 410–418 CrossRef CAS PubMed.
- S. Tagawa, C. Nakanishi, M. Mori, T. Yoshimuta, S. Yoshida, M. Shimojima, J. Yokawa, M. A. Kawashiri, M. Yamagishi and K. Hayashi, Determination of Early and Late Endothelial Progenitor Cells in Peripheral Circulation and Their Clinical Association with Coronary Artery Disease, Int. J. Vasc. Med., 2015, 2015, 674213 Search PubMed.
- R. G. Akwii, M. S. Sajib, F. T. Zahra and C. M. Mikelis, Role of Angiopoietin-2 in Vascular Physiology and Pathophysiology, Cells, 2019, 8, 471 CrossRef CAS PubMed.
- S. Chen, L. Guo, B. Chen, L. Sun and M. Cui, Association of serum angiopoietin-1, angiopoietin-2 and angiopoietin-2 to angiopoietin-1 ratio with heart failure in patients with acute myocardial infarction, Exp. Ther. Med., 2013, 5, 937–941 CrossRef PubMed.
- J. Muller-Ehmsen, D. Braun, T. Schneider, R. Pfister, N. Worm, K. Wielckens, C. Scheid, P. Frommolt and M. Flesch, Decreased number of circulating progenitor cells in obesity: beneficial effects of weight reduction, Eur. Heart J., 2008, 29, 1560–1568 CrossRef PubMed.
- T. Eizawa, U. Ikeda, Y. Murakami, K. Matsui, T. Yoshioka, M. Takahashi, K. Muroi and K. Shimada, Decrease in circulating endothelial progenitor cells in patients with stable coronary artery disease, Heart, 2004, 90, 685–686 CrossRef CAS PubMed.
- N. Werner, S. Kosiol, T. Schiegl and E. Al, Circulating endothelial progenitor cells and cardiovascular outcomes, ACC Curr. J. Rev., 2005, 14, 3–3 CrossRef.
- J. George, E. Goldstein, S. Abashidze, V. Deutsch, H. Shmilovich, A. Finkelstein, I. Herz, H. Miller and G. Keren, Circulating endothelial progenitor cells in patients with unstable angina: association with systemic inflammation, J. Am. Coll. Cardiol., 2004, 43, A489–A489 CrossRef.
- M. Massa, V. Rosti, M. Ferrario, R. Campanelli and L. Tavazzi, Increased circulating hematopoietic and endothelial progenitor cells in the early phase of acute myocardial infarction, Blood, 2005, 105, 199–206 CrossRef CAS PubMed.
Footnote |
† Electronic supplementary information (ESI) available. See DOI: 10.1039/d1fo02369a |
|
This journal is © The Royal Society of Chemistry 2022 |
Click here to see how this site uses Cookies. View our privacy policy here.