DOI:
10.1039/D1QI01230D
(Research Article)
Inorg. Chem. Front., 2022,
9, 186-194
Heterometallic Re/Mo and Re/W cubane-type cluster complexes†
Received
27th September 2021
, Accepted 14th November 2021
First published on 16th November 2021
Abstract
A series of heterometallic tetrahedral cluster complexes with the general formula [{MxRe4−xSe4}(CN)12]n− (M = Mo, W; x = 2, 3) were obtained using the reaction of ReI3 and MO3 with selenium and KCN at elevated temperatures. Compounds [ReW3Se4(CN)12]6− and [Re2W2Se4(CN)12]6− are the first rhenium–tungsten heterometallic clusters. Crystal structures, electrochemical properties in aqueous solutions and magnetic properties of crystalline samples have been studied in detail. It has been shown that new cluster anions demonstrate a regular change in geometric characteristics and redox potentials depending on the composition of the cluster core.
Introduction
Chalcogenide cluster complexes of group 5–7 transition metals with a tetrahedral metal core are one of the most numerous and widely studied groups of compounds with metal–metal bonds.1 These compounds are based on {M4Q4} (Q = S, Se, Te) cores, in which the triangular faces are capped by μ3 chalcogenide ligands, forming a symmetric “cubane” fragment. Clusters of this type have long been known in the composition of solid-state phases possessing interesting electronic and magnetic properties.2 Also, the chemistry of discrete soluble tetrahedral clusters of molybdenum, tungsten, and rhenium has been extensively developed. Due to the presence of strong bonding between the atoms of the cluster cores, these clusters are resistant to solvolysis and can be obtained using various methods: in solutions by reducing mononuclear and binuclear fragments,3 “building up” the fourth vertex to a trinuclear cluster4 or by reaction at elevated temperatures.5
Since the composition of the metallic core determines the physical and chemical properties of the related cluster compounds, controlled synthesis of cluster compounds with heterometallic cores is one of the most effective ways to vary the properties of clusters while maintaining their geometry. In the case of cubane clusters, a complete family of heterometallic Mo/W aquo ions with the general formula [MoxW4−xQ4(H2O)12]n+ (Q = S or Se; x = 1–3; n = 4–5) was synthesized by reactions of trinuclear homo- and heterometallic precursors with [Mo2Cl8]4− or M(CO)6 (M = Mo, W).6 It was shown that a change in the M/W ratio in the cluster core has a principal effect on the electrochemical potentials of the clusters and their absorption spectra. So far, examples of heterometallic cubane complexes of Mo/Re and W/Re composition have been limited to insoluble solid-state phases Re4−xMoxS4Te4
7 and M[Mo2Re2S8] (M = Fe, Co, Ni, Zn).8 In this article, we report on the preparation, and study of the structure and properties of soluble heterometallic clusters [{MxRe4−xSe4}(CN)12]n− (M = Mo, W; x = 2, 3; n = 6, 7).
Experimental section
Materials and methods
ReI3 was prepared as described previously.9 Other reagents and solvents were used as received from commercial sources.
Electrospray ionization mass spectrometry (ESI-MS) was carried out at the Center of Collective Use “Mass spectrometric investigations” SB RAS with a Bruker maXis 4G high-resolution quadrupole time-of-flight mass spectrometer (negative ion mode, direct injection with an automatic syringe at 180 μl per hour, voltage +2200 V, nebulizer pressure: 1 bar, dry gas flow: 4 L min−1, and dry gas temperature: 180 °C). UV/vis spectra in the wavelength range of 200–1100 nm were recorded with an Agilent Cary 60 spectrometer. FT-IR spectra using KBr pellets were recorded with a Bruker Scimitar FTS 2000 spectrometer in the range of 4000–400 cm−1. Elemental analysis was performed using a EuroVector EA3000 Elemental Analyzer. Energy dispersion spectroscopy (EDS) was performed using a Hitachi TM-3000 scanning electron microscope equipped with a Bruker Nano EDS analyzer. X-ray powder diffraction data were collected on a Philips APD 1700 instrument. Cyclic voltammetry was carried out with an Elins P-20X8 voltammetry analyzer using a three-electrode scheme with GC working, Pt auxiliary and Ag/AgCl/3.5 M KCl reference electrodes. Investigations were carried out for 2.5 × 10−3 M solutions of cluster compounds 1–4 in 0.1 M KCl in H2O under an Ar atmosphere.
The magnetic properties of polycrystalline samples were studied in the temperature range of T = 1.77–300 K at magnetic fields H up to 10 kOe using a Quantum Design MPMS-XL SQUID magnetometer. The paramagnetic component of the molar magnetic susceptibility, χp(T), was determined by subtracting the contributions of temperature-independent diamagnetism χd and ferromagnetism of trace impurities χF from the measured values of the total molar susceptibility χ = M/H (where M is the magnetization). The contribution χd was calculated according to the additive Pascal scheme, whereas field dependences M(H) and temperature dependences M(T) at different magnetic fields were measured to evaluate the ferromagnetic contribution χF. To determine the effective magnetic moment μeff and Weiss constant θ, the temperature dependences χp(T) were analyzed using the Curie–Weiss dependence χp(T) = NAμ2eff/3kB(T − θ), where NA and kB are the Avogadro number and Boltzmann constant, respectively.
Single-crystal diffraction studies
Single crystal XRD data for 1 were collected with a Bruker Apex DUO diffractometer equipped with a 4 K CCD area detector employing graphite-monochromated MoKα radiation (λ = 0.71073 Å). Single crystal XRD data for 2–4 were collected with a Bruker D8 Venture diffractometer with a CMOS PHOTON III detector and an IμS 3.0 source (mirror optics, λ(MoKα) = 0.71073 Å). Absorption corrections were applied with the use of the SADABS program.10 The crystal structures were solved using SHELXT11 and were refined using SHELXL12 programs with OLEX2 GUI.13 Atomic displacement parameters for non-hydrogen atoms were refined anisotropically with the exception of some solvate molecules. The positions of the hydrogen atoms of the methyl groups of methanol were calculated corresponding to their geometrical conditions and refined with the use of a riding model. Hydrogens at OH groups of water and methanol molecules were not located but included in the formula of the compounds. Crystallographic data and refinement details are summarized in Table S1.† CCDC 2111041–2111044† contain the supplementary crystallographic data for this paper.
Preparation of K6[{Mo2Re2Se4}(CN)12]·10H2O (1).
