DOI:
10.1039/D1RA09036D
(Paper)
RSC Adv., 2022,
12, 8443-8448
Palladium-catalyzed regioselective hydrosulfonylation of allenes with sulfinic acids†
Received
14th December 2021
, Accepted 9th March 2022
First published on 18th March 2022
Abstract
An atom-economic method of preparing allylic sulfones via hydrosulfonylation of allenes with sulfinic acids under Pd(0)-catalysis was reported. This process has a high degree of regio- and stereoselectivity, and provides the target product with a moderate to excellent yield. A wide range of nitrogen- or oxygen-containing linear E-allylic sulfones have been synthesized. With the support of experimental research, a possible mechanism was proposed.
Introduction
Since the allyl moiety has the potential for further functionalization and construction of new stereogenic centers through asymmetric synthesis, there is an urgent need to develop various atom-economic methods to convert simple and easily available raw materials into allylic derivatives.1 Allylic sulfones, as an important structural motif of allyl derivatives, are widely used in organic synthesis and the development of pharmaceutical active molecules.2 Understandably, many efforts have been made toward synthetic methodologies for allylic sulfones. Transition metal-catalyzed allylic substitution reactions represent one of the most fundamental and versatile methods widely used in modern organic chemistry to construct allylic sulfones.3 However, most of these methods require the preinstallation of a leaving group or the use of a stoichiometric amount of oxidant4 or base.5 Thus, how to realize the efficient construction of allylic sulfone compounds without the installation of leaving groups under mild conditions has become a challenging problem. In the past decades, allenes have progressively risen from an unenviable status of being a structural curiosity to becoming powerful and versatile synthetic building blocks in organic synthesis.6 For example, Yamamoto et al. reported an atom-economic example of palladium-catalyzed hydrosulfination of allenes employing sulfonyl hydrazides, providing the corresponding linear allylic sulfones (Scheme 1a).7 Subsequently, Breit's research group reported on the strategy of synthesizing branched allylic sulfone with allenes under the catalysis of Rh (Scheme 1a).8 However, in the previous studies, allylic sulfones was mainly produced in one step through addition of sulfonyl hydrazides to allenes in high temperature and have the by-products of hydrogen and nitrogen generation. Comparing to these strategies, Monnier and co-workers9 established a transition-metal-free approach to prepare allylic sulfones through the addition of aryl or alkyl sodium sulfinates to allenamides (Scheme 1b). Unfortunately, when arylsulfinic acid was used instead of the corresponding sodium salt, no product was formed.
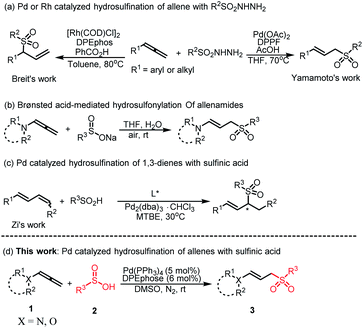 |
| Scheme 1 Profiles of hydrosulfination of allenes or allenamides. | |
As one of the electron-rich allenes, allenamide has recently become a versatile starting material and application in a variety of interesting transformations through sequential reactions, providing a new and effective method for the preparation of synthetic or biologically important nitrogen-containing compounds.10 In particular, the intermolecular addition reaction of allenes and nucleophiles catalyzed by transition metals has been widely used in recent years and become one of the effective methods for constructing multifunctional nitrogen-containing allylic derivatives.11 Despite these significant advances, the transition metal catalyzed assemble allylic sulfones from readily attained sulfinic acids are still highly demanded. Recently, Zi et al. reported the hydrosulfonylation of 1,3-dienes with sulfinic acids under the catalysis of palladium, which provides an economical way to obtain 1,3-disubstituted chiral allylic sulfones (Scheme 1c).12 Enlightened by these excellent works, we envisage that a simple palladium-based catalytic system for hydrosulfonylation of allenamides and sulfinic acids can be established. Herein, we describe the Pd-catalyzed nucleophilic addition of sulfinic acids to allenes (Scheme 1d). A wide range of nitrogen- or oxygen-containing linear E-allylic sulfones can be selectively obtained through the π-allylic palladium process assisted by the ligand.
