DOI:
10.1039/D1RA08616B
(Paper)
RSC Adv., 2022,
12, 3073-3080
A salicylaldehyde benzoyl hydrazone based near-infrared probe for copper(II) and its bioimaging applications†
Received
24th November 2021
, Accepted 15th January 2022
First published on 24th January 2022
Abstract
Developing highly sensitive and selective methods for Cu2+ detection in living systems is of great significance in clinical copper-related disease diagnosis. In this work, a near infrared (NIR) fluorescent probe, CySBH, with a salicylaldehyde benzoyl hydrazone group as a selective and sensitive receptor for Cu2+ was designed and synthesized. The specific coordination of the salicylaldehyde benzoyl hydrazone group in CySBH with Cu2+ can induce a distinct quench of the fluorescence intensity, allowing for real-time tracking of Cu2+. We have demonstrated that CySBH could rapidly recognize Cu2+ with good selectivity and high sensitivity. Moreover, on the basis of low cell cytotoxicity, the probe was used to visualize Cu2+ in two cell lines by fluorescence imaging. Furthermore, CySBH can also be used to monitor Cu2+ in vivo due to its NIR emission properties. These overall results illustrate that the NIR fluorescent probe CySBH provides a novel approach for the selective and sensitive monitoring of Cu2+ in living systems.
1. Introduction
Copper(II) ions (Cu2+) are the third most abundant transition metal ions in the human body and play significant roles in various fundamental physiological processes, such as detoxifying oxygen-derived radicals, signal transduction and coagulating blood.1–3 Excess accumulation of Cu2+ in the body can lead to serious consequences, such as cellular damage, Menkes disease, Wilson disease, cancer, cardiovascular disorders, Alzheimer's disease and even death.4–6 Therefore, maintaining Cu2+ at appropriate concentrations is vital for the growth and fitness of living organisms. Given the significance of copper to human health, the selective and sensitive monitoring of Cu2+ ions in living systems is of great importance to evaluate copper-related diseases.
In recent years, many methods, such as inductively coupled plasma-mass spectrometry,7,8 electrochemical and electrochemical luminescence sensors,9–11 colorimetric methods12,13 and fluorescent detection,14–17 have been successfully developed for the detection of Cu2+. Among them, technology based on fluorescence probes has become one of the most important methods to detect Cu2+ in living systems because of its quick response, high sensitivity, low detection limit reliability and low cost.18–21 Great efforts have been made in the development of novel fluorescence probes for Cu2+ detection. Additionally, fluorescence probes in the near-infrared window (NIR, 650–900 nm) have attracted great interest due to their incomparable advantages, such as low auto-fluorescence of biosystems and deep penetration into tissue.22–26 To date, various NIR fluorescence probes with different recognition mechanisms have been designed and used for Cu2+ detection.27–30 One of the most important approaches to design NIR fluorescent probes for copper ion detection is to graft a Cu2+ selective receptor unit to the fluorophore.31,32 For example, Li et al. developed a NIR fluorescence probe bearing 2-(1H-benzo[d]imidazol-2-yl)-phenol as a copper ion receptor.33 Zhang et al. described a 2-picolinic ester-based NIR probe, NIR-Cu, for monitoring Cu2+ in vivo.34 Tang's group developed a NIR fluorescent copper probe and successfully applied it to visualize Cu2+ in living cells, rat hippocampal tissues and zebrafish models.35 In addition, some probes can recognize Cu2+ based on rhodamine by a closed-state spiro ring.36,37 However, due to the limitations of the recognition group in selectivity and sensitivity, those probes still encounter some problems, such as poor selectivity, slow response and poor photostability.38,39 Therefore, the development of novel NIR fluorescent probes with selective and sensitive receptors for Cu2+ would be of great value.
Salicylidene acylhydrazone Schiff bases are one of most important π-conjugated ligands for Cu2+. In addition, these compounds display keto–enol tautomerism, and both the nitrogen atom and oxygen atom in the Schiff bases can act as ligand donors for Cu2+ and consequently produce stable coordination complexes via coordination effects.40,41 The phenol group in phenol-based Schiff base units is deprotonated in the coordination process, which could diminish the electron-donating ability of the oxygen atom.42–46 Moreover, it has been reported that the fluorescence signal of hemicyanine-based NIR fluorophores could be turned in a controllable manner by diminishing the electron-donating ability of the oxygen atom in its phenol group. Therefore, a hemicyanine-based NIR fluorescent probe using salicylidene acylhydrazone Schiff bases as a selective recognition group has great potential in the selective recognition of Cu2+.
In this study, we proposed a novel Cu2+ detection probe, CySBH, in which an important NIR fluorophore hemicyanine was connected with benzoyl hydrazine via an imine bond. The phenolic hydroxyl and acyl hydrazone groups in CySBH tactfully formed a special salicylaldehyde benzoyl hydrazone group. The salicylaldehyde benzoyl hydrazone group is known to be a good coordinating site for Cu2+ due to the imine nitrogen atom, amide oxygen atom and phenolic hydroxyl oxygen atom, which could be used as a selective coordination unit for Cu2+ (Scheme 1a). In the presence of Cu2+, the salicylaldehyde benzoyl hydrazone group on CySBH can effectively coordinate with copper ions, resulting in the NIR fluorescent signal of CySBH being quenched. The responses of CySBH toward Cu2+ were fully proven by UV-vis absorption, fluorescence and mass analysis. Furthermore, we also demonstrated that CySBH can react with intracellular Cu2+ and effectively turn off fluorescence in living cells and mice. Such fluorescence responses of CySBH in this work provide a novel approach for the selective and sensitive monitoring of Cu2+ in living systems.
