DOI:
10.1039/D1QO01069G
(Research Article)
Org. Chem. Front., 2022,
9, 32-38
K2S2O8-mediated acylarylation of unactivated alkenes via acyl radical addition/C–H annulation cascade of N-allyl-indoles with silver cocatalysis†
Received
20th July 2021
, Accepted 29th September 2021
First published on 29th September 2021
Abstract
A silver-catalyzed, K2S2O8-mediated protocol to access regioselective acylarylation of unactivated alkenes was developed. The reaction between N-allyl-indoles and α-oxocarboxylic acids proceeded smoothly and involved an acyl radical addition/C–H cyclization cascade. The protocol showed a broad substrate scope and good tolerance of functional groups. The reaction proceeded with both internal and terminal alkenes to furnish many functional pyrrolo[1,2-a]indoles bearing the ketone carbonyl group, and this feature also provides the potential to construct structurally complex N-containing heterocycles.
Introduction
Transition-metal-catalyzed difunctionalization of alkenes affords one of the most powerful strategies for constructing complex C(sp3)-hybridized molecular frameworks.1 The difunctionalization transformation of alkenes has been well exploited by varying the coupling partners.2 As for the transition-metal-catalyzed alkene dicarbofunctionalization, a radical addition/C–H functionalization cascade triggered by direct carboradical-addition to olefins represents one of the most efficient and promising processes, and features high atom/step economy and stands in stark contrast to the processes that deploy prefunctionalized substrates.3 In particular, the intramolecular dicarbofunctionalization reaction of alkenes with widely explored radical precursors offers an attractive synthetic strategy to construct diverse functional scaffolds for rapid access to complex molecules.4 Of the various radical precursors, user-friendly and readily available α-oxocarboxylic acids have attracted substantial attention, and can be used to produce valuable acyl radical species for carbon–carbon bond formations involving direct C–H acylation.5,6 Nevertheless, successfully employing α-oxocarboxylic acids in regioselective difunctionalization of unactivated alkenes involving C–H activation—and in which direct C–H acylation is completely avoided—remains very challenging. In this regard, the development of a general and practical strategy to address this issue is highly desirable.
On the other hand, polycyclic indoles such as pyrrolo[1,2-a]indole constitute one of the important heterocycle classes with biological activities, and are widely found in numerous natural products and pharmaceutical chemicals.7–9 Thus, tremendous efforts have been devoted to establishing diverse methodologies to construct these skeletons.10 In the alkene difunctionalizations, an activated alkene has generally been used as a “linchpin” to facilitate the regioselectivity of the transformation,11 but few successes have been achieved when the more challenging yet appealing unactivated alkenes were used (Fig. 1a).4q To address these limitations and as part of our continuing interest in heterocycle chemistry involving C–H activation,12,13 we sought to develop an acyl-radical-based cascade reaction enabled by oxidative C–H annulation of unactivated alkene-linked indoles. Herein, we describe a K2S2O8-mediated, silver-catalyzed process14 for regioselective acylarylation of unactivated alkenes with N-allyl-indoles and α-oxocarboxylic acids, a reaction that was shown to have a broad substrate scope and display good tolerance of functional groups (Fig. 1b). The reaction proceeded with both internal and terminal alkenes to furnish many functional pyrrolo[1,2-a]indoles bearing the ketone carbonyl group, which might be subject to readily accessible derivatization, thus leading to structurally complex N-containing heterocycles.
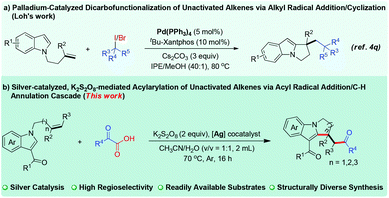 |
| Fig. 1 Construction of pyrrolo[1,2-a]indoles via the radical difunctionalization of unactivated alkenes. | |
Results and discussion
We commenced the study of the acylarylation of unactived alkenes using 1-(1-cinnamyl-1H-indol-3-yl)ethanone and 2-oxo-2-phenylacetic acid (2a) as the model substrates (Table 1; for more details, see the ESI†). To our delight, the desired transformation occurred successfully in the presence of AgOAc (0.1 equiv.) and K2S2O8 (2 equiv.) in CH3CN/H2O under an Ar atmosphere, giving the difunctionalized compound 3aa in 74% yield (Table 1, entry 1). While no reaction took place in the absence of K2S2O8, a moderate yield was obtained without addition of AgOAc (Table 1, entries 2 and 3). Then several potential oxidants including Na2S2O8, (NH4)2S2O8, and oxone were examined, but they did not provide better results (Table 1, entries 4–6). When a single solvent, namely H2O or CH3CN, was employed as the reaction medium, the reaction did not work at all (Table 1, entries 7 and 8). The reactivity was further improved when the loading of AgOAc was decreased to 0.05 equiv., generating the desired product in 84% yield (Table 1, entry 9). Lastly, the screening of other silver salts, namely Ag2CO3, Ag2O and AgOTf, did not lead to a better efficiency (Table 1, entries 10–12).
