DOI:
10.1039/D2QI00086E
(Research Article)
Inorg. Chem. Front., 2022,
9, 1847-1855
Phosphorus doped two-dimensional CoFe2O4 nanobelts decorated with Ru nanoclusters and Co–Fe hydroxide as efficient electrocatalysts toward hydrogen generation†
Received
11th January 2022
, Accepted 5th March 2022
First published on 7th March 2022
Abstract
Developing efficient and durable hydrogen evolution reaction (HER) electrocatalysts has attracted considerable attention for large-scale hydrogen generation. In this work, phosphorus doped two-dimensional (2D) CoFe2O4 nanobelts decorated with Ru and CoFe hydroxide clusters on iron foam (IF) (CoFeOxHy–Ru/P–CoFe2O4/IF) are synthesized via a solvothermal method followed by phosphorization procedures. The specific nanobelt morphology favors the exposure of active sites, advantageous electron transportation and intimate contact between the electrolyte and electrocatalyst. Interestingly, abundant metal hydroxide nanosheets are in situ generated on the nanobelt after the phosphorized sample is immersed in an alkaline electrolyte. Benefiting from the above merits, the developed CoFeOxHy–Ru/P–CoFe2O4/IF presents excellent electrocatalytic performances for the HER with low overpotentials of 38.6 mV, 43.2 mV, and 30.1 mV to attain 50 mA cm−2 and 54.4 mV, 71.5 mV, and 69.3 mV to attain 100 mA cm−2 in 1 M KOH, 1 M KOH seawater and 1 M PBS, respectively. As for overall water splitting, a cell voltage as low as 1.49 V is necessary to attain 10 mA cm−2 and the developed CoFeOxHy–Ru/P–CoFe2O4/IF also exhibits excellent long-term stability. This work provides a new avenue for designing efficient HER catalysts with high exposure of precious metals.
1. Introduction
It is of importance to exploit sustainable and green energies to alleviate the ever-increasing environmental contamination and energy crisis.1–4 Among them, hydrogen, a high energy density and eco-friendly energy carrier, is identified as a promising energy storage medium to solve the issues of intermittent sustainable energies, such as solar, wind and ocean energies.5–9 Electrocatalytic overall water-splitting has been considered the most eco-friendly and desired means to produce hydrogen without the generation of pollutant gases in comparison with the traditional synthetic technologies.10–13 Therefore, it is crucial to utilize catalysts to expedite the HER to achieve high electrocatalytic performance.14–19 So far, Pt-based nanomaterials are deemed to be the most efficient electrocatalysts for the HER with the most favorable reaction kinetics.20,21 Nevertheless, their scarcity and high cost limited their further large-scale implementation for hydrogen generation.22–24 Thus, tremendous efforts have been devoted to settle the challenges of exploring efficient and Pt-free catalysts for the HER.25–28
More recently, Ru-based electrocatalysts have been investigated extensively to substitute Pt for the HER due to their low cost, excellent electrocatalytic performance and remarkable stability.29,30 For example, Liu et al. reported phosphatized Ru–Fe bimetallic nanoclusters (RuxFeyP-NCs) dispersed on graphitized carbon nanofiber (CNF) supports with low overpotentials of 65.8 and 16.0 mV at 10 mA cm−2 in acidic and alkaline media with an excellent stability of 100 h for the HER.31 In general, a high content of Ru is necessary to drive the excellent electrocatalytic performance which then increases the cost of hydrogen energy. It is widely recognized that decreasing the particle size is a valid strategy to improve the electrocatalytic activity by exposing abundant active sites.32–34 Moreover, the morphology of the designed electrocatalysts also plays a pivotal role in regulating the electrocatalytic performance.35,36 Among the investigated nanostructures, the developed nanobelts possess particular merits, such as intimate contact between the catalyst and electrolyte, favorable electron and electrolyte transportation and the high exposure of active sites.37 For instance, Zeng et al. synthesized silver doped cobalt selenide (Ag–CoSe2) via a partial cation exchange strategy and the obtained electrocatalyst presented excellent electrocatalytic activity toward the OER.38 Except the above-mentioned nanostructure regulation, heteroatom doping is also considered as a promising avenue to promote the electrocatalytic activity. Recently, Yu and co-authors demonstrated that the introduced N atom plays a significant role in improving the Ni–Mo based sulfide for the HER with small overpotentials of 68, 250, and 322 mV to attain 10, 500 and 1000 mA cm−2, respectively.39 Then, the combination of morphology regulation and heteroatom doping would be an attractive approach to optimize the electrocatalytic activity.
In this work, we designed P doped CoFe2O4 nanobelts decorated with Ru nanoclusters and Co–Fe hydroxides through hydrothermal and annealing treatment, followed by hydrolysis in an aqueous solution of potassium hydroxide (Scheme 1). The obtained specific nanobelt structure favors the exposure of active sites, provides rich catalytic sites and facilitates mass transport during the electrocatalytic process. The Ru species was introduced by dripping onto the substrate after the solvothermal process and then it can contact the electrolyte directly. Moreover, the Co–Fe hydroxide species were in situ generated on the nanobelts and played a significant role in promoting the electrocatalytic performance by tuning the adsorption/desorption of the intermediates. Owing to the above merits, the as-developed CoFeOxHy–Ru/P–CoFe2O4/IF presented remarkable electrocatalytic activities and long-term stability in various electrolytes.
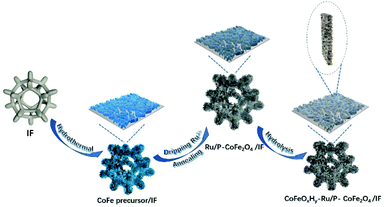 |
| Scheme 1 Schematic illustration of the synthesis of CoFeOxHy–Ru/P–CoFe2O4/IF. | |
2. Experimental section
2.1. Synthesis of Ru/P–CoFe2O4/IF
The IF (2.0 cm × 1.0 cm) was added into anhydrous ethanol, acetone, and anhydrous ethanol for 30 min under ultrasonic treatment to clean the surface impurities and then placed in a vacuum oven to dry overnight. After that, Co(NO3)2·6H2O (0.3 g) and Fe(NO3)2·9H2O (0.08 g) were dissolved in 15 mL H2O under stirring to obtain a homogeneous solution. Then the solution was transferred into a 100 mL Teflon-lined stainless-steel autoclave with a piece of IF and heated at 120 °C for 7 h. After that, the black product was taken out and washed with absolute ethanol and deionized water to remove the unreacted materials. The standby solution was obtained by dissolving ruthenium chloride in anhydrous ethanol (0.116 M) and then dropping it onto the solvothermal product. Finally, 0.5 g of sodium hypophosphite and the reactant were placed upstream and downstream of a tube furnace for 2 h at 350 °C with a heating rate of 5 °C min−1.