Results and discussion
Our initial investigation commenced with the reaction of N-methyl-N-(propa-1,2-dien-1-yl)benzamide (1a) and p-methylbenzenesulfinic acid (2a) in a 1.0
:
1.5 molar ratio by employing Pd(OAc)2 (5 mol%) as the catalyst and L1 (DPEphos, 6 mol%) as the ligand. The reaction was performed in anhydrous dimethyl sulfoxide (DMSO) at room temperature under N2 conditions, delivering the expected hydrosulfonylation product 3a in 60% yield (Table 1, entry 1). Subsequently, an alternative Pd-catalyst including Pd2(dba)3, Pd(PPh3)2Cl2, Pd(PPh3)4, Pd(dba)2 and Pd/C was examined respectively (entries 2–6). Among them, it was found that Pd(PPh3)4 showed the best performance in promoting the conversion of 1a into 3a. Other metal catalysts, such as Cu(OTf)2 and Ni(PPh3)2Cl2 showed no catalytic activities (entries 7 and 8). The replacement of L1 to frequently used mono-, bi- and tri-dentate ligands (L2–L5), which are often used in palladium catalysis, by combining with Pd(PPh3)4 did not further increase the reaction yield (entry 4 vs. entries 9–12). Then, we investigated the solvent effect by using different solvents such as THF, DMF, 1,4-dioxane, and toluene, but all these attempted solvents did not show any improvement in reaction yield (entry 4 vs. entries 13–16). Using 1.2 or 1.3 eq. p-methylbenzenesulfinic acid had no effect on the yield, but when it was reduced to 1.1 eq., the yield decreases (entry 17 vs. entries 18 and 19). Probably suitable acidic conditions can promote the reaction. No addition of Pd(PPh3)4 or L1 results in trace formation of the desired product 3a (entries 20 and 21).
Table 1 Screening of reaction conditionsa
With the optimized conditions established, we first explored the scope and limitations of the method for the hydrosulfonylation of various readily available allenamides 1a–1z with p-methylbenzenesulfinic acid 2a (Scheme 2). Different substituents on the aryl ring of benzoyl were well tolerated, including F, Cl, Br, CF3, Me, MeO, t-Bu, and afforded a set of nitrogen-containing linear allyl aryl sulfones 3a–3l in 82–96% yields. Among them, the electron has little effect on the reaction. Both electron-rich and electron-poor substituents were all successfully engaged in the reaction with a high efficiency. Of these groups, the o-chlorophenyl (1i) and o-fluorophenyl (1j) counterparts with strong steric congestion exhibited a good tolerance, but partial Z-configuration products were formed (3i and 3j). We conjectured that this may be due to the steric hindrance between the vicinal substituents of the aryl ring and the ligands. Thus, other positions of the substituents on the aroyl had no effect on the stereoselectivity and complete E-selectivity was observed. Notably, 2-thienyl substituted counterpart 1m also showed high reactivity, furnishing 95% yield and E-selectivity for product 3m. Various substituents on the nitrogen atom, such as Ph, Bn, Et, n-pentyl and t-Bu groups, were well-tolerated, and the desired products 3n–3q were afforded in 81–92% yields with E-selectivity. The absolute configuration of the compound was confirmed by examination of the X-ray crystal of 3a. In view of the influence of the substituents on the aromatic ring of the benzoyl group on the stereoselectivity of the product, we then investigated the electronic effects of the substituents on the N-aromatic ring. All the substituents can participate in the reaction well, and the ortho-substituents did not exhibit an effect on the stereoselectivity of the reaction, and all the products were obtained in the E configuration. Additionally, allenamides bearing cyclic carbamates 1z also showed high reactivity, furnishing 61% yield for product 3z with E-selectivity. Replacing the aroyl group with p-toluenesulfonyl also provides the desired product smoothly (3aa). In addition, O-substituted allene also performed well under this catalytic protocol (3ab). When replaced with electron withdrawing substituents, the desired product 3ac was not produced.