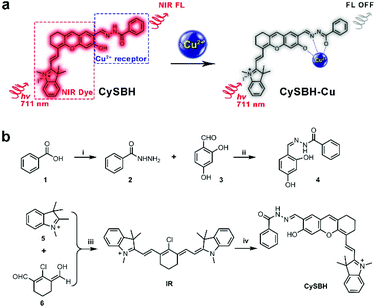 |
| Scheme 1 (a) Chemical structure and proposed activation mechanism of the probe CySBH mediated by Cu2+. (b) Synthesis of CySBH. Conditions: (i) thionyl chloride, EtOH, hydrazine hydrate; (ii) EtOH, r.t.; (iii) sodium acetate trihydrate, acetic anhydride, 75 °C; (iv) K2CO3, CH3CN, 60 °C. | |
2. Experimental
2.1 Materials and instruments
Benzoic acid and 2,4-dihydroxybenzaldehyde were obtained from J&K Scientific Co., Ltd (Beijing, China). Copper chloride (CuCl2), cuprous chloride (CuCl), zinc chloride (ZnCl2), calcium chloride (CaCl2) and mercury dichloride (HgCl2) were purchased from Tianjin Kemiou Chemical Reagent Co., Ltd (Tianjin, China). Ferric chloride (FeCl3·6H2O), manganese chloride (MnCl2), nickel chloride hexahydrate (NiCl2·6H2O), potassium chloride (KCl) and sodium chloride (NaCl) were purchased from Rionlon Bohua Pharmaceutical & Chemical Co., Ltd (Tianjin, China). All other chemicals were purchased from qualified reagent supplies with analytical reagent grade and used without further purification.
The NMR spectra were recorded on a Bruker AVANCE III-400 spectrometer, and the chemical shifts of 1H NMR were expressed in parts per million downfield relative to the internal tetramethylsilane (δ = 0 ppm). High-resolution electrospray ionization mass spectra (HR-ESIMS) were acquired on a Bruker microTOF-Q II mass spectrometer (Bruker Daltonics, Billerica, MA). UV-vis extinction spectra were recorded on a UVvis spectrophotometer (PerkinElmer, Waltham, USA). The fluorescence spectra were measured on a fluorescence spectrophotometer (Fluoromax-4, HORIBA, USA). Fluorescence images of cells were acquired on an Olympus Fluoview 1000 confocal laser scanning microscope (Olympus, Tokyo, Japan). Whole-body in vivo fluorescence images and ex vivo fluorescence images of different organs of mice were obtained using a VISQUE™ In Vivo Elite Preclinical Optical Imaging System (Vieworks Co., Ltd Korea). Ultrapure water was prepared by an OKP purification system (Shanghai Laikie Instrument Co., Ltd, China).
2.2 Synthesis of compound 4
A mixture of 2,4-dihydroxybenzaldehyde (1.38 g) and benzhydrazide (1.36 g) in ethanol (50 mL) was stirred at room temperature for 3 h. A large amount of precipitate was precipitated. The precipitate was collected and further purified to provide compound 4. 1H NMR (400 MHz, DMSO-d6): δ 11.89 (s, 1H), 11.46 (s, 1H), 9.94 (s, 1H), 8.48 (s, 1H), 7.88 (d, J = 7.6 Hz, 2H), 7.48–7.56 (m, 3H), 7.27 (d, J = 8.4 Hz, 1H), 6.33 (d, J = 8.4 Hz, 1H), 6.30 (s, 1H). 13C NMR (101 MHz, DMSO-d6): δ 163.0, 161.2, 160.0, 149.7, 133.5, 132.3, 131.9, 129.0, 128.0, 111.0, 108.2, 103.2. HRMS calcd for C14H12N2Na: 279.0740 [M + Na]+; found, 279.0752 [M + Na]+.
2.3 Synthesis of compound IR
For the synthesis of compound IR, a mixture of compound 5 (260 mg), sodium acetate trihydrate (408 mg) and compound 6 (0.9 g) in acetic anhydride (10 mL) was heated at 75 °C for 4 h under a nitrogen atmosphere. The mixture was cooled with green precipitation, which was collected by filtration. The mixture was cooled with a large amount of green precipitate. The precipitate was collected and further purified by column chromatography to provide compound IR as a dark green solid. 1H NMR (400 MHz, DMSO-d6): δ 8.23 (d, J = 14.0 Hz, 2H), 7.63 (d, J = 7.6 Hz, 2H), 7.40–7.44 (m, 4H), 7.26–7.28 (m, 2H), 6.30 (d, J = 14.0 Hz, 2H), 3.68 (s, 6H), 2.70–2.72 (m, 4H), 1.83–1.85 (m, 2H), 1.66 (s, 12H). 13C NMR (101 MHz, DMSO-d6): δ 172.6, 147.6, 142.8, 142.6, 140.9, 128.5, 126.0, 125.1, 122.3, 111.4, 101.8, 48.8, 31.5, 27.3, 25.8, 20.4.
2.4 Synthesis of the probe CySBH
K2CO3 (552 mg) was added to a solution of compound IR (610 mg) and compound 4 (281 mg) in dry CH3CN (10 mL). The mixture was stirred at 60 °C for 8 h under a nitrogen atmosphere. The solvent was evaporated, and the crude product was purified by column chromatography using CH2Cl2/MeOH (20
:
1) to provide compound CySBH as a dark blue solid. 1H NMR (400 MHz, CD3OD-CDCl3): δ 8.59 (s, 1H), 8.34 (d, J = 13.6 Hz, 1H), 8.20 (s, 1H), 7.91 (d, J = 7.2 Hz, 1H), 7.60 (s, 1H), 7.49–7.54 (m, 4H), 7.30–7.36 (m, 2H), 7.14 (t, J = 7.6 Hz, 1H), 7.00 (d, J = 8.0 Hz, 1H), 6.56 (s, 1H), 5.79 (d, J = 13.6 Hz, 1H), 3.47 (s, 3H), 2.72–2.75 (m, 2H), 2.61–2.64 (m, 2H), 1.90–1.92 (m, 2H), 1.70 (s, 6H). 13C NMR (400 MHz, CD3OD-CDCl3): δ 170.7, 66.0, 163.1, 159.2, 146.0, 143.5, 142.1, 140.5, 138.6, 133.3, 132.3, 128.8, 128.8, 127.9, 127.4, 124.3, 122.5, 120.4, 116.1, 114.7, 109.5, 104.5, 97.1, 30.8, 28.7, 28.6, 24.7, 21.3. HRMS calcd for C34H32N3O3: 530.2438 [M]+; found, 530.2426 [M]+.
