DOI:
10.1039/D1QO01392K
(Research Article)
Org. Chem. Front., 2022,
9, 210-215
Efficient enantioselective synthesis of CF2H-containing dispiro[benzo[b]thiophene-oxindole-pyrrolidine]s via organocatalytic cycloaddition†
Received
16th September 2021
, Accepted 23rd November 2021
First published on 24th November 2021
Abstract
An efficient and practical organocatalytic asymmetric [3 + 2] cycloaddition of difluoromethylated ketoimines and methyleneindolinones catalyzed by a quinine-derived squaramide has been disclosed. Under mild conditions, a broad range of CF2H-containing dispiro[benzo[b]thiophene-oxindole-pyrrolidine]s bearing four adjacent chiral centers including two vicinal spiro quaternary stereocenters were obtained in high yields (up to 99% yield) with excellent diastereoselectivities (>20
:
1 dr, in all cases) and enantioselectivities (up to 99% ee).
The incorporation of F into compounds is considered a useful approach to improve the physical, chemical and biological properties of bioactive molecules.1 Besides the general advantages brought about by the replacement of a hydrogen atom by fluorine, the double displacement of the H atom by the F atom in the –methyl group makes the resulting –CF2H exhibit slight acidity and can act as a H-bond donor group.2 So, it is becoming increasingly attractive to use –CF2H as a bioisostere of hydroxyl (OH) or thiol (SH) to optimize drug performance.3 At the point of the molecular mechanism of drug action, a suitable installation of –CF2H imparted into the molecule on the rule of steric complementarity is associated with enhanced binding affinity. Therefore, the asymmetric synthesis of the CF2H-containing molecule is highly desired in medicinal research, especially application in the improvement of the moderator of protein–protein interactions because an additional H-bond is of benefit to drug binding with the flat feature protein target.4
Natural products with the 3,3′-spirooxindole skeleton have shown various bioactivities, including insecticidal, cytotoxic, anthelmintic, calmodulin inhibition, osteoclast inhibition, antibacterial, and analgesic properties.5 Considerable efforts have been devoted to the construction of oxindole derivatives and multifunctional compounds with fascinating biological activities were obtained.6 For example, both compounds MI77301 and A (Fig. 1) can be used as non-peptide inhibitors of P53-MDM2 protein–protein interactions, and compound A has advanced to phase I clinical trials.7 Spurred by the demands of drug research, plenty of groups have been incorporated into the 3,3′-pyrrolidinyl-spirooxindole skeleton in an asymmetric manner.8 Attracted by the properties of molecules with fluorine groups, lots of asymmetric syntheses of CF3-containing molecules with 3,3′-pyrrolidinyl-spirooxindole motifs have been reported.9 Recently, a dual inhibitor of molecule B (Fig. 1) has been discovered.10 However, for –CF2H, there remains a blank area. Herein, we wish to describe a new approach in the synthesis of CF2H-containing 3,3′-pyrrolidinyl-spirooxindoles.
 |
| Fig. 1 Selected examples of biologically relevant compounds. | |
Synthetically, to construct a five-member nitrogen heterocycle especially for a spirocyclic pyrrolidine, 1,3-dipolar cycloaddition is one of the most direct methods.11 In this context, a polyfunctional pyrrolidine can be furnished by a N-central 1,3-dipole under appropriate conditions. Even thus, considering the great differences in the electronic property, group radius and acidity between –CF3 and –CF2H, there is no CF2H-containing 1,3-dipole that has been reported to date.12 Most recently, we have developed a CF2H-containing ketoimine by the condensation of thioisatin with difluoroethylamine.13 Promoted by a Brønsted base, the deprotonation group in methylene of thioisatin-derived α-(difluoromethyl)imine can be achieved. We supposed that the resulting methylene ylide intermediate could be used as a 1,3-dipole and may be applied to construct CF2H-containing 3,3′-pyrrolidinyl-dispirooxindoles.