Table 1 Optimization of the reaction conditionsa
With an optimized set of conditions in hand, the scope of each reaction component was evaluated. First, as shown in Table 2, we investigated the generality of N-allyl-indoles in this transformation. In general, the reaction showed a broad substrate scope, and various functional groups on the phenyl ring of the indole were tolerated when carrying out the bifunctionalization of unactivated alkenes to produce functionalized 2,3-dihydro-1H-pyrrolo[1,2-a]indoles; a variety of such indoles were produced (3aa–3fa, 3ha–3ja), in moderate to good yields. Notably, the strongly electron-withdrawing cyano group was found to be compatible with the catalytic system and its use afforded the corresponding product 3ga in moderate yield. In addition to the diverse array of indoles, a range of C3-functionalized indoles were evaluated and their reactions showed good efficiency levels, generating products that could serve as linchpins for subsequent diversification of the pyrrole region (3ka–3ma). However, the current acylarylation protocol was found to not be suitable for an N-allyl-indole bearing no substituent as well a one bearing a strongly electron-withdrawing group at the C3 position (3na and 3oa).
Table 2 Scope of N-allyl-indole substratesa,b
Reaction conditions: 1 (0.2 mmol), 2a (0.4 mmol), AgOAc (5 mol%), K2S2O8 (0.4 mmol), CH3CN/H2O (2.0 mL, v/v = 1 : 1), Ar, 70 °C, 16 h.
Isolated yield based on 1 and using flash column chromatography.
|
|
Subsequently, we turned our attention to the scope of α-oxocarboxylic acids (Table 3). The successful utilization of various 2-oxo-2-arylacetic acids as acyl radical precursors demonstrated the good generality of this radical addition/cyclization cascade, allowing the facile access to structurally diverse 2,3-dihydro-1H-pyrrolo[1,2-a]indoles (3ab–3al). The absolute chemical structure of one of these indoles was determined using X-ray crystallography (3ab, CCDC 1965912; for more details, see the ESI†).15 Notably, 2-oxo-2-(3,4,5-trimethoxyphenyl)acetic acid and 2-(naphthalen-2-yl)-2-oxoacetic acid also underwent this reaction smoothly to offer the corresponding products 3ak and 3al in 63% and 53% yields, respectively. Moreover, aliphatic α-oxocarboxylic acids, while generating less stable acyl species, were also suitable and delivered the desired adducts (3am–3an), albeit with diminished efficiency levels (35% and 40% yields). Unfortunately, the reaction did not take place when testing a couple of heteroaromatic α-oxocarboxylic acids (3ao and 3ap) and when using 2-(4-nitrophenyl)-2-oxoacetic acid (3aq).
Table 3 Substrate scope of α-oxocarboxylic acidsa,b
Reaction conditions: 1a (0.2 mmol), 2 (0.4 mmol), AgOAc (5 mol%), K2S2O8 (0.4 mmol), CH3CN/H2O (2.0 mL, v/v = 1 : 1), Ar, 70 °C, 16 h.
Isolated yield based on 1a and using flash column chromatography.
|
|
Interestingly, a series of indole-based unactivated alkenes also participated in this difunctionalization reaction smoothly, under slightly modified reaction conditions, to produce functionalized aliphatic cyclic fused indoles (Scheme 1). The flexibility of this transformation was investigated by deploying certain kinds of α-oxocarboxylic acids and indole substrates, which generated the desired products successfully (5a–5i), although the uncompleted conversion of indole-based alkene 4 led to a depressed yield. Notably, tetrahydropyrido[1,2-a]indole and tetrahydro-6H-azepino[1,2-a]indole motifs were generated successfully from indole-based pent-1-ene and hex-1-ene, respectively (5j and 5k). And the more bulky disubstituted alkenes also showed reactivity for this reaction to afford the corresponding products, albeit with low to moderate yields (5l and 5m). However, the cyclic alkene tested was not compatible with the current reaction conditions (5n). The type of substituent used at the C3-position of the indole seemed to be crucial for the success of this transformation, as several functional groups such as Me and CO2Et were not tolerated (5o and 5p).