2.5 Preparation of stock solutions
Stock solutions (10 mM) of the chlorate salts of Cu+, Mg2+, Ca2+, Ba2+, Pb2+, K+, Na+, Ni2+, Fe3+, Hg2+, Zn2+, Al3+, Mn2+, Ag+, Cr3+, Cd2+, Li+ and Cu2+ ions were prepared in distilled water. The stock solutions were then diluted to appropriate concentrations with PBS (10 mM, pH 7.4) for further use.
2.6 General procedures for UV-vis and fluorescence spectra methods
For the UV-vis absorption and fluorescence spectra of CySBH measurements, a 10 μM CySBH working solution in phosphate saline buffer (PBS, 10 mM, pH 7.4, 1% DMSO) was used. Various analytes were added to the working solution, and the reactions were performed at room temperature for different times. Finally, UV-vis absorption was measured in CH3CN/PBS buffer (v/v = 1
:
1, 5 mM, pH 7.4), while fluorescence spectra were acquired in CH3CN/PBS buffer (v/v = 1
:
1, 5 mM, pH 7.4). For comparison, the solution that did not contain any interfering metal ions (blank) was measured under the same conditions at the same time.
2.7 Cell cultures and imaging
Human non-small-cell lung cancer (A549) cells and human cervical cancer (HeLa) cells were purchased from Morey Biosciences, Inc., and the cells were cultured according to the reported method. Both A549 and HeLa cells were grown in 10% fetal bovine serum (FBS) at 37 °C under a 5% CO2 atmosphere. For fluorescence microscopy, the cells were seeded on glass-bottom culture dishes and cultured in culture medium for 24 h, after which CySBH (5 μM) was added to the dishes and incubated at 37 °C for 30 min. The medium was then removed, and the dishes were washed with PBS buffer three times to remove the remaining CySBH. The cells were then incubated in the absence or presence of Cu2+ (20 μM) in culture medium for another 10 min at 37 °C. After that, fluorescence images were obtained by confocal laser scanning microscopy NIR channels (excitation wavelength at 633 nm and emission wavelength from 650 nm to 750 nm).
2.8 Cell viability assay
The cytotoxicity of CySBH against A549 and HeLa cells was assessed utilizing a standard MTT method. Typically, cells were seeded into 96-well plates. After incubation at 37 °C for 24 h, the medium was replaced by CySBH aqueous solutions at different concentrations (0, 10, 20, 50 and 100 μM). After incubating cells at 37 °C for 24 h, the medium was replaced with freshly prepared MTT (1 mg mL−1 in PBS) and incubated for 4 h. After removing the MTT medium solution, 100 μL of dimethyl sulfoxide (DMSO) was added to the cells. The plate was shaken for 10 min, and the optical density (OD) was monitored. Each of the experiments was performed five times.
2.9 Fluorescence imaging of CySBH in vivo
Chinese Kunming mice (male, 20–25 g) were purchased from Gansu Provincial Maternity and Child-care Hospital and used for the in vivo fluorescence imaging of CySBH. All animal experiments were approved by the Experimental Animal Use and Care Committee, Gansu Provincial Maternity and Child-care Hospital and in accordance with the principles of laboratory animal operation regulation. For in vivo fluorescence imaging studies, mice were anesthetized by isoflurane. The anesthetized mice were injected with PBS buffer, CySBH (10 μM), CySBH (10 μM) with Cu2+ (5 μM), CySBH (10 μM) with Cu2+ (10 μM) and CySBH (10 μM) with Cu2+ (15 μM) for 0.1 mL under 5 mm of the skin at the right hindlimb, respectively. Finally, the fluorescence imaging signals were collected on a VISQUE™ In Vivo Elite Preclinical Optical Imaging System using a 630–680 nm excitation filter and a 690–740 nm emission filter.
3. Results and discussion
3.1 Design, synthesis and characterization of CySBH
Hemicyanine dye derivatives with NIR absorption and emission have attracted great attention due to their distinct advantages, such as hypotoxicity, high photostability and high fluorescence quantum yield. We noticed that reducing the electron-donating ability of the oxygen atom at hemicyanine dye can endow the NIR fluorophore with unique fluorescent turn-off properties.47 Therefore, we anticipated that introduction of a benzoyl hydrazine in the hemicyanine dye could form a special salicylaldehyde benzoyl hydrazone group on the fluorophore.
