DOI:
10.1039/D2QO00432A
(Research Article)
Org. Chem. Front., 2022,
9, 3084-3092
Triazolated calix[4]semitubes: assembling strategies towards long multicalixarene architectures†
Received
16th March 2022
, Accepted 14th April 2022
First published on 16th April 2022
Abstract
Cone and 1,3-alternate calix[4]arenes bearing pairs of 2-azidoethyl or propargyl groups, and 1,3-alternate calix[4]arenes having four 2-azidoethyl and four propargyl groups or pairs of 2-azidoethyl and silylated propargyl groups were explored as the components for the CuAAC-syntheses of triazolated calix[4]semitubes having three calixarene cores in the structures. Though condition tuning for each of the calixarene combinations was performed, the four-fold CuAACs using the tetrafunctional calixarenes returned the respective tris(calixarenes) in good yields only in the syntheses involving the calixarene tetrakis(alkyne) and hydroxylated bis(azides). The stepwise approach towards the triazolated calix[4]semitubes by using the 2-azidoethylated/propargylated calix[4]arene as the key synthone and de-silylation as the intermediate step was found to be a good alternative, which allowed the preparation of the semitubes with different substituents in the terminal calixarene units and can also be used for further extension of the semi-tubular assemblies.
Introduction
The well-developed approaches for chemical transformations of calixarenes and related macrocycles allow us to treat them as molecular platforms within a chemical ‘building kit’ for constructing diverse receptor molecules in which the required number of certain functional groups can be introduced and mutually pre-organized in a predictable manner.1 When several platforms are involved in chemical reactions, more diverse and complicated, and, thus, much more attractive architectures may be created by using such multicalixarene conjugates. To date, many examples have been published on the covalently joining ‘classical’ calixarene macrocycles,2 and those of thiacalixarenes,3 calixpyrroles,4 calixresorcinarenes,5 and heteromacrocyclic conjugates from the calixarene family have also been known.6 Most often, these conjugates comprise only two calixarene macrocycles (or/and related ones), which are connected to each other by one or several linkers. In the latter case, the calixarenes and the linkers form an additional macrocyclic site or cavity, which may possess special or even outstanding receptor abilities. Tris- and even larger multicalixarene assemblies are also known, of which those having a tubular shape are the most attractive,7 though they are less available. These compounds inherit all the structural benefits from the above biscalixarene assemblies and allow allocation of more than one macrocyclic site/cavity within one molecule and manage their connectivity in the range from pure independent mode where simple binding/recognition of multiple guests is possible, up to tightly bound mode, which is prospective for constructing sophisticated supramolecules, e.g. multipositional molecular switchers.
We have recently shown8 that diverse biscalixarene semi-tubes may be constructed from the respective bisazide and bisalkyne calixarene precursors using copper(I)-catalyzed azide–alkyne cycloaddition (CuAAC).9 Each of these assemblies comprises a single binding site formed by two 1,4-disubstituted 1,2,3-triazole groups surrounded by the calixarene cores and targeted to transition cations. Herein we explored the applicability of this synthetic approach for the construction of larger semi-tubular assemblies having three calix[4]arene cores in their structures and possessing two bistriazole receptor sites separated from each other by the central 1,3-alternate calix[4]arene unit.
Results and discussion
Alkyne and azide components of calix[4]semitubes
The design of the long triazolated calix[4]semitubes requires azide/alkyne-containing calixarenes of at least two types to be involved in CuAAC. The calixarenes of the first type are the respective bis(azides) or bis(alkynes) which can be used as the terminal units at both sides of a semi-tube, while the calixarenes of the other type are those having two pairs of azide or/and alkyne groups separated from each other by the macrocyclic core. Calix[4]arenes 1,10 and 2–48 bearing 2-azidoethyl groups (Fig. 1) were selected as the bisazide terminal units in the triazolated calix[4]semitubes, and the structurally related calixarene dipropargyl ethers 5,116,127,13 and 88 were used as the bisalkyne terminal units. Within each of the series, the bifunctional molecules have free phenolic OH groups or those propylated to fix the calix[4]arene macrocycle in a cone or 1,3-alternate molecular shape. Notably, the bis(azides) or bis(alkynes) of each type have been shown to react with each other to form the respective biscalixarene semitubes,8 so they were expected to be suitable for building longer semitubes as well.
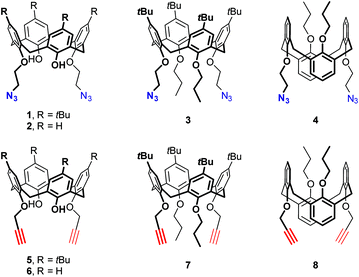 |
| Fig. 1 Calixarenes tested as the terminal units in the calix[4]semitubes. | |
As for the central macrocycles of the triscalixarene semitubes, calixarene 914 having two pairs of propargyl groups arranged by the two ‘sides’ of the 1,3-alternate core was selected for the CuAAC reactions with the bis(azides) 1–4. To study the ‘inverted’ azide/alkyne-combinations involving dipropargyl ethers 5–8, the respective tetrakis(azide) 11 was prepared for the first time from the tetrakis(alcohol) 107d and diphenylphosphoryl azide (DPPA) under the Mitsunobu reaction conditions (Scheme 1).
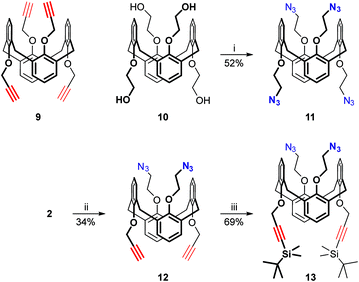 |
| Scheme 1 Calixarenes used as the central units in the calix[4]semitubes: (i) DPPA, DIAD, Ph3P, THF, rt; (ii) propargyl bromide, Cs2CO3, DMF, rt; (iii) TBDMSCl, LiHMDS, THF, rt. | |
The attractive idea to prepare the hybrid central core having pairs of propargyl and 2-azidoethyl groups within the same molecule was successfully implemented by alkylating the bis(azide) 2 with propargyl bromide in the presence of Cs2CO3. To avoid any self-reactions of the prepared calixarene 12 under the CuAAC conditions, its propargyl groups were de-protonated with lithium bis(trimethylsilyl)amide (LiHMDS) and then silylated with tert-butyldimethylsilyl chloride (TBDMSCl) to obtain the protected calixarene 13 in which only the azide groups can participate in the Cu-catalyzed cycloaddition.