To verify our hypothesis, methyleneindolinone 2a was selected as a dipolarophile. Interestingly, the cycloaddition of imine 1a with 2a proceeded smoothly in the presence of cinchonidine-derived thiourea C1 (Fig. 2) and the product was obtained in 73% ee and 75% yield (Table 1, entry 1). When thiourea C2 (Fig. 2) derived from quinine was the catalyst, an improved yield and ee were observed. When squaramide C7 was employed, the yield and ee of the product increased to 90% and 94%, respectively. Further screening provided the cycloadduct in 96% ee and 89% yield (Table 1, entry 8) when squaramide C8 (Fig. 2) was selected as the catalyst. The effects of the solvent and temperature were then evaluated with C8 as the catalyst. A 95% yield in 98% ee at 0 °C with DCE (1,2-dichloroethane) was obtained as a result.
 |
| Fig. 2 Typical catalysts. | |
Table 1 Screening of reaction conditionsa
Under the optimized conditions, the scope of the substrates was subsequently investigated. As shown in Scheme 1, methyleneindolinones substituted at the 1-position showed good reactivity. Partially because of the poor solubility, the addition was completed in a prolonged time even at room temperature for methylpolylinone without substitution at position 1. Strikingly, R2 groups had no significant effect on the reaction, and the single crystals of 3aa and 3ag showed that the same reaction pattern had occurred.
 |
| Scheme 1 The variation of PGs on methyleneindolinones. Reaction conditions: 1a (0.10 mmol), 2 (0.12 mmol), and catalyst C8 (10 mol%) in DCE (1 ml) at 0 °C; the reaction time required for each substrate is given. The yields of the isolated products are reported. The ee and dr values were determined by HPLC or 1H NMR and 19F NMR. a Furnished at room temperature. | |
The following analyses were focused on substituents on the benzene ring of methyleneindolinones and the results are shown in Scheme 2. Both electron-donating and electron-withdrawing substituents of 5-alkenyl methyleneindolinones worked well with excellent stereoselectivities. Compared with the 5, 6 and 7 positions, a substituent at the 4-position showed significantly lowered reactivity. For example, when 4-chloro-methyleneindolinone (Scheme 2, 3ah) was subjected to the standard conditions, the reaction completed within 24 hours, while for substrates substituted at the 5-position (Scheme 2, 3aj) and the 7-position (Scheme 2, 3ar), only 2.5 (Scheme 2, 3ah) and 1.5 h were needed. For 6-methoxy 3ap (Scheme 2), a very slow reaction was observed (28 h). Disubstituted substrates were generally well tolerated under standard conditions.
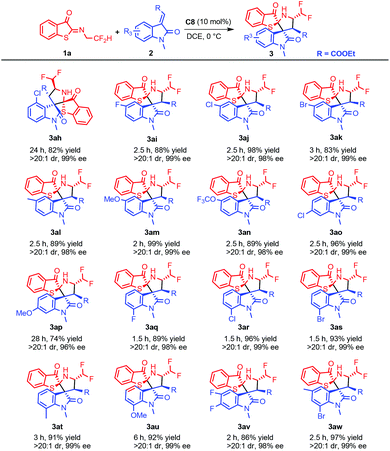 |
| Scheme 2 Substrate scope of methyleneindolinones. Reaction conditions: 1a (0.10 mmol), 2 (0.12 mmol), and catalyst C8 (10 mol%) in DCE (1 ml) at 0 °C; the reaction time required for each substrate is given. The yields of the isolated products are reported. The ee and dr values were determined by HPLC or 1H NMR and 19F NMR. | |
Furthermore, the generality of dipoles 1 was evaluated and the results are presented in Scheme 3. In general, electron-donating groups showed lower reactivity (Scheme 3, 3ca, 3ga, 3ha) while electron-withdrawing groups showed good reactivity (Scheme 3, 3ba, 3da, 3ea, 3fa). Disubstituted (Scheme 3, 3ja, 3ka) and polyaryl substrates (Scheme 3, 3ia) all performed well with excellent yields and stereoselectivities.
 |
| Scheme 3 Substrate scope of ketoimines. Reaction conditions: 1 (0.10 mmol), 2a (0.12 mmol), and catalyst C8 (10 mol%) in DCE (1 ml) at 0 °C; the reaction time required for each substrate is given. The yields of the isolated products are reported. The ee and dr values were determined by HPLC or 1H NMR and 19F NMR. | |
Encouraged by the good results of ester substrates, carbonyl aryl methyleneindolinones were also examined. As shown in Scheme 4, various aryl ketones were well tolerated and gave the corresponding cycloaddition products with good yields and stereoselectivities. Heterocyclic and naphthalene substrates (Scheme 4, 5ah, 5ai and 5aj) also worked well. Methyleneindolinones were also compatible, giving the product in 80% yield with >20
:
1 dr and 91% ee.
