DOI:
10.1039/D1QO01313K
(Research Article)
Org. Chem. Front., 2022,
9, 140-146
Gold self-relay catalysis for accessing functionalized cyclopentenones bearing an all-carbon quaternary stereocenter†
Received
3rd September 2021
, Accepted 17th November 2021
First published on 18th November 2021
Abstract
A new gold(I) self-relay catalysis consisting of a 3,3-rearrangement, Nazarov cyclization and Michael addition cascade of 1,3-enyne acetates with aurones and their derived azadienes is reported, and used to produce a series of densely functionalized cyclopentenones bearing a cyclic quaternary stereocenter in moderate to good yields under mild conditions. This tandem protocol demonstrates high regioselectivity, broad substrate flexibility and good functional group tolerance of substrates without inert atmosphere protection, providing a catalytic and convergent approach for creating all-carbon stereoscopic centers.
Introduction
Five-membered carbocycles exhibit a basic structural scaffold that frequently occurs in a myriad of natural products and synthetic bioactive molecules.1 Specifically abundant among these carbocycles are those incorporating a cyclopentenone skeleton, a privileged structure that represents the core of many natural products2 exhibiting potent biological activities, as exemplified by litseaverticillol A,3a (−)-nigrosporiones C and D,3b (−)-kjellmanianone,3c untenone A,3d and (−)-pentenomycins3e (Fig. 1). Furthermore, cyclopentenone frameworks are used as key intermediates to prepare some natural products and pharmaceuticals in total synthesis4 due to the broad diversity of chemical modifications available for the enone unit motif. Moreover, scaffolds with quaternary stereocenters are prevalent in a wide assortment of natural products, bioactive molecules, and pharmaceuticals as well as agrochemical ingredients.5 In recent years, great progress has been made in strategies for the construction of cyclopentenone skeletons with quaternary stereocenters.2 The vast majority of the catalytic syntheses of these molecules involve Pauson–Khand reactions,6 Nazarov cyclizations,7 Rautenstrauch Rearrangements,8 organocatalyzed reactions,9 functionalizations of the existing cyclopentenone unit10 and annulations of acyclic substrates.11 Although formidable advances have been made, the continuous development of catalytic and versatile methods that enable the direct generation of new functionalized cyclopentenones with three-dimensionality could have a major impact on the discovery of new pharmaceuticals.
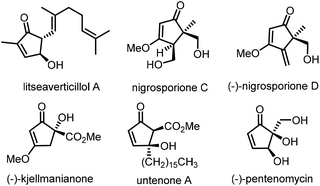 |
| Fig. 1 Cyclopentenone-containing natural products. | |
Homogeneous gold catalysis has attracted long-standing attention in the field of organic synthesis.12 This is attributed to the fact that such catalysis could fabricate functional molecules not obtained from other approaches, along with an array of attractive features including its high catalytic capabilities, high levels of regioselectivity and good functional group tolerance.13 Recently, the Zhang group pioneered the gold-catalyzed [3,3]-sigmatropic rearrangement/Nazarov cyclization of nucleophilic 1,3-enyne acetates toward cyclopentenones (Scheme 1a).14 In this direction, Rao and co-workers exquisitely demonstrated the gold-catalyzed intermolecular trapping of 1,3-enyne acetates with electrophilic halogenation for halogenated cyclopentenone synthesis (Scheme 1b).15 Inspired by these elegant advances and combined with our research interest in enyne transformations,16 we envisaged that the in situ generated nucleophilic cyclopentadiene intermediates from 1,3-enyne acetates 1 might add to Michael acceptors in the presence of a gold complex to forge the quaternary stereocenter. Accordingly, such an approach could merge 3,3-rearrangement and Nazarov cyclization with Michael addition to develop a new gold self-relay catalysis.17,18 Herein, we elaborate a conceptually new gold self-relay catalysis consisting of a 3,3-rearrangement, Nazarov cyclization and Michael addition cascade that demonstrates convergent and practical access to new C(sp3)-tethered cyclopentenone–benzofuran pairs from readily available 1,3-enyne acetates and aurones and their derived 1-azadienes in a one-pot manner (Scheme 1c). Interestingly, the gold-catalyzed reaction of 1,3-enyne acetates with aurones features 100% atomic economy.19
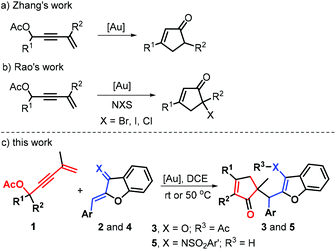 |
| Scheme 1 Gold-catalyzed synthesis of functional cyclopentenones. | |
To uncover an effective catalytic system for adding nucleophilic 1,3-enyne acetates into aurones, the reaction between 1,3-enyne acetate 1a and aurone 2a was investigated. A survey of reaction conditions is displayed in Table 1. The AuCl-catalyzed reaction in DCE at 50 °C produced the cyclopentanone product 3a in 39% yield through a Nazarov cyclization/Michael addition cascade (Table 1, entry 1). However, both AuCl3 and IPrAuCl as catalysts did not catalyze the formation of product 3a (entries 2 and 3). In sharp contrast, the use of IPrAuNTf2 and JohnPhosAu(MeCN)SbF6 could facilitate this process and provide a higher yield of the desired product (entries 4 and 5), in which the latter demonstrated the best catalytic capability, leading to 64% yield. This reaction proceeded readily at 50 °C: reactions at lower temperatures did not proceed to completion (entry 6), and higher temperatures showed no advantage (entry 7). The next step of our study was the determination of the solvent effect in the process by exploiting several other aprotic solvents such as 1,4-dioxane, N,N-dimethylformamide (DMF), tetrahydrofuran (THF), acetone, and toluene (entries 8–12). After careful screening, it is found that the use of the former three solvents remained almost ineffective, as only a trace amount of product 3a was detected (entries 8–10); whereas the latter two could drive the transformation, but led to a decrease in the efficiency as compared with DCE (entries 11 and 12).
