DOI:
10.1039/D2FO00560C
(Review Article)
Food Funct., 2022,
13, 7473-7486
Beneficial effects of psyllium on the prevention and treatment of cardiometabolic diseases
Received
16th March 2022
, Accepted 11th June 2022
First published on 13th June 2022
Abstract
Cardiometabolic diseases are reaching epidemic proportions worldwide. Dietary fiber intake can improve the risk factors associated with CMD. Psyllium, especially its husk, is one of the most widely used dietary fiber supplements, which is often used to enrich cereals and other food products. Numerous pharmacological studies have investigated the active ingredients and therapeutic effects of psyllium and its extracts, including antioxidant, anti-tumor, antidiabetic, hypotensive, anti-inflammation, neuroprotection, antidiarrheal, and antiviral activities. In this review, we will summarize the available studies on the therapeutic potential and possible mechanisms of psyllium in treating CMDs, such as hyperlipidemia, diabetes mellitus, and its complications, hypertension, hyperuricemia and obesity, and its applications in food systems.
Introduction
Cardiometabolic diseases (CMDs) are reaching epidemic proportions worldwide and are the leading cause of death in both developed and developing countries.1 In addition to genetic factors, dyslipidemia, poor glycemic control, abdominal obesity, smoking, lack of exercise, and dietary factors are associated with CMD.2 Dyslipidemia is one of the major risk factors contributing to cardiovascular diseases (CVD) through atherosclerosis development and progression.3 Sustained exposure to high glucose levels significantly increases the risk of CVD complications.4 Obesity, particularly severe obesity, is capable of producing hemodynamic alterations that result in alterations in cardiac structure and function which may contribute to the development of CVD.5 Individuals with CMDs face a higher risk of developing myocardial infarction, stroke, and heart failure. Current evidence indicates that interventions to control modifiable risk factors can reduce the occurrence of CVD or slow down its progression. A growing amount of evidence suggests that dietary fiber intake can improve the risk factors associated with CMD,6 and major organizations support increasing fiber intake as part of a healthy lifestyle promotion. Among the most popular, supplemental, soluble dietary fibers globally are the seed husks of psyllium,7 which is often used to enrich cereals and other food products.
Psyllium, also known as isabgol, is the seed of an annual plant of the Plantago genus, which is widely cultivated in tropical regions of the world, such as India, Iran, Egypt, China, etc.8 In China, psyllium is commonly called “Che Qian Zi”, and is used as folk medicine with a long history for antipyretic, diuretic, and expectorant purposes. It was recommended for “disorders of vital energy, pain, diuresis and rheumatism” in the Shen Nong Ben Cao Jing (Herbal of the Divine Plowman).9 Furthermore, psyllium is also commonly used in traditional Indian medicine for the treatment of skin irritations, constipation, and diarrhea.10 Recently, psyllium, especially its husk, has been widely used both as a pharmacological supplement and a food product.11 Pharmacological studies have shown that psyllium exhibits several biopharmacological activities, including against constipation,12–15 diarrhea,15,16 obesity,17,18 diabetes,19–23 nonalcoholic fatty liver disease (NAFLD),24 hypercholesterolemia,20,25–30 irritable bowel syndrome,31,32 and colon cancer,33 some of which are closely related to the development of CMDs. Psyllium husk can be used in food product development, such as in ice cream, bakery goods, dairy and gluten-free products, beverages, chocolates, noodles, and breakfast cereals.11,34–41 Most health-beneficial effects and food applications of psyllium are attributed to its high water-holding capacity and gel-forming nature.42–45 The purpose of this review is to summarize the available studies on the beneficial health effects of psyllium on CMDs and its applications in functional foods.
Chemical constituents
Psyllium is the common name related to the seeds of an annual herb of the Plantago genus.46 The genus Plantago is one of the largest of the three genera of the Plantaginaceae family, comprising about 270 species, such as Plantago ovata Forssk., Plantago asiatica L., Plantago major L., and Plantago depressa Willd., among others.46
The genus Plantago is widely distributed in temperate and high-elevation tropical regions.47 The seed and the whole plant are shown in Fig. 1. Once harvested, the husk is mechanically separated from the seed by milling.8 The husk is the white membranous structure covering the seed, and it constitutes about 30% of the dried seed's weight.
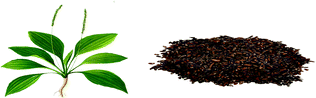 |
| Fig. 1 The Plantago genus plant (left), and traditional Chinese herb Che Qian Zi/psyllium (right). | |
The whole psyllium consists of soluble (mostly arabinoxylans) and insoluble polysaccharides (hemicellulose, cellulose, and lignin), as well as tannins, phenols, and flavonoids48–51 (Fig. 2). The husk of psyllium is a mixture of neutral and acid polysaccharides containing galacturonic acid with a ratio of soluble/insoluble fiber of 70/30.52 Most of the health-beneficial effects and food applications of psyllium and its husk are attributed to their high content of arabinoxylans (polysaccharides mainly composed of xylose and arabinose), with the husk containing more.53,53 Polysaccharide extracted from psyllium seeds is a very important biological active ingredient that has been widely reported in recent years.9,50,54,55 Psyllium polysaccharides are composed of xylose, arabinose, galactose, rhamnose, glucose, and mannose in different molar ratios. According to these reports, psyllium polysaccharide possesses various human health-promoting effects, such as antioxidant, antitumor, immunomodulatory, anti-proliferative,56 hypoglycemic, hypotensive, and antiviral activities,57 among others.55 It is worth mentioning that the raw materials, extraction temperature, and time will affect the composition, structure, and function of psyllium polysaccharides.55 Moreover, both psyllium seeds and husks are rich in bioactive compounds and different primary and secondary metabolites.51,58 Among these, the most abundant compounds are fatty acids, amino acids, polyphenols, and flavonoids.58,59 Metabolites such as polyphenols and flavonoids that possess antioxidant activity thus have the potential for the treatment of excessive reactive oxygen species (ROS)-triggered diseases.
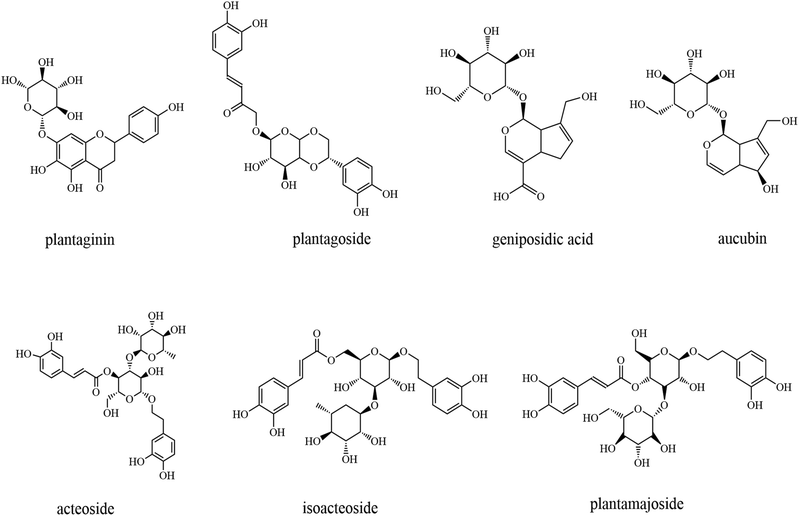 |
| Fig. 2 Chemical structures of the representative components of psyllium. | |
Psyllium applications in food systems
The growing awareness of a healthy diet has led to an increasing demand for functional food, which has induced the food industry to search for new functional products with health benefits.60 Meanwhile, the sensory properties, such as color, flavor, texture, and overall acceptability, will affect consumers’ acceptance.61 According to previous studies, some researchers have added psyllium husk into food products (such as ice cream, biscuits, gluten-free bread, noodles, pizza, pasta, and cakes), and reported health benefits such as cholesterol control, glycemic control, and satiety, among others (Fig. 3).11,41,61 In addition, the addition of psyllium has been shown to obtain a softer crumb in high-fiber wheat bread and improve dough mechanical properties and gluten-free bread shelf-life.35,37,38,40,62 However, the incorporation of psyllium husk in different foods is limited, as high levels of fiber may cause disagreeable changes in the texture and color of foods.46 Particularly in baked products, it was observed that cookies with psyllium husk were darker than those without it.61,63 Thus, further research may focus on minimizing the undesired effects of a great incorporation of psyllium husk in food to improve its quality and consumer acceptability.
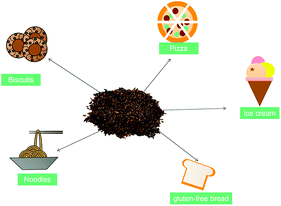 |
| Fig. 3 Psyllium applications in food systems. | |
Adverse effects
Psyllium is safe to use as a functional ingredient in food systems.11 A clinical trial64 involving 93 healthy subjects showed that psyllium husk (10.5 g, 52 weeks) was well tolerated and the majority of adverse events recorded were minor, of short duration, and either unrelated or only possibly related to the study treatment. Similarly, a clinical trial involving 12 type 2 diabetic patients showed that psyllium administration did not adversely affect either mineral or vitamin A and E concentrations.65 Despite this, several studies have reported possible adverse effects of psyllium intake, such as altering nutrient absorption and eliciting allergic reactions. One study reported that psyllium intake reduced calcium bioavailability and induced negative changes in bone composition in weanling Wistar rats, although there was virtually no binding of exogenous calcium by psyllium in vitro.66,67 Lantner briefly reviewed and discussed the allergic reactions to psyllium and suggested physicians and consumers should be aware of potentially serious reactions to it.68 Moreover, based on in vitro studies, psyllium has once been characterized as “fermentable”, which could cause symptoms related to excessive gas production. However, McRorie et al. reviewed six clinical studies and emphasized that gel-forming psyllium is not fermented in the human gastrointestinal tract and does not produce excess gas.69–71 Moreover, some researchers are concerned about whether long-term exposure to psyllium may adversely affect health or increase the risk of colon tumorigenesis.72 Psyllium has been shown to reduce colon cancer risk, both in humans and in animals.73,74 Citronberg et al. used a large clinical study (75
214 participants) which examined the association between colorectal cancer incidence and constipation, non-fiber laxative use, and fiber laxative use (e.g., psyllium).75 The authors concluded that the risk for colorectal cancer increases with non-fiber laxative use and decreases with fiber laxative use (e.g., psyllium). McRorie et al. reported that psyllium husk should be taken at higher doses with sufficient water to maximize its efficacy response.76 In summary, psyllium can be safely used in the run for numerous health benefits.