 |
| Scheme 4 The variation of methyleneindolinones. Reaction conditions: 1a (0.10 mmol), 4 (0.12 mmol), and catalyst C8 (10 mol%) in DCE (1 ml) at 0 °C; the reaction time required for each substrate is given. The yields of the isolated products are reported. The ee and dr values were determined by HPLC or 1H NMR and 19F NMR. | |
As indicated in Scheme 5, a gram-scale synthesis was carried out to demonstrate the utility of the present process. In the presence of 10 mol% C8, 1a (2.5 mmol, 0.567 g) and 2a (3.0 mmol, 0.693 g) were consumed within 4.5 h to give 3aa in 92% yield with 96% ee, and a further N-methylation product of 6a was obtained without racemization in the presence of potassium carbonate. Furthermore, 3aa was converted to optically pure sulfone 7a in 50% yield with 94% ee by treatment with HCO2H/H2O2. Pleasingly, a scale-up reaction between 1a and 2g still proceeded well and furnished the desired product 3ag with almost untouched yield (84% yield) and enantioselectivity (96% ee).
 |
| Scheme 5 Gram-scale experiments and further transformations. | |
Control experiments were subsequently performed to shed light on the reaction mechanism. As shown in Scheme 6, when phenyl or methyl 3-alkenyloxindole was used, this reaction did not occur under standard conditions. When adjusted to room temperature, no reaction was observed either. A possible reaction mechanism was suggested on the basis of control experiments and the absolute configuration of 3aa and 3ag (Scheme 1). The potential transition mechanism as shown in Fig. 3, the acidic N-2,2-difluoroethyl thioisatin ketoimines hydrogen bonded with the basic tertiary N atom of the catalyst to form a five-membered ring, concurrently, the two squaramide N–H bonds of catalyst hydrogen bonding with the methyleneindolinones. The chiral bifunctional squaramide-tertiary amine catalyst anchored the two substrates close to each other and the two consecutive re-face additions delivered the corresponding products with X-ray configurations.
 |
| Scheme 6 Control experiments. Reaction conditions: 1a (0.10 mmol), 2aa or 2ab (0.12 mmol), and catalyst C8 (10 mol%) in DCE (1 ml) at 0 °C. a Stirred at room temperature. | |
 |
| Fig. 3 Potential transition mechanism. | |
Conclusions
In summary, a novel kind of CF2H-containing 3,3′-dispiro-pyrrolidine-3,3′-oxindole has been achieved through an organocatalyzed 1,3-dipole reaction. In the presence of cinchona alkaloid-derived bifunctional squaramide, the cycloaddition of thioisatin-derived α-(difluoromethyl)imine and methyleneindolinone carbonyl gave the cycloadducts smoothly.
Various esters and ketones were well tolerated and, in general, gave the products in excellent yields, ee and dr.
Conflicts of interest
The authors declare no competing financial interest.
Acknowledgements
We are grateful for the grants from the National Natural Science Foundation of China (no. 81872723 and 82073679).
Notes and references
-
(a) M. Inoue, Y. Sumii and N. Shibata, Contribution of Organofluorine Compounds to Pharmaceuticals, ACS Omega, 2020, 5, 10633 CrossRef CAS PubMed;
(b) H. Mei, A. M. Remete, Y. Zou, H. Moriwaki, S. Fustero, L. Kiss, V. A. Soloshonoke and J. Han, Fluorine-containing drugs approved by the FDA in 2019, Chin. Chem. Lett., 2020, 31, 2401 CrossRef CAS;
(c) H. Mei, J. Han, S. Fustero, M. Medio-Simon, D. M. Sedgwick, C. Santi, R. Ruzziconi and V. A. Soloshonok, Fluorine-Containing Drugs Approved by the FDA in 2018, Chem. – Eur. J., 2019, 25, 11797 CrossRef CAS PubMed;
(d) Y. Zhou, J. Wang, Z. Gu, S. Wang, W. Zhu, J. L. Aceña, V. A. Soloshonok, K. Izawa and H. Liu, Next Generation of Fluorine-Containing Pharmaceuticals, Compounds Currently in Phase II−III Clinical Trials of Major Pharmaceutical Companies: New Structural Trends and Therapeutic Areas, Chem. Rev., 2016, 116, 422 CrossRef CAS PubMed.