Table 1 Condition optimization for product 3a
a
With the optimized conditions in hand, a set of 1,3-enyne acetates 1 and aurones 2 were prepared and exploited to systematically investigate the generalization and limitation of this gold-catalysis reaction. The results are presented in Scheme 2. First, the influence of the electronic properties and positions of substituents linked to the arene ring of 1,3-enyne acetates was determined by repeating the reaction with aurone 2a. The results showed that both electron-donating (e.g., methyl, 1b–1d) and electron-withdrawing (e.g., chloro, 1e–1g) R1 groups at different positions (ortho, meta, or para) demonstrated good compatibility of this transformation, and all these substituents were converted into the corresponding products 3b–3g in good yields with 1.4
:
1 to >19
:
1 dr. Of these functional groups, sterically encumbered o-methyl and o-chlorophenyl counterparts proved to be suitable for this protocol. Besides, the unsaturated styryl partner 1h was found to be compatible with the present catalytic conditions, accessing product 3h with 49% yield. Remarkably, various alkyl substituted substrates such as benzyl (1i), tert-butyl (1j), cyclohexyl (1k) and n-heptyl (1l) were accommodated, confirming the efficiency of the reaction, as products 3i–3l were generated with good yields, albeit with the observation of low diastereoselectivity. Next, a brief survey of the possible variation in the arene ring of substrates 2 was conducted. The reactions of various aurones 2 with o-tolyl substrate 1b as a representative 1,3-enyne ester were carried out under the standard conditions. Various substituents such as bromo (2b–2d) and methyl (2e–2g) were independently incorporated in the different positions of the phenyl ring in substrates 2. All these substituents were successfully engaged in this reaction, giving access to the desired products 3m–3r with comparable efficiency. As exemplified by substrate 2h, this gold catalysis is also well-tolerated with the aurone linked by a heteroarene such as thiophene in 84% yield with 1.1
:
1 dr. Cyclic ketone-derived 1,3-enyne acetates such as cyclobutyl (1m) and cyclopentyl (1n) proved to be effective for this transformation, as demonstrated by the formation of products 3t and 3u with good yields through the gold-catalyzed C–C σ bond cleavage of the cyclic ring.
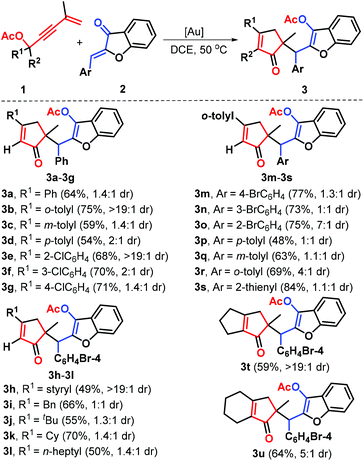 |
| Scheme 2 Substrate scope for the synthesis of products 3. Reaction conditions: 1,3-Enyne acetates 1 (0.2 mmol) with aurones 2 (0.2 mmol), JohnPhosAu(MeCN)SbF6 (2 mol%), DCE (2.5 mL), 50 °C for 20 h. The Dr ratio based on 1H NMR analysis. | |
Next, the scope of this gold self-relay catalysis reaction was carefully evaluated by exploiting a variety of aurone-derived azadienes 4 as Michael acceptors (Scheme 3). The reactions of 1,3-enyne acetates 1 with N-Ts protected azadienes 4 proceeded well when the reaction temperature was reduced to room temperature. Different substituents such as aryl, styryl and alkyl tethered by 1,3-enyne acetates did not hamper this reaction process, furnishing the target products 5a–5j with acceptable yields and diastereoselectivity. Cyclic ketone-derived 1,3-enyne acetates such as cyclobutyl and cyclopentyl were also highly reactive in this catalytic transformation (5k and 5l). Most importantly, this chemistry also tolerated naturally derived 1,3-enyne acetates 1 including estrone (1o) and epiandrosterone (1p), enabling gold-catalyzed ring expansion to access the corresponding products 5m and 5n in good yields, thus highlighting the versatility of this approach in the construction of molecular complexity via gold catalysis. The presence of other N-protections such as 4-methoxy- (4b) and 4-(tert-butyl)phenyl (4c) linked to the azadiene unit was also compatible, and the products 5o and 5p were produced with high efficiency. The subsequent change of the aryl group (Ar) relative to the azadiene unit showed that both the bromo and methyl groups at para-, meta- and ortho-positions could participate in the transformation, accessing products 5q–5v with good yields and moderate to high diastereoselectivity. The resultant cyclopentenones were fully confirmed by NMR spectroscopy and HRMS data. Furthermore, the stereo-structures of 3b and 5a were characterized by X-ray diffraction analysis (Fig. 2 and 3).