Therapeutic potential psyllium in CMDs
Hyperlipidemia
Hyperlipidemia, referring to a metabolism disorder, is characterized by increased total cholesterol, triglyceride, and low-density lipoprotein cholesterol (LDL-C), and decreased high-density lipoprotein cholesterol (HDL-C) in serum. Epidemiologic data suggest that dyslipidemia is the major causal factor for ischaemic heart disease and stroke worldwide, accounting for 84.9% of CVD deaths.3,77 Research has shown that dyslipidemia leads to the deposition of cholesterol and lipids in blood vessel walls, chronic inflammation, and the formation of atherosclerotic plaques that block blood flow and (when they rupture) lead to acute ischemic attacks.78 Measures to adjust abnormal lipid metabolism are commonly agreed to prevent the outbreak of CVD. However, many lipid-lowering drugs, such as statins, carry various side effects or contraindications.79
Plenty of randomized clinical studies and meta-analyses have evaluated the effect of psyllium on lipid levels (Table 1). McRorie et al.69 analyzed 21 randomized, well-controlled clinical studies (>1500 subjects) using doses of 6 to 15 g d−1 (most studies at 10 g d−1) and assessed the effectiveness of psyllium for lowering elevated serum cholesterol concentrations. In all studies, there were significant cholesterol-lowering effects of psyllium, ranging from a −6% to −24% change for LDL cholesterol and −2% to −20% for total cholesterol, versus placebo. The authors concluded that the cholesterol-lowering efficacy of psyllium was greatest in studies with unrestricted diets and patients with high baseline cholesterol concentrations.80 A meta-analysis of 28 studies (n = 1924) found that supplementation with a median dose of 10.2 g d−1 psyllium for 8 weeks significantly lowered LDL cholesterol by −0.33 mmol L−1, non-HDL cholesterol by −0.39 mmol L−1, and apolipoprotein B by −0.05 g L−1. The authors concluded that psyllium fiber potentially delayed atherosclerosis-associated CVD risk in individuals with or without hypercholesterolemia.27 According to the FDA, the consumption of 7 g or more of psyllium per day, associated with a diet with low amounts of saturated fat and cholesterol, can reduce the risk of CVD by lowering serum cholesterol levels.81 In addition, Wei et al.30 analyzed 21 studies (n = 1030) using doses of 3.0 to 20.4 g d−1 and reported a dose–response relationship between psyllium and serum cholesterol level and a time-dependent effect of psyllium in mildly to moderately hypercholesterolemic subjects. The cholesterol-lowering benefit of psyllium has also been shown to be an effective co-therapy for statin drugs. Moreover, a recent meta-analysis reported that the addition of psyllium (mean, 10.8 g d−1 in divided doses before meals) would add to the efficacy of statins and result in reductions in LDL cholesterol equivalent to doubling the statin dose.25 The authors concluded that combining statin therapy with psyllium supplementation may provide an option for patients who cannot tolerate the higher-dose statins. Furthermore, an economic analysis82 found that if adults aged 45 years and older with elevated LDL-cholesterol levels (e.g., ≥130 mg dL−1) consumed psyllium 10.2 g d−1, there could be an average saving of $870 million in healthcare costs and quality of life productivity gains (estimates from 2013–2020). Psyllium has a notable role in lowering elevated serum cholesterol concentrations, which is mainly due to its viscosity. Cholesterol is one of the main components of bile, and therefore, an increase in bile salt excretion causes increased cholesterol use for hepatic bile synthesis.83 Psyllium is rich in soluble fiber, which forms a viscous gel that binds to bile salts in the gut and increases their excretion with stools, leading to the reduction of the bile acid pool. This reduction causes hepatocytes to compensate by stimulating LDL receptor expression,84 which promotes the conversion of cholesterol to bile acids. When the conversion is increased in the body, it promotes the reduction of blood cholesterol concentrations, contributing to a reduction of the risk of CVD. Note that the cholesterol-lowering efficacy of psyllium is a viscosity-dependent phenomenon: the higher the fiber viscosity, the greater its cholesterol-lowering effect.85
Table 1 Table of meta-analyses investigating the hypolipidemic, hypoglycemic, antihypertensive, and anti-obesity effects of psyllium
Outcomes |
Evidence type and population |
Dose |
Duration |
Findings |
Ref. |
Hypolipidemic effect |
Meta-analysis (21 studies, n = 1030) |
3.0–20.4 g d−1 |
2 weeks |
↓Total cholesterol (−0.375 mmol L−1) |
30
|
↓LDL cholesterol (−0.278 mmol L−1) |
Hypolipidemic effect |
Meta-analysis (28 studies, n = 1924) |
10.2 g d−1 |
8 weeks |
↓LDL-cholesterol (−0.33 mmol L−1) |
27
|
↓Non-HDL cholesterol (−0.39 mmol L−1) |
↓apoB (−0.05 g L−1) |
Psyllium could produce dose- and time-dependent serum cholesterol-lowering effects in mildly and moderately hypercholesterolemic patients |
Hypolipidemic effect |
Meta-analysis (3 studies, n = 204) |
10.8 g d−1 |
4–12 weeks |
Adding psyllium fiber resulted in reductions in low-density lipoprotein-cholesterol equivalent to doubling the statin dose |
25
|
Hypoglycemic effect |
Meta-analysis (35 studies, n = 535) |
10 g d−1 |
1 meal to 6 months |
↓Fasting blood glucose (−37.0 mg dL−1) |
90
|
↓Glycated hemoglobin (−0.97%) |
The glycemic control effects of psyllium were proportional to baseline FBG |
Antihypertensive effect |
Meta-analysis (11 studies, n = 592) |
3.7–15 g d−1 |
4 weeks to 6 months |
↓SBP (−2.04 mmHg) |
7
|
Psyllium supplementation revealed significant reductions in blood pressure in subjects with higher baseline blood pressure |
Anti-obesity effect |
Meta-analysis (8 studies, n = 395) |
9 g d−1 (3.4 to 15 g d−1) |
8–20 weeks |
↓Triglycerides (−19.18 mg dl−1) |
131
|
↓LDL (−8.96 mg dl−1) |
↓Fasting blood sugar (−31.71 ml dl−1) |
↓Hemoglobin A1c (−0.91%) |
No significant change in high-density lipoprotein, body mass index, cholesterol, and weight |
Anti-obesity effect |
Meta-analysis (22 studies, n = 1458) |
1.7–15 g d−1 |
2–48 weeks |
Psyllium supplementation does not reduce body weight (−0.28 kg), body mass index (−0.19 kg m−2), and waist circumference (−1.2 cm) significantly |
132
|
In summary, psyllium possesses a favorable effect on lowering cholesterol levels, which is highly related to the ability to form a viscous gel to augment bile acid-binding and its fecal excretion, promoting the conversion of cholesterol to bile acids, and thus leading to a reduction of blood cholesterol concentrations. Furthermore, psyllium as adjuvant anti-lipid therapy could help achieve the target lipid plasma levels with lower drug dosages and fewer adverse effects.
Diabetes mellitus and its complications
Diabetes mellitus (DM) is a metabolic disease characterized by chronic hyperglycemia due to deficient insulin production (type 1 diabetes mellitus) or peripheral insulin resistance of the cells (type 2 diabetes mellitus).86 Both of them could lead to a variety of cardiovascular complications. Sustained exposure to high glucose levels is associated with the development of macrovascular complications, such as coronary artery disease, peripheral vascular disease, cerebrovascular disease, and microvascular complications, including retinopathy, nephropathy, and neuropathy.86
Currently, numerous studies suggest that psyllium can improve glycemic indicators, such as fasting blood glucose (FBG), glycated hemoglobin (HbA1c), and insulin resistance (HOMA-IR), and exert protective actions against these cardiovascular complications of diabetes, suggesting that psyllium administration is a promising therapy for diabetes (Table 1).19,20,65,87–89 A meta-analysis of 35 randomized, controlled, clinical studies showed that psyllium (mean, 10 g d−1 in divided doses before meals) showed a significant decrease in both FBG level and HbA1c.90 Similar to cholesterol-lowering, the glycemic effects of viscous/gel-forming fiber are also proportional to baseline FBG. There was no significant effect on FBG in euglycemic subjects, a modest effect in subjects with pre-T2DM (up to −4.7 mg dL−1), and the greatest effect in subjects who were being treated for T2DM (−37.0 mg dL−1).
McRorie et al. reported that the primary mechanism for improving glycemic control with a soluble, viscous fiber supplement is by significantly increasing the viscosity of chyme in a dose-dependent manner.69 Psyllium forms a viscous gel that increases chyme viscosity, which leads to a decrease in the interactions between the digestive enzymes and nutrients, slowing down the degradation and absorption of glucose and other nutrients. Nutrients are normally absorbed early in the small bowel, but this delay leads to an increased delivery of nutrients to the distal ileum. With more nutrients delivered to the distal ileum, mucosal L-cells are stimulated to release more glucagon-like peptide-1 (GLP-1) into the bloodstream,91 which plays a central role in the coordination of postprandial glucose homeostasis.