One-step assembling of triscalixarene semitubes
It has been shown for the preparation of calix[4]semitubes from bis(azides) and bis(alkynes) under CuAAC conditions that the formation of polymeric/oligomeric byproducts during the reaction could not be avoided, so the selection of proper reaction conditions for every bis(azide)/bis(alkyne) combination was crucial for obtaining targeted semitubes in more or less satisfactory yields, which did not exceed 46%.8 Reasonably, successful preparation of the triscalixarene semitubes using tetrafunctional central cores 9 or 11 requires the same or even more strict tuning of the reaction conditions. Still, the conditions obtained previously for the preparation of a semitube from 1,3-alternate bis(alkyne) 8 and cone bis(azide) 18 were found suitable also for the reaction between the bis(azide) 1 and the tetrakis(alkyne) 9 taken in a 2
:
1 molar ratio (Scheme 2). Indeed, the reaction conducted in the presence of 0.3 equiv. of CuI (per bis(azide)) and triethylamine in toluene at room temperature returned the triscalixarene semitube 14 in 38% yield, which was impressively high considering the formation of four triazole heterocycles in one molecule. The remaining products of the reaction were polymeric/oligomeric compounds as determined from the 1H NMR spectrum of the reaction mixture after removal of copper salts, in which no other sharp signals except for those from the semitube 14 were observed (Fig. 2a). Deceptively, it may seem that the reaction mixture consisted mainly of the semitube 14, while the amount of the polymeric/oligomeric product was relatively small. But in fact, the amount of separated polymeric/oligomeric fraction which showed a very broadened 1H NMR spectrum (Fig. 2c) was nearly equal to that of the pure calix[4]semitube 14 having a sharp NMR pattern (Fig. 2b).
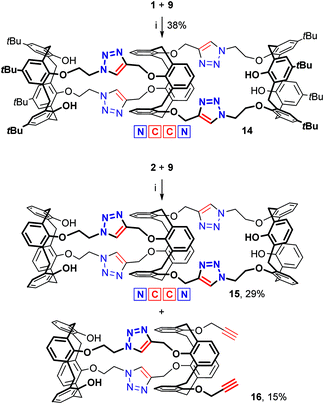 |
| Scheme 2 Syntheses of calix[4]semitubes 14–16: (i) CuI (0.3 equiv. per bis(azide)), Et3N, toluene, rt, 48 h; here and below, for a better distinguishability of the quite similar structures, the combination of and symbols in the squares denotes the arrangement of the triazole groups around the central calixarene core, where (carbon) and (nitrogen) mean the acetylene and azide ‘parts’ of the triazole ring. | |
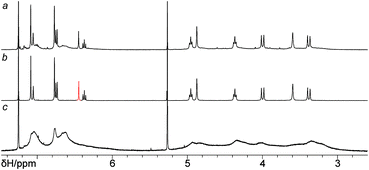 |
| Fig. 2 Parts of the 1H NMR spectra of (a) the mixture obtained from the reaction between calixarenes 1 and 9 after removal of copper salts, (b) pure triscalixarene semitube 14, and (c) separated polymeric/oligomeric fraction (scaled up); 400 MHz, CDCl3; the singlet from the triazole protons in the semitube is colored red. | |
At slow evaporation of a dichloromethane/methanol solution of calix[4]semitube 14 single crystals were collected and subjected to X-ray diffraction analysis.15 The collected data confirm the triscalixarene molecular structure of compound 14 with the 1,3-alternate central calix[4]arene core linked to two terminal cone calix[4]arene cores by two pairs of triazole groups (Fig. 3). Due to the absence of a second pair of substituents at the narrow rims of the terminal calixarenes, the whole system seems relatively flexible, so the overall shape of the molecule, at least in the crystalline state, is far from a straight tubular one.
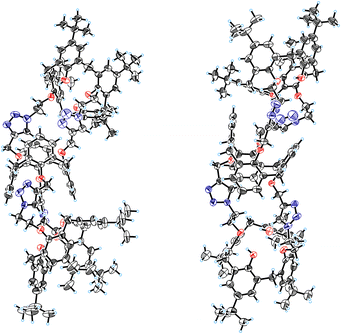 |
| Fig. 3 Molecular structure of the calix[4]semitube 14 in two projections; thermal ellipsoids are drawn at a 50% probability level. | |
When de-tert-butylated bis(azide) 2 was involved in CuAAC with the tetrakis(alkyne) 9 under the conditions used for the preparation of calix[4]semitube 14, the respective tris(calixarene) 15 was obtained in a good yield of 29% (Scheme 2). But in this case, a part of bis(azide) 2 returned unreacted and a non-polymeric by-product was formed. The latter one was separated and confirmed to be the bis(calixarene) 16 formed at the addition of only one molecule of bis(azide) 2 to the tetrakis(alkyne) 9. The reasons for the incomplete conversion of the bis(azide) 2 during the reaction are not clear and may be related to its less solubility in toluene at room temperature. Still, the appearance of bis(calixarene) 16 allows one to suggest it to intermediate the stepwise formation of the triscalixarene semitube 15.
Next, the pair of calixarenes 3 and 9 was studied for the ability to form a semitube. Following our previous observations, the CuAAC between the propylated cone bis(azide) 3 and bis(alkyne) 8 (having the same 1,3-alternate molecular shape as that of the calixarene 9 but only two of four propargyl groups) has given the respective bis(calixarene) separated from a complex mixture with other triazolated huge macrocycles composed of several 3/8 calixarene pairs.8 In the case of calixarenes 3 and 9, the direct implementation of the previously found reaction conditions (CuI (0.3 equiv. per bis(azide)), Et3N, toluene/1,2-dichloroethane, 60 °C, 9 h) failed, as the obtained reaction mixture consisted of both starting calixarenes, a polymeric/oligomeric material, and several triazolated multimacrocycles from which the desired semitube could not be separated using column chromatography with different eluents. Several alterations of the reaction conditions were performed (see Table S1 in the ESI†), which included the changing of solvents (toluene, toluene/1,2-dichloroethane, THF, and THF/H2O) and the catalytic system (CuI·P(OEt)3, CuI, and CuSO4·5H2O/sodium ascorbate), but none of the conditions tested gave a satisfactory outcome. In the best run (CuI (0.3 equiv. per bis(azide)), Et3N, toluene, 75 °C, 24 h) the complete conversion of the starting calixarenes was achieved and the formation of undesired multimacrocycles was suppressed, but the semitube was formed at nearly a trace level among non-separable polymeric products.