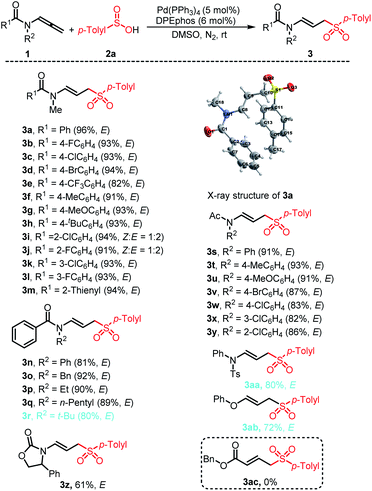 |
| Scheme 2 Substrate scope for the synthesis of products 3. aReaction conditions: 1a (0.20 mmol), 2 (0.24 mmol), Pd(PPh3)4 (5 mol%) and DPEphos (6 mol%) in dry DMSO (2.0 mL) at room temperature for 6 h. bAll yields refer to the isolated yields. cThe stereoselectivity was determined by 1H NMR. | |
A series of arylsulfinic acid 2 reacted with N-methyl-N-(propa-1,2-dien-1-yl)benzamide 1a to give the corresponding (E)-linear sulfone-containing allyl amides (Scheme 3, 4a–4h). The arylsulfinic acid containing large hindered substituents on the aromatic ring could also participate in the reaction smoothly, and didn't exhibit inhibition of stereoselectivity (4g and 4h). Under reaction conditions B, the alkyl and benzyl substituted allyl sulfone products can also be formed well with excellent yield and stereoselectivity (4i and 4j).
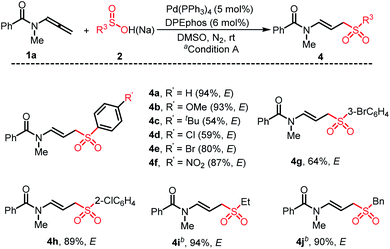 |
| Scheme 3 Substrate scope for the synthesis of products 4. aReaction condition A: 1a (0.20 mmol), 2 (arylsulfinic acid, 0.24 mmol), Pd(PPh3)4 (5 mol%) and DPEphos (6 mol%) in dry DMSO (2.0 mL) at room temperature for 6 h. bReaction condition B: 1a (0.20 mmol), 2 (sodium alkyl sulfinate, 0.24 mmol), PhCOOH (0.24 mmol), Pd(PPh3)4 (5 mol%) and DPEphos (6 mol%) in dry DMSO (2.0 mL) at room temperature for 6 h. cAll yields refer to the isolated yields. dThe stereoselectivity was determined by 1H NMR. | |
To gain mechanistic insight into the hydrosulfination of allenes, deuterium-labeling experiments were conducted. When the reaction of 1m was detected in the presence of 2 eq. D2O, d-3m was not observed, which confirmed that water is not a source of hydrogen (Scheme 4a). The same result was obtained using d6-DMSO as the solvent (Scheme 4b). These observations indicate that the hydrogen on allylic sulfones may come from the only other hydrogen source, sulfinic acids. In addition, the reaction cannot proceed without the catalyst under standard conditions, indicating that the catalyst and ligand was indispensable in the reaction process (Scheme 4c). In order to confirm the role of the carbonyl-directing group and N-substituent, the preformed p-toluenesulfonyl substituted allenamide 1aa or O-substituent allene was reacted with 2a under standard conditions respectively (Schemes 4d and e). These two transformations also worked well, indicating that the carbonyl group or N-substituent is not indispensable. To expand the potential application of this method, an amplification reaction was conducted under the standard conditions. We were delighted to find that product 3a was isolated in 80% yield on a 2.0 mmol scale (Scheme 5).