A mixture of ReI3 (0.200 g, 0.35 mmol), MoO3 (0.050 g, 0.35 mmol), Se (0.042 g, 0.53 mmol) and KCN (0.207 g, 3.18 mmol) was placed in a fused silica tube that was evacuated to 2 × 10−3 Torr and sealed. The tube was heated to 450 °C, held at this temperature for 48 h and then cooled at a rate of 50 °C h−1. The products of the reaction were dissolved in boiled water and filtered. The brown solution was evaporated to about 3 mL and cooled to room temperature. Crystallization in MeOH vapor led to the formation of brown prism crystals of 1. The yield was 0.163 g (59%). EDS: K
:
Mo
:
Re
:
Se = 6.3
:
2.1
:
2.0
:
4.2. Elemental analysis: calcd for C12H16K6Mo2N12O8Re2Se4 (K6[{Mo2Re2Se4}(CN)12]·8H2O): C 9.22, H 1.03, N 10.70, found C 9.22, H 0.71, N 10.58%. IR (cm−1): δ(OH) 1619, ν(CN) 2119, ν(OH) 3437. UV-vis (H2O): λ, nm (ε, M−1 cm−1) 234 (32
830), 263 (22
600), 316 (17
860), 356 (7710), 471 (1330).
Preparation of K7[{Mo3ReSe4}(CN)12]·8H2O·2MeOH (2).
ReI3 (0.100 g, 0.18 mmol), MoO3 (0.076 g, 0.53 mmol), Se (0.042 g, 0.53 mmol) and KCN (0.207 g, 3.18 mmol) were combined and reacted as described for 1 at 550 °C. Crystallization in MeOH vapor led to the formation of brown prism crystals of 2. The yield was 0.189 g (71%). EDS: K
:
Mo
:
Re
:
Se = 7.2
:
2.9
:
1.0
:
4.0. Elemental analysis: calcd for C12H20K7Mo3N12O10ReSe4: C 9.26, H 1.30, N 10.80, found C 9.30, H 1.05, N 10.83%. IR (cm−1): δ(OH) 1632, ν(CN) 2104, ν(OH) 3550. UV-vis (H2O): λ, nm (ε, M−1 cm−1) 225 (34
970), 300 (10
850), 330 (7755), 409 (2350).
Preparation of K6[{Re2W2Se4}(CN)12]·10H2O (3).
ReI3 (0.200 g, 0.35 mmol), WO3 (0.081 g, 0.35 mmol), Se (0.042 g, 0.53 mmol) and KCN (0.207 g, 3.18 mmol) were combined and reacted as described for 1 at 500 °C. Crystallization in MeOH vapor led to the formation of brown prism crystals of 3. The yield was 0.189 g (62%). EDS: K
:
Re
:
W
:
Se = 6.2
:
2.0
:
2.1
:
3.8. Elemental analysis: calcd for C12H14K6N12O7Re2Se4W2 (K6[{Re2W2Se4}(CN)12]·7H2O): C 8.34, H 0.82, N 9.72, found C 8.32, H 0.71, N 9.75. IR (cm−1): δ(OH) 1603, ν(CN) 2119, ν(OH) 3510. UV-vis (H2O): λ, nm (ε, M−1 cm−1) 221 (33
860), 249 (22
800), 297 (16
400), 328 (9345), 464 (1240).
Preparation of Cs6[{ReW3Se4}(CN)12]·5.2H2O·MeOH (4).
ReI3 (0.100 g, 0.18 mmol), WO3 (0.123 g, 0.53 mmol), Se (0.042 g, 0.53 mmol) and KCN (0.207 g, 3.18 mmol) were combined and reacted as described for 1 at 500 °C. Crystallization with the addition of 0.200 g of CsCl in MeOH vapor led to the formation of brown needle crystals of 4. The yield was 0.222 g (55%). EDS: Cs
:
Re
:
W
:
Se = 6,1
:
1,0
:
2,8
:
3,8. Elemental analysis: calcd for C13H14.4Cs6N12O6.2ReSe4W3: C 6.82, H 0.63, N 7.34, found C 6.77, H 0.51, N 7.25. IR (cm−1): δ(OH) 1611, ν(CN) 2119, ν(OH) 3430. UV-vis (H2O): λ, nm (ε, M−1 cm−1) 218 (36
810), 316 (12
830), 423 (2615).
Results and discussion
Synthesis
Most of the works devoted to the study of discrete cluster complexes of group 5–7 metals are focused on the study of the properties of compounds containing only one type of metal in the cluster core. However, several groups of heterometallic clusters are known, and their properties have been studied systematically. Apart from the abovementioned compounds [MoxW4−xQ4(H2O)12]n+ (Q = S or Se; x = 1–3; n = 4–5),6 clusters with {MFe3Q4} and {M2Fe2Q4} cores (M = Mo, W), which are the model compounds for the study of the FeMo-cofactor of nitrogenase, are widely known.14 A large series of heterometallic cuboidal compounds were obtained on the basis of trinuclear clusters [Mo3S4] by the addition of the fourth metal atom.1c,15 A common feature of the synthesis of all these compounds is that it proceeds under relatively mild conditions in solution.
As for discrete clusters synthesized by the high-temperature method, the possibility of obtaining octahedral clusters with {Re6−xOsxSe8} (x = 1–3) cores was demonstrated by the reaction between Re, Os, Se, ReCl5 and CsCl at 875 °C.16 It is interesting to note that the ratio of Re/Os atoms in these compounds was controlled by the stoichiometry of the reaction mixture. Compounds based on the {Mo5NbI8} cluster core were obtained in a similar way.17 In addition, in recent years, heterometallic clusters [Re6−xMoxQ8(CN)6]n (x = 1–3), initially obtained in the form of solid solutions a few years ago, have been actively studied.18 The interest in the study of all these compounds is due to their promising physicochemical properties, which are not typical of homometallic analogs. For example, octahedral cluster complexes of rhenium are capable of reversible one-electron oxidation from a state containing 24 CSE (cluster skeletal electrons) to a state with 23 CSE.19 The successive replacement of rhenium atoms with molybdenum in the {Re6−xMoxSe8} (x = 1–3) cluster core makes it possible to obtain electrochemically active complexes with CSE contents from 21 to 24.20 Clusters containing an even number of electrons are diamagnetic, and the ones with an odd number of CSE are paramagnetic, so it is possible to control the magnetic properties of such compounds by applying an electric potential. In addition, it was demonstrated that the cyanide groups of rhenium–molybdenum clusters can be replaced by N-donor ligands under mild conditions without destroying the cluster core,21 which is not typical of homometallic rhenium clusters. Thus, the replacement of metal core atoms with atoms of another metal is a powerful method that allows one to directly influence the properties of the cluster complex.