When the 1,3-alternate bis(azide) 4 was reacted with the tetrakis(alkyne) 9 (Scheme 3), additional tuning of the reaction conditions was required (see Table S2 in the ESI†). Again, the CuAAC conditions (CuSO4·5H2O/sodium ascorbate (1.0 equiv. per bis(azide)), THF/H2O, 60 °C, 24 h), which were found perfect to assemble two 1,3-alternate calixarenes (4 and 8) into a biscalixarene semitube, turned non-suitable for the synthesis of the tris(calixarene) 17 from the calixarenes 4 and 9. The best result was obtained under CuI/Et3N-catalysis with the catalyst loading decreased back to 0.3 equiv. per bis(azide). After purification by column chromatography the calix[4]semitube 17 was obtained in 15% yield.
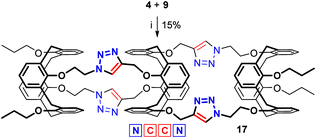 |
| Scheme 3 Synthesis of the calix[4]semitube 17: (i) CuI (0.3 equiv. per bis(azide)), Et3N, toluene, 60 °C, 24 h. | |
The difference in the CuAAC conditions suitable for the formation of bis- and triscalixarene semitubes can be rationalized in terms of the stepwise addition of the calixarenes to each other (see the example of the semitube 15) and by the formation of an efficient copper-binding site in the intermediate bis(calixarene). This site can entrap the cation and prevent it from migration to the next pair of reacting propargyl groups at the other side of calixarene 9, or/and prevent the reaction between the cation(s) from the bulk solution and the propargyl groups due to the Coulomb repulsion, or/and can spatially re-arrange the propargyl groups in a way they turn more suitable for polymer formation rather than for the desired two-point connection to the second molecule of a bis(azide).
When used as the central core in the triscalixarene semitubes, the tetrakis(azide) 11 enables a more distant arrangement of the pairs of the reacting functional groups than the tetrakis(alkyne) 9 does. Accordingly, this can not only diminish the above repulsive interactions between the two Cu-containing bisazide/bistriazole sites, but also make the azide groups within each site less pre-organized, which can hamper the semitube formation. For the CuAAC reaction between the tetrakis(azide) 11 and bis(alkyne) 5 (Scheme 4), condition tuning (see Table S3 in the ESI†) gave the best outcome in the case when CuI/Et3N (0.3 equiv. of Cu per bis(azide)) was used under heating. Nevertheless, the yield of the tris(calixarene) 18 was as small as 6%. Analogous synthesis involving de-tert-butylated bis(azide) 6 furnished the respective calix[4]semitube 19 in 14% yield. Notably, as in the case of tris(calixarene) 17, in both the above cases the Cu-catalyst loading was decreased (from 1.0 to 0.3 equiv. per bis(azide)) with respect to the reference reaction between the bis(azide) 4 and bis(alkyne) 5.8
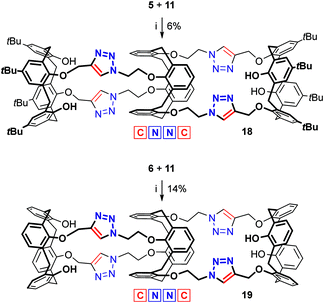 |
| Scheme 4 Syntheses of calix[4]semitubes 18 and 19: (i) CuI (0.3 equiv. per bis(azide)), Et3N, toluene, 60 °C, 12 h. | |
In contrast, the CuAAC between the propylated bis(alkyne) 7 and the tetrakis(azide) 11 (Scheme 5) was successfully conducted under the conditions found previously for the reference reaction between compounds 4 and 7 (1.0 equiv. of CuI and a huge excess of Et3N at heating),8 and no improvement was achieved upon varying the catalyst loading (see Table S4†). The tris(calixarene) 20 was obtained in 22% yield which is the highest one among the syntheses involving the tetrakis(azide) 11. The full-1,3-alternate calix[4]semitube 21 was obtained in 11% yield from calixarenes 8 and 11 using 1.0 equiv. of the CuI/Et3N catalyst at room temperature, which were found to be the best conditions (Table S5;† 1,2-dichloroethane was used as the co-solvent due to the lower solubility of the calixarene 8 in neat toluene).
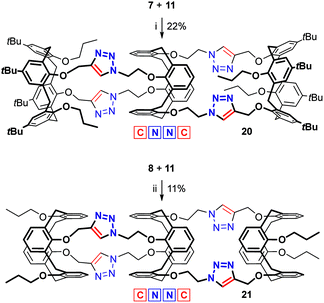 |
| Scheme 5 Syntheses of calix[4]semitubes 20 and 21: (i) CuI (1.0 equiv. per bis(azide)), Et3N, toluene, 70 °C, 9 h; and (ii) CuI (1.0 equiv. per bis(azide)), Et3N, toluene/1,2-dichloroethane, rt, 48 h. | |
Lower yields of the hydroxylated calix[4]semitubes 18 and 19 in comparison with those of the isomeric semitubes 14 and 15 allow us to propose that two azide groups (which are weak copper-targeted ligands) in calixarenes 1 and 2 are relatively well pre-organized by the reacting Cu-cations during CuAAC to allow their simultaneous connection to the same bisalkyne site of the tetrakis(alkyne) 9, whereas two propargyl groups in calixarenes 5 and 6 are not held together by the reacting cation(s) near the bisazide site of the calixarene 11, thus favoring the formation of polymeric/oligomeric products. As for the propylated terminal units of the semitubes (of either a cone or 1,3-alternate shape), the difference in the reactivities of the respective bis(azides) and bis(alkynes) with the central cores 9 or 11 is not easy to rationalize, because too many factors must be taken into account, including shielding the copper cation(s) by the propyl groups (cone isomers) or inverted calixarene aromatics (1,3-alternate isomers), repulsion between the cations bound to bistriazole sites,9d and their transfer between pairs of closely located azide9e or acetylene groups.9a
In general, though triazolated calix[4]semitubes can be obtained by CuAAC using the symmetrical central cores 9 and 11, only the hydroxylated semitubes (especially the tris(calixarenes) 14 and 15 prepared in good yields) seem to have synthetic perspectives upon further chemical transformations of the OH groups. As for the semitubes constructed from the exhaustively alkylated calixarenes, further development in this direction can be seriously hampered by the need for condition tuning for each combination of the CuAAC counterparts, by the low yields in the syntheses, and by the difficulties in the separation of triazolated by-products from the targeted semi-tubular assemblies.
