DOI:
10.1039/D2RA00197G
(Paper)
RSC Adv., 2022,
12, 7115-7119
DABCO as a practical catalyst for aromatic halogenation with N-halosuccinimides†
Received
11th January 2022
, Accepted 23rd February 2022
First published on 2nd March 2022
Abstract
A simple and practical synthetic approach for synthesis of aromatic halides is developed. Simple Lewis base, DABCO, is used as the catalyst. This arene halogenation process proceedes conveniently and efficiently at ambient conditions, providing the desired products in good to excellent yields and selectivity.
Aromatic halogenation is one of the most fundamental and commonly used organic transformations. The versatile applications of aryl halides in transition-metal-catalysed coupling reactions,1–4 biological properties modulation5–9 and material science10–12 highlight their crucial importance. Extensive efforts have been devoted to develop general and efficient methods for the preparation of aryl halides. Although classical electrophilic halogenation is still a promising protocol,13–15 the utilization of hazardous and toxic molecular halogens makes it superseded by more practical halogenating reagents, such as N-halosuccinimides (NCS, NBS, and NIS) due to their low-cost and convenient handling. Certain solvents were widely studied to promote the aromatic halogenation reactions with NXS (X = Cl, Br, and I),16–18 but substrate scope, time-consuming and environmental effects are severely limited and leaving more room for expanding more efficient methods. Metal catalysed C–H activation strategies have also been developed in last decades19–23 for the selective aromatic halogenation with NXS in which directing groups are usually necessary. In spite of the advantages of in situ formed electrophilic halogen intermediates from cheap halide ions in the oxidation process,24–29 it requires stoichiometric oxidants or elevated temperatures. In addition, to address the problem that N-halosuccinimides, especially NCS, are substantially less reactive, other versatile catalysts have also been employed. While Lewis or Brønsted acids are favorable to activate NXS,21,30–42 the reactions rely on activated substrates or suffer from harsh conditions including high temperatures or super acidic conditions. Alternatively, Lewis bases, with O, N, S or P centre, have also been verified to catalyse the halogenation via interaction with NXS to form the activated halonium complexes.43–53 Recently, Jiao's group45 discovered an efficient late-stage chlorination of complex substrates in the presence of DMSO, providing a practical method for further applications. Miura43 also developed a novel protocol using triptycenyl sulfide to realize electrophilic aromatic halogenation of a variety of unactivated compounds. However, certain Lewis bases show structural complexity or handling inefficiency, which indicates that more simple and convenient Lewis base catalysts are still desirable. Stimulated by the crucial role of Lewis base in organic synthesis54 and previous reports, it is vital to develop an efficient and mild system for aromatic halogenation with a simple and convenient Lewis base catalyst.
The feasibility of our approach was evaluated using m-dimethoxybenzene 1a and NCS in the presence of catalytical 1,8-diazabicyclo[5.4.0]undec-7-ene (DBU) in dichloroform (DCM). To our delight, desired chlorinated product 2a was obtained in 74% yield after 1 hour (Table 1, entry 1). Optimization was then carried out at first involving a set of catalysts and solvents. Triethylenediamine (DABCO) showed the best catalytic behaviour (98% yield, Table 1, entry 2) while Et3N gave similar result (79% yield, Table 1, entry 3) to that of DBU probably due to the naked electron pair of DABCO which can more efficiently activate NCS. The yield fell to 24% when 4-dimethylaminopyridine (DMAP) was employed while only 8% of the product was detected in the absence of any Lewis base catalyst even after stirring for 40 hours (Table 1, entry 4-5). Considering the satisfying behaviour as well as its easy of dealing, DABCO was selected for the further optimization. Polar solvents, such as dimethyl formamide (DMF) and acetonitrile, worked well for this aromatic chlorination (Table 1, entry 6-7), however, the reaction performed best in halogenated solvents, dichloromethane and dichloroethane. While toluene deteriorated the reaction, tetrahydrofuran gave comparable yield (Table 1, entry 9-10). DCM was chosen to explore how the identity of the catalyst loading and ratio of reaction partners influence the reactions outcome. The reaction proceeded well even in the presence of 1% of DABCO and there was no obvious decline observed in the presence of excess catalyst (Table 1, entry 11–13) which showed advantages of this protocol because certain Lewis bases used for the aromatic halogenation suppressed the reaction process if over loaded.44 It should be pointed out that the yield slightly decreased when the reaction was quenched after 30 min and no yield improvement was observed after stirring for longer time (Table 1, entry 14). Ultimately, a slight excess of NCS in combination with 5 mol% of DABCO as the catalyst in DCM afforded the optimal conditions.