-
(a) J. P. Shanahan, D. M. Mullis, M. Zeller and N. K. Szymczak, Reductively Stable Hydrogen-Bonding Ligands Featuring Appended CF2−H Units, J. Am. Chem. Soc., 2020, 142, 8809 CrossRef PubMed;
(b) J. B. Geri, M. M. W. Wolfe and N. K. Szymczak, The Difluoromethyl Group as a Masked Nucleophile: A Lewis Acid/Base Approach, J. Am. Chem. Soc., 2018, 140, 9404 CrossRef CAS PubMed;
(c) C. D. Sessler, M. Rahm, S. Becker, J. M. Goldberg, F. Wang and S. J. Lippard, CF2H, a Hydrogen Bond Donor, J. Am. Chem. Soc., 2017, 139, 9325 CrossRef CAS PubMed.
-
(a) Y. Zafrani, S. Saphier and E. Gershonov, Utilizing the CF2H moiety as a H-bond-donating group in drug discovery, Future Med. Chem., 2020, 12, 361 CrossRef CAS PubMed;
(b) Y. Zafrani, G. Sod-Moriah, D. Yeffet, A. Berliner, D. Amir, D. Marciano, S. Elias, S. Katalan, N. Ashkenazi, M. Madmon, E. Gershonov and S. Saphier, CF2H, a Functional Group-Dependent Hydrogen-Bond Donor: Is It a More or Less Lipophilic Bioisostere of OH, SH, and CH3?, J. Med. Chem., 2019, 62, 5628 CrossRef CAS PubMed;
(c) Y. Zafrani, D. Yeffet, G. Sod-Moriah, A. Berliner, D. Amir, D. Marciano, E. Gershonov and S. Saphier, Difluoromethyl Bioisostere: Examining the ′′Lipophilic Hydrogen Bond Donor′′ Concept, J. Med. Chem., 2017, 60, 797 CrossRef CAS PubMed.
- For reviews, see:
(a) F.-Y. Gao, B.-Y. Li, Y.-l. Wang, Q.-S. Chen, Y.-Z. Li, K.-R. Wang and W.-J. Yan, Stereoselective synthetic strategies of stereogenic carbon centers featuring a difluoromethyl group, Org. Chem. Front., 2021, 8, 2799 RSC;
(b) J. J. Miller, C. Gaiddon and T. Storr, A balancing act: using small molecules for therapeutic intervention of the p53 pathway in cancer, Chem. Soc. Rev., 2020, 49, 6995 RSC;
(c) Y. Liu, X. Wang, G. Wang, Y. Yang, Y. Yuan and L.-O. yang, The past, present and future of potential small-molecule drugs targeting p53-MDM2/MDMX for cancer therapy, Eur. J. Med. Chem., 2019, 176, 92 CrossRef CAS PubMed.
- For recent examples, see:
(a) N. J. Y. Chear, F. León, A. Sharma, S. R. R. Kanumuri, G. Zwolinski, K. A. Abboud, D. Singh, L. F. Restrepo, A. Patel, T. Hiranita, S. Ramanathan, A. J. Hampson, L. R. McMahon and C. R. McCurdy, Exploring the Chemistry of Alkaloids from Malaysian Mitragyna speciosa (Kratom) and the Role of Oxindoles on Human Opioid Receptors, J. Nat. Prod., 2021, 84, 1034 CrossRef CAS PubMed;
(b) N. Qin, X. Lu, Y. Liu, Y. Qiao, W. Qu, F. Feng and H. Sun, Recent research progress of Uncaria spp. based on alkaloids: phytochemistry, pharmacology and structural chemistry, Eur. J. Med. Chem., 2021, 210, 112960 CrossRef CAS PubMed;
(c) K. R. Klas, H. Kato, J. C. Frisvad, F. Yu, S. A. Newmister, A. E. Fraley, D. H. Sherman, S. Tsukamoto and R. M. Williams, Structural and stereochemical diversity in prenylated indole alkaloids containing the bicycle [2.2.2]diazaoctane ring system from marine and terrestrial fungi, Nat. Prod. Rep., 2018, 35, 532 RSC;
(d) M. Ishikura, T. Abe, T. Choshi and S. Hibino, Simple indole alkaloids and those with a nonrearranged monoterpenoid unit, Nat. Prod. Rep., 2015, 32, 1389 RSC.