2.2. Synthesis of CoFeOxHy–Ru/P–CoFe2O4/IF
The synthesized precursor of Ru/P–CoFe2O4/IF was immersed in 1.0 M KOH for 3 h under constant stirring. The resulting electrode was denoted as CoFeOxHy–Ru/P–CoFe2O4/IF which could directly serve as the working electrode for subsequent electrochemical measurements. For comparison, CoFeOxHy/P–CoFe2O4 was prepared without ruthenium chloride addition. Other samples prepared with different amounts (50 mg and 100 mg) of Fe(NO3)2·9H2O were named 50 mg–Fe(NO3)2·9H2O and 100 mg–Fe(NO3)2·9H2O, respectively.
2.3. Synthesis of RuO2/CoFe2O4
RuO2/CoFe2O4 was obtained using a similar synthetic procedure to that of CoFeOxHy–Ru/P–CoFe2O4/IF, except pyrolysis in a muffle furnace at 380 °C for 2 h.
2.4. Physical characterization
The morphologies of the as-designed electrocatalysts were obtained by scanning electron microscopy (SEM) (Hitachi S-4800) and transmission electron microscopy (TEM) (FEI Tecni G20, 200 kV) analysis. Energy-dispersive X-ray spectroscopy (EDX) (FEI Tecni G20, 200 kV) was used to analyze the composition and distribution of elements in the samples. The crystal information was obtained by X-ray diffraction (XRD) (Rigaku D/max-2500pc device with Cu Kα radiation (λ = 1.54 Å)). The information about valence states of samples was obtained by X-ray photoelectron spectroscopy (XPS) carried out in a Thermo Fisher Scientific II spectrometer with an Al Kα source (1486.6 eV). The contents of Fe, Co and P were measured by inductively coupled plasma emission spectroscopy (ICP-OES) using an Agilent 720 instrument.
2.5. Electrochemical characterization
Electrochemical tests were performed via an electrochemical workstation (Gamry Reference 3000) with a typical three-electrode setup in solution (1.0 M KOH, seawater (1 M KOH), and 1 M PBS) at room temperature. The electrochemical measurements were carried out with a saturated calomel electrode (SCE) and graphite rod which were used as the reference electrode and counter electrode, respectively. All final potentials were given by E(RHE) = E(SCE) + E°(SCE) + 0.059 pH (E°(SCE) + 0.243 V). Linear sweep voltammetry (LSV) curves for the HER were determined with a scanning rate of 1 mV s−1. EIS Nyquist plots for the obtained catalysts were obtained in a frequency range of 100 kHz–0.1 Hz at a potential of −1.15 V (vs. SCE) in 1 M KOH for the HER. Equivalent electrical circuit: Rs (R1): solution resistance, CPE: constant phase element, Rct (R2): charge transfer resistance. Multiple-step chronopotentiometry was used to measure the long-term stability of all samples.
3. Results and discussion
The crystal structure of the as-designed CoFeOxHy–Ru/P–CoFe2O4/IF is composed of CoFe2O4 (PDF#00-002-1045) without other impurities. The microstructure of the synthesized electrocatalysts was investigated by SEM and TEM. As shown in Fig. S1,† relative to the smooth surface of iron foam without specific decorated nanomaterials, smooth nanobelts were generated after the solvothermal reaction (Fig. S2 and S3†). Obviously, the nanobelt nanostructure was maintained well after decoration with Ru and the phosphorization process (Fig. 1b and Fig. S4†). Interestingly, the smooth surface of the nanobelt was covered with numerous nanosheets after immersion in 1 M KOH (Fig. 1c). In order to verify the species of the nanosheets, Raman spectra were recorded before and after immersion in 1 M KOH. Evidently, new peaks, ascribed to metal hydroxide,40 appeared after treatment with an alkaline solution, demonstrating the formation of Co–Fe hydroxide (Fig. S5†). On this basis, the electrocatalytic performances were recorded every 15 minutes (Fig. S6†). The catalytic activities improved with increasing soaking time and were well maintained after prolonged times, confirming that the generation of metal hydroxide plays a key role in regulating the catalytic performance. However, the smooth surface was retained well after soaking in deionized water, indicating that the hydroxide ion plays a pivotal role in forming metal hydroxide (Fig. S7†). The ICP-OES results (Table S1†) proved that the metal ions dissolved in the solution after soaking in 1 M KOH and then formed metal hydroxides. The nanosheets can be clearly observed by TEM and they are generated on the nanobelt homogeneously (Fig. 1d). As illustrated in Fig. 1e and f, Ru nanoclusters were decorated on the nanobelt homogenously with a lattice spacing of 0.206 nm of the (101) plane. Moreover, it can be observed in Fig. 1f that the interplanar spacings of 0.296 nm and 0.253 nm corresponded to the (200) and (311) facet of CoFe2O4, respectively. The elemental mappings confirmed that Co, Fe, P, O and Ru elements existed in the designed CoFeOxHy–Ru/P–CoFe2O4/IF (Fig. 1g and S8†). Moreover, for CoFeOxHy–Ru/P–CoFe2O4/IF, the BET surface area was 12.69 m2 g−1 (Fig. S9†) with pore size distribution centers at 3.4 and 8.9 nm. The result confirms the mesoporous structure of the prepared catalysts which favours mass transport and exposure of abundant active sites during the electrocatalytic process.