In this work, in continuation of our studies aimed at obtaining cluster complexes of rhenium based on ReI3,22 we report on the synthesis of heterometallic tetrahedral cluster complexes of rhenium and molybdenum or tungsten. MoO3 was used as a source of molybdenum, and the composition of the reaction mixture can be written as ReI3/MoO3/Se/KCN. At a molar ratio of ReI3
:
MoO3 = 1
:
1, the reaction at a temperature of 500 °C led to the formation of a tetranuclear heterometallic anion [{Mo2Re2Se4}(CN)12]6−, which was isolated as a potassium salt K6[{Mo2Re2Se4}(CN)12]·10H2O (1). In a similar reaction with the ratio of Re
:
Mo = 1
:
3, the anionic complex [{Mo3ReSe4}(CN)12]7−, isolated as the salt K7[{Mo3ReSe4}(CN)12]·8H2O·2MeOH (2), was formed.
Having obtained the heterometallic tetrahedral cyanide complexes of rhenium and molybdenum, we decided to try out the Re/W system. It was found that the interaction of a mixture of ReI3, WO3 (molar ratio: 1
:
1) and selenium with an excess of potassium cyanide at a temperature of 450–500 °C led to the formation of the tetranuclear complex, which was isolated from an aqueous solution in the form of a potassium salt K6[{Re2W2Se4}(CN)12]·10H2O (3). This compound is isostructural to the rhenium–molybdenum analogue 1. When the loading of the starting rhenium iodide and tungsten oxide is changed to a ratio of 1
:
3, under similar conditions at a temperature of 500 °C, an anionic complex with the cluster core {ReW3Se4} was formed and isolated as a cesium salt Cs6[{ReW3Se4}(CN)12]·5.2H2O·MeOH (4). All heterometallic clusters were isolated as individual phases, and their crystal structures were determined by single-crystal XRD. The synthesis temperature was optimized to maximize the reaction yield. To confirm the phase purity of all the obtained complexes, powder diffraction patterns were recorded, which were in good agreement with those calculated from the structural data (Fig. S1–S4†).
As a result of high-temperature synthesis, multicomponent mixtures of clusters were formed, which were unsuitable to chromatographic separation due to the similar geometries and charges of the anions.20a To confirm that compounds 1–4 are composed of only one respective cluster anion, mass spectra were recorded for their aqueous solutions. Mass spectrometry showed the absence of any impurities with different ratios of metals in the cluster core (Fig. 1 and Fig. S5–S7†). It was also found that cluster anions are able to dissociate during ionization, losing CN− ligands, which indicates their possible lability. The most intense signals in the mass spectra of solutions of compounds 1–4 refer to the anions of the composition K3[{Mo2Re2Se4}(CN)11]−, K3[{Mo3ReSe4}(CN)11]−, K4[{Re2W2Se4}(CN)11]− and K4[{ReW3Se4}(CN)11]−.
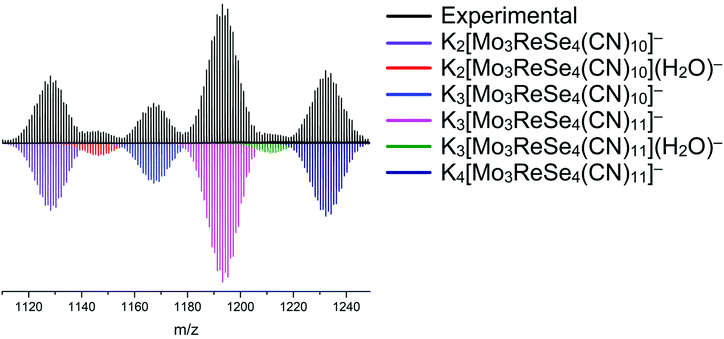 |
| Fig. 1 Experimental (top) vs. calculated (bottom) ESI mass spectra of the aqueous solution of compound 2. | |
All compounds 1–4 are brown in aqueous solutions and the color does not visually change depending on the composition of the cluster core and the number of valence electrons. Their absorption spectra are quite similar to those of the cubane cluster complexes of rhenium with 12 CSE and molybdenum or tungsten with 10 and 11 CSE (Fig. 2a and b). According to the literature data,5a,23 absorption between 200 nm and 400 nm is most intense in all complexes and can be assigned to CN → M (M = Re, Mo, W) charge-transfer transitions. This assumption can be confirmed by the observation of similar transitions in mononuclear octacyanomolybdates and tungstates.24 The lower energy transitions in the visible region take place mainly within the {M4X4} cluster core. For both complexes [{M2Re2Se4}(CN)12]6− and [{M3ReSe4}(CN)12]6−/7−, a slight blue shift of all transitions occurs as a result of “replacing” Mo with W atoms corresponding to a slightly larger gap between the occupied and unoccupied molecular orbitals for W-containing clusters. Fig. 2b shows that the UV/vis spectra of non-isoelectronic anions [{Mo3ReSe4}(CN)12]7− (12 CSE) and [{ReW3Se4}(CN)12]6− (11 CSE) have significant differences, mainly in the intensities of transitions between 250 and 350 nm. At the same time, no absorption peaks were found in the red region (600–900 nm) for all the cluster anions, in contrast to the previously reported homometallic tetrahedral clusters.
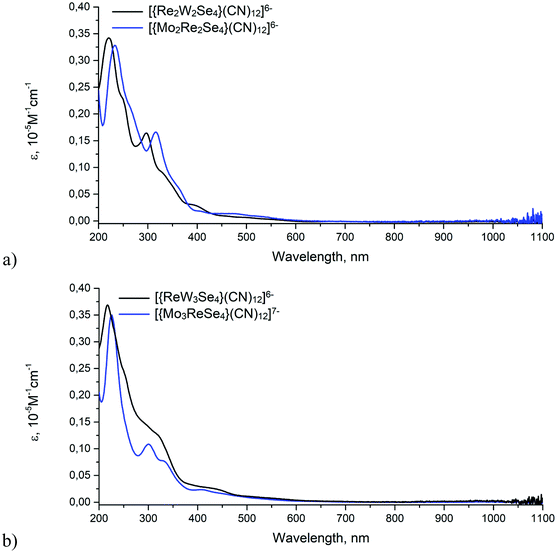 |
| Fig. 2 UV-Vis spectra of {M2Re2} (a) and {M3Re} (b) cluster complexes in aqueous solutions (M = Mo, W). | |
Cluster skeletal electron (CSE) number and electrochemical properties
Clusters [Mo2Re2Se4(CN)12]6−, [Mo3ReSe4(CN)11]7− and [Re2W2Se4(CN)12]6− belong to the family of tetrahedral cyanide complexes [M4Q4(CN)12]n− containing 12 CSE and having six two-electron metal–metal bonds (Table 1). It can be assumed that each metal atom is in the d3 configuration. Then, the formal oxidation state of rhenium atoms is +4, and the oxidation state of molybdenum and tungsten atoms is +3. These oxidation states are characteristic of the corresponding metal atoms in homometallic tetrahedral complexes [{M4Q4}(CN)12]n−.5a,25 For the {ReW3Se4} cluster, the electron-deficient anion [ReW3Se4(CN)12]6− (11 CSE) is in the stable form in the aqueous solution.