Stepwise assembling of triscalixarene semitubes
Unlike the tetrafunctional molecules 9 and 11, the partially protected azide/alkyne-containing calixarene 13 allows a stepwise synthesis of long triazolated calix[4]semitubes when involved in CuAAC with bis(acetylenes) followed by de-protection and further reaction with bis(azides). The obvious advantage of this approach is that two but not four triazole heterocycles are formed during each of the CuAAC steps, which shrinks the range of triazolated by-products and thus improves the yield of the targeted semi-tubular bis(calixarenes). On the other hand, the stepwise syntheses using the calixarene 13 cannot replace the one-step preparation of the C2v-symmetrical calix[4]semitubes 14, 15, and 17–21, as only the Cs-symmetrical calix[4]semitubes can be obtained by the stepwise approach.
The CuAAC between calixarenes 13 and 8 (Scheme 6) was conducted using a copper(II) sulphate/sodium ascorbate catalytic system following the conditions previously found for assembling calixarenes 4 and 8 into a biscalixarene semitube,8 which were inefficient for the preparation of the tris(calixarenes) 17 and 21 (see above). The yield of the silylated bis(calixarene) 22 reached 45%, so no further tuning of the reaction conditions was required in this case. tert-Butyldimethylsilyl protecting groups were removed by treating the compound 22 with tetrabutylammonium fluoride in aqueous THF, and the calix[4]semitube 23 bearing two terminal acetylene groups ready for further CuAAC was obtained.
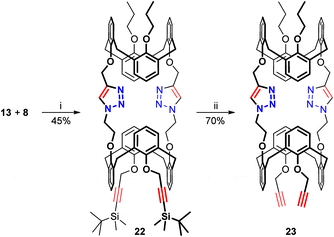 |
| Scheme 6 Syntheses of calix[4]semitubes 22 and 23: (i) CuSO4·5H2O (1.0 equiv. per bis(azide)/bis(alkyne)), sodium ascorbate, THF/H2O (5 : 1), 60 °C, 24 h; (ii) nBu4NF·3H2O, THF/H2O (100 : 1), 50 °C, 12 h. | |
With no additional tuning of the reaction conditions, the bis(calixarene) 23 was reacted with the bis(azide) 4 for obtaining the triscalixarene semitube 24 in 26% yield (Scheme 7). This compound is the isomer of both the calix[4]semitubes 17 and 21 but has lower symmetry as the two pairs of triazole linkers have different orientations with respect to the central calixarene core. The difference in the molecular symmetry immediately affects the patterns in the NMR spectra of the semitubes, thus allowing clear distinction of these three structures (Fig. 4). In particular, the 1H NMR spectrum of the less symmetrical calix[4]semitube 24 contains two signals from the triazole protons and three sets of signals from the methylene bridges of three different calixarene cores (Fig. 4b), while for the semitubes 17 (Fig. 4a) and 21 (Fig. 4c) single resonance from the triazole protons and only two sets of signals from the methylene bridges appear in each case.
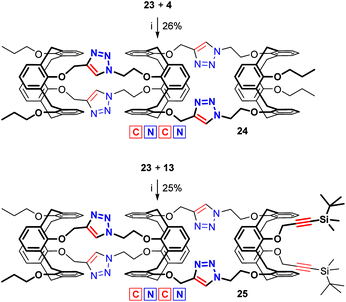 |
| Scheme 7 Syntheses of calix[4]semitubes 24 and 25: (i) CuSO4·5H2O (1.0 equiv. per bis(azide)/bis(alkyne)), sodium ascorbate, THF/H2O (5 : 1), 60 °C, 24 h. | |
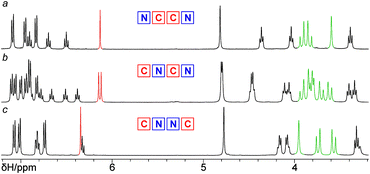 |
| Fig. 4 Parts of the 1H NMR spectra of (a) the calix[4]semitube 17, (b) calix[4]semitube 24, and (c) calix[4]semitube 21; 400 MHz, CDCl3; the signals from the triazole protons are colored red and those from the methylene groups of the calixarene cores are colored green. | |
Similar to the synthesis of the calix[4]semitube 24, the propargylated bis(calixarene) 23 was reacted with the bis(azide) 13, which was the synthetic precursor of the bis(calixarene) 23 (Scheme 7). As a result, the triscalixarene semitube 25 having protected acetylene units in the structure was obtained in 25% yield. This allows us to expect that further growth of the tubular architectures will be possible by step-by-step attachment of the bis(azide) 13 to the propargylated semitubes using alternating de-silylation and CuAAC procedures. It seems that the stepwise approach enables more possibilities for the preparation of the long triazolated calix[4]semitubes including those having additional functional groups in the terminal calixarene units, which can be achieved by replacing the propyl groups with the functional ones in the bis(alkyne) 8 or/and in the bis(azide) 4.
The prepared triscalixarene semitubes of different types seem to be able to form complexes with transition metal cations, which follows from our previous studies on the receptor properties of triazolated calix[4]arenes9d as well as from the preliminary data on the complexation ability of the related biscalixarene semitubes towards silver and zinc cations.8 Still, taking into account the homo/heteroditopic nature of the triscalixarene semitubes and possible inter/intramolecular migrations of the bound cations between the triazole-containing binding sites, the evaluation of their receptor abilities requires the introduction of additional reporter (e.g. fluorescent) or other functional/receptor units to the molecules, which can be achieved using the synthetic approaches developed in this work.