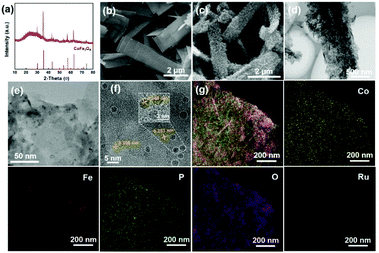 |
| Fig. 1 (a) XRD patterns of CoFeOxHy–Ru/P–CoFe2O4/IF. SEM images of Ru/P–CoFe2O4/IF (b) and CoFeOxHy–Ru/P–CoFe2O4/IF (c). (d and e) TEM images of CoFeOxHy–Ru/P–CoFe2O4/IF. (f) HRTEM image of CoFeOxHy–Ru/P–CoFe2O4/IF. (g) Corresponding element mappings of Co, Fe, P, O, and Ru in CoFeOxHy–Ru/P–CoFe2O4/IF. | |
XPS measurement was conducted to investigate the valence and composition of the as-prepared CoFeOxHy–Ru/P–CoFe2O4/IF. Obviously, P, O, Fe, Co, and Ru elements were detected from the XPS survey spectrum, which is in line with the EDS results. For high-resolution Co 2p (Fig. 2b), the peaks located at 782.3 eV and 780.7 eV are ascribed to Co2+ and Co3+, respectively.41–43 As illustrated in Fig. 2c, Fe2+ (710.5 eV) and Fe3+ (712.8 eV) are detected in the as-designed CoFeOxHy–Ru/P–CoFe2O4/IF.44,45 For Ru 3p, the peak at a binding energy of 484.3 eV is assigned to Ru 3p1/2 and the adjacent peak at 462.2 eV corresponds to Ru 3p3/2 in the high-resolution Ru 3p XPS spectrum which confirmed that Ru was successfully incorporated.31,46 The metal–oxide bond (531.6 eV, 530.8 eV and 529.7 eV) and the characteristic peaks of oxygen vacancies (530.8 eV) were obviously observed in the O 1s spectrum.44 The P 2p in Fig. 2f demonstrates that P was doped into the prepared electrocatalyst successfully.31,47–49 We investigated the XPS spectrum of Ru/P–CoFe2O4/IF and CoFeOxHy–Ru/P–CoFe2O4/IF to study the electronic interactions (Fig. S10†). For Co 2p, the peaks of CoFeOxHy–Ru/P–CoFe2O4/IF shift to a lower binding energy after the formation of CoFe–OH demonstrating that the electronic interactions play a pivotal role in promoting the electrocatalytic performance. Besides, different peaks of Ru 3p shifted to a higher binding energy of CoFeOxHy–Ru/P–CoFe2O4/IF compared to that of Ru/P–CoFe2O4/IF further indicating electron transfer in the catalyst. It has been widely recognized in some research studies that the introduction of hydroxide can effectively improve the electrocatalytic activity.50 Consequently, the formation of CoFeOxHy will act as an electronic regulator leading to the redistribution of electrons, and finally the exhibition of outstanding HER activity by the sample.
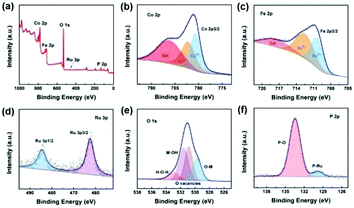 |
| Fig. 2 XPS survey spectrum (a) and high-resolution XPS spectra of Co 2p (b), Fe 2p (c), Ru 3p (d), O 1s (e) and P 2p (f) of the designed CoFeOxHy–Ru/P–CoFe2O4/IF. | |
The electrocatalytic HER performances of the as-designed CoFeOxHy–Ru/P–CoFe2O4/IF and reference samples were investigated in 1 M KOH via a typical three-electrode setup. Fig. 3a and b show the linear sweep voltammetry of CoFeOxHy–Ru/P–CoFe2O4/IF, CoFeOxHy–CoFe2O4/IF, Pt/C and IF. Remarkably, the designed CoFeOxHy–Ru/P–CoFe2O4/IF displays excellent HER activity with small overpotentials of 38.6 mV and 54.4 mV to attain 50 mA cm−2 and 100 mA cm−2, respectively, compared with CoFeOxHy/P–CoFe2O4/IF (156 mV@10 mA cm−2, 182 mV@100 mA cm−2), indicating that the introduced Ru nanoclusters play key roles in improving the electrocatalytic performance. Ru clusters anchored on oxides with high conductivity play a key role in electron traps, and rapidly occupy electron and reduce H+ to H2. The intrinsic activity of the catalyst is significantly increased due to the synergistic effects of electron coupling between Ru and CoFeOxHy.51 Besides, the Tafel plots demonstrated that CoFeOxHy–Ru/P–CoFe2O4/IF possesses a smaller value (43.2 mV dec−1) than CoFeOxHy–CoFe2O4/IF (85.6 mV dec−1), verifying its favorable reaction kinetics (Fig. 3c). The catalytic activity of the as-synthesized CoFeOxHy–Ru/P–CoFe2O4/IF surpasses most of the reported values (Fig. 3d and g and Tables S2 and S3†). As shown in Fig. S11,† CoFeOxHy–Ru/P–CoFe2O4/IF displays the smallest Nyquist semicircle diameter, demonstrating the lowest resistance of charge transfer during the electrocatalytic HER process.
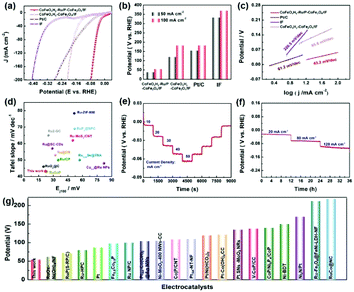 |
| Fig. 3 Electrochemical measurements: (a) HER polarization curves. (b) Overpotential comparison at 50 mA cm−2 and 100 mA cm−2. (c) Tafel slopes. (d) Comparison of the overpotentials and Tafel slopes with the references. (e and f) Stability tests of the obtained catalysts in 1 M KOH. (g) Comparison of the overpotentials (Ej100) with the reported electrocatalysts. | |
In addition, the prepared composite catalyst contains dense and tiny hydroxide nanosheets relative to Ru/P–CoFe2O4/IF, which plays a pivotal role in boosting the catalytic process, and the specific nanostructure benefits the transport of the electrolyte and weakens the O–H bond in water. Despite the remarkable electrocatalytic performance, the developed CoFeOxHy–Ru/P–CoFe2O4/IF also possesses excellent long-term stability. As illustrated in Fig. 3e and f, the current densities increased when the applied potentials were increased, and the current densities were well maintained during the reverse process, indicating its outstanding stability. For an optimal performance, we investigated the role of the mass content of Fe(NO3)2·9H2O in regulating the electrocatalytic activity for the HER (Fig. S12†). The results show that the electrocatalyst with 80 mg Fe(NO3)2·9H2O exhibits the lowest overpotential and the smallest Tafel slope.