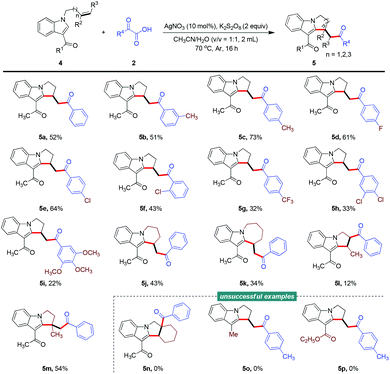 |
| Scheme 1 Reaction of N-allyl-1H-indoles with α-oxocarboxylic acids. Conditions: 4 (0.2 mmol), 2 (0.4 mmol), AgNO3 (10 mol%), K2S2O8 (0.4 mmol), CH3CN/H2O (2.0 mL, v/v = 1 : 1), Ar, 70 °C, 16 h. | |
To gain more insight into the reaction details, experiments with radicals were carried out. The reaction was dramatically inhibited when either the radical scavenger TEMPO or BHT was included in the reaction mixture, and the radical coupling compound 6 was isolated successfully, thus providing evidence for the generation of an acyl radical intermediate (Scheme 2).
 |
| Scheme 2 Mechanistic studies. | |
Based on experimental results and previous research,16 a plausible reaction mechanism was proposed (Scheme 3). According to this proposed mechanism, an initial decarboxylation process was promoted by K2S2O8 and AgOAc catalysis to form acyl radical species A, which participated in a radical addition to the alkene C
C bond to preferentially generate a more stable benzyl radical intermediate, namely B. The subsequent intramolecular radical annulation of the pyrrole core of the indole furnished a new radical, namely C, which participated in an oxidation and then deprotonation to terminate the process and afford the desired products 3.
 |
| Scheme 3 Plausible mechanism. | |
Conclusions
In summary, we successfully developed an efficient silver-catalyzed, K2S2O8-mediated reaction of N-allyl-indoles with α-oxocarboxylic acids, thus achieving acylarylations of unactivated alkenes with high regioselectivity and a broad substrate scope. Both internal and terminal alkenes participated in the reaction effectively to generate many pyrrolo[1,2-a]indole-based ketones. The mechanistic study carried out provided evidence for a radical process in this transformation by capturing the acyl radical species. Further investigation of the application potential of this method for the synthesis of other biologically active molecules is ongoing in our laboratory.
Conflicts of interest
There are no conflicts to declare.
Acknowledgements
We thank the National Natural Science Foundation of China (21702237, 21272004), the Natural Science Research Project for Anhui Universities, the Start-up Research Fund of Anhui Normal University and the Project for Students’ Innovative Experiment of Anhui Normal University for their financial support.
Notes and references
-
(a) R. K. Dhungana, S. Kc, P. Basnet and R. Giri, Transition Metal–Catalyzed Dicarbofunctionalization of Unactivated Olefins, Chem. Rec., 2018, 18, 1314 CrossRef CAS;
(b) J. Derosa, O. Apolinar, T. Kang, V. T. Tran and K. M. Engle, Recent developments in nickel-catalyzed intermolecular dicarbofunctionalization of alkenes, Chem. Sci., 2020, 11, 4287 RSC;
(c) Y. Ping and W. Kong, Ni-Catalyzed Reductive Difunctionalization of Alkenes, Synthesis, 2020, 52, 979 CrossRef.
- For recent reviews on alkene difunctionalization, see:
(a) Z.-L. Li, G.-C. Fang, Q.-S. Gu and X.-Y. Liu, Recent advances in copper-catalysed radical-involved asymmetric 1,2-difunctionalization of alkenes, Chem. Soc. Rev., 2020, 49, 32 RSC;
(b) Y. Li, D. Wu, H. Cheng and G. Yin, Difunctionalization of Alkenes Involving Metal Migration, Angew. Chem., 2020, 59, 7990 CrossRef CAS;
(c) X. Qi and T. Diao, Nickel-Catalyzed Dicarbofunctionalization of Alkenes, ACS Catal., 2020, 10, 8542 CrossRef CAS;
(d) H. Jiang and A. Studer, Intermolecular radical carboamination of alkenes, Chem. Soc. Rev., 2020, 49, 1790 RSC.
-
(a) X.-W. Lan, N.-X. Wang and Y. Xing, Recent Advances in Radical Difunctionalization of Simple Alkenes, Eur. J. Org. Chem., 2017, 2017, 5821 CrossRef CAS;
(b) G. S. Sauer and S. Lin, An Electrocatalytic Approach to the Radical Difunctionalization of Alkenes, ACS Catal., 2018, 8, 5175 CrossRef CAS;
(c) X. Wang, Y. Han, X. Ouyang, R. Song and J. Li, The photoredox alkylarylation of styrenes with alkyl N-hydroxyphthalimide esters and arenes involving C–H functionalization, Chem. Commun., 2019, 55, 14637 RSC;
(d) X. Ouyang, Y. Li, R. Song, M. Hu, S. Luo and J. Li, Intermolecular dialkylation of alkenes with two distinct C(sp3)—H bonds enabled by synergistic photoredox catalysis and iron catalysis, Sci. Adv., 2019, 5, eaav9839 CrossRef CAS PubMed;
(e) S. Xu, H. Chen, Z. Zhou and W. Kong, Three–Component Alkene Difunctionalization by Direct and Selective Activation of Aliphatic C−H Bonds, Angew. Chem., Int. Ed., 2021, 60, 7405 CrossRef CAS;
(f) M. W. Campbell, M. Yuan, V. C. Polites, O. Gutierrez and G. A. Molander, Photochemical C–H Activation Enables Nickel-Catalyzed Olefin Dicarbofunctionalization, J. Am. Chem. Soc., 2021, 143, 3901 CrossRef CAS;
(g) J.-H. Qin, M.-J. Luo, D.-L. An and J.-H. Li, Electrochemical 1,2−Diarylation of Alkenes Enabled by Direct Dual C–H Functionalizations of Electron–Rich Aromatic Hydrocarbons, Angew. Chem., Int. Ed., 2021, 60, 1861 CrossRef CAS.