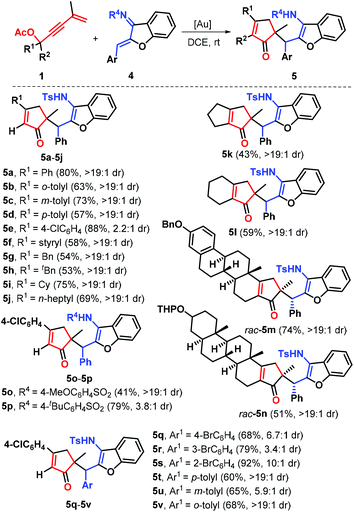 |
| Scheme 3 Substrate scope for the synthesis of products 5. Reaction conditions: 1,3-Enyne acetates 1 (0.2 mmol) with aurone-derived azadienes 4 (0.2 mmol), JohnPhosAu(MeCN)SbF6 (2 mol%), DCE (2.5 mL), rt for 6.0 h. The Dr ratio based on 1H NMR analysis. | |
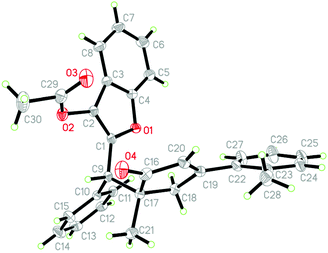 |
| Fig. 2 The ORTEP drawing of 3b. | |
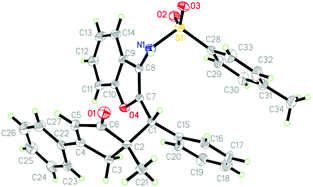 |
| Fig. 3 The ORTEP drawing of 5a. | |
To gain mechanistic insight into this gold-catalysis, several control experiments were performed as described in Scheme 4. In Li's procedure, gold-catalyzed Nazarov cyclization of 1,3-enyne acetates could give two different cyclic products of cyclopentenones and unstable cyclopentadienes. To evaluate the possibility of intermediates for this gold self-relay catalysis, the gold-catalyzed reaction of 1a was conducted under standard conditions. After 1 hour, 2a was added into this reaction system, and cyclopentenones 6a and 2a were isolated without the formation of the target (Scheme 4a). Next, the preformed cyclopentadiene 6b was reacted with 2a, leading to 51% yield of product 3a (Scheme 4b). These outcomes revealed that cyclopentadienes should be the great possibility of reaction intermediates, rather than cyclopentenones. Without gold catalysts, the reaction of 6b with 2a did not take place (Scheme 4c), showing that the gold catalyst is crucial for every elementary reaction step and thus a new gold self-relay catalysis involving Nazarov cyclization and Michael addition was established. After that, a crossover experiment of 1a and 1,3-enyne propionate 1q with 2a was performed under standard conditions, delivering four different substituted cyclopentenones that were detected by HRMS analysis (Scheme 4d), demonstrating that both intramolecular and intermolecular acyl transfer may be involved in the transformation.