The obtained compound could serve not only as an effective complexing agent for copper ions but also as a selective NIR probe to monitor Cu2+ in living systems. The synthesis procedure of probe CySBH is shown in Scheme 1b. Compounds 4 and IR were synthesized at first. The probe CySBH was prepared by reacting compound 4 with IR under basic conditions. The chemical structure of CySBH was fully characterized and is shown in the Experimental Section and the ESI.†
3.2 Spectroscopic properties
With CySBH in hand, its spectral responses toward Cu2+ were measured in CH3CN-PBS buffer (1
:
1) solution. As shown in Fig. 1a, probe CySBH initially exhibited two strong absorption peaks at 711 and 650 nm (red line). After being treated with Cu2+, significant spectral changes were observed. The characteristic absorption peaks of CySBH at 711 and 650 nm decreased, accompanied by two new blue-shifted absorption peaks at 690 and 637 nm (Fig. 1a, black line), and the solution color also changed from blue to cyan. Moreover, CySBH initially displays a strong fluorescence emission at 744 nm, but the addition of Cu2+ shows a 130-fold decrease in fluorescence intensity (Fig. 1b). These results suggest that probe CySBH is highly sensitive to Cu2+. The response time of the CySBH probe toward Cu2+ was studied by measuring the fluorescence intensity at 744 nm in real time. As shown in Fig. 1d, the fluorescence signal decreases instantaneously upon the addition of Cu2+ and reaches a plateau within 1.8 s. This result suggests that the coordination interaction between Cu2+ and CySBH can reach equilibrium very quickly. The sharp quenching of the original fluorescence signal of CySBH after incubation with Cu2+ could be ascribed to the fact that the salicylaldehyde benzoyl hydrazone group in CySBH can be efficiently coordinated with Cu2+, which reduces the electron-donating ability of the oxygen atom. The photostability of organic probes is crucial for practical applications in bioimaging. Therefore, the photostability of the CySBH probe was investigated by time-sequential scanning. As shown in Fig. S1,† after 1800 s of continuous irradiation with a 711 nm laser, negligible obvious fluorescence intensity changes were observed. These results indicated that the probe CySBH had sufficient photostability and can be used as an excellent NIR imaging agent for bioimaging. After that, the fluorescence titration of CySBH toward Cu2+ was carried out to examine the possibility of quantitative analysis of Cu2+. As shown in Fig. 1c, the addition of increasing amounts of Cu2+ triggered a gradual decrease in the fluorescence intensity at 744 nm when excited at a wavelength of 711 nm. The significant fluorescence on-off property suggests that the probe CySBH has excellent sensitivity toward Cu2+. Furthermore, plotting the fluorescence intensity at 744 nm against the concentration of Cu2+ resulted in a logarithmic response curve (Fig. 1c, inset), and a good linear equation (R2 = 0.994) was obtained in the Cu2+ concentration range from 0 to 2.5 μM, which can be used for the quantification of Cu2+ concentration. The detection limit (3S/m, in which S is the standard deviation of blank measurements, n = 10, and m is the slope of the linear equation) was calculated as 28.4 nM.
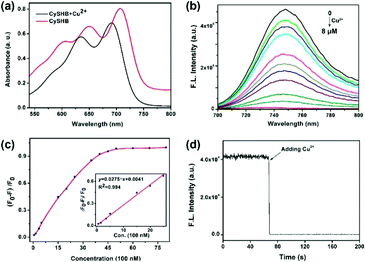 |
| Fig. 1 (a) UV-vis absorption spectra of CySBH (5 μM) before (red line) and after addiction of Cu2+ (8 μM, black line) in acetonitrile/PBS (5 mM PBS, 1 : 1, v/v; pH = 7.4). (b) Fluorescence spectra of CySBH (5 μM) after adding different concentrations of Cu2+ (0–8 μM) in acetonitrile/PBS (5 mM PBS, 1 : 1, v/v; pH = 7.4), λex = 690 nm. (c) Plot of the fluorescence emission intensity of CySBH (5 μM) at 744 nm toward the concentration of Cu2+. Inset: calibration curve in the concentration range of 0–2.5 μM Cu2+. F0 and F are the fluorescence intensities of CySBH (5 μM) in acetonitrile/PBS (5 mM PBS, 1 : 1, v/v; pH = 7.4) in the absence and presence of Cu2+, respectively. λex = 690 nm. (d). Real-time fluorescence intensity of CySBH (5.0 μM) at 744 nm (λex = 711 nm) upon the addition of Cu2+ (5 μM) in acetonitrile/PBS (5 mM PBS, 1 : 1, v/v; pH = 7.4). | |
3.3 Selectivity studies
The selectivity ability of the probe CySBH toward various potential interfering metal ions, including Cu+, Mg2+, Ca2+, Ba2+, Pb2+, K+, Na+, Ni2+, Fe3+, Hg2+, Zn2+, Al3+, Mn2+, Ag+, Cr3+, Cd2+ and Li+, was investigated. As shown in Fig. 2a, negligible fluorescence signal changes were observed when probe CySBH was added with the above substances under the same spectral conditions. In contrast, the fluorescence intensity ratio of CySBH increased nearly 130-fold after the addition of Cu2+. These results suggest that CySBH is highly sensitive and specific to Cu2+ ions in the presence of other metal ions. Competitive experiments were further employed with various metal ions to confirm the practical evaluation of CySBH as a selectivity probe for Cu2+. As shown in Fig. 2b, the addition of Cu2+ to the mixture of CySBH with different metal ions caused a significant increase in the fluorescence intensity ratio. These results show that the existence of excessive interference ions had little effect on the fluorescence response of CySBH to Cu2+, confirming the high selectivity of CySBH for the Cu2+ ion.
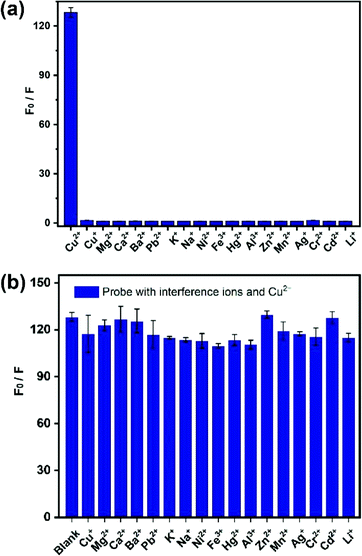 |
| Fig. 2 (a) Selectivity of the probe CySBH (5 μM) toward Cu2+ over 17 different metal ions (20 μM). (b) Competitive tests of CySBH (5 μM) toward various potential interfering metal ions (50 μM Cu+, Mg2+, Ca2+, Ba2+, Pb2+, K+, Na+, Ni2+, Fe3+, Hg2+, Al3+, Zn2+, Mn2+, Ag+, Cr2+, Cd2+ and Li+) in acetonitrile/PBS (5 mM PBS, 1 : 1, v/v; pH = 7.4) with Cu2+ (10 μM). F0 and F are the fluorescence intensities of CySBH (5 μM) in acetonitrile/PBS (5 mM PBS, 1 : 1, v/v; pH = 7.4) in the absence and presence of different concentrations of Cu2+, respectively. λex = 711 nm. | |
3.4 Stoichiometric reaction of the probe with Cu2+
It has been well documented that salicylidene acylhydrazone Schiff bases are one of most important π-conjugated ligands for Cu2+.38,44 In Schiff bases, the lone pair electrons of nitrogen and oxygen atoms in Schiff bases can occupy the 3d, 4s, and 4p empty electron orbitals of Cu2+ to form stable coordination complexes. In this work, we surmised that the nitrogen and oxygen atoms in Schiff bases could cooperate with Cu2+ to induce intramolecular electron transfer from the hemicyanine fluorophore moiety to Cu2+, resulting in the NIR fluorescence signal being quenched. The interaction between CySBH and Cu2+ was investigated by mass spectrometry and Job's plot. As shown in Fig. 3a, the HRMS results of the CySBH and Cu2+ complex provide the characteristic pecks of 591.1562 belonging to ([m/z CySBH] + [Cu2+]-2H), which confirms the existence of the 1:1 complex. In addition, the continuous variation method (Job's plot) was carried out to further verify the stoichiometry between CySBH and Cu2+. The differences in the fluorescence intensity before (F0) and after (F) the addition of Cu2+ at 744 nm exhibited a maximum at ∼0.5 (Fig. 3b), suggesting that the binding stoichiometry between CySBH and Cu2+ is 1:1. These results indicate the formation of a complex with 1:1 stoichiometric binding.