As mentioned above, the developed CoFeOxHy–Ru/P–CoFe2O4/IF exhibits excellent HER performance coupled with long-term stability. Then, we investigated its properties after the stability test by SEM and XPS measurements. As shown in Fig. 4a and b, the CoFeOxHy–Ru/P–CoFe2O4/IF maintained the nanobelt morphology covered with numerous Co–Fe hydroxides, demonstrating its robust structure. The XPS results (Fig. 4c–f and Fig. S13†) verify the elemental composition and the chemical valence shows negligible changes, verifying its stable property. Thus, all the tests confirmed the great potential of CoFeOxHy–Ru/P–CoFe2O4/IF as an effective electrocatalyst for practical hydrogen production.
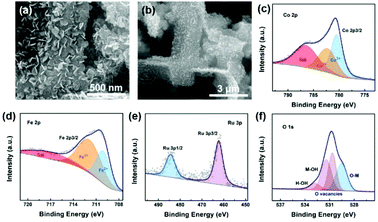 |
| Fig. 4 (a and b) SEM images and XPS spectra of Co (c), Fe (d), Ru (e) and P (f) of the CoFeOxHy–Ru/P–CoFe2O4/IF after the long-term stability test. | |
The ocean covers nearly 71% of the Earth's surface area and seawater accounts for about 97% of the total water on the Earth. Seawater is inexhaustible for human beings, so the reasonable development of electrolytic seawater is very promising for hydrogen production.52,53 As illustrated in Fig. 4a and b, the obtained electrocatalyst only needs low overpotentials of 43.2 mV and 71.5 mV to attain 50 mA cm−2 and 100 mA cm−2, respectively (Fig. 5a and b). Relatively, the reference sample CoFeOxHy–CoFe2O4/IF needs 162 mV and IF needs 377 mV to deliver 100 mA cm−2, respectively. Significantly, the synthesized CoFeOxHy–Ru/P–CoFe2O4/IF presented the lowest Tafel slope of 52.9 mV dec−1 compared with CoFeOxHy–CoFe2O4/IF (60.0 mV dec−1) and IF (134.1 mV dec−1), indicating its faster reaction kinetics for the HER (Fig. 5c). Fig. 5d shows the electrochemical impedance spectroscopy (EIS) results of the obtained catalysts in 1 M KOH seawater; the Rct value of CoFeOxHy–Ru/P–CoFe2O4/IF is 9.65 Ω, lower than those of CoFeOxHy–CoFe2O4/IF (15.31 Ω) and IF (135.6 Ω). In addition, we also studied the stability of CoFeOxHy–Ru/P–CoFe2O4/IF in 1 M KOH seawater using an identical method in an alkaline medium. As shown in Fig. 5e and f, the durability measurement was confirmed by multiple-step chronopotentiometry with negligible decay as long as 30 h demonstrating its excellent stability in seawater.
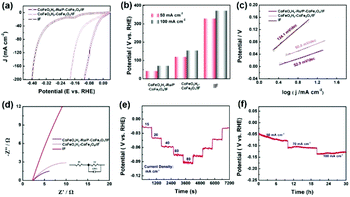 |
| Fig. 5 Electrochemical measurements: (a) HER polarization curves. (b) Overpotential comparison at 50 mA cm−2 and 100 mA cm−2. (c) Tafel slopes. (d) Nyquist plots. (e and f) Stability tests of the obtained catalysts in 1 M KOH seawater. | |
Encouraged by the remarkable HER catalytic performance in alkaline media, the catalytic activity of CoFeOxHy–Ru/P–CoFe2O4/IF was further investigated in neutral medium (1 M PBS). As shown in Fig. 6a and b, the as-prepared CoFeOxHy–Ru/P–CoFe2O4/IF also possesses better catalytic activity than CoFeOxHy–CoFe2O4/IF and IF with the lowest overpotential of 30.1 mV and 69.3 mV to achieve current densities of 50 mA cm−2 and 100 mA cm−2. Moreover, the Tafel slope of CoFeOxHy–Ru/P–CoFe2O4/IF (58.2 mV dec−1) is lower than those of CoFeOxHy–CoFe2O4/IF (161.2 mV dec−1) and IF (163.1 mV dec−1) (Fig. 6c). The catalytic performance of CoFeOxHy–Ru/P–CoFe2O4/IF is even better than those of most of the reported noble metal catalysts, such as CC@WO3/Ru-450 (64 mV),54 RuSA–N–Ti3C2Tx (81 mV),55 Ru/OMSNNC (70 mV),56 Rh2P/NPC (119 mV),57 Ru–Cr2O3/NG (53 mV),58 Ru/MEOH/THF (83 mV),59 Rh2P (38 mV)60 and RhP2@NPC (57 mV)61 (Fig. 6d and Table S4†). As shown in Fig. 6e and f, the as-prepared electrocatalyst presents excellent long-term stability in 1 M PBS.
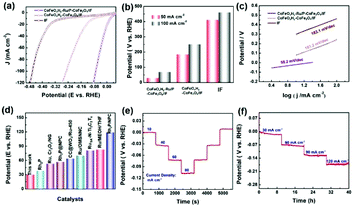 |
| Fig. 6 Electrochemical measurements: (a) HER polarization curves. (b) Overpotential comparison at 50 mA cm−2 and 100 mA cm−2. (c) Tafel slopes. (d) Comparison of the overpotentials with the references. (e and f) Stability tests of the obtained catalysts in 1 M PBS. | |
Encouraged by the excellent electrocatalytic activity toward the HER, a two-electrode water-splitting setup was built with the prepared CoFeOxHy–Ru/P–CoFe2O4/IF as the cathode and RuO2/CoFe2O4 (Fig. S14–S17†) as the anode for overall water-splitting in 1 M KOH solution (Fig. 7a). As shown in Fig. 7b, the electrolyzer can attain 10 mA cm−2 with a low voltage of 1.49 V which is lower than those of many other reported available catalysts (Fig. 7c and Table S5†).As solar energy is a sustainable and green energy, it attracts tremendous attention in hydrogen production. Thanks to the small voltage, the overall water-splitting setup is easily powered by solar energy then amounts of bubbles generated on the electrodes (Fig. 7d and Movie S1†). Importantly, the designed CoFeOxHy–Ru/P–CoFe2O4/IF∥RuO2/CoFe2O4 also exhibits excellent stability for overall water-splitting via multi-step measurements (Fig. 7e and f). As mentioned above, the developed electrocatalyst presents excellent catalytic activity which can be attributed to the following factors: the specific nanobelts favour the transport of the electrolyte; a trace amount of Ru nanoclusters plays a crucial role in improving the HER performance; and phosphorus can effectively optimize the density of electron clouds around metal atoms, resulting in an optimized hydrogen adsorption energy and favorable hydrogen release.62 P atoms facilitate proton adsorption and thus accelerate the reaction kinetics. In addition, the doped P can improve the electron mobility for attaining rapid electron transport, which can adjust the electron structure and play a role in regulating charge accumulation and consumption for an excellent HER.63 Due to the different electronegativities of phosphorus, the catalytic activity of the composite components can be regulated, so that the obtained catalyst has good hydrogen evolution performance in 1 M KOH, 1 M KOH seawater and 1 M PBS; the in situ generated metal hydroxides play a key role in promoting the electrocatalytic performance by accelerating the reaction kinetics; the IF substrate provides abundant channels for the transport of the electrolyte and avoids the usage of a binding agent.