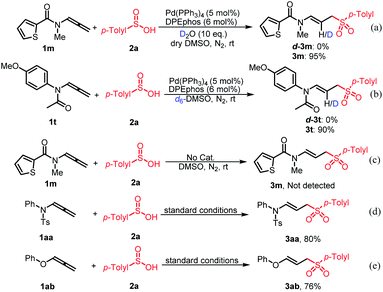 |
| Scheme 4 Control experiments. | |
 |
| Scheme 5 An amplification reaction of 1a with 2a. | |
Based on the above results and related literature precedents,12–14 a reasonable reaction mechanism of the palladium-catalyzed regioselective hydrosulfonylation is proposed in Scheme 6. We suggest that Pd(0) is firstly coordinated with DPEphos, arylsulfinic acid 2 and allene 1 to form Intermediate I. Then Intermediate I convert to π palladium-allyl species II by ligand-to ligand hydrogen transfer (LLHT)12,13 from sulfinic acid to allene. Intermediate II is then transformed to more thermally stable intermediate (E)-III. Subsequent reductive elimination from intermediate (E)-III affords E-3a selectively and regenerates Pd(0).
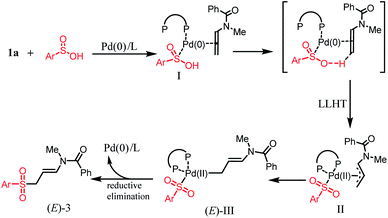 |
| Scheme 6 Proposed mechanisms for forming products 3a. | |
Conclusions
To conclude, we reported an unprecedented palladium-catalyzed hydrosulfonylation of allene by directly adding sulfinic acid to allenyl derivatives. The derivative selectively provides a series of N- or O-substituent linear E-allylic sulfones with medium to excellent yield in 100% atom utilization. With the support of experimental research, we also proposed a reaction mechanism that the hydrogen atom might be transferred directly from the sulfinic acid to allene via a ligand-to ligand hydrogen transfer process. We are currently focusing on further expanding our understanding of this reaction mechanism and developing more LLHT-assisted functionalization of allenes.
Conflicts of interest
There are no conflicts to declare.
Acknowledgements
We are grateful for financial support from the NSFC (No. 21801130) and the NSF of Jiangsu Province (BK20180689).
Notes and references
-
(a) N. A. Butt and W. Zhang, Transition metal-catalyzed allylic substitution reactions with unactivated allylic substrates, Chem. Soc. Rev., 2015, 44, 7929–7967 RSC
;
(b) Q. Cheng, H. F. Tu, C. Zheng, J. P. Qu, G. Helmchen and S. L. You, Iridium-Catalyzed Asymmetric Allylic Substitution Reactions, Chem. Rev., 2019, 119, 1855–1969 CrossRef CAS PubMed
;
(c) J. Feng, M. Holmes and M. J. Krische, Acyclic Quaternary Carbon Stereocenters via Enantioselective Transition Metal Catalysis, Chem. Rev., 2017, 117, 12564–12580 CrossRef CAS PubMed
;
(d) D. Kumar, S. R. Vemula, N. Balasubramanian and G. R. Cook, Indium-Mediated Stereoselective Allylation, Acc. Chem. Res., 2016, 49, 2169–2178 CrossRef CAS PubMed
;
(e) J. Qu and G. Helmchen, Applications of Iridium-Catalyzed Asymmetric Allylic Substitution Reactions in Target-Oriented Synthesis, Acc. Chem. Res., 2017, 50, 2539–2555 CrossRef CAS PubMed
;
(f) R. Jiang, L. Ding, C. Zheng and S.-L. You, Iridium-catalyzed Z-retentive asymmetric allylic substitution reactions, Science, 2021, 371, 380–386 CrossRef CAS PubMed
;
(g) C. Zheng and S. L. You, Exploring the Chemistry of Spiroindolenines by Mechanistically-Driven Reaction Development: Asymmetric Pictet-Spengler-type Reactions and Beyond, Acc. Chem. Res., 2020, 53, 974–987 CrossRef CAS PubMed