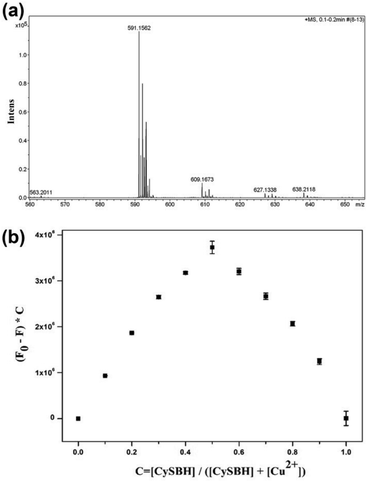 |
| Fig. 3 (a) HRMS spectra of CySBH after the addition of Cu2+. (b) Job's plot of the coordination ratio between CySBH and Cu2+. The fluorescence intensity at 744 nm was plotted as a function of the molar ratio (Cu2+/[CySBH + Cu2+]) (the total concentrations of CySBH and Cu2+ were 10 μM). | |
3.5 Effect of pH
Considering whether the probe can efficiently detect Cu2+ under varied pH values, the impact of pH on the sensing Cu2+ of CySBH was investigated. As shown in Fig. S2,† the fluorescence intensity of CySBH showed an obvious change over a wide pH range from 5 to 10 after the addition of Cu2+. Furthermore, the probe CySBH exhibited a remarkable fluorescence change under a neutral environment (pH = 7.0), which is beneficial for further application in living systems.
3.6 Method comparison
The performance of CySBH is investigated in terms of excitation/emission wavelength, limit of detection, selectivity and response time. A detailed comparison between CySBH and some related Cu2+ probes reported in recent years is shown in Table S1.† Compared with the Cu2+ probes in the list, the NIR probe proposed in this study has excellent selectivity and short response times, which can be attributed to the Cu2+ selective recognition group based on salicylidene acylhydrazone Schiff bases. In addition, the NIR emission characteristics of CySBH make it more suitable for the imaging analysis of Cu2+ in living systems. Taken together, these results suggested that CySBH can be used as an effective and promising tool to monitor Cu2+ ions in living systems.
3.7 Fluorescence imaging in living cells
Since we have proven that the CySBH probe can detect Cu2+ specifically among various potential interfering metal ions in an isolated chemical environment, its application as a fluorescent probe for monitoring Cu2+ in living cells was further investigated. First, to evaluate the possibility of CySBH being applied to the living system, the in vitro cytotoxicity of CySBH was evaluated by a standard MTT assay with HeLa and A549 cells. As shown in Fig. S3,† when HeLa and A549 cells were incubated with different concentrations of CySBH ranging from 0 to 100 μM for 24 h, both cell lines showed high cell viability, indicating the low cytotoxicity and favorable biocompatibility of CySBH. After that, the capability of CySBH to image Cu2+ in HeLa and A549 cells was determined. As shown in Fig. 4a and c, significant intracellular fluorescence signals were observed in both HeLa and A549 cells after incubation with CySBH (5 μM) at 37 °C for 30 min, demonstrating that CySBH had good cell permeability. However, when further treated with Cu2+ in the culture medium for another 10 min and washed with PBS to remove extracellular Cu2+, the intracellular fluorescence signals decreased dramatically in these cells (Fig. 4b and d). In fact, the A549 and HeLa cells after being treated with Cu2+ exhibited significantly lower fluorescence intensity than the untreated cells, with a P value less than 0.001 (Fig. S4†). This could be ascribed to the fact that the Cu2+ ion can effectively coordinate with CySBH in living cells and quench the fluorescence signal of CySBH. These results implied that CySBH can monitor Cu2+ sensitively and quickly in living cells.
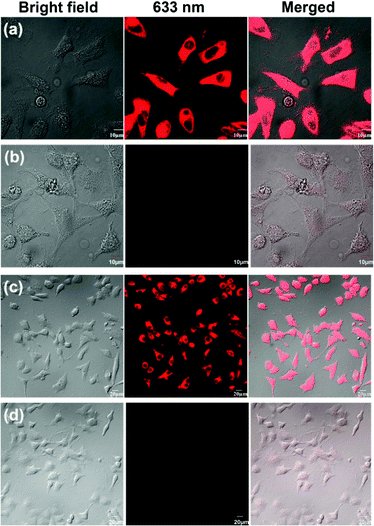 |
| Fig. 4 Fluorescence images of probe CySBH in the A 549 and HeLa cells. (a) and (c) are the corresponding images of A549 and HeLa cells incubated with CySBH (5 μM) for 30 min, respectively. (b) Images of A549 cells incubated with CySBH (5 μM) for 30 min and then further treated with Cu2+ (20 μM) for 10 min. (d) Images of HeLa cells incubated with CySBH (5 μM) for 30 min and then further treated with Cu2+ (20 μM) for 10 min. | |
3.8 In vivo fluorescence imaging of Cu2+ in mice
Near-infrared light can penetrate tissue deeply and has low background auto-fluorescence interference from biosystems. Since the probe CySBH displays favorable response properties toward Cu2+ in vitro, we simultaneously investigated the concentration-dependent response of CySBH toward Cu2+ in a living system via an in vivo fluorescence imaging method. Kunming mice were selected as the model and allocated into five groups. Fluorescence images in vivo were obtained using an in vivo optical imaging system. The first group of mice was treated with PBS buffer as a control group. As shown in Fig. 5a, there was no fluorescence emission when the mice were injected with PBS buffer. The second group of mice was treated with CySBH solution as a positive group. A strong fluorescence signal with a low background signal was observed in the right hindlimb of mice in the second group (Fig. 5b). The other groups of mice were treated with CySBH and Cu2+ at different concentrations. Interestingly, as the concentrations of Cu2+ injected with CySBH increased from 5 to 15 μM, the fluorescence intensity of the mice decreased gradually and was quenched after injection with 15 μM Cu2+ (Fig. 5c–e). This could be due to the effective complexation of Cu2+ ions and CySBH in vivo, which quenches fluorescence. These results suggest that the probe CySBH was practically effective and promising for monitoring and tracking Cu2+ ions in vivo.