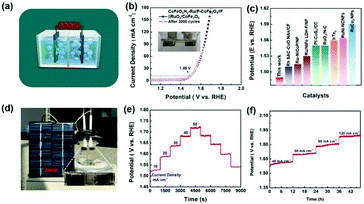 |
| Fig. 7 (a) Schematic diagram of water splitting in a two-electrode setup. (b) LSV curves of CoFeOxHy–Ru/P–CoFe2O4/IF∥RuO2/CoFe2O4 and the catalyst after the stability test of 2000 cycles for overall water splitting in 1 M KOH (the inset is the photograph of the water splitting cell). (c) Solar cell driving overall water-splitting. (d) Comparison of the overpotentials (Ej10) of the synthesized electrocatalysts with the reported catalysts. (e and f) Stability tests of the obtained catalysts in 1 M KOH. | |
4. Conclusions
In summary, a hierarchical structure of CoFeOxHy–Ru/P–CoFe2O4/IF nanobelts decorated with dense and tiny CoFe double hydroxide nanosheets supported on iron foam is developed via hydrothermal and annealing treatment and further hydrolyzed in alkaline media. Owing to the combination of metal oxide species and a trace amount of Ru nanoclusters, in addition to the Co–Fe double hydroxide nanosheets during the hydrolysis process, the obtained composite nanomaterial serves as a remarkable electrocatalyst for boosting the HER activity in alkaline, seawater and neutral electrolytes. Accordingly, the obtained electrocatalyst exhibits excellent catalytic performances toward overall water-splitting with remarkable long-term stability in 1 M KOH, which can also be powered by sustainable solar energy. Additionally, the iron foam substrate possesses an intricate skeleton with rich channels which improves the electrical conductivity and timely release of the generated bubbles to expose the active substances. Ru clusters can accelerate the HER rate by converting into a faster electron transport paths and possess a moderate H* adsorption value in favour of the HER process. Consequently, the developed CoFeOxHy–Ru/P–CoFe2O4/IF presents superior performances to that of previously reported materials toward the HER and overall water-splitting. This work provides a valuable strategy to develop other specific nanostructures as efficient electrocatalysts for energy-related applications.
Conflicts of interest
There are no conflicts to declare.
Acknowledgements
The authors acknowledge funding support from the National Natural Science Foundation of China (22002068, 51772162, and 52072197), the Youth Innovation and Technology Foundation of Shandong Higher Education Institutions, China (2019KJC004), the Outstanding Youth Foundation of Shandong Province, China (ZR2019JQ14), the Taishan Scholar Young Talent Program (tsqn201909114), the Major Scientific and Technological Innovation Project (2019JZZY020405), the Major Basic Research Program of Natural Science Foundation of Shandong Province under Grant No. ZR2020ZD09, the Project funded by the China Postdoctoral Science Foundation (2021M691700), and the Natural Science Foundation of Shandong Province of China (ZR2019BB002 and ZR2018BB031).
Notes and references
- L. Q. Gong, H. Yang, H. M. Wang, R. J. Qi, J. L. Wang, S. H. Chen, B. You, Z. H. Dong, H. F. Liu and B. Y. Xia, Corrosion formation and phase transformation of nickel-iron hydroxide nanosheets array for efficient water oxidation, Nano Res., 2021, 14, 4528–4533 CrossRef CAS
.
- G. Zhao, W. Ma, X. Wang, Y. Xing, S. Hao and X. Xu, Self-water-absorption-type two-dimensional composite photocatalyst with high-efficiency water absorption and overall water-splitting performance, Adv. Powder Mater., 2021 DOI:10.1016/j.apmate.2021.09.008
.
- Z. Li, X. Wu, X. Jiang, B. Shen, Z. Teng, D. Sun, G. Fu and Y. Tang, Surface carbon layer controllable Ni3Fe particles confined in hierarchical N-doped carbon framework boosting oxygen evolution reaction, Adv. Powder Mater., 2022 DOI:10.1016/j.apmate.2021.11.007
.
- J. Li, S. Zhang, J. Sha, H. Wang, M. Liu, L. Kong and G. Liu, Confined Molybdenum Phosphide in P-Doped Porous Carbon as Efficient Electrocatalysts for Hydrogen Evolution, ACS Appl. Mater. Interfaces, 2018, 10, 17140–17146 CrossRef CAS PubMed
.
- H. Yang, L. Gong, H. Wang, C. Dong, J. Wang, K. Qi, H. Liu, X. Guo and B. Xia, Preparation of nickel-iron hydroxides by microorganism corrosion for efficient oxygen evolution, Nat. Commun., 2020, 11, 5075 CrossRef CAS PubMed
.
- J. Li, L. Kong, Z. Wu, S. Zhang, X. Yang, J. Sha and G. Liu, Polydopamine-assisted construction of cobalt phosphide encapsulated in N-doped carbon porous polyhedrons for enhanced overall water splitting, Carbon, 2019, 145, 694–700 CrossRef CAS
.
- Z. Wu, Y. Zhao, W. Jin, B. H. Jia, J. Wang and T. Y. Ma, Recent Progress of Vacancy Engineering for Electrochemical Energy Conversion Related Applications, Adv. Funct. Mater., 2021, 31, 2009070 CrossRef CAS
.
- H. Jing, P. Zhu, X. Zheng, Z. Zhang, D. Wang and Y. Li, Theory-oriented screening and discovery of advanced energy transformation materials in electrocatalysis, Adv. Powder Mater., 2021 DOI:10.1016/j.apmate.2021.10.004
.
- P. F. Cheng, T. Feng, Z. W. Liu, D. Y. Wu and J. Yang, Laser-direct-writing of 3D self-supported NiS2/MoS2 heterostructures as an efficient electrocatalyst for hydrogen evolution reaction in alkaline and neutral electrolytes, Chin. J. Catal., 2019, 40, 1147–1152 CrossRef CAS
.