Table 1 Optimization of the reaction conditionsa
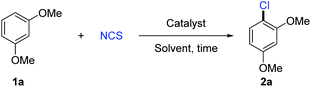
|
Entry |
Catalyst |
Solvent |
t (h) |
Yieldb (%) |
Conditions: 1a (0.5 mmol), NCS (0.55 mmol) was used. The yields were determined by NMR in the presence of certain internal standard, and in the parenthesis was the isolated yield. 1.0 equiv. of NCS was used. 1.2 equiv. of NCS was used. |
1 |
DBU (5 mol%) |
DCM |
1 |
74 |
2 |
DABCO (5 mol%) |
DCM |
1 |
98 (93) |
3 |
Et3N (5 mol%) |
DCM |
1 |
79 |
4 |
DMAP (5 mol%) |
DCM |
1 |
24 |
5 |
— |
DCM |
40 |
8 |
6 |
DABCO (5 mol%) |
DMF |
1 |
80 |
7 |
DABCO (5 mol%) |
CH3CN |
1 |
83 |
8 |
DABCO (5 mol%) |
DCE |
1 |
92 |
9 |
DABCO (5 mol%) |
Toluene |
1 |
45 |
10 |
DABCO (5 mol%) |
THF |
1 |
91 |
11 |
DABCO (1 mol%) |
DCM |
1 |
94 |
12 |
DABCO (10 mol%) |
DCM |
1 |
97 |
13 |
DABCO (50 mol%) |
DCM |
1 |
91 |
14 |
DABCO (5 mol%) |
DCM |
0.5 |
95 |
15c |
DABCO (5 mol%) |
DCM |
1 |
80 |
16d |
DABCO (5 mol%) |
DCM |
1 |
96 |
Upon optimization of the reaction conditions, the scope of (hetero)arenes was evaluated (Table 2). In reactions of anisole derivatives, excellent yields (93–99%) were obtained giving 2a–2d as the sole products although 1,3-dichloro-5,5-dimethylhydantoin (DCDMH), instead of NCS, was used for the construction of 2b and 2c to further improve the yield. Notably, high selectivity was observed in each reaction. The chlorination of naphthylethyl ether afforded the product 2e in almost quantitative yield (99%). It is noted that, aromatic compounds which show negative reactivity with sole NCS, could be converted to the corresponding chlorinated products in the presence of catalytic amount of DABCO.45 Under the same reaction conditions, free aniline 4-bromoaniline could be converted to the desired product 2f in 60% yield. Vanillin was chlorinated to the corresponding product 2g which indicated that phenol and aldehyde groups could also be tolerated well. However, aromatic derivatives bearing electron withdrawing groups, such as nitro group, showed no reactivity due to the electron deficient property. Aromatic carboxylic acid gave a trace amount of the corresponding product, probably resulting from the protonation of DABCO which poisoned its catalytic performance. With regard to heteroaromatic chlorination, we turned our attention to indoles, and it is found that free or protected indole derivatives worked well under the optimal conditions, affording the corresponding products (2j–2m) in good to excellent yields with complete C-3 selectivity. Furthermore, this chlorination protocol performed well on the gram-scale and 2e was obtained in high yield (92%).