Sustained exposure to high glucose levels contributes to oxidative stress. Oxidative stress is thought to be a major factor contributing to the pathogenesis and progression of diabetes-induced CVD.92 Recent experiments showed that psyllium could enhance the antioxidative defense system as illustrated by the increase in superoxide dismutase (SOD), catalase (CAT), and glutathione peroxidase (GSH-Px) levels and decreased endogenous ROS production, and malondialdehyde (MDA) content, thereby protecting the body from oxidative stress.93,94 Plantamajoside, a major compound of psyllium, effectively elevated the activities of SOD, CAT, and GSH-Px to inhibit hypoxia/reoxygenation-induced ROS production, and significantly enhanced the activation of the Akt/Nrf2/HO-1 signaling pathway.95 Studies have demonstrated that nuclear factor erythroid 2-related factor 2 (Nrf2)96 can upregulate the transcription of antioxidant enzymes involved in cellular antioxidant defenses, which protects cells from oxidative stress damage. One study indicated that psyllium can enhance the expression of Nrf2, thereby increasing the levels of antioxidant enzymes such as glutathione S-transferase mu 2 (GSTM2) and superoxide dismutase 2 (SOD2), and enhancing the expression of SIRT5, SIRT6, and SIRT7, which contributes to protecting against myocardial ischemia/reperfusion injury through the elimination of ROS.97
Diabetic retinopathy is envisioned as a biomarker of generalized hyperglycemic damage in the microvasculature.98 The mechanisms of hyperglycemia leading to diabetic retinopathy mainly include increased free radical production, AGEs, inflammatory factors, and vascular endothelial growth factor.99 Administration of psyllium (100, 200, or 300 mg kg−1 for 8 weeks) alleviated the blood glucose level, the diameter of the retinal vessels, and retinal vascular permeability in streptozotocin-induced diabetic rats.93 Moreover, it increased the activities of SOD and CAT, and GSH levels, and down-regulated the expression of TNF-α, interleukin-1beta (IL-1β), intercellular adhesion molecule 1 (ICAM-1), and vascular cell adhesion molecule-1 (VCAM-1) in diabetic retinae, which demonstrated that psyllium may be a potential therapeutic agent for the prevention of retinal microvascular complications of diabetes. In addition, plantagoside and its aglycone, isolated from Plantago major seeds, inhibited the formation of advanced glycation end products, which suggested that psyllium has potential therapeutic effects in the prevention of diabetic complications.100
According to these studies, psyllium, through its viscous property, forms gel-like substances in our gastrointestinal tract, which can lead to a decrease in the interactions between the digestive enzymes and nutrients, slowing down the degradation and absorption of glucose and other nutrients, thereby helping to manage blood sugar. Meanwhile, psyllium has great potential therapeutic effects in the prevention and treatment of diabetic complications for the antioxidant activity.
Hypertension
Hypertension is a multifactorial cardiovascular disorder that has a variety of genetic and environmental factors. Globally, the prevalence of hypertension is expected to reach approximately 1.6 billion by 2025.101 Long-term elevated or unstable blood pressure can lead to cardiac hypertrophy, and irreversible changes in the left ventricular wall, making it harder, thinner, and weaker, resulting in a larger left ventricular volume, and ultimately leading to cardiac insufficiency.102
A clinical trial showed that the psyllium group (10.5 g d−1, 6 months) showed a significant improvement in plasma TG concentration, SBP, and diastolic blood pressure (DBP), suggesting that psyllium was effective in lowering blood pressure.103 Another clinical trial yielded similar results; subjects with hyperlipidemia were instructed to take psyllium (10.5 g d−1, 3 months), and it was observed that their weight, SBP, and DBP reduced by 1.34%, 2.57%, and 4.57% respectively.104 A recent meta-analysis of 11 trials (n = 592) showed that supplementation with psyllium revealed a reduction of 2.04 mmHg in SBP, and its anti-hypertensive effect was stronger in subjects with a higher baseline blood pressure (Table 1).7 Similarly, another meta-analysis found that of the five viscous fiber supplementations, only psyllium revealed a reduction of 2.39 mmHg in SBP.105 Furthermore, as excess weight is associated with an increased risk of inhibited blood pressure control, Khan et al.105 suggested that the reduction of blood pressure is presumably secondary to weight loss.
The renin–angiotensin–aldosterone system (RAAS) is a major mechanism for blood pressure regulation. Previous animal studies reported that psyllium has an anti-hypertensive effect through the inhibition of angiotensin-converting enzyme (ACE) activity. In spontaneous phase-1 hypertension rats, psyllium extract (0.36 g kg−1) could play acute antihypertensive effects, with systolic pressure decreased by 31.5 mmHg and diastolic pressure decreased by 18.5 mmHg within an hour.106 In spontaneously hypertensive rats, psyllium extract (400 mg kg−1) reduced systolic, diastolic, and mean blood pressures, and the levels of serum angiotensin I (Ang I) and angiotensin II (Ang II), the ratio of Ang II to Ang I, and aldosterone (ALD) were lowered.107 Meanwhile, in vitro, it was observed that psyllium extracts and their major constituents have ACE inhibitory activity.108
In addition, previous studies found that psyllium was applied to treat hypertension clinically due to its traditional diuretic effect. High salt intake increases the burden on the kidneys, which has long been associated with high blood pressure. In the salt-accelerated hypertensive rat, psyllium increased fecal excretion of sodium and attenuated hypertension.109 Aquaporin 2 (AQP2) is associated with water reabsorption in the renal collecting ducts, which is the key process of body water homeostasis. In another saline-loaded rat, crude and salt-processed psyllium increased urine volume excretion and urine electrolyte excretion and decreased ALD and AQP2 levels.110
Endothelial dysfunction may contribute to increased systemic vascular resistance, thus leading to the development of hypertension. In obese Zucker rats, psyllium treatment improved endothelial dysfunction and hypertension.111 In human umbilical vein endothelial cells, it was observed that plantamajoside from psyllium treatment could improve advanced glycation end product-induced endothelial cell dysfunction.112 Oxidative stress and inflammation can cause endothelial cell dysfunction. In phase-1 hypertension rats, psyllium extract could play anti-inflammatory and antioxidant effects by inhibiting the activity of NF-κB, TNF-α, cyclooxygenase-1 (COX-1), COX-2, and NO production.58,93,94,113,114 Fu et al. suggested that the sustained administration of psyllium extract may improve chronic high blood pressure.106
Additionally, long-term hypertension usually causes cardiac hypertrophy. In isoproterenol-induced cardiac hypertrophy mice, psyllium extract treatment (20, 40, and 80 mg kg−1 day−1) improved cardiac function indexes, including ejection fraction, fractional shortening, stroke volume, and cardiac output, decreased the heart weight/body weight ratio and cardiomyocyte size, and downregulated the mRNA and protein expressions of the hypertrophic markers ANP, BNP, and β-MHC.115 In addition, isoproterenol-induced the alteration in autophagic markers, such as the increase of Beclin1 and LC3II, and decrease of p62, which were rescued by the treatment of psyllium extract. In terms of the proteins involved in the apoptosis, isoproterenol-induced the decrease of Bcl-2 but the increase of Bax and Cleaved-caspase3, which was reversed by psyllium extract treatment. These results implied that psyllium extract treatment might prevent cardiomyocyte injury through anti-autophagy and anti-apoptosis. Uniformly, plantamajoside, an active component that can be extracted from psyllium, inhibited the mRNA and protein expression of ANP, BNP, Myh7, COL1, and COL3, as well as suppressing the activity of histone deacetylase 2 and its downstream proteins, protein kinase B and glycogen synthase kinase-3beta, both in vitro and in vivo.116 These results suggested that psyllium may play a therapeutic role in CVDs caused by long-term hypertension.
In short, the antihypertensive effect of psyllium can be directly attributed to the regulation of the RAAS, the diuretic effects, and the improvement of endothelial function. Indirectly, psyllium can improve the control of body weight, exerting a hypotensive effect. Moreover, psyllium can also improve cardiac hypertrophy caused by long-term hypertension.
Hyperuricemia
Uric acid (UA) is the final product of purine metabolism, which is a well-known risk factor for gout.117 Recent epidemiological studies show that high levels of serum UA are important biomarkers for CVDs morbidity and mortality. Moreover, asymptomatic hyperuricemia has been reported to lead to a significantly increased risk for cardiometabolic conditions.118 Despite extensive research, the underlying molecular mechanisms remain unclear. Experimental studies show that hyperuricemia mainly promotes the occurrence and development of CVDs by regulating molecular signals, such as inflammatory responses, insulin resistance/diabetes, oxidative stress, endoplasmic reticulum stress, and endothelial dysfunction.119,120 It was reported that the mean level of uric acid was 10% lower after psyllium administration.65
Currently, several studies have shown that psyllium exerts a UA-lowering effect through two mechanisms: inhibiting UA synthesis (xanthine oxidase (XOD) inhibitors) and increasing UA excretion. Firstly, xanthine oxidase is a key enzyme that catalyzes xanthine to uric acid. Zeng et al. have demonstrated the XOD inhibitory effects of the psyllium extract acteoside, and 1H-indolo-3-carbaldehyde, by the electron transfer signal blocking activities (ETSBA) method, suggesting that psyllium was effective in inhibiting XOD activities to reduce hyperuricemia.121 Another in vitro research study has yielded similar results. Secondly, urate anion transporter 1 (URAT1) is the main transporter for UA reabsorption.122 URAT1 inhibition can reduce the reabsorption of UA in the renal tubules, accelerate the renal excretion of UA, reduce blood uric acid, and ultimately play a therapeutic role in hyperuricemia. In the potassium oxonate-induced hyperuricemia rat, psyllium treatment may achieve the effect of treating hyperuricemia by downregulating the mRNA expression of URAT1.123 Additionally, a high dose of psyllium possesses inhibitory activity over the phosphatidylinositol 3-kinase/protein kinase B (PI3K/Akt) pathway, which plays a role in anti-inflammatory and renal protection, and ultimately indirectly improves hyperuricemia.
These studies demonstrated that psyllium is a potential therapeutic agent for the treatment of hyperuricemia via inhibiting XOD activities and URAT1 expression.123
Obesity
Obesity, a worldwide epidemic, originates from an imbalance between food intake and energy expenditure, which leads to an excessive accumulation of adipose tissue, and is often associated with chronic inflammation.124 One epidemiological study estimated that the global obesity prevalence will reach 18% in men and surpass 21% in women by 2025.125 Recent clinical and epidemiological evidence has shown a strong association between obesity and CVDs including coronary heart disease, atrial fibrillation, ventricular arrhythmias, cerebrovascular disease, heart failure, and sudden cardiac death.126 Data from both humans and animals have suggested that psyllium can significantly suppress appetite and energy intake that can contribute to weight loss, body mass index (BMI), and waist circumference (WC).