Conclusions
We have shown that long triazolated calix[4]semitubes having three calixarene units in the structures can be assembled by using bifunctional and tetrafunctional calixarene azides and alkynes under CuAAC conditions. The four-fold CuAACs between the bifunctional terminal units of the cone or 1,3-alternate shape and the tetrafunctional 1,3-alternate central core have been shown to have limited applicability as condition tuning is required for each of the calixarene combinations, while the separated yields of the targeted assemblies have been modest in most cases. As an alternative, the two-step syntheses of the triscalixarene semitubes, which involved the calix[4]arene bearing two azide groups and two silylated acetylene groups as the key synthone, have been shown to be successfully conducted under similar conditions at each of the two-fold CuAAC steps and have allowed the preparation of the semitubes with different substituents in the terminal units or/and further growth of the semi-tubular assemblies.
It is believed that the obtained results will find application in the development of multitopic tubular hosts having different mutual orientations of the bistriazole cation-binding sites within the molecule, which in turn will allow the creation of stimuli-switchable and other sophisticated supramolecules operating by intramolecular oscillations of a bound cation between the binding sites.
Conflicts of interest
There are no conflicts to declare.
Acknowledgements
The financial support from the Russian Science Foundation (Grant No. 20-73-00109) is gratefully acknowledged. X-ray diffraction studies were performed at the Centre of Shared Equipment of IGIC RAS.
Notes and references
-
(a)
Calixarenes 2001, ed. Z. Asfari, V. Böhmer, J. Harrowfield and J. Vicens, Kluwer Academic, Dordrecht, 2001 Search PubMed
;
(b)
V. Böhmer, The Chemistry of Phenols, ed. Z. Rappoport, John Wiley & Sons, Ltd, Chichester, 2003, pp. 1369–1454 Search PubMed
;
(c)
Calixarenes in the Nanoworld, ed. J. Vicens, J. Harrowfield and L. Baklouti, Springer, Dordrecht, 2007 Search PubMed
;
(d)
Calixarenes and Beyond, ed. P. Neri, J. L. Sessler and M.-X. Wang, Springer International Publishing, 2016 Search PubMed
.
- For selected examples of ‘classic’ biscalixarene receptor molecules joined by at least two linkers, see:
(a) S. Ullmann, M. Börner, A. Kahnt, B. Abel and B. Kersting, Green-emissive Zn2+ complex supported by a macrocyclic Schiff-base/calix[4]arene-ligand: crystallographic and spectroscopic characterization, Eur. J. Inorg. Chem., 2021, 3691–3698 CrossRef CAS
;
(b) M. Gaeta, E. Rodolico, M. E. Fragalà, A. Pappalardo, I. Pisagatti, G. Gattuso, A. Notti, M. F. Parisi, R. Purrello and A. D'Urso, Self-assembly of discrete porphyrin/calix[4]tube complexes promoted by potassium ion encapsulation, Molecules, 2021, 26, 704 CrossRef CAS
;
(c) S. Ullmann, P. Hahn, P. Mini, K. L. Tuck, A. Kahnt, B. Abel, M. E. G. Suburu, C. A. Strassert and B. Kersting, Mixed-ligand lanthanide complexes supported by ditopic bis(imino-methyl)-phenol/calix[4]arene macrocycles: synthesis, structures, and luminescence properties of [Ln2(L2)(MeOH)2] (Ln = La, Eu, Tb, Yb), Dalton Trans., 2020, 49, 11179–11191 RSC
;
(d) K. Puchnin, M. Andrianova, A. Kuznetsov and V. Kovalev, Field-effect transition sensor for KI detection based on self-assembled calixtube monolayers, Biosens. Bioelectron., 2017, 98, 140–146 CrossRef CAS
;
(e) S. Moerkerke, V. Malytskyi, L. Marcélis, J. Wouters and I. Jabin, Selective recognition of quaternary ammonium ions and zwitterions by using a biomimetic bis-calix[6]arene-based receptor, Org. Biomol. Chem., 2017, 15, 8967–8974 RSC
;
(f) S. Moerkerke, J. Wouters and I. Jabin, Selective recognition of phosphatidylcholine lipids by a biomimetic calix[6]tube receptor, J. Org. Chem., 2015, 80, 8720–8726 CrossRef CAS
;
(g) K. Puchnin, D. Cheshkov, P. Zaikin, I. Vatsouro and V. Kovalev, Tuning conformations of calix[4]tubes by weak intramolecular interactions, New J. Chem., 2013, 37, 416–424 RSC
;
(h) K. Puchnin, P. Zaikin, D. Cheshkov, I. Vatsouro and V. Kovalev, Calix[4]tubes: an approach to functionalization, Chem. – Eur. J., 2012, 18, 10954–10968 CrossRef CAS
;
(i) C.-C. Tsai, I.-T. Ho, J.-H. Chu, L.-C. Shen, S.-L. Huang and W.-S. Chung, Synthesis of 9,10-bis-ketoenaminoanthryl and 9,10-bis-isoxazolylanthryl linked biscalix[4]arenes: atropisomers and molecular recognitions, J. Org. Chem., 2012, 77, 2254–2262 CrossRef CAS
;
(j) S.-Z. Li, J. Shi, K. Yang and J. Luo, Chemical resolution and chiral recognition of an inherently chiral biscalix[4]arene cone–partial cone conformer, Tetrahedron, 2012, 68, 8557–8563 CrossRef CAS
;
(k) H. Iwamoto, K. Niimi, T. Haino and Y. Fukazawa, Energetics of guest binding to calix[4]arene molecular containers, Tetrahedron, 2009, 65, 7259–7267 CrossRef CAS
;
(l) J. Morales-Sanfrutos, M. Ortega-Muñoz, J. Lopez-Jaramillo, F. Hernandez-Mateo and F. Santoyo-Gonzalez, Synthesis of calixarene-based cavitands and nanotubes by click chemistry, J. Org. Chem., 2008, 73, 7768–7771 CrossRef CAS PubMed
;
(m) G. V. Zyryanov and D. M. Rudkevich, Toward synthetic tubes for NO2/N2O4: design, synthesis, and host-guest chemistry, J. Am. Chem. Soc., 2004, 126, 4264–4270 CrossRef CAS PubMed
;
(n) S. E. Matthews, P. Schmitt, V. Felix, M. G. B. Drew and P. D. Beer, Calix[4]tubes:
a new class of potassium-selective ionophore, J. Am. Chem. Soc., 2002, 124, 1341–1353 CrossRef CAS
;
(o) P. Schmitt, P. D. Beer, M. G. B. Drew and P. D. Sheen, Calix[4]tube: a tubular receptor with remarkable potassium ion selectivity, Angew. Chem., Int. Ed. Engl., 1997, 36, 1840–1842 CrossRef CAS
.