Table 2 Aromatic chlorination of (hetero)arenesa
Next, to verify the generality of the aromatic halogenation reaction to construct C–Br and C–I bond, NBS and NIS were subsequently introduced (Table 3). Thus, reactions of arenes with NBS/NIS under the identical conditions were rationally conducted and smoothly proceeded, providing the desired monobromination/monoiodination products in 65–99% yields (2n–2x) with very high regioselectivity. Meanwhile, reaction time was shortened due to the higher reactivity of halogen precursors. Further inspection of the substrate scope also showed that a one-pot sequential halogenation reaction worked well. When 1,3,5-trimethoxybenzene was treated with NBS for 1 h after the addition of NCS in the presence of 5 mol% of DABCO, the bromo-chloro-substituted product (2y, Scheme 1) could be achieved in excellent yield (92%) indicating the long-term efficiency of Lewis base catalyst.
Table 3 Aromatic halogenation of (hetero)arenesa
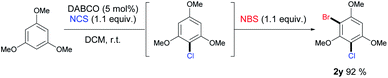 |
| Scheme 1 One-pot two-steps synthesis of multi-halogenated substrate. | |
The mechanism was proposed in Scheme 2. As the lone electron pair of hetero atoms could interact with the positive charged halogen atom of halogenating reagent,43–45,51,55,56 generating the activated halonium intermediate, the formation of DABCO-X+ was assumed as the first step of this catalytic process which was also supported by 1H-NMR and HRMS (see ESI†). The nucleophilicity of aromatic π electron system then promotes the transformation of halogen to realize the aromatic halogenation.
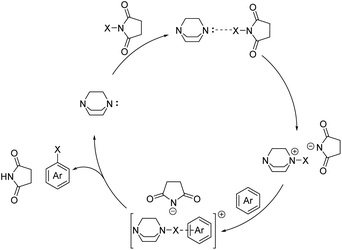 |
| Scheme 2 Proposed mechanism. | |
Conclusions
In summary, we developed an efficient and mild system for aromatic halogenation using DABCO, a simple, cheap and easy of handling Lewis base, as the catalyst. Aromatic halides could be obtained conveniently at ambient conditions even on a gram-scale with up to 99% yields and excellent selectivity by using N-halosuccinimides. The relatively less reactive NCS was well activated to promote the chlorination process. Multi-halogenated substrate could be achieved via one-pot, two-step synthesis with excellent yield which expands the potential of this protocol.
Conflicts of interest
There are no conflicts to declare.
Acknowledgements
We are grateful to the National Natural Science Foundation of China (Grant No. 22005158) and the Program of High-level Talents of Nantong (Grant No. 135420608145 and JC2021070) for financial support.
Notes and references
- J. Hassan, M. Sevignon, C. Gozzi, E. Schulz and M. Lemaire, Chem. Rev., 2002, 102, 1359–1469 CrossRef CAS PubMed.
- F. Bellina and R. Rossi, Chem. Rev., 2010, 110, 1082–1146 CrossRef CAS PubMed.
- R. Chinchilla and C. Najera, Chem. Soc. Rev., 2011, 40, 5084–5121 RSC.
- P. Ruiz-Castillo and S. L. Buchwald, Chem. Rev., 2016, 116, 12564–12649 CrossRef CAS PubMed.
- T. Cernak, K. D. Dykstra, S. Tyagarajan, P. Vachal and S. W. Krska, Chem. Soc. Rev., 2016, 45, 546–576 RSC.
- S. L. Pimlott and A. Sutherland, Chem. Soc. Rev., 2011, 40, 149–162 RSC.
- K. Müller, C. Faeh and F. Diederich, Science, 2007, 317, 1881–1886 CrossRef PubMed.
- S. Purser, P. R. Moore, S. Swallow and V. Gouverneur, Chem. Soc. Rev., 2008, 37, 320–330 RSC.