-
(a) W.-T. Wei, M.-B. Zhou, J.-H. Fan, W. Liu, R.-J. Song, Y. Liu, M. Hu, P. Xie and J.-H. Li, Synthesis of Oxindoles by Iron–Catalyzed Oxidative 1,2−Alkylarylation of Activated Alkenes with an Aryl C(sp2)—H Bond and a C(sp3)—H Bond Adjacent to a Heteroatom, Angew. Chem., Int. Ed., 2013, 52, 3638 CrossRef CAS;
(b) Y.-M. Li, M. Sun, H.-L. Wang, Q.-P. Tian and S.-D. Yang, Angew. Chem., Int. Ed., 2013, 52, 3972 CrossRef CAS PubMed;
(c) S.-L. Zhou, L.-N. Guo, H. Wang and X.-H. Duan, Copper–Catalyzed Oxidative Benzylarylation of Acrylamides by Benzylic C—H Bond Functionalization for the Synthesis of Oxindoles, Chem. – Eur. J., 2013, 19, 12970 CrossRef CAS;
(d) Y. Meng, L.-N. Guo, H. Wang and X.-H. Duan, Metal-free oxidative hydroxyalkylarylation of activated alkenes by direct sp3 C–H functionalization of alcohols, Chem. Commun., 2013, 49, 7540 RSC;
(e) H. Wang, L.-N. Guo and X.-H. Duan, Silver-catalyzed oxidative coupling/cyclization of acrylamides with 1,3-dicarbonyl compounds, Chem. Commun., 2013, 49, 10370 RSC;
(f) H. Wang, L.-N. Guo and X.-H. Duan, Metal-Free Oxidative Spirocyclization of Hydroxymethylacrylamide with 1,3-Dicarbonyl Compounds: A New Route to Spirooxindoles, Org. Lett., 2013, 15, 5254 CrossRef CAS;
(g) K. Matcha, R. Narayan and A. P. Antonchick, Metal–Free Radical Azidoarylation of Alkenes: Rapid Access to Oxindoles by Cascade C-N and C-C Bond–Forming Reactions, Angew. Chem., Int. Ed., 2013, 52, 7985 CrossRef CAS;
(h) P. Xu, J. Xie, Q. Xue, C. Pan, Y. Cheng and C. Zhu, Visible–Light–Induced Trifluoromethylation of N–Aryl Acrylamides: A Convenient and Effective Method To Synthesize CF3−Containing Oxindoles Bearing a Quaternary Carbon Center, Chem. – Eur. J., 2013, 19, 14039 CrossRef CAS;
(i) Y.-T. He, L.-H. Li, Y.-F. Yang, Y.-Q. Wang, J.-Y. Luo, X.-Y. Liu and Y.-M. Liang, Copper-catalyzed synthesis of trifluoromethyl-substituted isoxazolines, Chem. Commun., 2013, 49, 5687 RSC;
(j) T. Shen, Y. Yuan and N. Jiao, Metal-free nitro-carbocyclization of activated alkenes: a direct approach to synthesize oxindoles by cascade C–N and C–C bond formation, Chem. Commun., 2014, 50, 554 RSC;
(k) Z. Li, Y. Zhang, L. Zhang and Z.-Q. Liu, Free-Radical Cascade Alkylarylation of Alkenes with Simple Alkanes: Highly Efficient Access to Oxindoles via Selective (sp3)C–H and (sp2)C–H Bond Functionalization, Org. Lett., 2014, 16, 382 CrossRef CAS PubMed;
(l) F. Yin and X.-S. Wang, Silver-Mediated Radical Aryltrifluoromethylthiolaton of Activated Alkenes, Org. Lett., 2014, 16, 1128 CrossRef CAS;
(m) X. Xu, Y. Tang, X. Li, G. Hong, M. Fang and X. Du, Iron-Catalyzed Arylalkoxycarbonylation of N-Aryl Acrylamides with Carbazates, J. Org. Chem., 2014, 79, 446 CrossRef CAS PubMed;
(n) L. Li, M. Deng, S.-C. Zheng, Y.-P. Xiong, B. Tan and X.-Y. Liu, Metal-Free Direct Intramolecular Carbotrifluoromethylation of Alkenes to Functionalized Trifluoromethyl Azaheterocycles, Org. Lett., 2014, 16, 504 CrossRef CAS PubMed;
(o) W.-P. Mai, J.-T. Wang, L.-R. Yang, J.-W. Yuan, Y.-M. Xiao, P. Mao and L.-B. Qu, Silver-Catalyzed Radical Tandem Cyclization for the Synthesis of 3,4-Disubstituted Dihydroquinolin-2(1H)-ones, Org. Lett., 2014, 16, 204 CrossRef CAS;