Table 1 Relationship between CSE numbers and charge states of the tetrahedral cyanide cluster anions based on non-isoelectronic Re and M = Mo(W) atoms
Cluster |
12 CSE |
11 CSE |
10 CSE |
[Re4Q4(CN)12]n− |
{Re4Q4}8+ |
— |
— |
[Re4Q4(CN)12]4− |
[M2Re2Q4(CN)12]n− |
{Re2M2Q4}6+ |
{Re2M2Q4}7+ |
— |
[Re2M2(CN)12]6− |
[Re2M2(CN)12]5− |
[M3ReQ4(CN)12]n− |
{ReM3Q4}5+ |
{ReM3Q4}6+ |
{ReM3Q4}7+ |
[ReM3(CN)12]7− |
[ReM3(CN)12]6− |
[ReM3(CN)12]5− |
[M4Q4(CN)12]n− |
{M4Q4}4+ |
{M4Q4}5+ |
{M4Q4}6+ |
[M4Q4(CN)12]8− |
[M4Q4(CN)12]7− |
[M4Q4(CN)12]6− |
Voltammograms of the clusters [Mo2Re2Se4(CN)12]6− and [Re2W2Se4(CN)12]6− contain one electrochemical process (Fig. 3a and b) at E1/2 equal to 0.717 и 0.491 V vs. Ag/AgCl, respectively. This process corresponds to the one-electron oxidation [M2Re2Se4(CN)12]6− (12 CSE) ↔ [M2Re2Se4(CN)12]5− (11 CSE). The ratio of anodic to cathodic peak currents (ipa and ipc, respectively) is close to 1; however, the ΔE of the processes exceeds 100 mV, which indicates the quasi-reversible nature of the electrochemical reactions. It should be noted that values of ΔE larger than those of reversible one-electron transitions (0.059 V) are observed for many cluster complexes, which may be associated with a slow change in the geometry of the compound as compared to the rate of the electrochemical reaction.
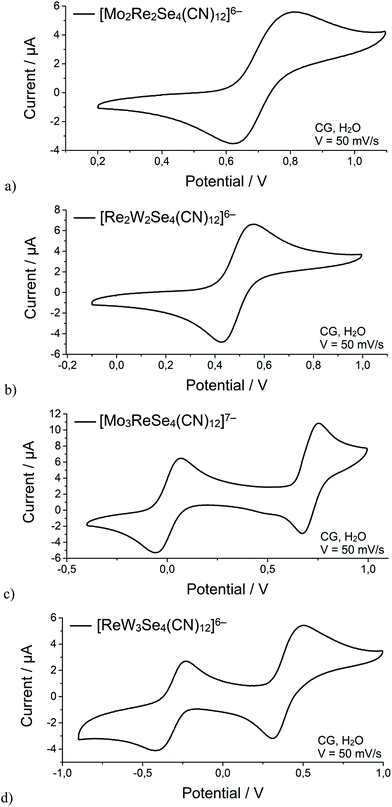 |
| Fig. 3 Cyclic voltammogram curves for aqueous solutions of 1 (a), 2 (b), 3 (c) and 4 (d). Potentials are measured vs. the Ag/AgCl reference electrode. | |
For complexes with a metal ratio in the cluster core of 3
:
1, a different behavior was found (Fig. 3c and d). Thus, the voltammogram of the [Mo3ReSe4(CN)12]7− cluster shows two electrochemical processes with E1/2 = 0.005 V (ΔE = 0.125 V) and E1/2 = 0.713 V (ΔE = 0.081 V). The voltammogram of the [ReW3Se4(CN)12]6− complex also shows two quasi-reversible electrochemical waves with E1/2 = – 0.324 V (ΔE = 0.151 V) and E1/2 = 0.396 V (ΔE = 0.155 V). In both cases, the first wave can be attributed to the one-electron process [M3ReSe4(CN)12]7− (12 CSE) ↔ [M3ReSe4(CN)12]6− (11 CSE). The second wave corresponds to the process [M3ReSe4(CN)12]6− (11 CSE) ↔ [M3ReSe4(CN)12]5− (10 CSE). Therefore, an increase of the Mo or W content in the cluster core stabilizes more electron-deficient states in aqueous solutions.
Summarizing the obtained data (Table 2), we can conclude that the ratio of metals in heterometallic clusters [MxRe4−xSe4(CN)12]n− completely determines their electrochemical activity. With a decrease in the content of rhenium, the potentials of the 12 to 11 CSE transitions sharply shift to the negative potential region, and the value of this shift reaches −0.8 V per Mo(W) atom when moving from the {M2Re2} to {M3Re} cores. This leads, in particular, to the oxidation of the [ReW3Se4(CN)12]7− cluster by the atmospheric oxygen in aqueous solution and to the stabilization of the [ReW3Se4(CN)12]6− form (11 CSE).
Table 2 Redox potentials (E1/2, V) for 10 to 11 CSE and 11 to 12 CSE transitions for heterometallic clusters 1–4 and known homometallic clusters with {M4Se4}n+ cores. For the ease of comparison, all potentials are given vs. NHE
Cluster |
E
1/2 (11 to 12 CSE) |
E
1/2 (10 to 11 CSE) |
Ref. |
[Mo2Re2Se4(CN)12]n− |
0.922 |
n/a |
This work |
[Mo3ReSe4(CN)12]n− |
0.210 |
0.918 |
This work |
[Mo4Se4(CN)12]n− |
–0.422 |
0.233 |
26
|
[Re2W2Se4(CN)12]n− |
0.696 |
n/a |
This work |
[ReW3Se4(CN)12]n− |
–0.119 |
0.601 |
This work |
[W4Se4(CN)12]n− |
–0.347 |
0.694 |
5a
|
The observed trends are in full agreement with the literature data. Homometallic tetrahedral chalcogenide cluster complexes of molybdenum and tungsten have two reversible one-electron oxidative transitions from the state with 12 CSE to the states with 11 and 10 CSE.4,5 At the same time, homometallic clusters of rhenium [Re4Q4(CN)12]4− do not undergo redox transformations in aqueous solutions. It has been shown that as the content of tungsten in heterometallic clusters [MoxW4−xQ4(H2O)12]n+ increases, the potentials of both processes shift to the region of negative values.6 However, the value of this shift per one heteroatom is much smaller (of the order of 0.15–0.30 V), which may be a consequence of the same number of valence electrons of Mo and W atoms and, hence, the same charge of clusters with different Mo/W ratios and the same number of CSE. A systematic change in the number and position of electrochemical waves is characteristic of other heterometallic clusters with the same set of inner and apical ligands.1a,16a,20
Crystal structures
The {MxRe4−xSe4} (M = Mo, W; x = 2, 3) cluster core in all the obtained heterometallic complexes has a typical structural characteristic of tetrahedral chalcogenide clusters of the {M4Q4} type (Fig. 4). Rhenium and molybdenum or tungsten atoms in all structures are disordered occupying the same positions and form a tetrahedral metal core {MxRe4−x}. Chalcogen atoms are coordinated to each face of the tetrahedron in the μ3 mode. Each of the metal atoms is additionally coordinated by three apical cyanide ligands.