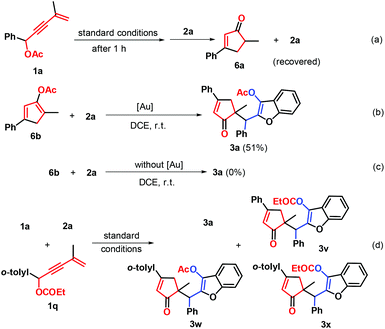 |
| Scheme 4 Control experiments. | |
Based on the above experimental results and the literature precedents, a plausible explanation of the reaction outcome is depicted in Scheme 5. Initially, when 1,3-enyne ester 1a was treated with JohnPhosAu(MeCN)SbF6 in DCE, coordination with the triple bond gives gold complex A, in which the carbonyl group from the ester involves in activating the bond, followed by a [3,3]-rearrangement to yield the cationic pentadienylic gold species B.17 Intermediate B undergoes an electrocyclic Nazarov cyclization to access the gold carbene complex C, which enables the 1,2-hydride migration and deauration sequence to furnish cyclopentadiene 6a and release the gold catalyst. Next, the coordination of the gold complex to aurone 2a provides the Au(I) σ-complex E, trapped by cyclopentadiene 6b to generate intermediate F through Michael addition. Intramolecular acyl transfer affords intermediate H, which then results in the expected product 3a, together with the regeneration of the gold catalyst for the next catalytic cycle. Besides, intermolecular acyl transfer could also give product 3a. Therefore, the generation of 3a included a tandem reaction catalyzed by the same gold complex (self-relay catalysis). In the overall process, the gold catalyst played the dual role of π-Lewis acid-type activation of the triple bond for Nazarov cyclization and σ-Lewis acid-type activation of ketones for Michael addition. This case represents a new example of gold-catalyzed self-relay catalysis.
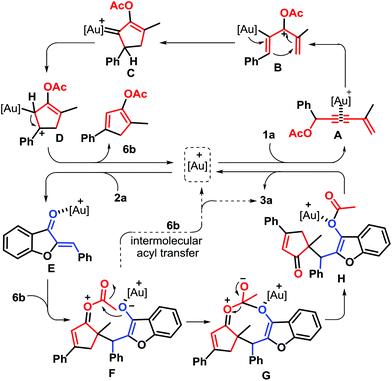 |
| Scheme 5 Proposed reaction mechanism. | |
Conclusions
In summary, a new self-relay gold-catalyzed tandem 3,3-rearrangement/Nazarov cyclization/Michael addition has been accomplished by using easily preformed 1,3-enyne acetates and aurones and their derived azadienes. The overall process, which took advantage of and unifies the π- and σ-Lewis acid capability of gold complexes, furnished a new family of synthetically important C(sp3)-tethered cyclopentenone–benzofuran pairs, an important structural modification pattern for several natural products. The current catalytic strategy features high regioselectivity, high functional group tolerance and mild conditions. Furthermore, the reaction proceeded smoothly without any inert atmosphere protection, thus highlighting the good oxygen resistance and simple operation process. Further application of gold(I) self-relay catalysis in assembling functionalized molecules of biological importance is underway in our laboratory.
Conflicts of interest
There are no conflicts to declare.
Acknowledgements
We are grateful for financial support from the National Natural Science Foundation of China (no. 21971090) and the Top-Notch Academic Programs Project of Jiangsu Higher Education Institution (TAPP).
Notes and references
- For selected reviews, see:
(a) X.-Z. Shi, A. Sasmal and J. F. Soulé, Metal-Catalyzed C-H Bond Activation of 5-Membered Carbocyclic Rings: A Powerful Access to Azulene, Acenaphthylene and Fulvene Derivatives, Chem. – Asian J., 2018, 13, 143–157 CrossRef CAS PubMed
;
(b) S. Mondal, S. R. Yetra, S. Mukherjee and A. T. Biju, NHC-Catalyzed Generation of α,β-Unsaturated Acylazoliums for the Enantioselective Synthesis of Heterocycles and Carbocycles, Acc. Chem. Res., 2019, 52, 425–436 CrossRef CAS PubMed
;
(c) T. Itoh, T. Nokami and M. Kawatsura, Recent Progress on Nazarov Cyclizations: The Use of Iron Salts as Catalysts in Ionic Liquid Solvent Systems, Chem. Rec., 2016, 16, 1676–1689 CrossRef CAS PubMed
.
- S. P. Simeonov, J. P. M. Nunes, K. Guerra, V. B. Kurteva and C. A. M. Afonso, Synthesis of Chiral Cyclopentenones, Chem. Rev., 2016, 116, 5744–5893 CrossRef CAS
.
-
(a) G. Vassilikogiannakis and M. Stratakis, Biomimetic Total Synthesis of Litseaverticillols A, C, D, F, and G: Singlet-Oxygen-Initiated Cascades, Angew. Chem., Int. Ed., 2003, 42, 5465–5626 CrossRef CAS
;
(b) Z.-H. Wu, Z.-H. Xie, M.-L. Wu, X.-Q. Li, W.-L. Li, W.-J. Ding, Z.-G. She and C.-Y. Li, New Antimicrobial Cyclopentenones from Nigrospora sphaerica ZMT05, a Fungus Derived from Oxya chinensis Thunber, J. Agric. Food Chem., 2018, 66, 5368–5372 CrossRef CAS
;
(c) J. Christoffers, T. Werner, W. Frey and A. Baro, Straightforward Synthesis of (R)-(−)-Kjellmanianone, Chem. – Eur. J., 2004, 10, 1042–1045 CrossRef CAS
;
(d) Q.-W. Gui, J. Wang, S. Ng, A. Dancevic, T. B. Wright and P. A. Evans, Copper-catalyzed desymmetrization of prochiral 4,4-disubstituted cyclopentenes via a site-selective allylic oxidation: a concise total synthesis of untenone A, Chem. Commun., 2019, 55, 12368–12371 RSC
;
(e) B. S. Kumar, G. P. Mishra and B. V. Rao, Synthesis of (+)/(−) pentenomycins via Me2AlCl induced cascade reaction, Tetrahedron Lett., 2013, 54, 2845–2848 CrossRef CAS
.