;
(h) C. X. Zhuo, C. Zheng and S. L. You, Transition-metal-catalyzed asymmetric allylic dearomatization reactions, Acc. Chem. Res., 2014, 47, 2558–2573 CrossRef CAS PubMed
. -
(a) D. Rotulo-Sims and J. Prunet, Tandem Conjugate Addition-Elimination for the Diastereoselective Synthesis of 4E-Alkenyl syn-1,3-Diols, Org. Lett., 2007, 9, 4147–4150 CrossRef CAS PubMed
;
(b) P. Chen, Y. Wu, S. Zhu, H. Jiang and Z. Ma, Ir-Catalyzed reactions in natural product synthesis, Org. Chem. Front., 2018, 5, 132–150 RSC
;
(c) D. Díez, P. Garcia, I. S. Marcos, N. M. Garrido, P. Basabe, H. B. Broughton and J. G. Urones, Stereocontrolled Synthesis of Cyclopropanol Amino Acids from Allylic Sulfones: Conformationally Restricted Building Blocks, Org. Lett., 2003, 5, 3687–3690 CrossRef PubMed
;
(d) V. Gembus, S. Postikova, V. Levacher and J. F. Briere, Highly regio- and diastereoselective anionic [3 + 2] cycloaddition under phase transfer catalytic conditions, J. Org. Chem., 2011, 76, 4194–4199 CrossRef CAS PubMed
;
(e) Z. S. Liu, W. K. Li, T. R. Kang, L. He and Q. Z. Liu, Palladium-catalyzed asymmetric cycloadditions of vinylcyclopropanes and in situ formed unsaturated imines: construction of structurally and optically enriched spiroindolenines, Org. Lett., 2015, 17, 150–153 CrossRef CAS PubMed
;
(f) P. Vogel, M. Turks, L. Bouchez, D. Markovic, A. Varela-Álvarez and J. Á. Sordo, New Organic Chemistry of Sulfur Dioxide, Acc. Chem. Res., 2007, 40, 931–942 CrossRef CAS PubMed
;
(g) B. M. Trost and C. A. Kalnmals, Annulative Allylic Alkylation Reactions between Dual Electrophiles and Dual Nucleophiles: Applications in Complex Molecule Synthesis, Chem.–Eur. J., 2020, 26, 1906–1921 CrossRef CAS PubMed
;
(h) G. C. Tsui and M. Lautens, Linear-selective rhodium(I)-catalyzed addition of arylboronic acids to allyl sulfones, Angew. Chem., Int. Ed., 2010, 49, 8938–8941 CrossRef CAS PubMed
. -
(a) B. M. Trost, M. G. Organ and G. A. O'Doherty, Asymmetric Synthesis of Allylic Sulfones. Useful Asymmetric Building Blocks, J. Am. Chem. Soc., 1995, 117, 9662–9670 CrossRef CAS
;
(b) M. Ueda and J. F. Hartwig, Iridium-Catalyzed, Regio- and Enantioselective Allylic Substitution with Aromatic and Aliphatic Sulfinates, Org. Lett., 2010, 12, 92–94 CrossRef CAS PubMed
;
(c) A. B. Pritzius and B. Breit, Asymmetric rhodium-catalyzed addition of thiols to allenes: synthesis of branched allylic thioethers and sulfones, Angew. Chem., Int. Ed., 2015, 54, 3121–3125 CrossRef CAS PubMed
;
(d) A. B. Pritzius and B. Breit, Z-Selective Hydrothiolation of Racemic 1,3-Disubstituted
Allenes: An Atom-Economic Rhodium-Catalyzed Dynamic Kinetic Resolution, Angew. Chem., Int. Ed., 2015, 54, 15818–15822 CrossRef CAS PubMed
;
(e) S. Zheng, N. Gao, W. Liu, D. Liu, X. Zhao and T. Cohen, Regio- and Enantioselective Iridium-Catalyzed Allylation of Thiophenol: Synthesis of Enantiopure Allyl Phenyl Sulfides, Org. Lett., 2010, 12, 4454–4457 CrossRef CAS PubMed