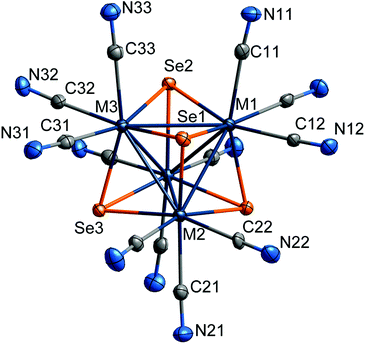 |
| Fig. 4 Structure of the [{Mo3ReSe4}(CN)12]7− anion in 2 with the numbering of atoms of the asymmetric fragment. | |
The isostructural compounds K6[{Mo2Re2Se4}(CN)12]·10H2O (1) and K6[{Re2W2Se4}(CN)12]·10H2O (3) crystallize in the trigonal crystal system (P3 space group). The asymmetric units contain two and three thirds of cluster anions [{M2Re2Se4}(CN)12]6−, 18 positions of K+ cations and 30 positions of solvate H2O molecules. Free refinement of the occupancy of metal positions in the structure led to the ratio of rhenium and molybdenum atoms slightly differing from 2
:
2; we applied restraints to maintain the correct ratio.
The compound K7[{Mo3ReSe4}(CN)12]·8H2O·2MeOH (2) crystallizes in the orthorhombic crystal system (Pnma space group). The asymmetric unit contains half of the cluster anions, five K+ positions, four of which are fully occupied and one is half occupied due to the proximity to the inversion center, four positions of solvate water molecules and one methanol molecule. The occupancy of metal positions in the cluster core was refined as 0.83/0.17 (Mo1/Re1), 0.75/0.25 (Mo2/Re2) and 0.71/0.29 (Mo3/Re3).
The compound Cs6[{ReW3Se4}(CN)12]·5.2H2O·MeOH (4) crystallizes in the monoclinic system (P21/n space group). The asymmetric unit contains all atoms of the [{ReW3Se4}(CN)12]6− cluster anion, ten Cs+ cations, six solvate H2O molecules, and one methanol molecule. Two Cs+ cations exhibit high disorder over at least three positions.
Let us consider how the substitution of metals in the cluster core affects its geometry. The main bond lengths in the new anions are given in Table 3. For comparison, the distances are also indicated for the currently known 12-electron cluster complexes – K4[{Re4Se4}(CN)12]25a and K7Na[Mo4Se4(CN}12].26 Note that twelve-electron tetrahedral complexes have not been described for tungsten, and the overwhelming majority of the known compounds are electron-deficient and contain 10 CSE; therefore, only the distances for molybdenum and rhenium complexes are given in the table.
Table 3 Comparison of M–M and M–Se bond lengths for heterometallic cluster anions in the structures of 1–4 with those for homometallic clusters [{Re4Se4}(CN)12]4− and [Mo4Se4(CN}12]8−. Range of bond lengths and their mean value are listed
Compound |
M–M |
M–Se |
K4[{Re4Se4}(CN)12]25a |
2.7876(3)–2.8048(3) |
2.4521(5)–2.4877(6) |
2.796(6) |
2.462(8) |
K6[{Mo2Re2Se4}(CN)12] |
2.8341(2)–2.8642(2) |
2.4742(3)–2.4964(3) |
2.851(8) |
2.485(5) |
K6[{Re2W2Se4}(CN)12] |
2.8331(9)–2.8590(9) |
2.4809(18)–2.4937(18) |
2.849(7) |
2.488(4) |
K7[{Mo3ReSe4}(CN)12] |
2.8716(7)–2.8944(5) |
2.4862(6)–2.5056(9) |
2.886(10) |
2.494(5) |
Cs6[{ReW3Se4}(CN)12] |
2.8515(3)–2.8911(3) |
2.4864(7)–2.5025(7) |
2.869(13) |
2.494(6) |
K7Na[Mo4Se4(CN}12]26 |
2.9004(4)–2.9255(4) |
2.4928(5)–2.5218(5) |
2.910(8) |
2.507(9) |
The table shows that the bond lengths in the molybdenum selenide complex are slightly longer than those in the rhenium one (M–M by ∼0.1 Å, M–Se by ∼0.05 Å). Thus, it can be assumed that with a decrease in the rhenium content, the cluster core will “expand”. Indeed, on going from {Re4} to {M2Re2} (M = Mo, W) the metal-to-metal distance increases by ∼0.05 Å for both complexes. At the same time, when moving from {M2Re2} to {M3Re} the M–M mean bond lengths change from 2.851(8) Å to 2.886(10) Å for the rhenium–molybdenum complex and from 2.849(7) Å to 2.869(13) Å for the rhenium–tungsten one (Fig. S8†). The Se–M bond distances in the heterometallic clusters are intermediate between the values for their homometallic analogues.
Magnetic properties
Among the studied heterometallic compounds the only one exhibiting paramagnetic behavior over the entire temperature range of 1.77–300 K is Cs6[{ReW3Se4}(CN)12]·5.2H2O·MeOH (4) (Fig. 5a). In the temperature range of 50–300 K, its magnetic susceptibility χp(T) roughly follows the Curie–Weiss law with μeff ≈ 1.88μB per formula unit and θ ≈ −8 K (Fig. 5b). The obtained value of an effective magnetic moment is quite close to the theoretical spin-only value of 1.73μB for one unpaired electron (S = 1/2), which was naturally expected for the [{ReW3Se4}(CN)12]6− anion possessing an odd number of cluster skeletal electrons. Given the absence of any sign of antiferromagnetic ordering down to T = 1.77 K, the Weiss constant θ ≈ −8 K formally evaluated at high temperatures should not be taken as an indication of substantial antiferromagnetic exchange interactions between the clusters. Indeed, θ turns out to be dependent on the temperature interval selected for Curie–Weiss fitting and decreases dramatically at low T approaching θ ≈ −0.35–0.4 K. The latter value implies a detectable, but very weak, antiferromagnetic exchange interaction between adjacent tetranuclear metal clusters. This weak interaction manifests itself below T ∼ 10 K as a sharp drop of the effective moment μeff calculated for θ = 0 (Fig. 5b). In turn, the gradual temperature dependence of μeff above 10 K reflects the variation of the actual magnetic moment of the cluster.