-
(a) A. J. H. Klunder, J. Zhu and B. Zwanenburg, The Concept of Transient Chirality in the Stereoselective Synthesis of Functionalized Cycloalkenes Applying the Retro-Diels-Alder Methodology, Chem. Rev., 1999, 99, 1163–1190 CrossRef CAS
;
(b) B. B. Touré and D. G. Hall, Natural Product Synthesis Using Multicomponent Reaction Strategies, Chem. Rev., 2009, 109, 4439–4486 CrossRef
;
(c) S. Poplata, A. Tröster, Y.-Q. Zou and T. Bach, Recent Advances in the Synthesis of Cyclobutanes by Olefin [2+2] Photocycloaddition Reactions, Chem. Rev., 2016, 116, 9748–9815 CrossRef CAS PubMed
.
- X.-P. Zeng, Z.-Y. Cao, Y.-H. Wang, F. Zhou and J. Zhou, Catalytic Enantioselective Desymmetrization Reactions to All-Carbon Quaternary Stereocenters, Chem. Rev., 2016, 116, 7330–7396 CrossRef CAS
.
-
(a) Z. Huang, J. Huang, Y. Qu, W. Zhang, J. Gong and Z. Yang, Total Syntheses of Crinipellins Enabled by Cobalt-Mediated and Palladium-Catalyzed Intramolecular Pauson-Khand Reactions, Angew. Chem., Int. Ed., 2018, 57, 8744–8748 CrossRef CAS PubMed
;
(b) A. G. Gallagher, H. Tian, O. A. Torres-Herrera, S. Yin, A.-X. Xie, D. M. Lange, J. K. Wilson, L. G. Mueller, M. R. Gau, P. J. Carroll and D. Martinez-Solorio, Access to Highly Functionalized Cyclopentenones via Diastereoselective Pauson-Khand Reaction of Siloxy-Tethered 1,7-Enynes, Org. Lett., 2019, 21, 8646–8651 CrossRef CAS
;
(c) Y. Chang, L. Shi, J. Huang, L. Shi, Z.-C. Zhang, H.-D. Hao, J.-X. Gong and Z. Yang, Stereoselective Total Synthesis of (±)-5-epi-Cyanthiwigin I via an Intramolecular Pauson-Khand Reaction as the Key Step, Org. Lett., 2018, 20, 2876–2879 CrossRef CAS PubMed
.
-
(a) J. Cao, M.-Y. Hu, S.-Y. Liu, X.-Y. Zhang, S.-F. Zhu and Q.-L. Zhou, Enantioselective Silicon-Directed Nazarov Cyclization, J. Am. Chem. Soc., 2021, 143, 6962–6968 CrossRef CAS
;
(b) K. Komatsuki, Y. Sadamitsu, K. Sekine, K. Saito and T. Yamada, Stereospecific Decarboxylative Nazarov Cyclization Mediated by Carbon Dioxide for the Preparation of Highly Substituted 2-Cyclopentenones, Angew. Chem., Int. Ed., 2017, 56, 11594–11598 CrossRef CAS
;
(c) A. K. Basak, N. Shimada, W. F. Bow, D. A. Vicic and M. A. Tius, An Organocatalytic Asymmetric Nazarov Cyclization, J. Am. Chem. Soc., 2010, 132, 8266–8267 CrossRef CAS PubMed
.
-
(a) W. Zi, H.-M. Wu and F. D. Toste, Gold(I)-Catalyzed Dearomative Rautenstrauch Rearrangement: Enantioselective Access to Cyclopenta[b]indoles, J. Am. Chem. Soc., 2015, 137, 3225–3228 CrossRef CAS PubMed
;
(b) X.-D. Shi, D. J. Gorin and F. D. Toste, Synthesis of 2-Cyclopentenones by Gold(I)-Catalyzed Rautenstrauch Rearrangement, J. Am. Chem. Soc., 2005, 127, 5802–5803 CrossRef CAS
.