As previously mentioned, the rate of digestion and absorption is delayed because of the formation of the psyllium gel within the small intestine, which prolongs satiety/delays hunger, thus leading to decreased energy intake and weight loss. Furthermore, as a kind of fiber-rich food, the act of chewing it needs a longer time, which may increase sensory satiety and diminish meal size. One publication comprises two clinical trials that investigated the satiety effects of psyllium in healthy adults.127 The first study determined the effects of 3 different doses of psyllium (3.4 g, 6.8 g, and 10.2 g; n = 30) before breakfast, lunch, and dinner for 3 consecutive days. The authors reported that all 3 psyllium doses resulted in directional or statistically significant mean reductions in hunger and desire to eat, and increased fullness between meals compared with placebo, with both higher doses better than placebo or 3.4 g. As the 6.8 g dose provided more consistent satiety benefits versus placebo in the first study, the second study selected the 6.8 g dose (taken before breakfast and lunch on days 1 and 2 and before breakfast on day 3) to verify whether the satiety effect of psyllium following the consumption of a less satiating breakfast in a similar population of healthy individuals (n = 44) was reproducible. The results showed a significant decrease in the 3-day mean hunger and desire to eat, as well as an increase in fullness for psyllium compared with placebo. Other studies have also confirmed the efficacy of psyllium for increasing satiety/decreasing hunger.128–130 A study assessed the efficacy of psyllium on gastric emptying, hunger feeling, and food intake in 14 normal volunteers. Results showed that psyllium (7.4 g d−1) reduced hunger feelings and energy intake.128 Meanwhile, the postprandial increase in serum glucose, triglyceride and insulin levels was less with psyllium than with placebo. Overweight and obesity are two important risk factors for NAFLD, and a clinical study reported that calorie intake, carbohydrate intake, liver enzyme alanine aminotransferase, waist circumference, BMI, weight and body fat percentage were reduced in the psyllium supplement group (10 g d−1, n = 80) as compared with the placebo group. The authors concluded that the weight loss diet program which was supplemented with psyllium in parallel with increasing physical activity can be useful in reducing NAFLD outcomes.24
The effectiveness of psyllium in weight loss was also reported in some clinical studies. A 12-week study in 51 patients with type 2 diabetes and chronic constipation compared the effects of psyllium (10 g d−1, divided doses before meals) on weight.22 The authors reported that psyllium significantly reduced body weight by 2.0 kg versus placebo. Two recent meta-analyses reviewed the effects of psyllium consumption on weight loss (Table 1). Xiao et al.131 analyzed 8 studies containing nine arms with 395 participants and found a relationship between the consumption of psyllium and weight, body mass index, lipid profile, and glucose metabolism in diabetic patients. The results showed no significant change in HDL, body mass index, cholesterol, and weight. Another meta-analysis included 23 randomized clinical trials and concluded that psyllium did not reduce body weight, BMI, and WC significantly.132 Due to the fact that the dosages, forms, and intervention periods of psyllium, as well as the physiological statuses of the participants, were varied among those studies, this cannot negate the beneficial effects of psyllium in obesity or overweight patients.
In addition, it has been reported that CVD is the collateral damage of obesity-driven adipose tissue dysfunction that promotes a chronic inflammatory state within the organism. This chronic inflammatory state could trigger or even worsen CVD by upregulating pro-inflammatory adipokines and downregulating anti-inflammatory adipokines.133 A recent piece of clinical evidence has demonstrated that psyllium is effective in reducing IL-6 in obese adolescents.17 Despite this, there was a study that demonstrated that psyllium supplements had no ameliorating effect on CRP levels or other inflammatory markers in overweight or obese individuals.134 It was the length of the study that may affect the psyllium supplementation intervention. Moreover, the activation of nuclear factor-kappa B (NF-kB) plays a central role in inflammation, which positively regulates the gene transcription of many pro-inflammatory cytokines, inflammatory cytokines, and adhesion molecules such as interleukin-6 (IL-6), TNF-α, monocyte chemoattractant protein-1 (MCP-1), ICAM-1, VCAM-1, inducible nitric oxide synthase (iNOS), and matrix metalloproteinase-9 (MMP-9). What is more, the intake of psyllium husk supplements not only decreased the TNF-α concentration but also prevented endothelial dysfunction in obese rats.111 In advanced glycation end products-induced human umbilical vein endothelial cells, plantamajoside has been shown to suppress inflammatory cytokines and adhesion molecule expression, due to inhibition of the MAPK/NF-κB pathways.112 Another in vitro study on RAW 264.7 mouse macrophage cells showed that the extracts of psyllium suppressed the expression of inflammatory cytokines, such as IL-1β, IL-6, and cyclooxygenase-2 (COX-2).135
In a word, psyllium can suppress appetite and energy intake, and reduce body weight. Furthermore, psyllium can also upregulate pro-inflammatory adipokines and downregulate anti-inflammatory adipokines to improve the obesity-driven chronic inflammatory state of CVDs.
Conclusions
Due to its high medicinal and economic values, psyllium, especially its husk, has attracted significant attention from researchers. Psyllium husks encompass rich dietary fiber that is highly viscous, highly soluble in water, and higher in gel-forming capacity with a long history of multiple health beneficial effects, preventing or withstanding a series of common diseases, including hyperlipemia, diabetes, obesity, CVD, constipation, irritable bowel syndrome symptoms, diarrhea, and cancer, some of which are closely related to the development of CMDs.
Observational and experimental studies have indicated that most of the health-beneficial effects and applications of psyllium are mainly due to its viscosity (Fig. 4): psyllium forms a viscous gel in the water, which binds to bile acids in the gut and eliminates bile acids via the stools, and promotes the conversion of cholesterol to bile acids, thus leading to a reduction of blood cholesterol concentrations; the gel increases chyme viscosity, which can lead to a decrease in the interactions between the digestive enzymes and nutrients within the gastrointestinal system, slowing down the degradation and absorption of glucose and other nutrients, thereby helping to manage blood sugar; the delay in nutrient digestion and absorption prolongs satiety/delays hunger, leading to decreased energy intake and weight loss in overweight/obese patients. Notably, the high water-holding capacity and gel-forming nature of psyllium fiber are viscosity-dependent phenomena. Moreover, previous studies have revealed that psyllium and its extracts can directly or indirectly exert a hypotensive effect, and improve the cardiac hypertrophy caused by long-term hypertension. In addition, hyperuricemia has become the fourth major risk factor after hypertension, hyperlipidemia, and hyperglycemia, and is often associated with all three, causing damage to the cardiovascular system. Our previous study showed that psyllium and its extracts are a potential therapeutic agent for the treatment of hyperuricemia. Questions remain to be answered and fully explored about the uric acid-lowering effects of psyllium and its mechanisms. Such answers may pave the way to developing more effective pharmaceutical strategies using psyllium for the treatment of patients with CMDs.
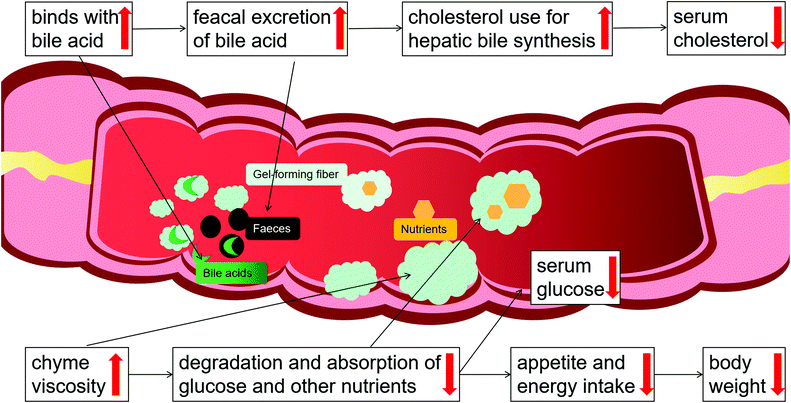 |
| Fig. 4 The health benefits derived from the physical effects of psyllium gel in the intestine. | |
The growing awareness of a healthy diet has led to an increasing demand for functional food; some researchers have added psyllium husk into food products, which has been proved to exert positive effects on health. Further research should focus on minimizing the undesired effects of the great incorporation of psyllium in food to improve its quality and consumer acceptability.
Collectively, the activities against CMDs and clinical implications of psyllium have been extensively studied (Fig. 5). Based on the pleiotropic health benefits and fewer adverse effects, other high-quality trials with long-term follow-up are required to provide more compelling evidence of its safety and efficacy. In addition, with the growing awareness of a healthy diet, psyllium has been added to food products, and further research should focus on minimizing the undesired effects of a great incorporation of psyllium in food to improve its quality and consumer acceptability.
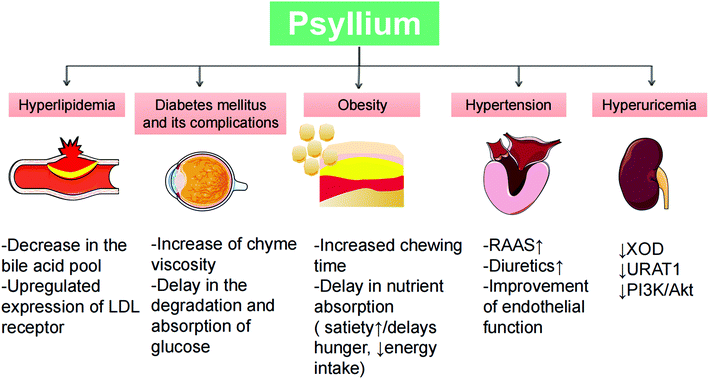 |
| Fig. 5 An overview of the major beneficial effects of psyllium on CMDs and their underlying mechanisms. | |
Author contributions
CC and CS wrote the main text. LY-X, MX, YL-W, ZH-S, LK-J and FD participated in the conception of the review. XN-C revised the manuscript.
Conflicts of interest
The authors declare that they do not have anything to disclose regarding conflict of interest with respect to this manuscript.
Acknowledgements
This work was funded by grants from the National Natural Science Foundation of China (no. 81973842). We thank X. N. Cui for the guidance and revision of this manuscript.
References
-
G. H. Rao, Cardiometabolic Diseases, Risk Stratification, and, Management, 2020 Search PubMed.
- D. K. Arnett, R. S. Blumenthal, M. A. Albert, A. B. Buroker, Z. D. Goldberger, E. J. Hahn, C. D. Himmelfarb, A. Khera, D. Lloyd-Jones, J. W. McEvoy, E. D. Michos, M. D. Miedema, D. Muñoz, S. C. Smith, S. S. Virani, K. A. Williams, J. Yeboah and B. Ziaeian, 2019 ACC/AHA Guideline on the Primary Prevention of Cardiovascular Disease: A Report of the American College of Cardiology/American Heart Association Task Force on Clinical Practice Guidelines, Circulation, 2019, 140, e596–e646 Search PubMed.