- For selected examples of bis(thiacalixarenes) joined by at least two linkers, see:
(a) A. A. Muravev, E. A. Trushina, A. T. Yakupov, S. E. Solovieva and I. S. Antipin, Ag-selective nanotubes based on bisthiacalix[4]arene with ethylene sulfide bridges, Dokl. Chem., 2019, 487, 212–214 CrossRef CAS
;
(b) F. Yang, F. Yin, H. Guo, Z. Huang and X. Zhang, Syntheses and cations complexation properties of novel thiacalix[4]-1,3-aza-crown and bisthiacalix[4]-1,3-aza-crown, J. Inclusion Phenom. Macrocyclic Chem., 2010, 67, 49–54 CrossRef CAS
;
(c) V. Bhalla, J. N. Babu, M. Kumar, T. Hattori and S. Miyano, Synthesis and binding studies of novel thiacalixpodands and bisthiacalixarenes having O,O′′-dialkylated thiacalix[4]arene unit(s) of 1,3-alternate conformation, Tetrahedron Lett., 2007, 48, 1581–1585 CrossRef CAS
;
(d) C. Pérez-Casas and T. Yamato, 1,3-Alternate-thiacalix[4]arene-based dimer: synthesis and inclusion behavior, Chem. Eng. Commun., 2006, 193, 1185–1193 CrossRef
;
(e) V. Bhalla, M. Kumar, H. Katagiri, T. Hattori and S. Miyano, Synthesis and binding studies of novel bisthiacalix[4]arenes with diimime linkages, Tetrahedron Lett., 2005, 46, 121–124 CrossRef CAS
;
(f) V. Csokai, B. Balázs, G. Tóth, G. Horváth and I. Bitter, Unprecedented cyclisations of calix[4]arenes under the Mitsunobu protocol. Part 3: Thiacalix[4]crowns versus dimers, Tetrahedron, 2004, 60, 12059–12066 CrossRef CAS
;
(g) V. Csokai, A. Grün and I. Bitter, Unprecedented cyclisations of calix[4]arenes with glycols under the Mitsunobu protocol. Part 1: A new perspective for the synthesis of calixcrowns, Tetrahedron Lett., 2003, 44, 4681–4684 CrossRef CAS
;
(h) S. E. Matthews, V. Felix, M. G. B. Drew and P. D. Beer, Thiacalix[4]tube: synthesis, X-ray crystal structure and preliminary binding studies, New J. Chem., 2001, 25, 1355–1358 RSC
.
- For selected examples of bis(calixpyrroles) joined by at least two linkers, see:
(a) R. Molina-Muriel, J. R. Romero, Y. Li, G. Aragay and P. Ballester, The effect of solvent on the binding of anions and ion-pairs with a neutral [2]rotaxane, Org. Biomol. Chem., 2021, 19, 9986–9995 RSC
;
(b) S. Xiong, F. Chen, T. Zhao, A. Li, G. Xu, J. L. Sessler and Q. He, Selective inclusion of fluoride within the cavity of a two-wall bis-calix[4]pyrrole, Org. Lett., 2020, 22, 4451–4455 CrossRef CAS
;
(c) R. Molina-Muriel, G. Aragay, E. C. Escudero-Adán and P. Ballester, Switching from negative-cooperativity to no-cooperativity in the binding of ion-pair dimers by a bis(calix[4]pyrrole) macrocycle, J. Org. Chem., 2018, 83, 13507–13514 CrossRef CAS
;
(d) J. R. Romero, G. Aragay and P. Ballester, Ion-pair recognition by a neutral [2]rotaxane based on a bis-calix[4]pyrrole cyclic component, Chem. Sci., 2017, 8, 491–498 RSC
;
(e) A. Galán, E. C. Escudero-Adán and P. Ballester, Template-directed self-assembly of dynamic covalent capsules with polar interiors, Chem. Sci., 2017, 8, 7746–7750 RSC
;
(f) W. Wu, Z. Tian, S. Wang, C. Peng, H. Liu, S. Dai and D.-e. Jiang, Design of calix-based cages for CO2 capture, Ind. Eng. Chem. Res., 2017, 56, 4502–4507 CrossRef CAS
;
(g) Q. He, M. Kelliher, S. Bähring, V. M. Lynch and J. L. Sessler, A bis-calix[4]pyrrole enzyme mimic that constrains two oxoanions in close proximity, J. Am. Chem. Soc., 2017, 139, 7140–7143 CrossRef CAS PubMed
;
(h) I. Saha, J. H. Lee, H. Hwang, T. S. Kim and C.-H. Lee, Remarkably selective, non-linear allosteric regulation of anion binding by a tetracationic calix[4]pyrrole homodimer, Chem. Commun., 2015, 51, 5679–5682 RSC
;
(i) V. Valderrey, E. C. Escudero-Adán and P. Ballester, Highly cooperative binding of ion-pair dimers and ion quartets by a bis(calix[4]pyrrole) macrotricyclic receptor, Angew. Chem., Int. Ed., 2013, 52, 6898–6902 CrossRef CAS
;
(j) V. Valderrey, E. C. Escudero-Adán and P. Ballester, Polyatomic anion assistance in the assembly of [2]pseudorotaxanes, J. Am. Chem. Soc., 2012, 134, 10733–10736 CrossRef CAS
.