-
(a) Q.-H. Deng, H. Wadepohl and L. H. Gade, Highly Enantioselective Copper-Catalyzed Alkylation of β-Ketoesters and Subsequent Cyclization to Spirolactones/Bi-spirolactones, J. Am. Chem. Soc., 2012, 134, 2946–2949 CrossRef CAS
;
(b) T. Bootwicha, X. Liu, R. Pluta, I. Atodiresei and M. Rueping, N-Trifluoromethylthiophthalimide: A Stable Electrophilic SCF3-Reagent and its Application in the Catalytic Asymmetric Trifluoromethylsulfenylation, Angew. Chem., Int. Ed., 2013, 52, 12856–12859 CrossRef CAS
;
(c) B. M. Trost and G. M. Schroeder, Cyclic 1,2-Diketones as Building Blocks for Asymmetric Synthesis of Cycloalkenones, J. Am. Chem. Soc., 2000, 122, 3785–3786 CrossRef CAS
.
- S. P. Tanis, E. D. Robinson, M. C. McMills and W. Watt, Furans in synthesis. 11. Total syntheses of (.+-.)- and (-)-fastigilin C, J. Am. Chem. Soc., 1992, 114, 8349–8362 CrossRef CAS
.
-
(a) L.-M. Zhang, A Non-Diazo Approach to α-Oxo Gold Carbenes via Gold-Catalyzed Alkyne Oxidation, Acc. Chem. Res., 2014, 47, 877–888 CrossRef CAS PubMed
;
(b) G.-Z. Dong, M. Bao, X.-D. Xie, S.-K. Jia, W.-H. Hu and X.-F. Xu, Asymmetric Allylation by Chiral Organocatalyst-Promoted Formal Hetero-Ene Reactions of Alkylgold Intermediates, Angew. Chem., Int. Ed., 2021, 60, 1992–1999 CrossRef CAS PubMed
;
(c) B. Zhou, Y.-Q. Zhang, X. Liu and L.-W. Ye, Gold-catalyzed intermolecular reaction of ynamides with 3-indolyl azides via an unexpected 1,2-alkyl migration, Sci. Bull., 2017, 62, 1201–1206 CrossRef CAS
;
(d) Z.-T. Zheng, Z.-X. Wang, Y.-L. Wang and L.-M. Zhang, Au-Catalysed oxidative cyclisation, Chem. Soc. Rev., 2016, 45, 4448–4458 RSC
;
(e) S. Witzel, A. S. K. Hashmi and J. Xie, Light in Gold Catalysis, Chem. Rev., 2021, 121, 8868–8925 CrossRef CAS
;
(f) L. Nunes dos Santos Comprido, J. E. M. N. Klein, G. Kästner, J. Knizia and A. S. K. Hashmi, On the Accessible Reaction Channels of Vinyl Gold(I) Species: π- and σ-Pathways, Chem. – Eur. J., 2017, 23, 10901–10905 CrossRef
;
(g) L.-W. Ye, X.-Q. Zhu, R. L. Sahani, Y. Xu, P.-C. Qian and R.-S. Liu, Nitrene Transfer and Carbene Transfer in Gold Catalysis, Chem. Rev., 2021, 121, 9039–9112 CrossRef CAS PubMed
.
-
(a) A. S. K. Hashmi, Homogeneous Gold Catalysis Beyond Assumptions and Proposals-Characterized Intermediates, Angew. Chem., Int. Ed., 2010, 49, 5232–5241 CrossRef CAS PubMed
;
(b) C. Zhang, K. Hong, C. Pei, S. Zhou, W.-H. Hu, A. S. K. Hashmi and X.-F. Xu, Gold(I)-catalyzed intramolecular cyclization/intermolecular cycloaddition cascade as a fast track to polycarbocycles and mechanistic insights, Nat. Commun., 2021, 12, 1182 CrossRef CAS
;
(c) D. Campeau, D. F. León Rayo, A. Mansour, K. Muratov and F. Gagosz, Gold-Catalyzed Reactions of Specially Activated Alkynes, Allenes, and Alkenes, Chem. Rev., 2021, 121, 8756–8867 CrossRef CAS PubMed
;
(d) Z.-T. Zheng, X. Ma, X.-P. Cheng, K. Zhao, K. Gutman, T.-Y. Li and L.-M. Zhang, Homogeneous Gold-Catalyzed Oxidation Reactions, Chem. Rev., 2021, 121, 8979–9038 CrossRef CAS PubMed
;
(e) Y. Zheng, J.-Q. Zhang, X.-P. Cheng, X.-F. Xu and L.-M. Zhang, Wolff Rearrangement of Oxidatively Generated α-Oxo Gold Carbenes: An Effective Approach to Silylketenes, Angew. Chem., Int. Ed., 2019, 58, 5241–5245 CrossRef CAS PubMed
;