- G. W. Gribble, Acc. Chem. Res., 1998, 31, 141–152 CrossRef CAS.
- A. M. Hiszpanski, J. D. Saathoff, L. Shaw, H. Wang, L. Kraya, F. Lüttich, M. A. Brady, M. L. Chabinyc, A. Kahn, P. Clancy and Y.-L. Loo, Chem. Mater., 2015, 27, 1892–1900 CrossRef CAS.
- M. L. Tang and Z. Bao, Chem. Mater., 2010, 23, 446–455 CrossRef.
- M. L. Tang, J. H. Oh, A. D. Reichardt and Z. Bao, J. Am. Chem. Soc., 2009, 131, 3733–3740 CrossRef CAS PubMed.
- I. Saikia, A. J. Borah and P. Phukan, Chem. Rev., 2016, 116, 6837–7042 CrossRef CAS PubMed.
- C. Chen, Y. Cao, X. Wu, Y. Cai, J. Liu, L. Xu, K. Ding and L. Yu, Chin. Chem. Lett., 2020, 31, 1078–1082 CrossRef CAS.
- F. Wang, J. Huang, Y. Yang, L. Xu and L. Yu, Ind. Eng. Chem. Res., 2020, 59, 1025–1029 CrossRef CAS.
- E. Zysman-Colman, K. Arias and J. S. Siegel, Can. J. Chem., 2009, 87, 440–447 CrossRef CAS.
- M. C. Carreno, J. L. Garcia Ruano, G. Sanz, M. A. Toledo and A. Urbano, J. Org. Chem., 1995, 60, 5328–5331 CrossRef CAS.
- R. J. Tang, T. Milcent and B. Crousse, J. Org. Chem., 2018, 83, 930–938 CrossRef CAS PubMed.
- J. Zhao, X. Cheng, J. Le, W. Yang, F. Xue, X. Zhang and C. Jiang, Org. Biomol. Chem., 2015, 13, 9000–9004 RSC.
- R. Das and M. Kapur, Asian J. Org. Chem., 2018, 7, 1524–1541 CrossRef CAS.
- F. Mo, J. M. Yan, D. Qiu, F. Li, Y. Zhang and J. Wang, Angew. Chem., Int. Ed., 2010, 49, 2028–2032 CrossRef CAS PubMed.
- S. Mo, Y. Zhu and Z. Shen, Org. Biomol. Chem., 2013, 11, 2756–2760 RSC.
- F. Lied, T. Patra and F. Glorius, Isr. J. Chem., 2017, 57, 945–952 CrossRef CAS.
- R. Semwal, C. Ravi, R. Kumar, R. Meena and S. Adimurthy, J. Org. Chem., 2019, 84, 792–805 CrossRef CAS PubMed.
- J. Li, J. Tang, Y. Wu, Q. He and Y. Yu, RSC Adv., 2018, 8, 5058–5062 RSC.
- U. Bora, G. Bose, M. K. Chaudhuri, S. S. Dhar, R. Gopinath, A. T. Khan and B. K. Patel, Org. Lett., 2000, 2, 247–249 CrossRef CAS PubMed.
- J. Xu, X. Zhu, G. Zhou, B. Ying, P. Ye, L. Su, C. Shen and P. Zhang, Org. Biomol. Chem., 2016, 14, 3016–3021 RSC.
- X.-L. Li, W. Wu, X.-H. Fan and L.-M. Yang, RSC Adv., 2013, 3, 12091–12095 RSC.
- A. Granados, A. Shafir, A. Arrieta, F. P. Cossio and A. Vallribera, J. Org. Chem., 2020, 85, 2142–2150 CrossRef CAS PubMed.
- L. Lu, Y. Li and X. Jiang, Green Chem., 2020, 22, 5989–5994 RSC.