(p) S.-L. Zhou, L.-N. Guo, S. Wang and X.-H. Duan, Copper-catalyzed tandem oxidative cyclization of cinnamamides with benzyl hydrocarbons through cross-dehydrogenative coupling, Chem. Commun., 2014, 50, 3589 RSC;
(q) X. Wu, G. Xiao, Y. Ding, Y. Zhan, Y. Zhao, R. Chen and T.-P. Loh, Palladium-Catalyzed Intermolecular Polarity-Mismatched Addition of Unactivated Alkyl Radicals to Unactivated Alkenes, ACS Catal., 2020, 10, 14107 CrossRef CAS;
(r) B. Cai, H. W. Cheo, T. Liu and J. Wu, Light–Promoted Organic Transformations Utilizing Carbon–Based Gas Molecules as Feedstocks, Angew. Chem., Int. Ed., 2021, 60, 18950 CrossRef CAS.
-
(a) Z. Chen, B. Wang, J. Zhang, W. Yu, Z. Liu and Y. Zhang, Transition metal-catalyzed C–H bond functionalizations by the use of diverse directing groups, Org. Chem. Front., 2015, 2, 1107 RSC;
(b) L.-N. Guo, H. Wang and X.-H. Duan, Recent advances in catalytic decarboxylative acylation reactions via a radical process, Org. Biomol. Chem., 2016, 14, 7380 RSC;
(c) F. Penteado, E. F. Lopes, D. Alves, G. Perin, R. G. Jacob and E. J. Lenardão, α-Keto Acids: Acylating Agents in Organic Synthesis, Chem. Rev., 2019, 119, 7113 CrossRef CAS.
-
(a) J.-Q. Shang, X.-X. Wang, Y. Xin, Y. Li, B. Zhou and Y.-M. Li, Decarboxylative cascade cyclization of α-keto acids with 2-cyano-3-arylaniline-derived acrylamides, Org. Biomol. Chem., 2019, 17, 9447 RSC;
(b) F. Zhou, L. Li, K. Lin, F. Zhang, G.-J. Deng and H. Gong, Iron–Catalyzed Cleavage Reaction of Keto Acids with Aliphatic Aldehydes for the Synthesis of Ketones and Ketone Esters, Chem. – Eur. J., 2020, 26, 4246 CrossRef CAS PubMed;
(c) C. R. Reddy, D. H. Kolgave, M. Subbarao, M. Aila and S. K. Prajapti, Ag-Catalyzed Oxidative ipso-Cyclization via Decarboxylative Acylation/Alkylation: Access to 3-Acyl/Alkyl-spiro[4.5]trienones, Org. Lett., 2020, 22, 5342 CrossRef CAS PubMed;
(d) P. Bao, F. Liu, Y. Lv, H. Yue, J.-S. Li and W. Wei, Visible-light-promoted acridine red catalyzed aerobic oxidative decarboxylative acylation of α-oxo-carboxylic acids with quinoxalin-2(1H)-ones, Org. Chem. Front., 2020, 7, 492 RSC;
(e) J. Lu, X.-K. He, X. Cheng, A.-J. Zhang, G.-Y. Xu and J. Xuan, Photoredox Catalyst Free, Visible Light–Promoted C3−H Acylation of Quinoxalin–2(1H)–ones in Water, Adv. Synth. Catal., 2020, 362, 2178 CrossRef;
(f) Z. Gan, G. Li, X. Yang, Q. Yan, G. Xu, G. Li, Y.-Y. Jiang and D. Yang, Visible-light-induced regioselective cross-dehydrogenative coupling of 2-isothiocyanatonaphthalenes with amines using molecular oxygen, Sci. China: Chem., 2020, 63, 1652 CrossRef CAS;
(g) L.-Y. Xie, Y.-S. Bai, X.-Q. Xu, X. Peng, H.-S. Tang, Y. Huang, Y.-W. Lin, Z. Cao and W.-M. He, Visible-light-induced decarboxylative acylation of quinoxalin-2(1H)-ones with α-oxo carboxylic acids under metal-, strong oxidant- and external photocatalyst-free conditions, Green Chem., 2020, 22, 1720 RSC;
(h) G. Xie, Y. Zhao, C. Cai, G.-J. Deng and H. Gong, Palladium-Catalyzed Direct and Specific C-7 Acylation of Indolines with 1,2-Diketones, Org. Lett., 2021, 23, 410 CrossRef CAS;
(i) H.-L. Zhu, F.-L. Zeng, X.-L. Chen, K. Sun, H.-C. Li, X.-Y. Yuan, L.-B. Qu and B. Yu, Acyl Radicals from α-Keto Acids: Metal-Free Visible-Light-Promoted Acylation of Heterocycles, Org. Lett., 2021, 23, 2976 CrossRef CAS PubMed.