- For recent reviews, see:
(a) D. Bora, A. Kaushal and N. Shankaraiah, Anticancer potential of spirocompounds in medicinal chemistry: A pentennial expedition, Eur. J. Med. Chem., 2021, 215, 113263 CrossRef CAS PubMed;
(b) M. Kaur, M. Singh, N. Chadha and O. Silakari, Oxindole: A chemical prism carrying plethora of therapeutic benefits, Eur. J. Med. Chem., 2016, 123, 858 CrossRef CAS PubMed;
(c) B. Yu, D.-Q. Yu and H.-M. Liu, Spirooxindoles: Promising scaffolds for anticancer agents, Eur. J. Med. Chem., 2015, 97, 683 CrossRef PubMed.
- A. Aguilar, J. Lu, L. Liu, D. Du, D. Bernard, D. McEachern, S. Przybranowski, X. Li, R. Luo, B. Wen, D. Sun, H. Wang, J. Wen, G. Wang, Y. Zhai, M. Guo, D. Yang and S. Wang, Discovery of 4-((3′R,4′S,5′R)-6′′-Chloro-4′-(3-chloro-2-fluorophenyl)-1′-ethyl -2′′-oxodispiro [cyclohexane - 1,2′ – pyrrolidine - 3′,3′′ - indoline]-5′-carboxamido) bicyclo [2.2.2] octane-1-carboxylic Acid (AA-115/APG-115): A Potent and Orally Active Murine Double Minute 2 (MDM2) Inhibitor in Clinical Development, J. Med. Chem., 2017, 60, 2819 CrossRef CAS PubMed.
- For selected recent reviews, see:
(a) A. J. Boddy and J. A. Bull, Stereoselective synthesis and applications of spirocyclic oxindoles, Org. Chem. Front., 2021, 8, 1026 RSC;
(b) E. Sansinenea, E. F. Martínez and A. Ortiz, Organocatalytic Synthesis of Chiral Spirooxindoles with Quaternary Stereogenic Centers, Eur. J. Org. Chem., 2020, 5101 CrossRef CAS;
(c) P.-W. Xu, J.-S. Yu, C. Chen, Z.-Y. Cao, F. Zhou and J. Zhou, Catalytic Enantioselective Construction of Spiro Quaternary Carbon Stereocenters, ACS Catal., 2019, 9, 1820 CrossRef CAS;
(d) G.-J. Mei and F. Shi, Catalytic asymmetric synthesis of spirooxindoles: recent developments, Chem. Commun., 2018, 54, 6607 RSC.
- For reviews, see:
(a) H.-Z. Gui, W. Yin and M. Shi, Recent Advances in the Construction of Trifluoromethyl-Containing Spirooxindoles through Cycloaddition Reactions, Chem. – Asian J., 2020, 15, 1225 CrossRef CAS PubMed. For recent examples, see:
(b) Z. Jiang, X. Liu, H. Zhang, J.-P. Tan, X. Ren, G. Gao and T. Wang, Bifunctinoal Phosphonium Salt–Catalyzed Asymmetric Cyclodearomatization of 2-Nitroindoles and 2−Nitrobenzofurans for Constructing CF3 -Containing Spiro-Polycycles, Adv. Synth. Catal., 2021, 363, 3115 CrossRef CAS;
(c) F.-Y. Chen, L. Xiang, G. Zhan, H. Liu, B. Kang, S.-C. Zhang, C. Peng and B. Han, Highly stereoselective organocatalytic synthesis of pyrrolidinyl spirooxindoles containing halogenated contiguous quaternary carbon stereocenters, Tetrahedron Lett., 2020, 61, 151806 CrossRef CAS;
(d) X. Liu, D. Lu, J.-H. Wu, J.-P. Tan, C. Jiang, G. Gao and T. Wang, Stereoselective Synthesis of CF3 -Containing Spirooxindoles via 1,3-Dipolar Cycloaddition by Dipeptide–Based Phosphonium Salt Catalysis, Adv. Synth. Catal., 2020, 362, 1490 CrossRef CAS;
(e) Y. Yi, Y.-Z. Hua, H.-J. Lu, L.-T. Liu and M.-C. Wang, Brønsted Base and Lewis Acid Cooperatively Catalyzed Asymmetric exo’ - Selective [3 + 2] Cycloaddition of Trifluoromethylated Azomethine Ylides and Methyleneindolinones, Org. Lett., 2020, 22, 2527 CrossRef CAS PubMed;