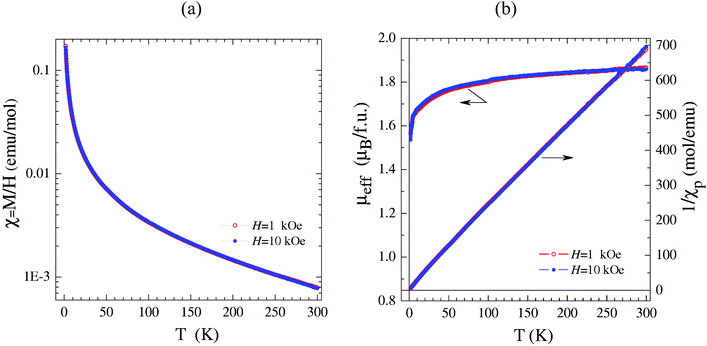 |
| Fig. 5 Magnetic properties of the Cs6[{ReW3Se4}(CN)12]·5.2H2O·MeOH (4) cluster complex. (a) Temperature dependencies of the magnetic susceptibility χ measured at H = 1 kOe (o) and 10 kOe (•). (b) Temperature dependencies of 1/χp and μeff; the latter is calculated for the case of non-interacting magnetic moments (θ = 0). | |
The field dependences of magnetization M(H) agree well with the presence of one unpaired electron per formula unit and the weak antiferromagnetic interaction between the magnetic moments of clusters.
Conclusion
It has been shown that the use of ReI3 and MoO3/WO3 as precursors makes it possible to obtain heterometallic tetrahedral cluster complexes with the general formula [MxRe4−xSe4(CN)12]n− (M = Mo, W; x = 2, 3). Compounds [ReW3Se4(CN)12]6− and [Re2W2Se4(CN)12]6− are the first rhenium–tungsten heterometallic clusters. A one-step process for the preparation of these compounds occurs by the reaction of precursors with KCN at relatively low temperatures. An important finding is the possibility of obtaining compounds with different ratios of metals in the cluster core by varying the stoichiometry of the reaction mixture, which was shown using mass-spectrometric analysis of the reaction products. The geometry of cluster anions remains almost unchanged with varying metal core composition. At the same time, the voltammetric study of anions in an aqueous solution showed a dramatic anodic shift of redox potentials of 12 CVE to 11 CVE transitions with a decrease in the rhenium content in the metal center. Finally, it has been shown that the preparation of heterometallic clusters is an effective way to change the properties of cyanide tetranuclear cluster compounds preserving their geometry and solubility.
Conflicts of interest
There are no conflicts to declare.
Acknowledgements
The reported study was funded by the RFBR (project No. 20-33-90086) and by the Russian Science Foundation (project No. 18-13-00058). The work of NIIC SB RAS team was also supported by the Ministry of Science and Higher Education of the Russian Federation.
References
-
(a) R. Hernandez-Molina and A. G. Sykes, Chalcogenide-bridged cuboidal clusters with M4Q4 (M=Mo, W; Q=S, Se, Te cores, J. Chem. Soc., Dalton Trans., 1999, 3137–3148 RSC;
(b) M. N. Sokolov and V. P. Fedin, Chalcogenide clusters of vanadium, niobium and tantalum, Coord. Chem. Rev., 2004, 248, 925–944 CrossRef CAS;
(c) A. L. Gushchin, Y. A. Laricheva, M. N. Sokolov and R. Llusar, Tri- and tetranuclear molybdenum and tungsten chalcogenide clusters: on the way to new materials and catalysts, Russ. Chem. Rev., 2018, 87, 670–706 CrossRef CAS.
-
(a) R. Pocha, D. Johrendt and R. Pottgen, Electronic and structural instabilities in GaV4S8 and GaMo4S8, Chem. Mater., 2000, 12, 2882–2887 CrossRef CAS;
(b) H. Barz, New ferromagnetic molybdenum spinels, Mater. Res. Bull., 1973, 8, 983–988 CrossRef CAS;
(c) K. Routledge, P. Vir, N. Cook, P. A. E. Murgatroyd, S. J. Ahmed, S. N. Savvin, J. B. Claridge and J. Alaria, Mode Crystallography Analysis through the Structural Phase Transition and Magnetic Critical Behavior of the Lacunar Spinel GaMo4Se8, Chem. Mater., 2021, 33, 5718–5729 CrossRef CAS PubMed.
-
(a) F. A. Cotton, M. P. Diebold, Z. Dori, R. Llusar and W. Schwotzer, The Cuboidal Mo4S46+ Aquo Ion and Its Derivatives, J. Am. Chem. Soc., 1985, 107, 6735–6736 CrossRef CAS;
(b) G. Henkel, G. Kampmann, B. Krebs, G. J. Lamprecht, M. Nasreldin and A. G. Sykes, Preparation Structure, and Properties of the Mo/Se Ions [Mo2O2(μ2-Se)2(H2O)6]2+ and [Mo4(μ3-Se)4(H2O)12]5+, J. Chem. Soc., Chem. Commun., 1990, 1014–1016 RSC;
(c) T. Shibahara, H. Kuroya, H. Akashi, K. Matsumoto and S. Ooi, Syntheses and characterization of cubane-type clusters, [Mo4S4(edta)2]n− (n=2–4), [Mo4S4(H2O)12]n+ (n=4–6) and [Mo4S4(NH3)12]4+. X-ray structures of Na2[Mo4S4(edta)2]·6H2O, Ca1.5[Mo4S4(edta)2]·13H2O, Mg2[Mo4S4(edta)2]·20H2O, [Mo4S4(H2O)12](CH3C6H4SO3)5·14H2O and [Mo4S4(NH3)12]Cl4·7H2O, Inorg. Chim. Acta, 1993, 212, 251–263 CrossRef CAS.
- M. N. Sokolov, D. N. Dybtsev, A. V. Virovets, V. P. Fedin, P. Esparza, R. Hernandez-Molina, D. Fenske and A. G. Sykes, Preparation and properties of the aqua ions [W4S4(H2O)12]n+ (n=5, 6) and crystal structure of (Me2NH2)6[W4S4(NCS)12]·0.5H2O, Inorg. Chem., 2002, 41, 1136–1139 CrossRef CAS PubMed.