- For selected examples of bis(calixresorcinarenes), see:
(a) W. Wang, K. Su, E.-S. M. El-Sayed, M. Yang and D. Yuan, Solvatomorphism influence of porous organic cage on C2H2/CO2 separation, ACS Appl. Mater. Interfaces, 2021, 13, 24042–24050 CrossRef CAS PubMed
;
(b) Q. Xiao, W.-W. Haoyang, T. Lin, Z.-T. Li, D.-W. Zhang and J.-L. Hou, Unimolecular artificial transmembrane channels showing reversible ligand-gating behavior, Chem. Commun., 2021, 57, 863–866 RSC
;
(c) D. Danielsiek and G. Dyker, An adaptive resorcinarene hemicarcerand, Eur. J. Org. Chem., 2021, 1026–1034 CrossRef CAS
;
(d) K. Harada, R. Sekiya and T. Haino, Folding and unfolding of acetoxy group-terminated alkyl chains inside a size-regulable hemicarcerand, J. Org. Chem., 2021, 86, 4440–4447 CrossRef CAS PubMed
;
(e) K. Tamaki, A. Ishigami, Y. Tanaka, M. Yamanaka and K. Kobayashi, Self-assembled boronic ester cavitand capsules with various bis(catechol) linkers: cavity-expanded and chiral capsules, Chem. – Eur. J., 2015, 21, 13714–13722 CrossRef CAS
;
(f) M. Vazdar, A. S. Mitchell, R. N. Warrener and D. Margetić, High pressure promoted exchange of guests from hemicarceplexes, Tetrahedron, 2015, 71, 550–553 CrossRef CAS
;
(g) S. Bringmann, R. Brodbeck, R. Hartmann, C. Schäfer and J. Mattay, Anthracene-resorcin[4]arene-based capsules: synthesis and photoswitchable features, Org. Biomol. Chem., 2011, 9, 7491–7499 RSC
;
(h) N. K. Beyeh, A. Valkonen and K. Rissanen, Piperazine bridged resorcinarene cages, Org. Lett., 2010, 12, 1392–1395 CrossRef CAS
.
- For selected examples of hybrid multimacrocycles from the calixarene family, see:
(a) S. K. Kim, V. M. Lynch, B. P. Hay, J. S. Kim and J. L. Sessler, Ion pair-induced conformational motion in calix[4]arene-strapped calix[4]pyrroles, Chem. Sci., 2015, 6, 1404–1413 RSC
;
(b) B. Sadhu, M. Sundararajan, G. Velmurugan and P. Venuvanalingam, Elucidating the structures and cooperative binding mechanism of cesium salts to the multitopic ion-pair receptor through density functional theory calculations, Dalton Trans., 2015, 44, 15450–15462 RSC
;
(c) S. K. Kim, V. M. Lynch and J. L. Sessler, Cone calix[4]arene diethyl ester strapped calix[4]pyrrole: a selective receptor for the fluoride anion, Org. Lett., 2014, 16, 6128–6131 CrossRef CAS PubMed
;
(d) S. K. Kim, V. M. Lynch, N. J. Young, B. P. Hay, C.-H. Lee, J. S. Kim, B. A. Moyer and J. L. Sessler, KF and CsF recognition and extraction by a calix[4]crown-5 strapped calix[4]pyrrole multitopic receptor, J. Am. Chem. Soc., 2012, 134, 20837–20843 CrossRef CAS PubMed
;
(e) P. Thiampanya, N. Muangsin and B. Pulpoka, Azocalix[4]arene strapped calix[4]pyrrole: a confirmable fluoride sensor, Org. Lett., 2012, 14, 4050–4053 CrossRef CAS
;
(f) I.-W. Park, S.-K. Kim, M.-J. Lee, V. M. Lynch, J. L. Sessler and C.-H. Lee, Calix[4]pyrrole–calix[4]arene–crown-5 conjugate with flexible linkers as a model for a selective ion-pair container, Chem. – Asian J., 2011, 6, 2911–2915 CrossRef CAS
;
(g) S. K. Kim, J. L. Sessler, D. E. Gross, C.-H. Lee, J. S. Kim, V. M. Lynch, L. H. Delmau and B. P. Hay, A calix[4]arene strapped calix[4]pyrrole: an ion-pair receptor displaying three different cesium cation recognition modes, J. Am. Chem. Soc., 2010, 132, 5827–5836 CrossRef CAS PubMed
;
(h) M. H. Patel and P. S. Shrivastav, Casting heteracalixarenes from calixarene templates: a unique synthetic strategy, Chem. Commun., 2009, 586–588 RSC
;
(i) J. L. Sessler, S. K. Kim, D. E. Gross, C.-H. Lee, J. S. Kim and V. M. Lynch, Crown-6-calix[4]arene-capped calix[4]pyrrole: an ion-pair receptor for solvent-separated CsF ions, J. Am. Chem. Soc., 2008, 130, 13162–13166 CrossRef CAS PubMed
;
(j) F. Yang, X. Zhao, H. Guo, J. Lin and Z. Liu, Syntheses and extraction properties of novel biscalixarene and thiacalix[4]arene hydrazone derivatives, J. Inclusion Phenom. Macrocyclic Chem., 2008, 61, 139–145 CrossRef CAS
;
(k) E. Khomich, M. Kashapov, I. Vatsouro, E. Shokova and V. Kovalev, Substituent control of potassium and rubidium uptake by asymmetric calix[4]-thiacalix[4]tubes, Org. Biomol. Chem., 2006, 4, 1555–1560 RSC
.