- A. Pirillo, M. Casula, E. Olmastroni, G. D. Norata and A. L. Catapano, Global epidemiology of dyslipidaemias, Nat. Rev. Cardiol., 2021, 18, 689–700 CrossRef CAS PubMed.
- M. Laakso and J. Kuusisto, Insulin resistance and hyperglycaemia in cardiovascular disease development, Nat. Rev. Endocrinol., 2014, 10, 293–302 CrossRef CAS PubMed.
- M. A. Alpert, K. Karthikeyan, O. Abdullah and R. Ghadban, Obesity and Cardiac Remodeling in Adults: Mechanisms and Clinical Implications, Prog. Cardiovasc. Dis., 2018, 61, 114–123 CrossRef PubMed.
- K. N. Grooms, M. J. Ommerborn, D. Q. Pham, L. Djoussé and C. R. Clark, Dietary fiber intake and cardiometabolic risks among US adults, NHANES 1999–2010, Am. J. Med., 2013, 126, 1059–1067.e1-4 CrossRef CAS PubMed.
- C. C. T. Clark, M. Salek, E. Aghabagheri and S. Jafarnejad, The effect of psyllium supplementation on blood pressure: a systematic review and meta-analysis of randomized controlled trials, Korean J. Intern. Med., 2020, 35, 1385–1399 CrossRef PubMed.
- V. Vaughan, The encyclopedia of medicinal plants - Chevallier,A, Library Journal, 1996, 121, 82–82 Search PubMed.
- S. Zhang, J. Hu, Y. Sun, H. Tan, J. Yin, F. Geng and S. Nie, Review of structure and bioactivity of the (Plantaginaceae) polysaccharides, Food Chem.: X, 2021, 12, 100158 CAS.
-
R. Masood and M. Miraftab, Psyllium: Current and Future Applications, in Medical and Healthcare Textiles, 2010, vol. 35, pp. 244–253 Search PubMed.
- M. Belorio and M. Gómez, Psyllium: a useful functional ingredient in food systems, Crit. Rev. Food Sci. Nutr., 2022, 62, 527–538 CrossRef CAS PubMed.
- W. Ashraf, R. F. Pfeiffer, F. Park, J. Lof and E. M. M. Quigley, Constipation in Parkinson's disease: Objective assessment and response to psyllium, Mov. Disord., 1997, 12, 946–951 CrossRef CAS PubMed.
- J. Jalanka, G. Major, K. Murray, G. Singh, A. Nowak, C. Kurtz, I. Silos-Santiago, J. M. Johnston, W. M. de Vos and R. Spiller, The Effect of Psyllium Husk on Intestinal Microbiota in Constipated Patients and Healthy Controls, Int. J. Mol. Sci., 2019, 20, 433 CrossRef PubMed.
- A. Erdogan, S. S. C. Rao, D. Thiruvaiyaru, Y. Y. Lee, E. Coss Adame, J. Valestin and M. O’Banion, Randomised clinical trial: mixed soluble/insoluble fibre vs. psyllium for chronic constipation, Aliment Pharmacol Ther, 2016, 44, 35–44 CrossRef CAS PubMed.
- M. H. Mehmood, N. Aziz, M. N. Ghayur and A. H. Gilani, Pharmacological Basis for the Medicinal Use of Psyllium Husk (Ispaghula) in Constipation and Diarrhea, Dig. Dis. Sci., 2011, 56, 1460–1471 CrossRef PubMed.
- N. Washington, M. Harris, A. Mussellwhite and R. C. Spiller, Moderation of lactulose-induced diarrhea by psyllium: effects on motility and fermentation, Am. J. Clin. Nutr., 1998, 67, 317–321 CrossRef CAS PubMed.
- A. P. González, A. Flores-Ramírez, K. P. Gutiérrez-Castro, C. Luévano-Contreras, A. Gómez-Ojeda, G. P. Sosa-Bustamante, R. Caccavello, J. C. Barrera-de León, M. E. Garay-Sevilla and A. Gugliucci, Reduction of small dense LDL and Il-6 after intervention with Plantago psyllium in adolescents with obesity: a parallel, double blind, randomized clinical trial, Eur. J. Pediatr., 2021, 180, 2493–2503 CrossRef PubMed.
- L. Ji-Ping, T. Ren-Chao, S. Xiao-Meng, Z. Hao-Yue, S. Shuai, X. Ai-Zhen, W. Zheng-Tao and Y. Li, Comparison of main chemical composition of Plantago asiatica L. and P. depressa Willd. seed extracts and their anti-obesity effects in high-fat diet-induced obese mice, Phytomedicine, 2021, 81, 153362 CrossRef PubMed.
- A. S. Abutair, I. A. Naser and A. T. Hamed, Soluble fibers from psyllium improve glycemic response and body weight among diabetes type 2 patients (randomized control trial), Nutr. J., 2016, 15, 86 CrossRef PubMed.
- J. W. Anderson, L. D. Allgood, J. Turner, P. R. Oeltgen and B. P. Daggy, Effects of psyllium on glucose and serum lipid responses in men with type 2 diabetes and hypercholesterolemia, Am. J. Clin. Nutr., 1999, 70, 466–473 CrossRef CAS PubMed.
- J. M. A. Hannan, L. Ali, J. Khaleque, M. Akhter, P. R. Flatt and Y. H. A. Abdel-Wahab, Aqueous extracts of husks of Plantago ovata reduce hyperglycaemia in type 1 and type 2 diabetes by inhibition of intestinal glucose absorption, Br. J. Nutr., 2006, 96, 131–137 CrossRef CAS PubMed.
- S. Noureddin, J. Mohsen and A. Payman, Effects of psyllium vs. placebo on constipation, weight, glycemia, and lipids: A randomized trial in patients with type 2 diabetes and chronic constipation, Complement. Ther. Med., 2018, 40, 1–7 CrossRef PubMed.
- N. Soltanian and M. Janghorbani, Effect of flaxseed or psyllium vs. placebo on management of constipation, weight, glycemia, and lipids: A randomized trial in constipated patients with type 2 diabetes, Clin. Nutr. ESPEN, 2019, 29, 41–48 CrossRef PubMed.
- Z. Akbarzadeh, M. Nourian, G. Askari and M. R. Maracy, The effect of Psyllium on body composition measurements and liver enzymes in overweight or obese adults with nonalcoholic fatty liver disease (NAFLD), Int. J. Adv. Biotechnol. Res., 2016, 7, 1545–1554 Search PubMed.
- J. Brum, D. Ramsey, J. McRorie, B. Bauer and S. L. Kopecky, Meta-Analysis of Usefulness of Psyllium Fiber as Adjuvant Antilipid Therapy to Enhance Cholesterol Lowering Efficacy of Statins, Am. J. Cardiol., 2018, 122, 1169–1174 CrossRef CAS PubMed.
- V. Ganji and J. Kuo, Serum lipid responses to psyllium fiber: differences between pre- and post-menopausal, hypercholesterolemic women, Nutr. J., 2008, 7, 22 CrossRef PubMed.
- E. Jovanovski, S. Yashpal, A. Komishon, A. Zurbau, S. Blanco Mejia, H. V. T. Ho, D. Li, J. Sievenpiper, L. Duvnjak and V. Vuksan, Effect of psyllium (Plantago ovata) fiber on LDL cholesterol and alternative lipid targets, non-HDL cholesterol and apolipoprotein B: a systematic review and meta-analysis of randomized controlled trials, Am. J. Clin. Nutr., 2018, 108, 922–932 CrossRef PubMed.
- S. A. Ribas, D. B. Cunha, R. Sichieri and L. C. Santana da Silva, Effects of psyllium on LDL-cholesterol concentrations in Brazilian children and adolescents: a randomised, placebo-controlled, parallel clinical trial, Br. J. Nutr., 2015, 113, 134–141 CrossRef CAS PubMed.
- R. Solà, E. Bruckert, R.-M. Valls, S. Narejos, X. Luque, M. Castro-Cabezas, G. Doménech, F. Torres, M. Heras, X. Farrés, J.-V. Vaquer, J.-M. Martínez, M.-C. Almaraz and A. Anguera, Soluble fibre (Plantago ovata husk) reduces plasma low-density lipoprotein (LDL) cholesterol, triglycerides, insulin, oxidised LDL and systolic blood pressure in hypercholesterolaemic patients: A randomised trial, Atherosclerosis, 2010, 211, 630–637 CrossRef PubMed.
- Z. H. Wei, H. Wang, X. Y. Chen, B. S. Wang, Z. X. Rong, B. S. Wang, B. H. Su and H. Z. Chen, Time- and dose-dependent effect of psyllium on serum lipids in mild-to-moderate hypercholesterolemia: a meta-analysis of controlled clinical trials, Eur. J. Clin. Nutr., 2009, 63, 821–827 CrossRef CAS PubMed.
- R. J. Shulman, E. B. Hollister, K. Cain, D. I. Czyzewski, M. M. Self, E. M. Weidler, S. Devaraj, R. A. Luna, J. Versalovic and M. Heitkemper, Psyllium Fiber Reduces Abdominal Pain in Children With Irritable Bowel Syndrome in a Randomized, Double-Blind Trial, Clin. Gastroenterol. Hepatol., 2017, 15, 712–719.e4 CrossRef CAS PubMed.
- B. Singh, Psyllium as therapeutic and drug delivery agent, Int. J. Pharm., 2007, 334, 1–14 CrossRef CAS PubMed.
- O. Alabaster, Z. C. Tang, A. Frost and N. Shivapurkar, Potential Synergism between wheat Bran and Psyllium - Enhanced Inhibition of Colon-Cancer, Cancer Lett., 1993, 75, 53–58 CrossRef CAS PubMed.
- G. S. Souza, R. D. Bergamasco, A. P. Stafussa and G. S. Madrona, Ultrasound-assisted extraction of Psyllium mucilage: Evaluation of functional and technological properties, Emir. J. Food Agric., 2020, 32, 238–244 CrossRef.