-
(a) V. P. Fedin, I. V. Kalinina, D. G. Samsonenko, Y. V. Mironov, M. N. Sokolov, S. V. Tkachev, A. V. Virovets, N. V. Podberezskaya, M. R. J. Elsegood, W. Clegg and A. G. Sykes, Synthesis structure, and properties of molybdenum and tungsten cyano complexes with cuboidal M4(μ3-E)4 (M=Mo, W; E=S, Se, Te cores, Inorg. Chem., 1999, 38, 1956–1965 CrossRef CAS PubMed;
(b) V. P. Fedin, D. G. Samsonenko, A. V. Virovets, I. V. Kalinina and D. Y. Naumov, Synthesis structures, and properties of molybdenum and tungsten chalcogenide cubane complexes (NH4)6[M4Q4(CN)12]·6H2O (M=Mo or W; Q=S or Se), Russ. Chem. Bull., 2000, 49, 19–25 CrossRef CAS.
-
(a) I. J. McLean, R. Hernandez-Molina, M. N. Sokolov, M. S. Seo, A. V. Virovets, M. R. J. Elsegood, W. Clegg and A. G. Sykes, Preparation structure and properties of three [MoxW4-xS4(H2O)12]5+ (x = 1-3) and [MoW3Se4(H2O)12]5+ cuboidal complexes alongside [Mo4S4(H2O)12]5+ and [Mo4Se4(H2O)12]5+, J. Chem. Soc., Dalton Trans., 1998, 15, 2557–2562 RSC;
(b) M. Sokolov, P. Esparza, R. Hernandez-Molina, J. G. Platas, A. Mederos, J. A. Gavin, R. Llusar and C. Vicent, Preparation and properties of the full series of cuboidal clusters [MoxW4-xSe4(H2O)12]n+ (n=4–6) and their derivatives, Inorg. Chem., 2005, 44, 1132–1141 CrossRef CAS.
- G. F. Khudorozhko, E. A. Kravtsova, L. N. Mazalov, V. E. Fedorov, L. G. Bulusheva, I. P. Asanov, G. K. Parygina and Y. V. Mironov, X-ray emission and X-ray photoelectron study of the electronic structure of polymeric cubanocluster compounds Re4-xMoxS4Te4, J. Struct. Chem., 1996, 37, 767–772 CrossRef.
- C. Perrin, R. Chevrel and M. Sergent, New Molybdenum Ruthenium Thio Compounds with Mixed Tetrahedral Cluster, J. Solid State Chem., 1976, 19, 305–308 CrossRef CAS.
- L. Malatesta, Rhenium(III) Iodide, Inorg. Synth., 1963, 7, 185–189 CAS.
-
Bruker Apex3 software suite: Apex3, SADABS-2016/2 and SAINT, version 2018.7-2, Bruker AXS Inc., Madison, WI, 2017, In Search PubMed.
- G. M. Sheldrick, SHELXT - Integrated space-group and crystal-structure determination, Acta Crystallogr., Sect. A: Found. Adv., 2015, 71, 3–8 CrossRef PubMed.
- G. M. Sheldrick, Crystal structure refinement with SHELXL, Acta Crystallogr., Sect. C: Struct. Chem., 2015, 71, 3–8 CrossRef.
- O. V. Dolomanov, L. J. Bourhis, R. J. Gildea, J. A. K. Howard and H. Puschmann, OLEX2: a complete structure solution, refinement and analysis program, J. Appl. Crystallogr., 2009, 42, 339–341 CrossRef CAS.
-
(a) G. Xu, Z. Wang, R. Ling, J. Zhou, X. D. Chen and R. H. Holm, Ligand metathesis as rational strategy for the synthesis of cubane-type heteroleptic iron-sulfur clusters relevant to the FeMo cofactor, Proc. Natl. Acad. Sci. U. S. A., 2018, 115, 5089–5092 CrossRef CAS PubMed;
(b) B. Zheng, X. D. Chen, S. L. Zheng and R. H. Holm, Selenium as a Structural Surrogate of Sulfur: Template-Assisted Assembly of Five Types of Tungsten-Iron-Sulfur/Selenium Clusters and the Structural Fate of Chalcogenide Reactants, J. Am. Chem. Soc., 2012, 134, 6479–6490 CrossRef CAS;
(c) C.-H. Wang and S. DeBeer, Structure reactivity, and spectroscopy of nitrogenase-related synthetic and biological clusters, Chem. Soc. Rev., 2021, 50, 8743–8761 RSC.
-
(a) R. Llusar and S. Uriel, Heterodimetallic chalcogen-bridged cubane-type clusters of molybdenum and tungsten containing first-row transition metals, Eur. J. Inorg. Chem., 2003, 1271–1290 CrossRef CAS;
(b) Y. Ohki, K. Uchida, R. Hara, M. Kachi, M. Fujisawa, M. Tada, Y. Sakai and W. M. C. Sameera, Cubane-Type [Mo3S4M] Clusters with First-Row Groups 4–10 Transition-Metal Halides Supported by C5Me5 Ligands on Molybdenum, Chem. – Eur. J., 2018, 24, 17138–17147 CrossRef CAS.
-
(a) E. G. Tulsky and J. R. Long, Heterometal substitution in the dimensional reduction of cluster frameworks: Synthesis of soluble [Re6-nOsnSe8Cl6](4-n)- (n=1–3) cluster-containing solids, Inorg. Chem., 2001, 40, 6990–7002 CrossRef CAS;
(b) E. G. Tulsky, N. R. M. Crawford, S. A. Baudron, P. Batail and J. R. Long, Cluster-to-metal magnetic coupling: Synthesis and characterization of 25-electron [Re6-nOsnSe8(CN)6](5-n)- (n=1, 2) clusters and {Re6-nOsnSe8[CNCu(Me6tren)]6}9+ (n=0, 1, 2) assemblies, J. Am. Chem. Soc., 2003, 125, 15543–15553 CrossRef CAS.
- S. B. Artemkina, N. G. Naumov, K. N. Kondrakov, A. V. Virovets, S. G. Kozlova and V. E. Fedorov, Cluster Complexes with the Novel Heterometallic Cluster Core {Mo5NbI8}: Synthesis, Excision Reactions, and Crystal Structures, Z. Anorg. Allg. Chem., 2010, 636, 483–491 CrossRef CAS.