(f) A. S. K. Hashmi, T. M. Frost and J. W. Bats, Highly Selective Gold-Catalyzed Arene Synthesis, J. Am. Chem. Soc., 2000, 122, 11553–11554 CrossRef CAS
;
(g) A. S. K. Hashmi, L. Schwarz, J.-H. Choi and T. M. Frost, A New Gold-Catalyzed C−C Bond Formation, Angew. Chem., Int. Ed., 2000, 39, 2285–2288 CrossRef CAS
;
(h) A. S. K. Hashmi, Homogeneous gold catalysts and alkynes: A successful liaison, Gold Bull., 2003, 36, 3–9 CrossRef CAS
;
(i) A. S. K. Hashmi, Homogeneous Catalysis by Gold, Gold Bull., 2004, 37, 51–65 CrossRef CAS
;
(j) A. Hoffmann-Röder and N. Krause, The golden gate to catalysis, Org. Biomol. Chem., 2005, 3, 387–391 RSC
;
(k) A. S. K. Hashmi, Gold-Catalyzed Organic Reactions, Chem. Rev., 2007, 107, 3180–3211 CrossRef CAS PubMed
;
(l) A. Arcadi, Alternative Synthetic Methods through New Developments in Catalysis by Gold, Chem. Rev., 2008, 108, 3266–3325 CrossRef CAS
;
(m) A. Corma, A. Leyva-Pérez and M. J. Sabater, Gold-Catalyzed Carbon−Heteroatom Bond-Forming Reactions, Chem. Rev., 2011, 111, 1657–1712 CrossRef CAS
;
(n) R. J. Harrisa and R. A. Widenhoefer, Gold carbenes, gold-stabilized carbocations, and cationic intermediates relevant to gold-catalysed enyne cycloaddition, Chem. Soc. Rev., 2016, 45, 4533–4551 RSC
.
-
(a) L.-M. Zhang and S.-Z. Wang, Efficient Synthesis of Cyclopentenones from Enynyl Acetates via Tandem Au(I)-Catalyzed 3,3-Rearrangement and the Nazarov Reaction, J. Am. Chem. Soc., 2006, 128, 1442–1443 CrossRef CAS
;
(b) W.-L. Wang, C.-L. Ji, K. Liu, C.-G. Zhao, W.-P. Li and J. Xie, Dinuclear gold catalysis, Chem. Soc. Rev., 2021, 50, 1874–1912 RSC
;
(c) D. Li, W. Zang, M. J. Bird, C. J. T. Hyland and M. Shi, Gold-Catalyzed Conversion of Highly Strained Compounds, Chem. Rev., 2021, 121, 8685–8755 CrossRef CAS
;
(d) R. L. Reyes, T. Iwai and Ma. Sawamura, Construction of Medium-Sized Rings by Gold Catalysis, Chem. Rev., 2021, 121, 8926–8947 CrossRef CAS
;
(e) L.-W. Ye, X.-Q. Zhu, R. L. Sahani, Y. Xu, P.-C. Qian and R.-S. Liu, Nitrene Transfer and Carbene Transfer in Gold Catalysis, Chem. Rev., 2021, 121, 9039–9112 CrossRef CAS
;
(f) X. Zhao, M. Rudolph and A. S. K. Hashmi, Dual gold catalysis – an update, Chem. Commun., 2019, 55, 12127–12135 RSC
;
(g) W. Fang and M. Shi, Recent Advances in the Cycloisomerizations of Methylenecyclopropanes using Gold Catalysis, Chem. – Eur. J., 2018, 24, 9998–10005 CrossRef CAS
;
(h) C. C. Chintawar, A. K. Yadav, A. Kumar, P. Sancheti and N. T. Patil, Divergent Gold Catalysis: Unlocking Molecular Diversity through Catalyst Control, Chem. Rev., 2021, 121, 8478–8558 CrossRef CAS
.
- L.-M. Zhang and S.-Z. Wang, Efficient Synthesis of Cyclopentenones from Enynyl Acetates via Tandem Au(I)-Catalyzed 3,3-Rearrangement and the Nazarov Reaction, J. Am. Chem. Soc., 2006, 128, 1442–1443 CrossRef CAS
.
-
(a) X. Chen, Y. Zhou, M. Hong, Y. Ling, D. Yin, S. Wang, X. Zhang and W. Rao, Gold(I)-Catalyzed Tandem Cycloisomerization and Fluorination of 1,3(4)-Enyne Esters with NFSI: One-Pot Assembly of 5-Fluoro- Cyclopentenones, Adv. Synth. Catal., 2018, 360, 3700–3708 CrossRef CAS
;
(b) Y. Zhou, X. Chen, D.-L. Yin, Y. Ling, S.-F. Wang, X. Zhang and W.-D. Rao, Gold-Catalyzed Cycloisomerization-Halogenation Sequence of 1,3-Enyne Esters with NXS: Efficient Synthesis of 5-Bromo/Iodo Cyclopentenones, Eur. J. Org. Chem., 2019, 999–1007 CrossRef
.