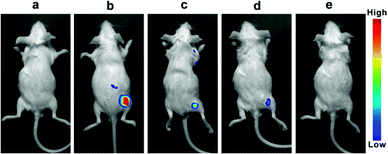 |
| Fig. 5 In vivo fluorescence imaging of Cu2+ in KM mice. (a) Control; (b) mice given an injection of CySBH (10 μM); (c) mice given an injection of CySBH (10 μM) with Cu2+ (5 μM); (d) mice given an injection of CySBH (10 μM) with Cu2+ (10 μM); (e) mouse given an injection of CySBH (10 μM) with Cu2+ (15 μM). (n = 3). | |
4. Conclusions
In summary, we have developed a novel NIR probe for Cu2+ detection in vitro and in vivo by incorporating the benzoyl hydrazone unit into a hemicyanine dye. The coordination of the CySBH salicylaldehyde benzoyl hydrazone group with Cu2+ induces the deprotonation of the phenol group, resulting in the distinct quenching of the fluorescence intensity. By taking advantage of the specific coordination, CySBH exhibits high sensitivity and selectivity toward Cu2+. The detection limit of CySBH for Cu2+ was as low as 28.4 nM. Moreover, since the probe CySBH has a long excitation wavelength (NIR) and favorable biocompatibility, the probe has been successfully applied in the bioimaging and detection of Cu2+ in diverse living cells and mice. We believe that this probe holds promising utility for bioimaging and diagnosing Cu2+-associated diseases.
Conflicts of interest
There are no conflicts to declare.
Acknowledgements
This work was financially supported by the Health Industry Scientific Research Project of Gansu Province (No. GSWSKY2020-70), the scientific research start-up funds for openly recruited doctors of Gansu Agricultural University (No. GAU-KYQD-2019-23), National Natural Science Foundation of China (No. 21804135), and Natural Science Foundation of Gansu Province (20JR5RA133).
References
- A. V. Davis and T. V. O'Halloran, A place for thioether chemistry in cellular copper ion recognition and trafficking, Nat. Chem. Biol., 2008, 4, 148–151 CrossRef CAS PubMed.
- T. Hirayama, G. C. Van de Bittner, W. G. Lawrence, S. Lutsenko and C. G. Chang, Near-infrared fluorescent sensor for in vivo copper imaging in a murine Wilson disease model, Proc. Natl. Acad. Sci. U. S. A., 2012, 109, 2228–2233 CrossRef CAS PubMed.
- J. Liu, Z. Liu, W. Wang and Y. Tian, Real-time tracking and sensing of Cu+ and Cu2+ with a single SERS probe in the live brain: toward understanding why copper ions were increased upon ischemia, Angew. Chem., Int. Ed., 2021, 60, 21351–21359 CrossRef CAS PubMed.
- E. Madsen and J. D. Gitlin, Copper and iron disorders of the brain, Annu. Rev. Neurosci., 2007, 30, 317–337 CrossRef CAS PubMed.
- Z. Aydin, B. Yan, Y. Wei and M. Guo, A novel near-infrared turn-on and ratiometric fluorescent probe capable of copper(II) ion determination in living cells, Chem. Commun., 2020, 56, 6043–6046 RSC.
- T. Shu, Z. Yang, Z. Cen, X. Deng, Y. Deng, C. Dong and Y. Yu, A novel ratiometric fluorescent probe based on a BODIPY derivative for Cu2+ detection in aqueous solution, Anal. Methods, 2018, 10, 5755–5762 RSC.
- N. Solovyev, M. Vinceti, P. Grill, J. Mandrioli and B. Michalke, Redox speciation of iron, manganese, and copper in cerebrospinal fluid by strong cation exchange chromatography-sector field inductively coupled plasma mass spectrometry, Anal. Chim. Acta, 2017, 973, 25–33 CrossRef CAS PubMed.
- J. Hu, R. E. Sturgeon, K. Nadeau, X. Hou, C. Zheng and L. Yang, Copper ion assisted photochemical vapor generation of chlorine for its sensitive determination by sector field inductively coupled plasma mass spectrometry, Anal. Chem., 2018, 90, 4112–4118 CrossRef CAS PubMed.
- A. Ramdass, V. Sathish, E. Babu, M. Velayudham, P. Thanasekaran and S. Rajagopai, Recent developments on optical and electrochemical sensing of copper(II) ion based on transition metal complexes, Coord. Chem. Rev., 2017, 343, 278–307 CrossRef CAS.
- H. Wang, Q. Lu, M. Li, H. Li, Y. Liu, H. Li, Y. Zhang and S. Yao, Electrochemically prepared oxygen and sulfur co-doped graphitic carbon nitride quantum dots for fluorescence determination of copper and silver ions and biothiols, Anal. Chim. Acta, 2018, 1027, 121–129 CrossRef CAS PubMed.