-
(a) N. G. Naumov, K. A. Brylev, Y. V. Mironov, A. V. Virovets, D. Fenske and V. E. Fedorov, Synthesis and structures of new octahedral water-soluble heterometal rhenium-molybdenum clusters, Polyhedron, 2004, 23, 599–603 CrossRef CAS;
(b) Y. M. Gayfulin, N. G. Naumov, M. R. Rizhikov, A. I. Smolentsev, V. A. Nadolinny and Y. V. Mironov, Heterometallic clusters with a new {Re3Mo3S8} core: direct synthesis, properties and DFT calculations, Chem. Commun., 2013, 49, 10019–10021 RSC;
(c) V. K. Muravieva, Y. M. Gayfulin, M. R. Ryzhikov, I. N. Novozhilov, D. G. Samsonenko, D. A. Piryazev, V. V. Yanshole and N. G. Naumov, Mixed-metal clusters with a {Re3Mo3Se8}core: from a polymeric solid to soluble species with multiple redox transitions, Dalton Trans., 2018, 47, 3366–3377 RSC.
- N. G. Naumov, E. V. Ostanina, A. V. Virovets, M. Schmidtman, A. Muller and V. E. Fedorov, 23-Electron Re6 metal clusters: Syntheses and crystal structures of (Ph4P)3[Re6S8(CN)6], (Ph4P)2(H)[Re6Se8(CN)6]·8H2O, and (Et4N)2(H)[Re6Te8(CN)6]·2H2O, Russ. Chem. Bull., 2002, 51, 866–871 CrossRef CAS.
-
(a) V. K. Muravieva, Y. M. Gayfulin, C. Prestipino, P. Lemoine, M. R. Ryzhikov, V. V. Yanshole, S. Cordier and N. G. Naumov, Tailoring Heterometallic Cluster Functional Building Blocks: Synthesis, Separation, Structural and DFT Studies of [Re6-xMoxSe8(CN)6]n−, Chem. – Eur. J., 2019, 25, 15040–15045 CrossRef CAS;
(b) V. K. Muravieva, I. P. Loginov, T. S. Sukhikh, M. R. Ryzhikov, V. V. Yanshole, V. A. Nadolinny, V. Dorcet, S. Cordier and N. G. Naumov, Synthesis Structure, and Spectroscopic Study of Redox-Active Heterometallic Cluster-Based Complexes [Re5MoSe8(CN)6]n, Inorg. Chem., 2021, 60, 8838–8850 CrossRef CAS.
- V. K. Muravieva, Y. M. Gayfulin, T. I. Lappi, V. Dorcet, T. S. Sukhikh, P. Lemoine, M. R. Ryzhikov, Y. V. Mironov, S. Cordier and N. G. Naumov, Apical Cyanide Ligand Substitution in Heterometallic Clusters [Re3Mo3Q8(CN)6]n− (Q=S, Se), Eur. J. Inorg. Chem., 2019, 2019, 2685–2690 CrossRef CAS.
-
(a) A. S. Pronin, A. I. Smolentsev, S. G. Kozlova, I. N. Novozhilov and Y. V. Mironov, PO23– and AsO3–: New Pnictogenide Ligands in the Highly Charged Re4 Cluster Anions [{Re4(PO)3(PO2)}(CN)12]8-, [{Re4As2(AsO)2}(CN)12]8- and [{Re4(AsO)4}(CN)12]8-, Inorg. Chem., 2019, 58, 7368–7373 CrossRef CAS;
(b) A. S. Pronin, Y. M. Gayfulin, A. I. Smolentsev and Y. V. Mironov, Tetrahedral Rhenium Cluster Complexes with Mixed-Ligand Cores {Re4As3Q}5+ (Q=S, Se) and {Re4As2S2}6+, J. Cluster Sci., 2019, 30, 1253–1257 CrossRef CAS;
(c) A. S. Pronin, Y. M. Gayfulin, A. I. Smolentsev, S. G. Kozlova, V. V. Yanshole and Y. V. Mironov, The {Re4} Tetrahedral Cyanometalate Cluster Anion [{Re4(μ3-CCN)4}(CN)12]8- with Inner (μ3-CCN)3- Ligands and Its Features in Coordination of Cu2+ Cations, Inorg. Chem., 2020, 59, 9710–9717 CrossRef CAS PubMed;
(d) A. S. Pronin, A. I. Smolentsev and Y. V. Mironov, Inorganic Ligands Sb3- and Bi3-: Synthesis and Crystal Structures of Complexes with Mixed-Ligand Cluster Cores {Re4Se3Sb}7+ and {Re4Se3Bi}7+, Inorg. Chem., 2021, 60, 4371–4374 CrossRef CAS.
- A. Muller, R. Jostes, W. Eltzner, C. S. Nie, E. Diemann, H. Bogge, M. Zimmermann, M. Dartmann, U. Reinschvogell, S. Che, S. J. Cyvin and B. N. Cyvin, Synthetic Spectroscopic, X-Ray Structural, and Quantum-Chemical Studies of Cyanothiomolybdates with Mo2S, Mo2S2, Mo3S4, and Mo4S4 Cores - a Remarkable Class of Species Existing with Different Electron Populations and Having the Same Central Units as the Ferredoxins, Inorg. Chem., 1985, 24, 2872–2884 CrossRef.
- A. Golebiewski and H. Kowalski, Electronic Structure of Octacyanides of Molybdenum IV and V According to the SCCC MO Method, Theor. Chim. Acta, 1968, 12, 293–306 CrossRef.
-
(a) Y. V. Mironov, A. V. Virovets, W. S. Sheldrick and V. E. Fedorov, Novel inorganic polymeric compounds based on the Re4 chalcocyanide cluster complexes: synthesis and crystal structures of Mn2[Re4Se4(CN)12]·6H2O, Cd2[Re4Te4(CN)12]·6H2O, Cu2[Re4Te4(CN)12]·4H2O and K4Re4Se4(CN)12·6H2O, Polyhedron, 2001, 20, 969–974 CrossRef CAS;
(b) V. P. Fedin, M. R. J. Elsegood, W. Clegg and A. G. Sykes, High-yield synthesis of the cuboidal rhenium cluster [Re4S4(CN)12]4- by reaction of the triangular cluster [Re3S7Br6]+ with cyanide, Polyhedron, 1996, 15, 485–488 CrossRef CAS.
- C. Magliocchi, X. B. Xie and T. Hughbanks, Cyanide-melt synthesis of reduced molybdenum selenide clusters, Inorg. Chem., 2004, 43, 1902–1911 CrossRef CAS PubMed.
Footnote |
† Electronic supplementary information (ESI) available. CCDC 2111041–2111044. For ESI and crystallographic data in CIF or other electronic format see DOI: 10.1039/d1qi01230d |
|
This journal is © the Partner Organisations 2022 |
Click here to see how this site uses Cookies. View our privacy policy here.