- H. Chen, X. Liang, Y. Liu, X. Ai, T. Asefa and X. Zou, Active site engineering in porous electrocatalysts, Adv. Mater., 2020, 32, 2002435 CrossRef PubMed
.
- J. Wang, Z. Zhang, H. Song, B. Zhang, J. Liu, X. Shai and L. Miao, Water Dissociation Kinetic–Oriented Design of Nickel Sulfides via Tailored Dual Sites for Efficient Alkaline Hydrogen Evolution, Adv. Funct. Mater., 2021, 31, 2008578 CrossRef CAS
.
- X. P. Sun, Ni foam-supported NiCoP nanosheets as bifunctional electrocatalysts for efficient overall water splitting, Chin. J. Catal., 2019, 40, 1405–1407 CrossRef CAS
.
- M. Zhao, W. Li, J. Y. Li, W. H. Hu and C. M. Li, Strong electronic interaction enhanced electrocatalysis of metal sulfide clusters embedded metal–organic framework ultrathin nanosheets toward highly efficient overall water splitting, Adv. Sci., 2020, 7, 2001965 CrossRef CAS PubMed
.
- Q. Liu, J. Tian, W. Cui, P. Jiang, N. Cheng, A. M. Asiri and X. Sun, Carbon nanotubes decorated with CoP nanocrystals: a highly active non-noble-metal nanohybrid electrocatalyst for hydrogen, Evol., Angew. Chem., Int. Ed., 2014, 53, 6710–6714 CrossRef CAS PubMed
.
- X. Li, L. Yang, T. Su, X. Wang, C. Sun and Z. Su, Graphene-coated hybrid electrocatalysts derived from bimetallic metal–organic frameworks for efficient hydrogen generation, J. Mater. Chem. A, 2017, 5, 5000–5006 RSC
.
- C. T. Dinh, A. Jain, F. P. G. de Arquer, P. De Luna, J. Li, N. Wang, X. Zheng, J. Cai, B. Z. Gregory, O. Voznyy, B. Zhang, M. Liu, D. Sinton, E. J. Crumlin and E. H. Sargent, Multi-site electrocatalysts for hydrogen evolution in neutral media by destabilization of water molecules, Nat. Energy, 2018, 4, 107–114 CrossRef
.
- Z. Chen, X. Duan, W. Wei, S. Wang and B. J. Ni, Recent advances in transition metal based electrocatalysts for alkaline hydrogen evolution, J. Mater. Chem. A, 2019, 7, 14971–15005 RSC
.
- J. Sun, W. Xu, C. Lv, L. Zhang, M. Shakouri, Y. Peng, Q. Wang, X. Yang, D. Yuan, M. Huang, Y. Hu, D. Yang and L. Zhang, Co/MoN hetero-interface nanoflake array with enhanced water dissociation capability achieves the Pt-like hydrogen evolution catalytic performance, Appl. Catal., B, 2021, 286, 119882 CrossRef CAS
.
- B. Liu, Y. F. Zhao, H. Q. Peng, Z. Y. Zhang, C. K. Sit, M. F. Yuen, T. R. Zhang, C. S. Lee and W. J. Zhang, Nickel-cobalt diselenide 3D mesoporous nanosheet networks supported on Ni foam: an all-pH highly efficient integrated electrocatalyst for hydrogen evolution, Adv. Mater., 2017, 29, 1606521 CrossRef PubMed
.
- J. Y. Zhang, Y. Yan, B. Mei, R. Qi, T. He, Z. Wang, W. Fang, S. Zaman, Y. Su, S. Ding and B. Y. Xia, Local spin-state tuning of cobalt–iron selenide nanoframes for the boosted oxygen evolution, Energy Environ. Sci., 2021, 14, 365–373 RSC
.
- Q. Shi, C. Zhu, D. Du and Y. Lin, Robust noble metal-based electrocatalysts for oxygen evolution reaction, Chem. Soc. Rev., 2019, 48, 3181–3192 RSC
.
- H. Sun, W. Zhang, J.-G. Li, Z. Li, X. Ao, K.-H. Xue, K. K. Ostrikov, J. Tang and C. Wang, Rh-engineered ultrathin NiFe-LDH nanosheets enable highly-efficient overall water splitting and urea electrolysis, Appl. Catal., B, 2021, 284, 119740 CrossRef CAS
.
- L. Bao, S. Zhu, Y. Chen, Y. Wang, W. Meng, S. Xu, Z. Lin, X. Li, M. Sun and L. Guo, Anionic defects engineering of Co3O4 catalyst for toluene oxidation, Fuel, 2022, 314, 122774 CrossRef CAS
.
- Z. Wu, Y. Zhao, H. Wu, Y. Gao, Z. Chen, W. Jin, J. Wang, T. Ma and L. Wang, Corrosion Engineering on Iron Foam toward Efficiently Electrocatalytic Overall Water Splitting Powered by Sustainable Energy, Adv. Funct. Mater., 2021, 31, 2010437 CrossRef CAS
.
- T. Zhang, B. Zhang, Q. Peng, J. Zhou and Z. M. Sun, Mo2B2 MBene-supported single-atom catalysts as bifunctional HER/OER and OER/ORR electrocatalysts, J. Mater. Chem. A, 2021, 9, 433–441 RSC
.
- F. Zhang, R. Ji, Y. Liu, Y. Pan, B. Cai, Z. Li, Z. Liu, S. Lu, Y. Wang, H. Jin, C. Ma and X. Wu, A novel nickel-based honeycomb electrode with microtapered holes and abundant multivacancies for highly efficient overall water splitting, Appl. Catal., B, 2020, 276, 119141 CrossRef CAS
.
- Y. Liu, Q. Feng, W. Liu, Q. Li, Y. Wang, B. Liu, L. Zheng, W. Wang, L. Huang, L. Chen, X. Xiong and Y. Lei, Boosting interfacial charge transfer for alkaline hydrogen evolution via rational interior Se modification, Nano Energy, 2021, 81, 105641 CrossRef CAS
.
- D. Guo, J. Wang, L. Zhang, X. Chen, Z. Wan and B. Xi, Strategic Atomic Layer Deposition and Electrospinning of Cobalt Sulfide/Nitride Composite as Efficient Bifunctional Electrocatalysts for Overall Water Splitting, Small, 2020, 6, e2002432 CrossRef PubMed
.