-
(a) X.-Y. Qin, J.-Y. Wang, F.-Z. Geng, W.-J. Hao, S.-J. Tu and B. Jiang, Engaging yne-allenones in tunable catalytic silane-mediated conjugate transfer reductions, Chem. Commun., 2021, 57, 5394–5397 RSC
;
(b) T.-S. Zhang, W.-J. Hao, R. Wang, S.-C. Wang, S.-J. Tu and B. Jiang, Electrocatalytic three-component annulation-halosulfonylation of 1,6-enynes toward 1-indanones using sodium halides as both halogen sources and electrolytes, Green Chem., 2020, 22, 4259–4269 RSC
;
(c) H.-D. Zuo, W.-J. Hao, C.-F. Zhu, C. Guo, S.-J. Tu and B. Jiang, Electrochemical Annulation-Iodosulfonylation of 1,5-Enyne-containing para-Quinone Methides (p-QMs) to Access (E)-Spiroindenes, Org. Lett., 2020, 22, 4471–4477 CrossRef CAS PubMed
;
(d) Q. Zhao, W.-J. Hao, H.-N. Shi, T. Xu, S.-J. Tu and B. Jiang, Photocatalytic Annulation–Alkynyl Migration Strategy for Multiple Functionalization of Dual Unactivated Alkenes, Org. Lett., 2019, 21, 9784–9789 CrossRef CAS
;
(e) S.-C. Wang, P.-Yu. Liu, Y.-X. Chen, Z.-J. Shen, W.-J. Hao, S.-J. Tu and B. Jiang, Copper/silver co-mediated three-component bicyclization for accessing indeno[1,2-c]azepine-3,6-diones, Chem. Commun., 2021, 57, 7966–7969 RSC
.
-
(a) V. Gobé, P. Retailleau and X. Guinchard, Self-Relay Gold(I)-Catalyzed Pictet-Spengler/Cyclization Cascade Reaction for the Rapid Elaboration of Pentacyclic Indole Derivatives, Chem. – Eur. J., 2015, 21, 17587–17590 CrossRef
;
(b) S. Fustero, J. Miró, M. Sánchez-Roselló and C. del Pozo, Tandem Gold Self-Relay Catalysis for the Synthesis of 2,3-Dihydropyridin-4(1H)-ones: Combination of σ and π Lewis Acid Properties of Gold Salts, Chem. – Eur. J., 2014, 20, 14126–14131 CrossRef CAS
;
(c) X.-Y. Qin, F.-T. Meng, M. Wang, S.-J. Tu, W.-J. Hao, J.-Y. Wang and B. Jiang, Gold-Catalyzed Skeletal Rearrangement of Alkenes: Regioselective Synthesis of Skeletally Diverse Tricyclic Heterocycles and Mechanistic Investigations, ACS Catal., 2021, 11, 6951–6959 CrossRef CAS
;
(d) Y. Yu, W.-B. Yang, F. Rominger and A. S. K. Hashmi, In Situ Generation of Nucleophilic Allenes by the Gold-Catalyzed Rearrangement of Propargylic Esters for the Highly Diastereoselective Formation of Intermolecular C(sp3)-C(sp2) Bonds, Angew. Chem., Int. Ed., 2013, 52, 7586–7689 CAS
;
(e) T. Lauterbach, S. Gatzweiler, P. Nösel, M. Rudolph, F. Rominger and A. S. K. Hashmi, Carbene Transfer – A New Pathway for Propargylic Esters in Gold Catalysis, Adv. Synth. Catal., 2013, 355, 2481–2487 CrossRef CAS
.
-
(a) M. Janka, W. He, I. E. Haedicke, F. R. Fronczek, A. J. Frontier and R. Eisenberg, Tandem Nazarov Cyclization−Michael Addition Sequence Catalyzed by an Ir(III) Complex, J. Am. Chem. Soc., 2006, 128, 5312–5313 CrossRef CAS
;
(b) M. Fujiwara, M. Kawatsura, S. Hayase, M. Nanjo and T. Itoh, Iron(III) Salt-Catalyzed Nazarov Cyclization/Michael Addition of Pyrrole Derivatives, Adv. Synth. Catal., 2009, 351, 123–128 CrossRef CAS
.
- B. M. Trost, The Atom Economy—A Search for Synthetic Efficiency, Science, 1991, 254, 1471–1477 CrossRef CAS
.
Footnote |
† Electronic supplementary information (ESI) available. CCDC 2104366 (3b). For ESI and crystallographic data in CIF or other electronic format see DOI: 10.1039/d1qo01313k |
|
This journal is © the Partner Organisations 2022 |