- M. M. Abdullah, A. D. H. Aldughpassi, J. S. Sidhu, M. Y. Al-Foudari and A. R. A. Al-Othman, Effect of psyllium husk addition on the instrumental texture and consumer acceptability of high-fiber wheat pan bread and buns, Ann. Agric. Sci., 2021, 66, 75–80 CrossRef.
- S. Beikzadeh, S. H. Peighambardoust, A. Homayouni-Rad and M. Beikzadeh, Effects of Psyllium and Marve Seed Mucilages on Physical, Sensory and Staling Properties of Sponge Cake, J. Agric. Sci. Technol., 2017, 19, 1079–1089 Search PubMed.
- C. Fratelli, F. G. Santos, D. G. Muniz, S. Habu, A. R. C. Braga and V. D. Capriles, Psyllium Improves the Quality and Shelf Life of Gluten-Free Bread, Foods, 2021, 10(5) DOI:10.1080/15428052.2022.2060160.
- C. Cappa, M. Lucisano and M. Mariotti, Influence of Psyllium, sugar beet fibre and water on gluten-free dough properties and bread quality, Carbohydr. Polym., 2013, 98, 1657–1666 CrossRef CAS PubMed.
- M. Mariotti, M. Lucisano, M. A. Pagani and P. K. W. Ng, The role of corn starch, amaranth flour, pea isolate, and Psyllium flour on the rheological properties and the ultrastructure of gluten-free doughs, Food Res. Int., 2009, 42, 963–975 CrossRef CAS.
- A. Ziemichod, M. Wojcik and R. Rozylo, Seeds of Plantago psyllium and Plantago ovata: Mineral composition, grinding, and use for gluten-free bread as substitutes for hydrocolloids, J. Food Process Eng., 2019, 42, e12931 CrossRef.
- J. M. Cowley, L. A. O'Donovan and R. A. Burton, The composition of Australian Plantago seeds highlights their potential as nutritionally-rich functional food ingredients, Sci. Rep., 2021, 11, 12692 CrossRef CAS PubMed.
- M. H. Fischer, N. X. Yu, G. R. Gray, J. Ralph, L. Anderson and J. A. Marlett, The gel-forming polysaccharide of psyllium husk (Plantago ovata Forsk), Carbohydr. Res., 2004, 339, 2009–2017 CrossRef CAS PubMed.
- P. Fradinho, R. Soares, A. Niccolai, I. Sousa and A. Raymundo, Psyllium husk gel to reinforce structure of gluten-free pasta?, LWT–Food Sci. Technol., 2020, 131, 109787 CrossRef CAS.
- Z. S. Ladjevardi, S. M. T. Gharibzahedi and M. Mousavi, Development of a stable low-fat yogurt gel using functionality of psyllium (Plantago ovata Forsk) husk gum, Carbohydr. Polym., 2015, 125, 272–280 CrossRef CAS PubMed.
- A. T. Noguerol, M. M. Igual and M. J. Pagan, Developing psyllium fibre gel-based foods: Physicochemical, nutritional, optical and mechanical properties, Food Hydrocolloids, 2022, 122, 107108 CrossRef CAS.
- R. Santos, N. Melo and A. Sanches-Silva, Psyllium (Plantago ovata Forsk): From evidence of health benefits to its food application, Trends Food Sci. Technol., 2020, 96, 166–175 CrossRef.
- A. B. Samuelsen, I. Lund, J. M. Djahromi, B. S. Paulsen, J. K. Wold and S. H. Knutsen, Structural features and anti-complementary activity of some heteroxylan polysaccharide fractions from the seeds of Plantago major L, Carbohydr. Polym., 1999, 38, 133–143 CrossRef CAS.
- R. W. W. Chong, M. Ball, C. McRae and N. H. Packer, Comparing the chemical composition of
dietary fibres prepared from sugarcane, psyllium husk and wheat dextrin, Food Chem., 2019, 298, 125032 CrossRef CAS PubMed.
- A. M. Bernstein, B. Titgemeier, K. Kirkpatrick, M. Golubic and M. F. Roizen, Major Cereal Grain Fibers and Psyllium in Relation to Cardiovascular Health, Nutrients, 2013, 5, 1471–1487 CrossRef CAS PubMed.
- H. Pawar and C. Varkhade, Isolation, characterization and investigation of Plantago ovata husk polysaccharide as superdisintegrant, Int. J. Biol. Macromol., 2014, 69, 52–58 CrossRef CAS PubMed.
- M. K. Patel, A. Mishra and B. Jha, Non-targeted Metabolite Profiling and Scavenging Activity Unveil the Nutraceutical Potential of Psyllium (Plantago ovata Forsk), Front. Plant Sci., 2016, 7, 431 Search PubMed.
- L. A. Moreno, B. Tresaco, G. Bueno, J. Fleta, G. Rodriguez, J. M. Garagorri and M. Bueno, Psyllium fibre and the metabolic control of obese children and adolescents, J. Physiol. Biochem., 2003, 59, 235–242 CrossRef CAS PubMed.
- M. A. Hussain, G. Muhammad, I. Jantan and S. N. A. Bukhari, Psyllium Arabinoxylan: A Versatile Biomaterial for Potential Medicinal and Pharmaceutical Applications, Polym. Rev., 2016, 56, 1–30 CrossRef CAS.
- X. Ji, C. Hou and X. Guo, Physicochemical properties, structures, bioactivities and future prospective for polysaccharides from Plantago L. (Plantaginaceae): A review, Int. J. Biol. Macromol., 2019, 135, 637–646 CrossRef CAS PubMed.
- J. Zhang, C. Wen, H. Zhang and Y. Duan, Review of isolation, structural properties, chain conformation, and bioactivities of psyllium polysaccharides, Int. J. Biol. Macromol., 2019, 139, 409–420 CrossRef CAS PubMed.
- M. K. Patel, B. Tanna, H. Gupta, A. Mishra and B. Jha, Physicochemical, scavenging and anti-proliferative analyses of polysaccharides extracted from psyllium (Plantago ovata Forssk) husk and seeds, Int. J. Biol. Macromol., 2019, 133, 190–201 CrossRef CAS PubMed.
- S. Mukherjee, C. A. Pujol, S. Jana, E. B. Damonte, B. Ray and S. Ray, Chemically sulfated arabinoxylans from Plantago ovata seed husk: Synthesis, characterization and antiviral activity, Carbohydr. Polym., 2021, 256, 117555 CrossRef CAS PubMed.
- P. Talukder, S. Talapatra, N. Ghoshal and S. Sen Raychaudhuri, Antioxidant activity and high-performance liquid chromatographic analysis of phenolic compounds during in vitro callus culture of Plantago ovata Forsk. and effect of exogenous additives on accumulation of phenolic compounds, J. Sci. Food Agric., 2016, 96, 232–244 CrossRef CAS PubMed.
- L.-C. Chiang, L. T. Ng, W. Chiang, M.-Y. Chang and C.-C. Lin, Immunomodulatory activities of flavonoids, monoterpenoids, triterpenoids, iridoid glycosides and phenolic compounds of Plantago species, Planta Med., 2003, 69, 600–604 CrossRef CAS PubMed.
- D. Granato, F. J. Barba, D. Bursać Kovačević, J. M. Lorenzo, A. G. Cruz and P. Putnik, Functional Foods: Product Development, Technological Trends, Efficacy Testing, and Safety, Annu. Rev. Food Sci. Technol., 2020, 11, 93–118 CrossRef CAS PubMed.
- P. Fradinho, M. C. Nunes and A. Raymundo, Developing consumer acceptable biscuits enriched with Psyllium fibre, J. Food Sci. Technol., 2015, 52, 4830–4840 CrossRef CAS PubMed.
- F. G. Santos, E. V. Aguiar, A. R. C. Braga, N. M. M. Alencar, C. M. Rosell and V. D. Capriles, An integrated instrumental and sensory approach to describe the effects of chickpea flour, psyllium, and their combination at reducing gluten-free bread staling, Food Packag. Shelf Life, 2021, 28, 100659 CrossRef.
- B. Pareyt and J. A. Delcour, The role of wheat flour constituents, sugar, and fat in low moisture cereal based products: A review on sugar-snap cookies, Crit. Rev. Food Sci. Nutr., 2008, 48, 824–839 CrossRef CAS PubMed.
- S. D. Oliver, The long-term safety and tolerability of ispaghula husk, J. R. Soc. Promot. Health, 2000, 120, 107–111 CrossRef CAS PubMed.
- M. Sierra, J. J. García, N. Fernández, M. J. Diez and A. P. Calle, Therapeutic effects of psyllium in type 2 diabetic patients, Eur. J. Clin. Nutr., 2002, 56, 830–842 CrossRef CAS PubMed.
- K. M. E. Luccia, B Psyllium reduces relative calcium bioavailability and induces negative changes in bone composition in weanling Wistar rats, Nutr. Res., 2002, 22, 1027–1040 CrossRef.
- M. E. K. Barbara and H. D. Luccia, In vitro availability of calcium from sources of cellulose, methylcellulose, and psyllium, Food Chem., 2002, 77, 139–146 CrossRef.
- R. R. Lantner, B. R. Espiritu, P. Zumerchik and M. C. Tobin, Anaphylaxis following ingestion of a psyllium-containing cereal, J. Am. Med. Assoc., 1990, 264, 2534–2536 CrossRef CAS PubMed.
- J. W. McRorie and N. M. McKeown, Understanding the Physics of Functional Fibers in the Gastrointestinal Tract: An Evidence-Based Approach to Resolving Enduring Misconceptions about Insoluble and Soluble Fiber, J. Acad. Nutr. Diet., 2017, 117, 251–264 CrossRef PubMed.
- J. W. McRorie, Psyllium is not fermented in the human gut, Neurogastroenterol. Motil., 2015, 27, 1681–1682 CrossRef PubMed.
- J. McRorie, Clinical Data Support that Psyllium Is Not Fermented in the Gut, Am. J. Gastroenterol., 2013, 108, 1541–1541 CrossRef PubMed.
- R. A. Goodlad, B. Ratcliffe, C. Y. Lee and N. A Wright, Dietary fibre and the gastrointestinal tract: differing trophic effects on muscle and mucosa of the stomach, small intestine and colon, Eur. J. Clin. Nutr., 1995, 49(Suppl 3), S178–S181 Search PubMed.