;
(f) T. T. Wang, F. X. Wang, F. L. Yang and S. K. Tian, Palladium-catalyzed aerobic oxidative coupling of enantioenriched primary allylic amines with sulfonyl hydrazides leading to optically active allylic sulfones, Chem. Commun., 2014, 50, 3802–3805 RSC
;
(g) X. S. Wu, Y. Chen, M. B. Li, M. G. Zhou and S. K. Tian, Direct substitution of primary allylic amines with sulfinate salts, J. Am. Chem. Soc., 2012, 134, 14694–14697 CrossRef CAS PubMed
;
(h) P. X. Zhou, Y. Y. Ye, L. B. Zhao, J. Y. Hou, X. Kang, D. Q. Chen, Q. Tang, J. Y. Zhang, Q. X. Huang, L. Zheng, J. W. Ma, P. F. Xu and Y. M. Liang, Using N-tosylhydrazone as a double nucleophile in the palladium-catalyzed cross-coupling reaction to synthesize allylic sulfones, Chem.–Eur. J., 2014, 20, 16093–16096 CrossRef CAS PubMed
. -
(a) X. Li, X. Xu and Y. Tang, Tetrabutylammonium iodide catalyzed allylic sulfonylation of Baylis-Hillman acetates with sulfonylhydrazides in water, Org. Biomol. Chem., 2013, 11, 1739–1742 RSC
;
(b) Y. Wang, G. Xiong, C. Zhang and Y. Chen, Controllable Activation of beta-Alkyl Nitroalkenes: Regioselective Synthesis of Allyl and Vinyl Sulfones, J. Org. Chem., 2021, 86, 4018–4026 CrossRef CAS PubMed
. -
(a) H. J. Gais, T. Jagusch, N. Spalthoff, F. Gerhards, M. Frank and G. Raabe, Highly selective palladium catalyzed kinetic resolution and enantioselective substitution of racemic allylic carbonates with sulfur nucleophiles: asymmetric synthesis of allylic sulfides, allylic sulfones, and allylic alcohols, Chem.–Eur. J., 2003, 9, 4202–4221 CrossRef CAS PubMed
;
(b) W. B. Liu, S. C. Zheng, H. He, X. M. Zhao, L. X. Dai and S. L. You, Iridium-catalyzed regio- and enantioselective allylic alkylation of fluorobis(phenylsulfonyl)methane, Chem. Commun., 2009, 43, 6604–6606 RSC
;
(c) K. Spielmann, M. Xiang, L. A. Schwartz and M. J. Krische, Direct Conversion of Primary Alcohols to 1,2-Amino Alcohols: Enantioselective Iridium-Catalyzed Carbonyl Reductive Coupling of Phthalimido-Allene via Hydrogen Auto-Transfer, J. Am. Chem. Soc., 2019, 141, 14136–14141 CrossRef CAS PubMed
;
(d) S. Zheng, W. Huang, N. Gao, R. Cui, M. Zhang and X. Zhao, One pot iridium-catalyzed asymmetrical double allylations of sodium sulfide: a fast and economic way to construct chiral C2-symmetric bis(1-substituted-allyl)sulfane, Chem. Commun., 2011, 47, 6969–6971 RSC
. -
(a) M. Bandini, Gold-catalyzed decorations of arenes and heteroarenes with C–C multiple bonds, Chem. Soc. Rev., 2011, 40, 1358–1367 RSC
;
(b) M. Holmes, L. A. Schwartz and M. J. Krische, Intermolecular Metal-Catalyzed Reductive Coupling of Dienes, Allenes, and Enynes with Carbonyl Compounds and Imines, Chem. Rev., 2018, 118, 6026–6052 CrossRef CAS PubMed
;
(c) X. Huang and S. Ma, Allenation of Terminal Alkynes with Aldehydes and Ketones, Acc. Chem. Res., 2019, 52, 1301–1312 CrossRef CAS PubMed
;