-
(a) S. E. Wolkenberg and D. L. Boger, Mechanisms of in Situ Activation for DNA-Targeting Antitumor Agents, Chem. Rev., 2002, 102, 2477 CrossRef CAS PubMed;
(b) U. Galm, M. H. Hager, S. G. Van Lanen, J. Ju, J. S. Thorson and B. Shen, Antitumor Antibiotics: Bleomycin, Enediynes, and Mitomycin, Chem. Rev., 2005, 105, 739 CrossRef CAS.
-
(a) M. Tanaka, S. Sagawa, J.-I. Hoshi, F. Shimoma, K. Yasue, M. Ubukata, T. Ikemoto, Y. Hase, M. Takahashi, T. Sasase, N. Ueda and M. Matsushita, Synthesis, SAR studies, and pharmacological evaluation of 3-anilino-4-(3-indolyl) maleimides with conformationally restricted structure as orally bioavailable PKCβ-selective inhibitors, Bioorg. Med. Chem., 2006, 14, 5781 CrossRef CAS PubMed;
(b) R. Kakadiya, H. Dong, P.-C. Lee, N. Kapuriya, X. Zhang, T.-C. Chou, T.-C. Lee, K. Kapuriya, A. Shah and T.-L. Su, Potent antitumor bifunctional DNA alkylating agents, synthesis and biological activities of 3a-aza-cyclopenta[a]indenes, Bioorg. Med. Chem., 2009, 17, 5614 CrossRef CAS PubMed;
(c) R. Vallakati and A. May, Biomimetic Synthesis of the Antimalarial Flindersial Alkaloids, J. Am. Chem. Soc., 2012, 134, 6936 CrossRef CAS PubMed.
-
(a) G. A. Elmegeed, A. R. Baiuomy and O. M. E. Abdel-Salam, Evaluation of the anti-inflammatory and anti-nociceptive activities of novel synthesized melatonin analogues, Eur. J. Med. Chem., 2007, 42, 1285 CrossRef CAS PubMed;
(b) J.-F. Liu, Z.-Y. Jiang, R.-R. Wang, Y.-T. Zheng, J.-J. Chen, X.-M. Zhang and Y.-B. Ma, Isatisine A, a Novel Alkaloid with an Unprecedented Skeleton from Leaves of Isatis indigotica, Org. Lett., 2007, 9, 4127 CrossRef CAS;
(c) L. S. Fernandez, M. S. Buchanan, A. R. Carroll, Y. J. Feng, R. J. Quinn and V. M. Avery, Flinderoles A−C: Antimalarial Bis-indole Alkaloids from Flindersia Species, Org. Lett., 2009, 11, 329 CrossRef CAS PubMed.