- W. Li, Y. Liu, M. Wu, X. Feng, S. A. T. Redfern, Y. Shang, X. Yong, T. Feng, K. Wu, Z. Liu, B. Li, Z. Chen, J. S. Tse, S. Lu and B. Yang, Carbon-Quantum-Dots-Loaded Ruthenium Nanoparticles as an Efficient Electrocatalyst for Hydrogen Production in Alkaline Media, Adv. Mater., 2018, 30, 1800676 CrossRef PubMed
.
- Y. Liu, X. Li, Q. Zhang, W. Li, Y. Xie, H. Liu, L. Shang, Z. Liu, Z. Chen, L. Gu, Z. Tang, T. Zhang and S. Lu, Angew. Chem., 2021, 283, 119583 Search PubMed
.
- B. B. Yang, J. Y. Xu, D. Bin, J. Wang, J. Z. Zhao, Y. X. Liu, B. X. Li, X. N. Fang, Y. Liu, L. Qiao, L. F. Liu and B. H. Liu, Amorphous phosphatized ruthenium-iron bimetallic nanoclusters with Pt-like activity for hydrogen evolution reaction, Appl. Catal., B, 2021, 414, 128784 Search PubMed
.
- W. S. Fang, L. Huang, S. Zaman, Z. Wang, Y. J. Han and B. Y. Xia, Chem. Res. Chin. Univ., 2020, 36, 611–621 CrossRef CAS
.
- W. B. Chen, C. S. Wang, S. B. Sua, H. Wang and D. D. Cai, Synthesis of ZIF-9(III)/Co LDH layered composite from ZIF-9(I) based on controllable phase transition for enhanced electrocatalytic oxygen evolution reaction, Chem. Eng. J., 2021, 414, 128784 CrossRef CAS
.
- D. Gao, R. Liu, J. Biskupek, U. Kaiser, Y.-F. Song and C. Streb, Modular design of noble-metal-free mixed metal oxide electrocatalysts for complete water splitting, Angew. Chem., Int. Ed., 2019, 58, 4644 CrossRef CAS PubMed
.
- R. Atchudan, T. N. Jebakumar Immanuel Edison, S. Perumal, R. Vinodh, N. Muthuchamy and Y. R. Lee, One-pot synthesis of Fe3O4@graphite sheets as electrocatalyst for water electrolysis, Fuel, 2020, 277, 118235 CrossRef CAS
.
- S. J. Liu, J. Zhu, M. Sun, Z. X. Ma, K. Hu, T. Nakajima, X. H. Liu, P. Schmuki and L. Wang, Promoting Hydrogen Evolution Reaction through Oxygen Vacancies and Phase Transformation Engineering on Layered Double Hydroxide Nanosheets, J. Mater. Chem. A, 2020, 8, 2490–2497 RSC
.
- X. L. Luo, Q. Shao, Y. C. Pi and X. Q. Huang, Trimetallic Molybdate Nanobelts as Active and Stable Electrocatalysts for the Oxygen Evolution Reaction, ACS Catal., 2019, 9, 1013–1018 CrossRef CAS
.
- X. Zhao, H. T. Zhang, Y. Yan, J. H. Cao, X. Q. Li, S. M. Zhou, Z. M. Peng and J. Zeng, Engineeringthe Electrical Conductivity of Lamellar Silver-Doped Cobalt(II) Selenide Nanobelts for Enhanced Oxygen Evolution, Angew. Chem., Int. Ed., 2017, 56, 328–332 CrossRef CAS PubMed
.
- C. Q. Huang, L. Yu, W. Zhang, Q. Xiao, J. Q. Zhou, Y. L. Zhang, P. F. An, J. Zhang and Y. Yu, N-doped Ni-Mo
based sulfides for high-efficiency and stable hydrogen evolution reaction, Appl. Catal., B, 2020, 276, 119137 CrossRef CAS
.
- Z. H. Yan, H. M. Sun, X. Chen, H. H. Liu, Y. R. Zhao, H. X. Li, W. Xie, F. Y. Cheng and J. Chen, Anion insertion enhanced electrodeposition of robust metal hydroxide/oxide electrodes for oxygen evolution, Nat. Commun., 2018, 9, 2373 CrossRef PubMed
.
- C. H. Zhao, N. Li, R. Z. Zhang, Z. Q. Zhu, J. H. Lin, K. F. Zhang and C. J. Zhao, Surface Reconstruction of La0.8Sr0.2Co0.8Fe0.2O3−δ for Superimposed OER Performance, ACS Appl. Mater. Interfaces, 2019, 11, 47858–47867 CrossRef CAS PubMed
.
- C. Zhao, P. Li, D. Shao, R. Zhang, S. Wang, Z. Zhu and C. Zhao, Phytic Acid-derived Co2−xNixP2O7-C/RGO and its Superior OER Electrocatalytic Performance, Int. J. Hydrogen Energy, 2019, 44, 844–852 CrossRef CAS
.
- L. Zhang, W. H. He, X. Xiang, Y. Li and F. Li, Roughening of windmill-shaped spinel Co3O4 microcrystals grown on a flexible metal substrate by a facile surface treatment to enhance their performance in the oxidation of water, RSC Adv., 2014, 4, 43357 RSC
.
- L. Li, G. W. Zhang, B. Wang and S. C. Yang, Constructing the Fe/Cr double (oxy)hydroxides on Fe3O4 for Boosting the Electrochemical Oxygen Evolution in Alkaline Seawater and Domestic Sewage, Appl. Catal., B, 2022, 302, 120847 CrossRef CAS
.
- H. Zhang, Y. Zhao, Y. Zhang, M. Zhang, M. Cheng, J. Yu, H. Liu, M. Ji, C. Zhu and J. Xu, Fe3O4 encapsulated in porous carbon nanobowls as efficient oxygen reduction reaction catalyst for Zn-air batteries, Chem. Eng. J., 2019, 375, 122058 CrossRef CAS
.
- L. Wang, Q. Zhou, Z. H. Pu, Q. Zhang, X. Q. Mu, H. Y. Jing, S. L. Liu, C. Y. Chen and S. C. Mu, Surface Reconstruction Engineering of Cobalt Phosphides by Ru Inducement to form Hollow Ru-RuPx-CoxP Pre-electrocatalysts with Accelerated Oxygen Evolution Reaction, Nano Energy, 2018, 53, 270–276 CrossRef CAS
.
- N. L. W. Septiani, Y. V. Kaneti, K. B. Fathoni, Y. Guo, Y. Ide, B. Yuliarto, X. Jiang, H. K. Nugraha, D. Dipojono and Y. Y. Golberg, Tailorable nanoarchitecturing of bimetallic nickel–cobalt hydrogen phosphate via the self-weaving of nanotubes for efficient oxygen evolution, J. Mater. Chem. A, 2020, 8, 3035–3047 RSC
.