- J. C. Jin, J. Wu, G. P. Yang and Y. Y. Wang, A microporous anionic metal-organic framework for a highly selective and sensitive electrochemical sensor of Cu2+ ions, Chem. Commun., 2016, 52, 8475–8478 RSC.
- G. J. Park, G. R. You, Y. W. Choi and C. Kim, A naked-eye chemosensor for simultaneous detection of iron and copper ions and its copper complex for colorimetric/fluorescent sensing of cyanide, Sens. Actuators, B, 2016, 229, 257–271 CrossRef CAS.
- B. Li, G. Lai, H. Zhang, S. Hu and A. Yu, Copper chromogenic reaction based colorimetric immunoassay for rapid and sensitive detection of a tumor biomarker, Anal. Chim. Acta, 2017, 963, 106–111 CrossRef CAS PubMed.
- D. Udhayakumari, S. Naha and S. Velmathi, Colorimetric and fluorescent chemosensors for Cu2+. A comprehensive review from the years 2013–15, Anal. Methods, 2017, 9, 552–578 RSC.
- Y. P. Dai, P. Wang, J. X. Fu, K. Yao, K. X. Xu and X. B. Pang, A quinoline-based Cu2+ ion complex fluorescence probe for selective detection of inorganic phosphate anion in aqueous solution and its application to living cells, Spectrochim. Acta, Part A, 2017, 183, 30–36 CrossRef CAS PubMed.
- J. Li, D. Yim, W. D. Jang and J. Yoon, Recent progress in the design and applications of fluorescence probes containing crown ethers, Chem. Soc. Rev., 2017, 46, 2437–2458 RSC.
- Y. S. Hu, J. Kang, P. P. Zhou, X. Han, J. Y. Sun, S. D. Liu, L. W. Zhang and J. G. Fang, A elective colorimetric and red-emitting fluorometric probe for sequential detection of Cu2+ and H2S, Sens. Actuators, B, 2018, 255, 3155–3162 CrossRef CAS.
- R. Kaushik, R. Sakla, N. Kumar, A. Ghosh, V. D. Ghule and D. A. Jose, Multianalytes sensing probe: fluorescent moisture detection, smartphone assisted colorimetric phosgene recognition and colorimetric discrimination of Cu2+and Fe3+ ions, Sens. Actuators, B, 2021, 328, 129026 CrossRef CAS.
- Y. Huang, C. F. Li, W. J. Shi, H. Y. Tan, Z. Z. He, L. Zheng, F. Liu and J. W. Yan, A near-infrared BODIPY-based fluorescent probe for ratiometric and discriminative detection of Hg2+ and Cu2+ ions in living cells, Talanta, 2019, 198, 390–397 CrossRef CAS PubMed.
- Z. Li, Y. Xu, H. Xu, M. Cui, T. Liu, X. Ren, J. Sun, D. Deng, Y. Gu and P. Wang, A dicyanomethylene-4H-pyran-based fluorescence probe with high selectivity and sensitivity for detecting copper(II) and its bioimaging in living cells and tissue, Spectrochim. Acta, Part A, 2021, 244, 118819 CrossRef CAS PubMed.
- Y. Li, Y. Zhao, W. Chan, Y. Wang, Q. You, C. Liu, J. Zheng, J. Li, S. Yang and R. Yang, Selective tracking of lysosomal Cu2+ ions using simultaneous target- and location-activated fluorescent nanoprobes, Anal. Chem., 2015, 87, 584–591 CrossRef CAS PubMed.
- L. Yuan, W. Lin, S. Zhao, W. Gao, B. Chen, L. He and S. Zhu, A unique approach to development of near-infrared fluorescent sensors for in vivo imaging, J. Am. Chem. Soc., 2012, 134, 13510–13523 CrossRef CAS PubMed.
- X. Zhen, J. Zhang, J. Huang, C. Xie, Q. Miao and K. Pu, Macrotheranostic probe with disease-activated near-infrared fluorescence, photoacoustic, and photothermal signals for imaging-guided therapy, Angew. Chem., Int. Ed., 2018, 57, 7804–7808 CrossRef CAS PubMed.
- P. Llano-Suárez, D. Bouzas-Ramos, J. M. Costa-Fernández, A. Soldado and M. T. Fernández-Argüelles, Near-infrared fluorescent nanoprobes for highly sensitive cyanide quantification in natural waters, Talanta, 2019, 192, 463–470 CrossRef PubMed.
- Y. Tian, Y. Li, W. L. Jiang, D. Y. Zhou, J. Fei and C. Y. Li, In-situ imaging of azoreductase activity in the acute and chronic ulcerative colitis mice by a near-infrared fluorescent probe, Anal. Chem., 2019, 91, 10901–10907 CrossRef CAS PubMed.
- Y. Tian, Y. Li, W. X. Wang, W. L. Jiang, J. Fei and C. Y. Li, Novel strategy for validating the existence and mechanism of the “gut–liver axis” in vivo by a hypoxia-sensitive NIR fluorescent probe, Anal. Chem., 2020, 92(6), 4244–4250 CrossRef CAS PubMed.
- K. Huang, D. Han, X. Li, M. Peng, X. Zeng, L. Jiang and D. Qin, A new Cu2+-selective fluorescent probe with six-membered spirocyclic hydrazide and its application in cell imaging, Dyes Pigm., 2019, 171, 107701 CrossRef CAS.
- Y. Liu, Q. Su, M. Chen, Y. Dong, Y. Shi, W. Feng, Z. Y. Wu and F. Li, Near-infrared upconversion chemodosimeter for in vivo detection of Cu2+ in Wilson disease, Adv. Mater., 2016, 28, 6625–6630 CrossRef CAS PubMed.
- F. Chen, F. Xiao, W. Zhang, C. Lin and Y. Wu, Highly Stable and NIR luminescent Ru-LPMSN hybrid materials for sensitive detection of Cu2+ in vivo, ACS Appl. Mater. Interfaces, 2018, 10, 26964–26971 CrossRef CAS PubMed.