-
(a) R. K. Thalji, J. A. Ellman and R. G. Bergman, Highly Efficient and Enantioselective Cyclization of Aromatic Imines via Directed C-H Bond Activation, J. Am. Chem. Soc., 2004, 126, 7192 CrossRef CAS PubMed;
(b) G. Abbiati, A. Casoni, V. Canevari, D. Nava and E. Rossi, TiCl4/t-BuNH2-Promoted Hydroamination/Annulation of δ-Keto-acetylenes:Synthesis of Novel Pyrrolo[1,2-a]indol-2-carbaldehydes, Org. Lett., 2006, 8, 4839 CrossRef CAS PubMed;
(c) H. Ren, Z. Li and P. Knochel, Chemoselective C(sp3)-H Bond Activation for the Preparation of Condensed N–Heterocycles, Chem. – Asian J., 2007, 2, 416 CrossRef CAS PubMed;
(d) S. J. Hwang, S. H. Cho and S. Chang, Synthesis of Condensed Pyrroloindoles via Pd-Catalyzed Intramolecular C−H Bond Functionalization of Pyrroles, J. Am. Chem. Soc., 2008, 130, 16158 CrossRef CAS;
(e) N. Saleh and A. Voituriez, Synthesis of 9H-Pyrrolo[1,2-a]indole and 3H-Pyrrolizine Derivatives via a Phosphine-Catalyzed Umpolung Addition/Intramolecular Wittig Reaction, J. Org. Chem., 2016, 81, 4371 CrossRef CAS PubMed;
(f) S. Chen, P. Zhang, W. Shu, Y. Gao, G. Tang and Y. Zhao, Cascade Phosphinoylation/Cyclization/Isomerization Process for the Synthesis of 2-Phosphinoyl-9H-pyrrolo[1,2-a]indoles, Org. Lett., 2016, 18, 5712 CrossRef CAS PubMed;
(g) H. Zhang, W. Li and C. Zhu, Copper-Catalyzed Cascade Phosphorylation Initiated Radical Cyclization: Access to 2-Phosphorylated Pyrrolo[1,2-a]indole, J. Org. Chem., 2017, 82, 2199 CrossRef CAS PubMed;
(h) X. Y. Zhu, M. Li, Y. P. Han, S. Chen, X. S. Li and Y. M. Liang, Copper-Catalyzed Oxidative Cyclization of Alkynes with Sulfonylhydrazides Leading to 2-Sulfonated 9H-pyrrolo[1,2-a]indol-9-ones, J. Org. Chem., 2017, 82, 8761 CrossRef CAS PubMed;
(i) S. J. Gharpure and Y. G. Shelke, Cascade Radical Cyclization of N-Propargylindoles: Substituents Dictate Stereoselective Formation of N-Fused Indolines versus Indoles, Org. Lett., 2017, 19, 5022 CrossRef CAS;
(j) J. Zhu, S. Sun, M. Xia, N. Gu and J. Cheng, Copper-catalyzed radical 1,2-cyclization of indoles with arylsulfonyl hydrazides: access to 2-thiolated 3H-pyrrolo[1,2-a]indoles, Org. Chem. Front., 2017, 4, 2153 RSC;
(k) X.-Y. Zhu, Y.-P. Han, M. Li, X.-S. Li and Y.-M. Liang, Copper–Catalyzed Radical Sulfonylation of N–Propargylindoles with Concomitant 1,2−Aryl Migration, Adv. Synth. Catal., 2018, 360, 3460 CrossRef CAS;
(l) L. Bai, J. Liu, W. Hu, K. Li, Y. Wang and X. Luan, Palladium/Norbornene–Catalyzed C−H Alkylation/Alkyne Insertion/Indole Dearomatization Domino Reaction: Assembly of Spiroindolenine–Containing Pentacyclic Frameworks, Angew. Chem., Int. Ed., 2018, 57, 5151 CrossRef CAS;
(m) C. Lorton and A. Voituriez, Phosphine-Promoted Synthesis of 9H-Pyrrolo[1,2-a]indole Derivatives via an γ-Umpolung Addition/Intramolecular Wittig Reaction, J. Org. Chem., 2018, 83, 5801 CrossRef CAS PubMed;
(n) H. E. Ho, T. C. Stephens, T. J. Payne, P. O'Brien, R. J. K. Taylor and W. P. Unsworth, Merging π-Acid and Pd Catalysis: Dearomatizing Spirocyclization/Cross-Coupling Cascade Reactions of Alkyne-Tethered Aromatics, ACS Catal., 2019, 9, 504 CrossRef CAS;
(o) H. Chen, M. Liu, G. Qiu and J. Wu, A Three–Component Reaction of Aryldiazonium Tetrafluoroborates, Sulfur Dioxide, and 1−(Prop–2−yn–1−yl)indoles under Catalyst–Free Conditions, Adv. Synth. Catal., 2019, 361, 146 CrossRef CAS;
(p) P. Zhang, S. Shi, X. Gao, S. Han, J. Lin and Y. Zhao, Photoredox-catalyzed cascade annulation of N-propargylindoles with sulfonyl chlorides: access to 2-sulfonated 9H-pyrrolo[1,2-a]indoles, Org. Biomol. Chem., 2019, 17, 2873 RSC;
(q) X. Xie, P. Li and L. Wang, Synthesis of 2−Sulfonated–9H–Pyrrolo[1,2−a]indoles via a Ag–Promoted Cascade Sulfonation and Cyclization, Eur. J. Org. Chem., 2019, 221 CrossRef CAS;
(r) C. R. Reddy, R. C. Kajare and N. Punna, Silver-catalyzed acylative annulation of N-propargylated indoles with α-keto acids: access to acylated pyrrolo[1,2-a]indoles, Chem. Commun., 2020, 56, 3445 RSC.