(f) T.-L. An and D.-M. Du, Chiral Squaramide Catalyzed Asymmetric [3 + 2] Cycloaddition Reaction for Synthesis of Trifluoromethylated Barbituric Acid Derivatives, ChemistrySelect, 2019, 4, 11302 CrossRef CAS;
(g) X. Zhao, J. Xiong, J. An, J. Yu, L. Zhu, X. Feng and X. Jiang, Diastereodivergent construction of bispiro[oxindole-bi-pyrrolidine]s with four consecutive stereocenters via asymmetric [3 + 2] cycloaddition of 2,3-dioxopyrrolidines using identical catalysts, Org. Chem. Front., 2019, 6, 1989 RSC;
(h) C. Wang, D.-W. Wen, H. Chen, Y.-B. Deng, X. Liu, L. Wang, F.-Y. Gao, Y.-F. Guo, M.-M. Sun, K.-R. Wang and W.-J. Yan, The catalytic asymmetric synthesis of CF3-containing spiro-oxindole–pyrrolidine–pyrazolone compounds through squaramide-catalyzed 1,3-dipolar cycloaddition, Org. Biomol. Chem., 2019, 17, 5514 RSC;
(i) B.-Y. Li, F.-Y. Gao, X. Feng, M.-M. Sun, Y.-F. Guo, D.-W. Wen, Y.-B. Deng, J.-Q. Huang, K.-R. Wang and W.-J. Yan, Highly efficient enantioselective synthesis of bispiro [benzofuran - oxindole -pyrrolidine]s through organocatalytic cycloaddition, Org. Chem. Front., 2019, 6, 1567 RSC.
- S.-J. Liu, Q. Zhao, C. Peng, Q. Mao, F. Wu, F.-H. Zhang, Q.-S. Feng, G. He and B. Han, Design, synthesis, and biological evaluation of nitroisoxazole - containing spiro [pyrrolidine - oxindole] derivatives as novel glutathione peroxidase 4/mouse double minute 2 dual inhibitors that inhibit breast adenocarcinoma cell proliferation, Eur. J. Med. Chem., 2021, 217, 113359 CrossRef CAS PubMed.
- For a recent review, see: X. Fang and C.-J. Wang, Catalytic asymmetric construction of spiropyrrolidines via 1,3-dipolar cycloaddition of azomethine ylides, Org. Biomol. Chem., 2018, 16, 2591 RSC.
-
(a) E. Miller, S. Kim, K. Gibson, J. S. Derrick and F. D. Toste, Regio- and Enantioselective Bromocyclization of Difluoroalkenes as a Strategy to Access Tetrasubstituted Difluoromethylene-Containing Stereocenters, J. Am. Chem. Soc., 2020, 142, 8946 CrossRef CAS PubMed;
(b) J. Rong, C.-F. Ni and J.-B. Hu, Metal-Catalyzed Direct Difluoromethylation Reactions, Asian J. Org. Chem., 2017, 6, 139 CrossRef CAS;
(c) X. Yang, T. Wu, R. J. Phipps and F. D. Toste, Advances in Catalytic Enantioselective Fluorination, Mono-, Di-, and Trifluoromethylation, and Trifluoromethylthiolation Reactions, Chem. Rev., 2015, 115, 826 CrossRef CAS PubMed.
- F.-Y. Gao, Y.-F. Guo, M.-M. Sun, Y.-L. Wang, C.-Y. Yang, Y. Wang, K.-R. Wang and W.-J. Yan, Catalytic Asymmetric Construction of Tertiary Carbon Centers Featuring an α-Difluoromethyl Group with CF2H-CH2-NH2 as the “Building Block”, Org. Lett., 2021, 23, 2584 CrossRef PubMed.
Footnote |
† Electronic supplementary information (ESI) available. CCDC 2087188 and 2087189. For ESI and crystallographic data in CIF or other electronic format see DOI: 10.1039/d1qo01392k |
|
This journal is © the Partner Organisations 2022 |
Click here to see how this site uses Cookies. View our privacy policy here.