(d) G. Li, X. Huo, X. Jiang and W. Zhang, Asymmetric synthesis of allylic compounds via hydrofunctionalisation and difunctionalisation of dienes, allenes, and alkynes, Chem. Soc. Rev., 2020, 49, 2060–2118 RSC
;
(e) J. L. Mascarenas, I. Varela and F. Lopez, Allenes and Derivatives in Gold(I)- and Platinum(II)-Catalyzed Formal Cycloadditions, Acc. Chem. Res., 2019, 52, 465–479 CrossRef CAS PubMed
;
(f) Z. Wang, X. Xu and O. Kwon, Phosphine catalysis of allenes with electrophiles, Chem. Soc. Rev., 2014, 43, 2927–2940 RSC
;
(g) W. Yang and A. S. Hashmi, Mechanistic insights into the gold chemistry of allenes, Chem. Soc. Rev., 2014, 43, 2941–2955 RSC
;
(h) Y.-M. Wang, A. D. Lackner and F. D. Toste, Development of Catalysts and Ligands for Enantioselective Gold Catalysis, Acc. Chem. Res., 2014, 47, 889–901 CrossRef CAS PubMed
;
(i) J. Ye and S. Ma, Palladium-Catalyzed Cyclization Reactions of Allenes in the Presence of Unsaturated Carbon–Carbon Bonds, Acc. Chem. Res., 2014, 47, 989–1000 CrossRef CAS PubMed
. - S. Kamijo, M. Al-Masum and Y. Yamamoto, Palladium Catalyzed Hydrosulfination of Allenes with Tosyihydrazine Leading to Allylsulfones, Tetrahedron Lett., 1998, 39, 691–694 CrossRef CAS
. - V. Khakyzadeh, Y. H. Wang and B. Breit, Rhodium-catalyzed addition of sulfonyl hydrazides to allenes: regioselective synthesis of branched allylic sulfones, Chem. Commun., 2017, 53, 4966–4968 RSC
. - L. Pages, S. Lemouzy, M. Taillefer and F. Monnier, J. Org. Chem., 2021, 86, 15695–15701 CrossRef CAS PubMed
. -
(a) R. Blieck, M. Taillefer and F. Monnier, Metal-Catalyzed Intermolecular Hydrofunctionalization of Allenes: Easy Access to Allylic Structures via the Selective Formation of C–N, C–C, and C–O Bonds, Chem. Rev., 2020, 120, 13545–13598 CrossRef CAS PubMed
;
(b) X. Li, Y. Liu, N. Ding, X. Tan and Z. Zhao, Recent progress in transition-metal-free functionalization of allenamides, RSC Adv., 2020, 10, 36818–36827 RSC
;
(c) L.-L. Wei, H. Xiong and R. P. Hsung, The Emergence of Allenamides in Organic Synthesis,, Acc. Chem. Res., 2003, 36, 773–782 CrossRef CAS PubMed
;
(d) T. Lu, Z. Lu, Z. X. Ma, Y. Zhang and R. P. Hsung, Allenamides: a powerful and versatile building block in organic synthesis, Chem. Rev., 2013, 113, 4862–4904 CrossRef CAS PubMed
. -
(a) R. Blieck, R. Abed Ali Abdine, M. Taillefer and F. Monnier, Regio- and Stereoselective Copper-Catalyzed Allylation of 1,3-Dicarbonyl Compounds with Terminal Allenes, Org. Lett., 2018, 20, 2232–2235 CrossRef CAS PubMed
;
(b) R. Blieck, M. Taillefer and F. Monnier, Copper-Catalyzed Hydrocarboxylation of N-Allenyl Derivatives, J. Org. Chem., 2019, 84, 11247–11252 CrossRef CAS PubMed
;
(c) E. Skucas, J. R. Zbieg and M. J. Krische, anti-Aminoallylation of Aldehydes via Ruthenium-Catalyzed Transfer Hydrogenative Coupling of Sulfonamido Allenes: 1,2-Aminoalcohols, J. Am. Chem. Soc., 2009, 131, 5054–5055 CrossRef CAS PubMed
;
(d) M. C. Kimber and A. Facile, Mild Synthesis of Enamides using a Gold-Catalyzed Nucleophilic Addition to Allenamides, Org. Lett., 2010, 12, 1128–1131 CrossRef CAS PubMed