-
(a) D. V. Patil, M. A. Cavitt and S. France, Diastereoselective Intramolecular Friedel–Crafts Cyclizations of Substituted Methyl 2-(1H-indole-1-carbonyl)acrylates: Efficient Access to Functionalized 1H-Pyrrolo[1,2-a]indoles, Org. Lett., 2011, 13, 5820 CrossRef CAS PubMed;
(b) J. Xu, X. Yu and Q. Song, Silver-Catalyzed Radical-Involved Cascade Cyclization of Diphenylphosphine with Cinnamamides: Access to 2-Phosphinoyl-3H-pyrrolo[1,2-a]indoles, Org. Lett., 2017, 19, 980 CrossRef CAS;
(c) J. Liu, S. Zhao, W. Song, R. Li, X. Guo, K. Zhuo and Y. Yue, Silver–Catalyzed Carbon–Phosphorus Functionalization for Polyheterocycle Formation, Adv. Synth. Catal., 2017, 359, 609 CrossRef CAS;
(d) Z. Cui and D.-M. Du, FeCl2-Catalyzed Decarboxylative Radical Alkylation/Cyclization of Cinnamamides: Access to Dihydroquinolinone and Pyrrolo[1,2-a]indole Analogues, J. Org. Chem., 2018, 83, 5149 CrossRef CAS PubMed.
-
(a) J. Fan, S. Wang, J. Chen, M. Wu, J. Zhang and M. Xie, Synthesis of 2-acetyl trisubstituted furans via copper-mediated deacylation cleavage of unstrained C(sp3)–C(sp2) bonds, Org. Chem. Front., 2019, 6, 437 RSC;
(b) C. Liu, B. Wang, Z. Guo, J. Zhang and M. Xie, Metal-free cascade rearrangement/radical addition/oxidative C–H annulation of propargyl alcohols with sodium sulfinates: access to 2-sulfenylindenones, Org. Chem. Front., 2019, 6, 2796 RSC;
(c) J. Fan, L. Li, J. Zhang and M. Xie, Expeditious synthesis of phenanthridines through a Pd/MnO2-mediated C–H arylation/oxidative annulation cascade from aldehydes, aryl iodides and amino acids, Chem. Commun., 2020, 56, 2775 RSC.
-
(a) J. Zhang, M. Wu, J. Fan, Q. Xu and M. Xie, Selective C–H acylation of indoles with α-oxocarboxylic acids at the C4 position by palladium catalysis, Chem. Commun., 2019, 55, 8102 RSC;
(b) J. Zhang, Q. Xu, J. Wu, J. Fan and M. Xie, Construction of N–C Axial Chirality through Atroposelective C–H Olefination of N-Arylindoles by Palladium/Amino Acid Cooperative Catalysis, Org. Lett., 2019, 21, 6361 CrossRef CAS PubMed.
-
(a) S. Seo, M. Slater and M. F. Greaney, Decarboxylative C-H Arylation of Benzoic Acids under Radical Conditions, Org. Lett., 2012, 14, 2650 CrossRef CAS PubMed;
(b) L. Cui, H. Chen, C. Liu and C. Li, Silver-Catalyzed Decarboxylative Allylation of Aliphatic Carboxylic Acids in Aqueous Solution, Org. Lett., 2016, 18, 2188 Search PubMed;
(c) W. Wu, S. Yi, W. Huang, D. Luo and H. Jiang, Ag-Catalyzed Oxidative Cyclization Reaction of 1,6-Enynes and Sodium Sulfinate: Access to Sulfonylated Benzofurans, Org. Lett., 2017, 19, 2825 CrossRef CAS PubMed;
(d) Y. Gu, L. Dai, J. Zhang, X. Lu, X. Liu, C. Wang, J. Zhang and L. Rong, Silver-Catalyzed Radical Cascade Sulfonation/Cycloaddition for the Construction of Multifunctional Succimides Containing Separable Z/E–Isomers, J. Org. Chem., 2021, 86, 2173 CrossRef CAS PubMed.
- CCDC 1965912 contains the supplementary crystallographic data for this paper.†.
-
(a) H.-X. Zou, Y. Li, Y. Yang, J.-H. Li and J. Xiang, Silver–Catalyzed Decarboxylative Couplings of Acids and Anhydrides: An Entry to 1,2−Diketones and Aryl–Substituted Ethanes, Adv. Synth. Catal., 2018, 360, 1439 CrossRef CAS;
(b) M. Meng, G. Wang, L. Yang, K. Cheng and C. Qi, Silver–catalyzed Double Decarboxylative Radical Alkynylation/Annulation of Arylpropiolic Acids with α–keto Acids: Access to Ynones and Flavones under Mild Conditions, Adv. Synth. Catal., 2018, 360, 1218 CrossRef CAS.
Footnotes |
† Electronic supplementary information (ESI) available: Experimental procedures, characterization data, and 1H and 13C NMR charts. CCDC 1965912. For ESI and crystallographic data in CIF or other electronic format see DOI: 10.1039/d1qo01069g |
‡ These three authors contributed equally to this work. |
|
This journal is © the Partner Organisations 2022 |
Click here to see how this site uses Cookies. View our privacy policy here.