-
(a) V. G. Organo, V. Sgarlata, F. Firouzbakht and D. M. Rudkevich, Long synthetic nanotubes from calix[4]arenes, Chem. – Eur. J., 2007, 13, 4014–4023 CrossRef CAS PubMed
;
(b) V. G. Organo and D. M. Rudkevich, Emerging host–guest chemistry of synthetic nanotubes, Chem. Commun., 2007, 3891–3899 RSC
;
(c) E. Wanigasekara, A. V. Leontiev, V. G. Organo and D. M. Rudkevich, Supramolecular, calixarene-based complexes that release NO gas, Eur. J. Org. Chem., 2007, 2254–2256 CrossRef CAS
;
(d) V. G. Organo, A. V. Leontiev, V. Sgarlata, H. V. R. Dias and D. M. Rudkevich, Supramolecular features of calixarene-based synthetic nanotubes, Angew. Chem., Int. Ed., 2005, 44, 3043–3047 CrossRef CAS PubMed
;
(e) V. Sgarlata, V. G. Organo and D. M. Rudkevich, A procedure for filling calixarene nanotubes, Chem. Commun., 2005, 5630–5632 RSC
;
(f) S. K. Kim, J. Vicens, K.-M. Park, S. S. Lee and J. S. Kim, Complexation chemistry. Double- and multi-1,3-alternate-calixcrowns, Tetrahedron Lett., 2003, 44, 993–997 CrossRef CAS
;
(g) S. K. Kim, J. K. Lee, S. H. Lee, M. S. Lim, S. W. Lee, W. Sim and J. S. Kim, Silver ion shuttling in the trimer-mimic thiacalix[4]crown tube, J. Org. Chem., 2004, 69, 2877–2880 CrossRef CAS
;
(h) A. Ikeda and S. Shinkai, Multiple connection of 1,3-alternate-calix[4]arenes. An approach to synthetic ‘Nano-tubes’, J. Chem. Soc., Chem. Commun., 1994, 2375–2376 RSC
.
- A. Gorbunov, N. Ozerov, M. Malakhova, A. Eshtukov, D. Cheshkov, S. Bezzubov, V. Kovalev and I. Vatsouro, Assembling triazolated calix[4]semitubes by means of copper(I)-catalyzed azide–alkyne cycloaddition, Org. Chem. Front., 2021, 8, 3853–3866 RSC
.
- For our studies on the features and applications of CuAAC in calixarene chemistry, see:
(a) A. Gorbunov, D. Cheshkov, V. Kovalev and I. Vatsouro, Copper(I)-catalyzed cycloaddition of azides to multiple alkynes: a selectivity study using a calixarene framework, Chem. – Eur. J., 2015, 21, 9528–9534 CrossRef
;
(b) A. Gorbunov, N. Sokolova, E. Kudryashova, V. Nenajdenko, V. Kovalev and I. Vatsouro, Chiral heteroditopic baskets designed from triazolated calixarenes and short peptides, Chem. – Eur. J., 2016, 22, 12415–12423 CrossRef CAS PubMed
;
(c) B. Bolshchikov, S. Volkov, D. Sokolova, A. Gorbunov, A. Serebryannikova, I. Gloriozov, D. Cheshkov, S. Bezzubov, W.-S. Chung, V. Kovalev and I. Vatsouro, Constructing bridged multifunctional calixarenes by intramolecular indole coupling, Org. Chem. Front., 2019, 6, 3327–3341 RSC
;
(d) A. Gorbunov, J. Kuznetsova, K. Puchnin, V. Kovalev and I. Vatsouro, Triazolated calix[4]arenes from 2-azidoethylated precursors: is there a difference in the way the triazoles are attached to narrow rims?, New J. Chem., 2019, 43, 4562–4580 RSC
;
(e) A. Gorbunov, J. Kuznetsova, I. Deltsov, A. Molokanova, D. Cheshkov, S. Bezzubov, V. Kovalev and I. Vatsouro, Selective azide–alkyne cycloaddition reactions of azidoalkylated calixarenes, Org. Chem. Front., 2020, 7, 2432–2441 RSC
;
(f) A. Gorbunov, A. Iskandarova, K. Puchnin, V. Nenajdenko, V. Kovalev and I. Vatsouro, A route to virtually unlimited functionalization of water-soluble p-sulfonatocalix[4]arenes, Chem. Commun., 2020, 56, 4122–4125 RSC
;
(g) S. Bezzubov, K. Ermolov, A. Gorbunov, P. Kalle, I. Lentin, G. Latyshev, V. Kovalev and I. Vatsouro, Inherently dinuclear iridium(III) meso architectures accessed by cyclometalation of calix[4]arene-based bis(aryltriazoles), Dalton Trans., 2021, 50, 16765–16769 RSC
.
- Z.-G. Luo, Y. Zhao, F. Xu, C. Ma, X.-M. Xu and X.-M. Zhang, Synthesis and thermal properties of novel calix[4]arene derivatives containing 1,2,3-triazole moiety via, K2CO3-catalyzed 1,3-dipolar cycloaddition reaction, Chin. Chem. Lett., 2014, 25, 1346–1348 CrossRef CAS
.
- Z. Asfari, A. Bilyk, C. Bond, J. M. Harrowfield, G. A. Koutsantonis, N. Lengkeek, M. Mocerino, B. W. Skelton, A. N. Sobolev, S. Strano, J. Vicens and A. H. White, Factors influencing solvent adduct formation by calixarenes in the solid state, Org. Biomol. Chem., 2004, 2, 387–396 RSC
.
- W. Xu, J. J. Vittal and R. J. Puddephatt, Propargyl calix[4]arenes and their complexes with silver(I) and gold(I), Can. J. Chem., 1996, 74, 766–774 CrossRef CAS
.
- S. Cecioni, R. Lalor, B. Blanchard, J.-P. Praly, A. Imberty, S. E. Matthews and S. Vidal, Achieving high affinity towards a bacterial lectin through multivalent topological isomers of calix[4]arene glycoconjugates, Chem. – Eur. J., 2009, 15, 13232–13240 CrossRef CAS PubMed
.
- V. A. Burilov, N. A. Epifanova, E. V. Popova, S. F. Vasilevsky, S. E. Solovieva, I. S. Antipin and A. I. Konovalov, Regioselective synthesis of 1,2,3-triazolyl derivatives of calix[4]arenes based on 1,3-dipolar cycloaddition, Russ. Chem. Bull., 2013, 62, 767–772 CrossRef CAS
.
- The CIF file has been deposited with the Cambridge Crystallographic Data Centre: CCDC 2157422.† Details of X-ray diffraction measurements are provided in the ESI.†.
Footnote |
† Electronic supplementary information (ESI) available: Experimental details and the NMR spectra of novel compounds. CCDC 2157422. For ESI and crystallographic data in CIF or other electronic format see DOI: https://doi.org/10.1039/d2qo00432a |
|
This journal is © the Partner Organisations 2022 |
Click here to see how this site uses Cookies. View our privacy policy here.