;
(e) Y. Liu, A. Cerveri, A. De Nisi, M. Monari, O. Nieto Faza, C. S. Lopez and M. Bandini, Nickel catalyzed regio- and stereoselective arylation and methylation of allenamides via coupling reactions. An experimental and computational study, Org. Chem. Front., 2018, 5, 3231–3239 RSC
;
(f) T. R. Pradhan, H. W. Kim and J. K. Park, Regiodivergent Synthesis of 1,3- and 1,4-Enynes through Kinetically Favored Hydropalladation and Ligand-Enforced Carbopalladation, Angew. Chem., Int. Ed., 2018, 57, 9930–9935 CrossRef CAS PubMed
;
(g) Y. Wang, P. Zhang, D. Qian and J. Zhang, Highly Regio-, Diastereo-, and Enantioselective Gold(I)-Catalyzed Intermolecular Annulations with N-Allenamides at the Proximal C=C Bond, Angew. Chem., Int. Ed., 2015, 54, 14849–14852 CrossRef CAS PubMed
. - Q. Zhang, D. Dong and W. Zi, Palladium-Catalyzed Regio- and Enantioselective Hydrosulfonylation of 1,3-Dienes with Sulfinic Acids: Scope, Mechanism, and Origin of Selectivity, J. Am. Chem. Soc., 2020, 142, 15860–15869 CrossRef CAS PubMed
. - For LLHT in metal catalysis:
(a) J. S. Bair, Y. Schramm, A. G. Sergeev, E. Clot, O. Eisenstein and J. F. Hartwig, J. Am. Chem. Soc., 2014, 136, 13098–13101 CrossRef CAS PubMed
;
(b) L. J. Xiao, X. N. Fu, M. J. Zhou, J. H. Xie, L. X. Wang, X. F. Xu and Q. L. Zhou, J. Am. Chem. Soc., 2016, 138, 2957–2960 CrossRef CAS PubMed
;
(c) J. Guihaumé, S. Halbert, O. Eisenstein and R. N. Perutz, Organometallics, 2011, 31, 1300–1314 CrossRef
;
(d) S. Tang, O. Eisenstein, Y. Nakao and S. Sakaki, Organometallics, 2017, 36, 2761–2771 CrossRef CAS
. -
(a) R. A. A. Abdine, L. Pagès, M. Taillefer and F. Monnier, Hydroarylation of N-Allenyl Derivatives Catalyzed by Copper, Eur. J. Org. Chem., 2020, 48, 7466–7469 CrossRef
;
(b) U. Gellrich, A. Meissner, A. Steffani, M. Kahny, H. J. Drexler, D. Heller, D. A. Plattner and B. Breit, Mechanistic investigations of the rhodium catalyzed propargylic CH activation, J. Am. Chem. Soc., 2014, 136, 1097–1104 CrossRef CAS PubMed
;
(c) Y. Liu, N. Ding, X. Tan, X. Li and Z. Zhao, Iron(ii)-chloride-catalyzed regioselective azidation of allenamides with TMSN3, Chem. Commun., 2020, 56, 7507–7510 RSC
;
(d) L. A. Perego, R. Blieck, A. Groué, F. Monnier, M. Taillefer, I. Ciofini and L. Grimaud, Copper-Catalyzed Hydroamination of Allenes: from Mechanistic Understanding to Methodology Development, ACS Catal., 2017, 7, 4253–4264 CrossRef CAS
;
(e) K. Xu, V. Khakyzadeh, T. Bury and B. Breit, Direct transformation of terminal alkynes to branched allylic sulfones, J. Am. Chem. Soc., 2014, 136, 16124–16127 CrossRef CAS PubMed
.
Footnotes |
† Electronic supplementary information (ESI) available. CCDC 2122809 (3a). For ESI and crystallographic data in CIF or other electronic format see DOI: 10.1039/d1ra09036d |
‡ These authors contributed equally. |
|
This journal is © The Royal Society of Chemistry 2022 |
Click here to see how this site uses Cookies. View our privacy policy here.