- Y. Zhang, Q. Shao, S. Long and X. Q. Huang, Cobalt-molybdenum nanosheet arrays as highly efficient and stable earth-abundant electrocatalysts for overall water splitting, Nano Energy, 2018, 45, 448–455 CrossRef CAS
.
- Y. S. Jin, S. L. Huang, X. Yue, H. Y. Du and P. K. Shen, Mo- and Fe-Modified Ni(OH)2/NiOOH Nanosheets as Highly Active and Stable Electrocatalysts for Oxygen Evolution Reaction, ACS Catal., 2018, 8, 2359–2363 CrossRef CAS
.
- P. X. Ji, X. Luo, D. Chen, H. H. Jin, Z. H. Pu, W. H. Zeng, J. W. He, H. W. Bai, Y. C. Liao and S. C. Mu, Significantly Improved Water Oxidation of CoP Catalysts by Electrochemical Activation, ACS Sustainable Chem. Eng., 2020, 8, 17851–17859 CrossRef CAS
.
- S. Z. Chen, Y. Zhang, J. Jin, H. X. Qu, T. L. Zhu and Q. Zhong, 3D network Ni-based electrocatalysts with interfacial active sites of Ru QDs and Ni(OH)2 for efficient hydrogen evolution reaction under large current density in alkaline environment, Electrochim. Acta, 2020, 356, 136732 CrossRef CAS
.
- X. J. Zhai, Q. P. Yu, G. S. Liu, J. L. Bi, Y. Zhang, J. Q. Chi, J. P. Lai, B. Yang and L. Wang, Hierarchical microsphere MOF arrays with ultralow Ir doping for efficient hydrogen evolution coupled with hydrazine oxidation in seawater, J. Mater. Chem. A, 2021, 9, 27424 RSC
.
- L. B. Wu, L. Yu, F. H. Zhang, B. McElhenny, D. Luo, A. Karim, S. Chen and Z. F. Ren, Heterogeneous Bimetallic Phosphide Ni2P-Fe2P as an Efficient Bifunctional Catalyst for Water/Seawater Splitting, Adv. Funct. Mater., 2020, 31, 2006484 CrossRef
.
- J. C. Li, C. Zhang, C. Zhang, H. J. Ma, Y. Yang, Z. Q. Guo, Y. Y. Wang and H. X. Ma, Electronic configuration of single ruthenium atom immobilized in urchin-like tungsten trioxide towards hydrazine oxidation-assisted hydrogen evolution under wide pH media, Chem. Eng. J., 2022, 430, 132953 CrossRef CAS
.
- H. G. Liu, Z. Hu, Q. L. Liu, P. Sun, Y. F. Wang, S. L. Chou, Z. Z. Hu and Z. Q. Zhang, Single-atom Ru anchored in nitrogen-doped MXene (Ti3C2Tx) as an efficient catalyst for the hydrogen evolution reaction at all pH values, J. Mater. Chem. A, 2020, 8, 24710–24717 RSC
.
- Y. L. Wu, X. F. Li, Y. S. Wei, Z. M. Fu, W. B. Wei, X. T. Wu, Q. L. Zhu and Q. Xu, Ordered Macroporous Superstructure of Nitrogen-Doped Nanoporous Carbon Implanted with Ultrafine Ru Nanoclusters for Efficient pH-Universal Hydrogen Evolution Reaction, Adv. Mater., 2021, 33, 2006965 CrossRef CAS PubMed
.
- S. J. Liu, Y. J. Chen, L. Yu, Y. Lin, Z. Liu, M. M. Wang, Y. J. Chen, C. Zhang, Y. Pan, Y. Q. Liu and C. G. Liu, A supramolecular-confinement pyrolysis route to ultrasmall rhodium phosphide nanoparticles as a robust electrocatalyst for hydrogen evolution in the entire pH range and seawater electrolysis, J. Mater. Chem. A, 2020, 8, 25768–25779 RSC
.
- L. Tang, J. J. Yu, Y. Zhang, Z. Z. Tang and Y. Qin, Boosting the Hydrogen Evolution Reaction Activity of Ru in Alkaline and Neutral Media by Accelerating Water Dissociation, RSC Adv., 2021, 11, 6107 RSC
.
- S. Drouet, J. Creus, V. Collière, C. Amiens, J. García-Antón, X. Sala and K. Philippot, A porous Ru nanomaterial as an efficient electrocatalyst for the hydrogen evolution reaction under acidic and neutral conditions, Chem. Commun., 2017, 53, 11713 RSC
.
- F. L. Yang, Y. M. Zhao, Y. S. Du, Y. T. Chen, G. Z. Cheng, S. L. Chen and W. Luo, A Monodisperse Rh2P-Based Electrocatalyst for Highly Efficient and pH-Universal Hydrogen Evolution Reaction, Adv. Energy Mater., 2018, 8, 1703489 CrossRef
.
- Z. H. Pu, I. S. Amiinu, Z. K. Kou, W. Q. Li and S. C. Mu, RuP2-Based Catalysts with Platinum-like Activity and Higher Durability for the Hydrogen Evolution Reaction at All pH Values, Angew. Chem., Int. Ed., 2017, 56, 11559 CrossRef CAS PubMed
.
- X. Y. Zhang, F. T. Li, R. Y. Fan, J. Zhao, B. Dong, F. L. Wang, H. J. Liu, J. F. Yu, C. G. Liu and Y. M. Chai, F, P double-doped Fe3O4 with abundant defect sites for efficient hydrogen evolution at high current density, J. Mater. Chem. A, 2021, 9, 15836–15845 RSC
.
- M. Zhu, Q. Yan, Y. Q. Xue, Y. D. Yan, K. Zhu, K. Ye, J. Yan, D. X. Cao, H. J. Xie and G. L. Wang, Free-Standing P-Doped NiSe2/MoSe2 Catalyst for Efficient Hydrogen Evolution in Acidic and Alkaline Media, ACS Sustainable Chem. Eng., 2022, 10, 279–287 CrossRef CAS
.
Footnote |
† Electronic supplementary information (ESI) available: SEM images, XRD patterns, XPS spectra, electrochemical data. See DOI: 10.1039/d2qi00086e |
|
This journal is © the Partner Organisations 2022 |
Click here to see how this site uses Cookies. View our privacy policy here.