- O. Alabaster, Z. C. Tang, A. Frost and N. Shivapurkar, Potential synergism between wheat bran and psyllium: enhanced inhibition of colon cancer, Cancer Lett., 1993, 75, 53–58 CrossRef CAS PubMed.
- J. Roberts-Andersen, T. Mehta and R. B. Wilson, Reduction of DMH-induced colon tumors in rats fed psyllium husk or cellulose, Nutr. Cancer, 1987, 10, 129–136 CrossRef CAS PubMed.
- J. Citronberg, E. D. Kantor, J. D. Potter and E. White, A prospective study of the effect of bowel movement frequency, constipation, and laxative use on colorectal cancer risk, Am. J. Gastroenterol., 2014, 109, 1640–1649 CrossRef PubMed.
- J. W. McRorie and N. M. McKeown, Psyllium Husk Should Be Taken at Higher Dose with Sufficient Water to Maximize Its Efficacy Response, J. Acad. Nutr. Diet., 2017, 117, 681–682 CrossRef PubMed.
- GBD 2017 Causes of Death Collaborators, Global, regional, and national age-sex-specific mortality for 282 causes of death in 195 countries and territories, 1980-2017: a systematic analysis for the Global Burden of Disease Study 2017, Lancet, 2018, 392, 1736–1788 CrossRef.
- P. E. Penson and M. Banach, Nutraceuticals for the Control of Dyslipidaemias in Clinical Practice, Nutrients, 2021, 13, 2957 CrossRef CAS PubMed.
- J. Pedro-Botet, J. Millán Núñez-Cortés, J. J. Chillarón, J. A. Flores-Le Roux and J. Rius, Severity of statin-induced adverse effects on muscle and associated conditions: data from the DAMA study, Expert Opin. Drug Saf., 2016, 15, 1583–1587 CrossRef CAS PubMed.
- K. V. Lambeau and J. W. McRorie, Fiber supplements and clinically proven health benefits: How to recognize and recommend an effective fiber therapy, J. Am. Assoc. Nurse Pract., 2017, 29, 216–223 CrossRef PubMed.
- CFR - Code of Federal Regulations Title 21, https://www.accessdata.fda.gov/scripts/cdrh/cfdocs/cfcfr/CFRSearch.cfm?fr=101.81.
- C. J. Shanahan, R. D. Gibb, J. W. McRorie, J. M. Brum and M. E. Ritchey, Economic impact analysis of the coronary heart disease-attributed health care cost savings and productivity gains from the use of Psyllium, Nutr. Food Sci., 2019, 50, 497–513 CrossRef.
- G. Banderali, M. E. Capra, C. Viggiano, G. Biasucci and C. Pederiva, Nutraceuticals in Paediatric Patients with Dyslipidaemia, Nutrients, 2022, 14, 569 CrossRef CAS PubMed.
- J. D. Horton, J. A. Cuthbert and D. K. Spady, Regulation of hepatic 7 alpha-hydroxylase expression by dietary psyllium in the hamster, J. Clin. Invest., 1994, 93, 2084–2092 CrossRef CAS PubMed.
- V. Vuksan, A. L. Jenkins, A. L. Rogovik, C. D. Fairgrieve, E. Jovanovski and L. A. Leiter, Viscosity rather than quantity of dietary fibre predicts cholesterol-lowering effect in healthy individuals, Br. J. Nutr., 2011, 106, 1349–1352 CrossRef CAS PubMed.
- B. Sun, Z. Luo and J. Zhou, Comprehensive elaboration
of glycemic variability in diabetic macrovascular and microvascular complications, Cardiovasc. Diabetol., 2021, 20, 9 CrossRef PubMed.
- J. G. Pastors, P. W. Blaisdell, T. K. Balm, C. M. Asplin and S. L. Pohl, Psyllium fiber reduces rise in postprandial glucose and insulin concentrations in patients with non-insulin-dependent diabetes, Am. J. Clin. Nutr., 1991, 53, 1431–1435 CrossRef CAS PubMed.
- S. A. Ziai, B. Larijani, S. Akhoondzadeh, H. Fakhrzadeh, A. Dastpak, F. Bandarian, A. Rezai, H. N. Badi and T. Emami, Psyllium decreased serum glucose and glycosylated hemoglobin significantly in diabetic outpatients, J. Ethnopharmacol., 2005, 102, 202–207 CrossRef CAS PubMed.
- V. Dall'Alba, F. M. Silva, J. P. Antonio, T. Steemburgo, C. P. Royer, J. C. Almeida, J. L. Gross and M. J. Azevedo, Improvement of the metabolic syndrome profile by soluble fibre - guar gum - in patients with type 2 diabetes: a randomised clinical trial, Br. J. Nutr., 2013, 110, 1601–1610 CrossRef PubMed.
- R. D. Gibb, J. W. McRorie, D. A. Russell, V. Hasselblad and D. A. D'Alessio, Psyllium fiber improves glycemic control proportional to loss of glycemic control: a meta-analysis of data in euglycemic subjects, patients at risk of type 2 diabetes mellitus, and patients being treated for type 2 diabetes mellitus, Am. J. Clin. Nutr., 2015, 102, 1604–1614 CrossRef CAS PubMed.
- Y.-S. Lee and H.-S. Jun, Anti-diabetic actions of glucagon-like peptide-1 on pancreatic beta-cells, Metab., Clin. Exp., 2014, 63, 9–19 CrossRef CAS PubMed.
- Y. Kayama, U. Raaz, A. Jagger, M. Adam, I. N. Schellinger, M. Sakamoto, H. Suzuki, K. Toyama, J. M. Spin and P. S. Tsao, Diabetic Cardiovascular Disease Induced by Oxidative Stress, Int. J. Mol. Sci., 2015, 16, 25234–25263 CrossRef CAS PubMed.
- T.-F. Tzeng, W. Y. Liu, S.-S. Liou, T.-Y. Hong and I. M. Liu, Antioxidant-Rich Extract from Plantaginis Semen Ameliorates Diabetic Retinal Injury in a Streptozotocin-Induced Diabetic Rat Model, Nutrients, 2016, 8, 572 CrossRef PubMed.
- M. A. Hashem, N. A. Abd-Allah, E. A. Mahmoud, S. A. Amer and M. Alkafafy, A Preliminary Study on the Effect of Psyllium Husk Ethanolic Extract on Hyperlipidemia, Hyperglycemia, and Oxidative Stress Induced by Triton X-100 Injection in Rats, Biology, 2021, 10, 335 CrossRef CAS PubMed.
- G. Zeng, H. An, D. Fang, W. Wang, Y. Han and C. Lian, Plantamajoside protects H9c2 cells against hypoxia/reoxygenation-induced injury through regulating the akt/Nrf2/HO-1 and NF-κB signaling pathways, J. Recept. Signal Transduction Res., 2020, 1–8, DOI:10.1080/10799893.2020.1859534.
- Q. Ma, Role of nrf2 in oxidative stress and toxicity, Annu. Rev. Pharmacol. Toxicol., 2013, 53, 401–426 CrossRef CAS PubMed.
- S. H. Lim and J. Lee, Supplementation with psyllium seed husk reduces myocardial damage in a rat model of ischemia/reperfusion, Nutr. Res. Pract., 2019, 13, 205–213 CrossRef CAS PubMed.
- N. Cheung and T. Y. Wong, Diabetic retinopathy and systemic vascular complications, Prog. Retinal Eye Res., 2008, 27, 161–176 CrossRef CAS PubMed.
- N. Cheung, P. Mitchell and T. Y. Wong, Diabetic retinopathy, Lancet, 2010, 376, 124–136 CrossRef.
- N. Matsuura, T. Aradate, C. Kurosaka, M. Ubukata, S. Kittaka, Y. Nakaminami, K. Gamo, H. Kojima and M. Ohara, Potent protein glycation inhibition of plantagoside in Plantago major seeds, BioMed Res. Int., 2014, 2014, 208539 Search PubMed.
- U. Ojha, S. Ruddaraju, N. Sabapathy, V. Ravindran, P. Worapongsatitaya, J. Haq, R. Mohammed and V. Patel, Current and Emerging Classes of Pharmacological Agents for the Management of Hypertension, Am. J. Cardiovasc. Drugs, 2022, 22, 271–285 CrossRef PubMed.
- L. Li, X. Zhou, N. Li, M. Sun, J. Lv and Z. Xu, Herbal drugs against cardiovascular disease: traditional medicine and modern development, Drug Discovery Today, 2015, 20, 1074–1086 CrossRef PubMed.
- A. F. G. Cicero, G. Derosa, M. Manca, M. Bove, C. Borghi and A. V. Gaddi, Different effect of psyllium and guar dietary supplementation on blood pressure control in hypertensive overweight patients: a six-month, randomized clinical trial, Clin. Exp. Hypertens., 2007, 29, 383–394 CrossRef CAS PubMed.
- S. Murad, R. Waseem, A. Bashir, H. H. Ashraf and R. A. Sheikh, Keep heart healthy by normalising body weight, blood pressure and weight reduction, Pakistan J. Medical Health Sci., 2011, 5, 228–231 Search PubMed.
- K. Khan, E. Jovanovski, H. V. T. Ho, A. C. R. Marques, A. Zurbau, S. B. Mejia, J. L. Sievenpiper and V. Vuksan, The effect of viscous soluble fiber on blood pressure: A systematic review and meta-analysis of randomized controlled trials, Nutr., Metab. Cardiovasc. Dis., 2018, 28, 3–13 CrossRef CAS PubMed.
- Y.-S. Fu, S.-I. Lue, S.-Y. Lin, C.-L. Luo, C.-C. Chou and C.-F. Weng, Seed Extracts Alleviated Blood Pressure in Phase I-Spontaneous Hypertension Rats, Molecules, 2019, 24, 1734 CrossRef CAS PubMed.
- R.-C. Tong, M. Qi, Q.-M. Yang, P.-F. Li, D.-D. Wang, J.-P. Lan, Z.-T. Wang and L. Yang, Extract of L. Seeds Ameliorates Hypertension in Spontaneously Hypertensive Rats by Inhibition of Angiotensin Converting Enzyme, Front. Pharmacol., 2019, 10, 403 CrossRef CAS PubMed.