- X. Tang, J. Han, Y. Wang, L. Ni, L. Li, L. Wang and W. Zhang, A fluorescent chemosensor for Cu2+ ions and its application in cell imaging, Tetrahedron, 2017, 73, 1367–1373 CrossRef CAS.
- B. Valeur and I. Leray, Design principles of fluorescent molecular sensors for cation recognition, Coord. Chem. Rev., 2000, 205, 3–40 CrossRef CAS.
- D. Wu, L. Chen, W. Lee, G. Ko, J. Yin and J. Yoon, Recent progress in the development of organic dye based near-infrared fluorescence probes for metal ions, Coord. Chem. Rev., 2018, 354, 74–97 CrossRef CAS.
- Y. Li, M. Sun, K. Zhang, Y. Zhang, Y. Yan, K. Lei, L. Wu, H. Yu and S. Wang, A near-infrared fluorescent probe for Cu2+ in living cells based on coordination effect, Sens. Actuators, B, 2017, 243, 36–42 CrossRef CAS.
- H. Zhang, L. Feng, Y. Jiang, Y. T. Wang, Y. He, G. Zheng, J. He, Y. Tian, H. Sun and D. Ho, A reaction-based near-infrared fluorescent sensor for Cu2+ detection in aqueous buffer and its application in living cells and tissues imaging, Biosens. Bioelectron., 2017, 94, 24–29 CrossRef CAS PubMed.
- P. Li, X. Duan, Z. Z. Chen, Y. Liu, T. Xie, L. B. Fang, X. R. Li, M. Yin and B. Tang, A near-infrared fluorescent probe for detecting copper(II) with high selectivity and sensitivity and its biological imaging applications, Chem. Commun., 2011, 47, 7755–7757 RSC.
- K. C. Ko, J. S. Wu, H. J. Kim, P. S. Kwon, J. W. Kim, R. A. Bartsch, J. Y. Lee and J. S. Kim, Rationally designed fluorescence ‘turn-on’ sensor for Cu2+, Chem. Commun., 2011, 11, 3165–3167 RSC.
- R. L. Sheng, P. F. Wang, Y. H. Gao, Y. Wu, W. M. Liu, J. J. Ma, H. P. Li and S. K. Wu, Colorimetric test kit for Cu2+ detection, Org. Lett., 2008, 21, 5015–5018 CrossRef PubMed.
- Y. H. Zhao, Y. Lio, H. Wang, H. Wei, T. Cuo, H. Tian, L. Yuan and X. B. Zhang, A novel ratiometric and reversible fluorescence probe with a large Stokes shift for Cu2+ based on a new clamp-on unit, Anal. Chim. Acta, 2019, 1065, 134–141 CrossRef CAS PubMed.
- Y. H. Zhao, Y. Li, Y. Long, Z. Zhou, Z. Tang, K. Deng and S. Zhang, Highly selective fluorescence turn-on determination of fluoride ions via chromogenic aggregation of a silyloxy-functionalized salicylaldehyde azine, Tetrahedron Lett., 2017, 58, 1351–1355 CrossRef CAS.
- B. Tharmalingam, M. Mathivanan, G. Dhamodiran, K. S. Mani, M. Paranjothy and M. Murugesapandian, Star-shaped ESIPT-active mechanoresponsive luminescent AIEgen and its on-off-on emissive response to Cu2+/S2−, ACS Omega, 2019, 4, 12459–12469 CrossRef CAS PubMed.
- Y. Xu, L. Yang, H. Wang, Y. Zhang, X. Yang, M. Pei and G. Zhang, A new “off-on-off” sensor for sequential detection of Al3+ and Cu2+ with excellent sensitivity and selectivity based on different sensing mechanisms, J. Photochem. Photobiol., A, 2020, 391, 112372 CrossRef CAS.
- W. Nasomphan, P. Tangboriboonrat and S. Smanmoo, Dansyl based “Turn-On” fluorescent sensor for Cu2+ ion detection and the application to living cell imaging, J. Fluoresc., 2017, 27, 2201–2212 CrossRef CAS PubMed.
- X. Pan, J. Jiang, J. Li, W. Wu and J. Zhang, Theoretical design of near-infrared Al3+ fluorescent probes based on salicylaldehyde acylhydrazone Schiff base derivatives, Inorg. Chem., 2019, 58, 12618–12627 CrossRef CAS PubMed.
- W. Shan, F. Liu, J. Liu, Y. Chen, Z. Yang and D. Guo, Synthesis and luminescence properties of salicylaldehyde isonicotinoyl hydrazone derivatives and their europium complexes, J. Inorg. Biochem., 2015, 150, 100–107 CrossRef CAS PubMed.
- S. Kumar and M. Nath, New diorganotin(IV) complexes of salicylaldehyde based hydrazones bearing furan heterocycle moiety: X-ray structural investigation of dimethyltin(IV) and diphenyltin(IV) complexes, J. Organomet. Chem., 2018, 856, 87–99 CrossRef CAS.
- S. Sharma and K. S. Ghosh, Recent advances (2017–20) in the detection of copper ion by using fluorescence sensors working through transfer of photo-induced electron (PET), excited-state intramolecular proton (ESIPT) and Förster resonance energy (FRET), Spectrochim. Acta, Part A, 2021, 254, 119610 CrossRef CAS PubMed.
- H. Chen, P. Yang, Y. Li, L. Zhang, F. Ding, X. He and J. Shen, Insight into triphenylamine and coumarin serving as copper(II) sensors with “OFF” strategy and for bio-imaging in living cells, Spectrochim. Acta, Part A, 2020, 224, 117384 CrossRef CAS PubMed.
Footnotes |
† Electronic supplementary information (ESI) available: Table S1, Fig. S1–S4, NMR and HRMS spectra of synthetic compounds. See DOI: 10.1039/d1ra08616b |
‡ These authors contributed equally to this work and are both considered to the first author. |
|
This journal is © The Royal Society of Chemistry 2022 |
Click here to see how this site uses Cookies. View our privacy policy here.