- F. Geng, L. Yang, G. Chou and Z. Wang, Bioguided isolation of angiotensin-converting enzyme inhibitors from the seeds of Plantago asiatica L, Phytother. Res., 2010, 24, 1088–1094 CrossRef CAS PubMed.
- K. Obata, K. Ikeda, M. Yamasaki and Y. Yamori, Dietary fiber, psyllium, attenuates salt-accelerated hypertension in stroke-prone spontaneously hypertensive rats, J. Hypertens., 1998, 16, 1959–1964 CrossRef CAS PubMed.
- C. Li, R. Wen, D. W. Liu, Q. Liu, L. P. Yan, J. X. Wu, Y. J. Guo, S. Y. Li, Q. F. Gong and H. Yu, Diuretic Effect and Metabolomics Analysis of Crude and Salt-Processed Plantaginis Semen, Front. Pharmacol., 2020, 11, 563157 CrossRef CAS PubMed.
- M. Galisteo, M. Sánchez, R. Vera, M. González, A. Anguera, J. Duarte and A. Zarzuelo, A diet supplemented with husks of Plantago ovata reduces the development of endothelial dysfunction, hypertension, and obesity by affecting adiponectin and TNF-alpha in obese Zucker rats, J. Nutr., 2005, 135, 2399–2404 CrossRef CAS PubMed.
- W.-R. Son, M.-H. Nam, C.-O. Hong, Y. Kim and K.-W. Lee, Plantamajoside from Plantago asiatica modulates human umbilical vein endothelial cell dysfunction by glyceraldehyde-induced AGEs via MAPK/NF-κB, BMC Complementary Altern. Med., 2017, 17, 66 CrossRef PubMed.
- J.-L. Hu, S.-P. Nie and M.-Y. Xie, High pressure homogenization increases antioxidant capacity and short-chain fatty acid yield of polysaccharide from seeds of Plantago asiatica L, Food Chem., 2013, 138, 2338–2345 CrossRef CAS PubMed.
- B. H. Kim, K. S. Park and I.-M. Chang, Elucidation of anti-inflammatory potencies of Eucommia ulmoides bark and Plantago asiatica seeds, J. Med. Food, 2009, 12, 764–769 CrossRef CAS PubMed.
- W. Fan, B. Zhang, C. Wu, H. Wu, J. Wu, S. Wu, J. Zhang, X. Yang, L. Yang, Z. Hu and X. Wu, Plantago asiatica L. seeds extract protects against cardiomyocyte injury in isoproterenol- induced cardiac hypertrophy by inhibiting excessive autophagy and apoptosis in mice, Phytomedicine, 2021, 91, 153681 CrossRef CAS PubMed.
- L. Shang, L. Pin, S. Zhu, X. Zhong, Y. Zhang, M. Shun, Y. Liu and M. Hou, Plantamajoside attenuates isoproterenol-induced cardiac hypertrophy associated with the HDAC2 and AKT/GSK-3β signaling pathway, Chem.-Biol. Interact., 2019, 307, 21–28 CrossRef CAS PubMed.
- C. Meisinger, W. Koenig, J. Baumert and A. Döring, Uric acid levels are associated with all-cause and cardiovascular disease mortality independent of systemic inflammation in men from the general population: the MONICA/KORA cohort study, Arterioscler., Thromb., Vasc. Biol., 2008, 28, 1186–1192 CrossRef CAS PubMed.
- A. Maloberti, C. Giannattasio, M. Bombelli, G. Desideri, A. F. G. Cicero, M. L. Muiesan, E. A. Rosei, M. Salvetti, A. Ungar, G. Rivasi, R. Pontremoli, F. Viazzi, R. Facchetti, C. Ferri, B. Bernardino, F. Galletti, L. D'Elia, P. Palatini, E. Casiglia, V. Tikhonoff, C. M. Barbagallo, P. Verdecchia, S. Masi, F. Mallamaci, M. Cirillo, M. Rattazzi, P. Pauletto, P. Cirillo, L. Gesualdo, A. Mazza, M. Volpe, G. Tocci, G. Iaccarino, P. Nazzaro, L. Lippa, G. Parati, R. Dell'Oro, F. Quarti-Trevano, G. Grassi, A. Virdis and C. Borghi, Hyperuricemia and Risk of Cardiovascular Outcomes: The Experience of the URRAH (Uric Acid Right for Heart Health) Project, High Blood Pressure Cardiovasc. Prev., 2020, 27, 121–128 CrossRef PubMed.
- M. Kuwabara, K. Niwa, I. Hisatome, T. Nakagawa, C. A. Roncal-Jimenez, A. Andres-Hernando, P. Bjornstad, T. Jensen, Y. Sato, T. Milagres, G. Garcia, M. Ohno, M. A. Lanaspa and R. J. Johnson, Asymptomatic Hyperuricemia Without Comorbidities Predicts Cardiometabolic Diseases: Five-Year Japanese Cohort Study, Hypertension, 2017, 69, 1036–1044 CrossRef CAS PubMed.
- W. Yu and J.-D. Cheng, Uric Acid and Cardiovascular Disease: An Update From Molecular Mechanism to Clinical Perspective, Front. Pharmacol., 2020, 11, 582680 CrossRef CAS PubMed.
- J. X. Zeng, J. Wang, S. W. Zhang, J. X. Zhu, M. Li, W. H. Huang, J. Y. Wan, H. Q. Yao, C. Z. Wang and C. S. Yuan, Antigout Effects of Plantago asiatica: Xanthine Oxidase Inhibitory Activities Assessed by Electrochemical Biosensing Method, Evidence-Based Complementary Altern. Med., 2018, 2018, 1364617 Search PubMed.
- L. D. Kong, Y. Cai, W. W. Huang, C. H. Cheng and R. X. Tan, Inhibition of xanthine oxidase by some Chinese medicinal plants used to treat gout, J. Ethnopharmacol., 2000, 73, 199–207 CrossRef CAS PubMed.
- F. Yang, W. Shi, L. Wang, N. Qin, C. Wang, Y. Guo, G. Xu, J. Fang, X. Yu and Q. Ma, Lipidomics study of the therapeutic mechanism of Plantaginis Semen in potassium oxonate-induced hyperuricemia rat, BMC Complementary Med. Ther., 2021, 21, 175 CrossRef CAS PubMed.
- G. Marcelin, A. L. M. Silveira, L. B. Martins, A. V. Ferreira and K. Clément, Deciphering the cellular interplays underlying obesity-induced adipose tissue fibrosis, J. Clin. Invest., 2019, 129, 4032–4040 CrossRef PubMed.
- NCD Risk Factor Collaboration (NCD-RisC), Trends in adult body-mass index in 200 countries from 1975 to 2014: a pooled analysis of 1698 population-based measurement studies with 19·2 million participants, Lancet, 2016, 387, 1377–1396 CrossRef.
- C. Koliaki, S. Liatis and A. Kokkinos, Obesity and cardiovascular disease: revisiting an old relationship, Metab., Clin. Exp., 2019, 92, 98–107 CrossRef CAS PubMed.
- J. M. Brum, R. D. Gibb, J. C. Peters and R. D. Mattes, Satiety effects of psyllium in healthy volunteers, Appetite, 2016, 105, 27–36 CrossRef PubMed.
- D. Rigaud, F. Paycha, A. Meulemans, M. Merrouche and M. Mignon, Effect of psyllium on gastric emptying, hunger feeling and food intake in normal volunteers: a double blind study, Eur. J. Clin. Nutr., 1998, 52, 239–245 CrossRef CAS PubMed.
- J. F. Bergmann, O. Chassany, A. Petit, R. Triki, C. Caulin and J. M. Segrestaa, Correlation between echographic gastric emptying and appetite: influence of psyllium, Gut, 1992, 33, 1042–1043 CrossRef CAS PubMed.
- L. J. Karhunen, K. R. Juvonen, S. M. Flander, K.-H. Liukkonen, L. Lähteenmäki, M. Siloaho, D. E. Laaksonen, K.-H. Herzig, M. I. Uusitupa and K. S. Poutanen, A psyllium fiber-enriched meal strongly attenuates postprandial gastrointestinal peptide release in healthy young adults, J. Nutr., 2010, 140, 737–744 CrossRef CAS PubMed.
- Z. Xiao, H. Chen, Y. Zhang, H. Deng, K. Wang, A. S. Bhagavathula, S. J. Almuhairi, P. M. Ryan, J. Rahmani, M. Dang, V. Kontogiannis, A. Vick and Y. Wei, The effect of psyllium consumption on weight, body mass index, lipid profile, and glucose metabolism in diabetic patients: A systematic review and dose-response meta-analysis of randomized controlled trials, Phytother. Res., 2020, 34, 1237–1247 CrossRef CAS PubMed.
- M. D. Mofrad, H. Mozaffari, S. M. Mousavi, A. Sheikhi and A. Milajerdi, The effects of psyllium supplementation on body weight, body mass index and waist circumference in adults: A systematic review and dose-response meta-analysis of randomized controlled trials, Crit. Rev. Food Sci. Nutr., 2020, 60, 859–872 CrossRef PubMed.
- J. J. Fuster, N. Ouchi, N. Gokce and K. Walsh, Obesity-Induced Changes in Adipose Tissue Microenvironment and Their Impact on Cardiovascular Disease, Circ. Res., 2016, 118, 1786–1807 CrossRef CAS PubMed.
- D. E. King, A. G. Mainous, B. M. Egan, R. F. Woolson and M. E. Geesey, Effect of psyllium fiber supplementation on C-reactive protein: the trial to reduce inflammatory markers (TRIM), Ann. Fam. Med., 2008, 6, 100–106 CrossRef PubMed.
- Q. Zhou, W. Lu, Y. Niu, J. Liu, X. Zhang, B. Gao, C. C. Akoh, H. Shi and L. L. Yu, Identification and quantification of phytochemical composition and anti-inflammatory, cellular antioxidant, and radical scavenging activities of 12 Plantago species, J. Agric. Food Chem., 2013, 61, 6693–6702 CrossRef CAS PubMed.
Footnotes |
† First author. |
‡ Co-first author. |
|
This journal is © The Royal Society of Chemistry 2022 |
Click here to see how this site uses Cookies. View our privacy policy here.