- D. T. Racys, C. E. Warrilow, S. L. Pimlott and A. Sutherland, Org. Lett., 2015, 17, 4782–4785 CrossRef CAS PubMed.
- C.-Y. Zhou, J. Li, S. Peddibhotla and D. Romo, Org. Lett., 2010, 12, 2104–2107 CrossRef CAS PubMed.
- A. J. Close, P. Kemmitt, S. Mark Roe and J. Spencer, Org. Biomol. Chem., 2016, 14, 6751–6756 RSC.
- K. Mori, Y. Ichikawa, M. Kobayashi, Y. Shibata, M. Yamanaka and T. Akiyama, J. Am. Chem. Soc., 2013, 135, 3964–3970 CrossRef CAS PubMed.
- V. K. Chaikovskii, V. D. Filimonov, V. I. Skorokhodov and V. D. Ogorodnikov, Russ. J. Org. Chem., 2007, 43, 1278–1281 CrossRef CAS.
- D. T. Racys, S. A. I. Sharif, S. L. Pimlott and A. Sutherland, J. Org. Chem., 2016, 81, 772–780 CrossRef CAS PubMed.
- M. A. B. Mostafa, R. M. Bowley, D. T. Racys, M. C. Henry and A. Sutherland, J. Org. Chem., 2017, 82, 7529–7537 CrossRef CAS PubMed.
- M. Bergström, G. Suresh, V. R. Naidu and C. R. Unelius, Eur. J. Org. Chem., 2017, 3234–3239 CrossRef.
- X. He, X. Wang, Y. S. Tse, Z. Ke and Y. Y. Yeung, Angew. Chem., Int. Ed., 2018, 57, 12869–12873 CrossRef CAS PubMed.
- Y. Shi, Z. Ke and Y.-Y. Yeung, Green Chem., 2018, 20, 4448–4452 RSC.
- X. Xiong and Y.-Y. Yeung, ACS Catal., 2018, 8, 4033–4043 CrossRef CAS.
- X. He, X. Wang, Y.-L. S. Tse, Z. Ke and Y.-Y. Yeung, ACS Catal., 2021, 11, 12632–12642 CrossRef CAS.
- Y. Nishii, M. Ikeda, Y. Hayashi, S. Kawauchi and M. Miura, J. Am. Chem. Soc., 2020, 142, 1621–1629 CrossRef CAS PubMed.
- R. C. Samanta and H. Yamamoto, Chem.–Eur. J., 2015, 21, 11976–11979 CrossRef CAS PubMed.
- S. Song, X. Li, J. Wei, W. Wang, Y. Zhang, L. Ai, Y. Zhu, X. Shi, X. Zhang and N. Jiao, Nat. Catal., 2019, 3, 107–115 CrossRef.
- S. M. Maddox, C. J. Nalbandian, D. E. Smith and J. L. Gustafson, Org. Lett., 2015, 17, 1042–1045 CrossRef CAS PubMed.
- Y. Hirose, M. Yamazaki, M. Nogata, A. Nakamura and T. Maegawa, J. Org. Chem., 2019, 84, 7405–7410 CrossRef CAS PubMed.
- P. Bovonsombat, P. Sophanpanichkul, A. Pandey, S. Tungsirisurp, P. Limthavornlit, K. Chobtumskul, P. Kuhataparuk, S. Sathityatiwat and P. Teecomegaet, Tetrahedron Lett., 2015, 56, 2193–2196 CrossRef CAS.
- K. Iida, S. Ishida, T. Watanabe and T. Arai, J. Org. Chem., 2019, 84, 7411–7417 CrossRef CAS PubMed.
- P. Bovonsombat, P. Teecomegaet, P. Kulvaranon, A. Pandey, K. Chobtumskul, S. Tungsirisurp, P. Sophanpanichkul, S. Losuwanakul, D. Soimaneewan, P. Kanjanwongpaisan, P. Siricharoensang and S. Choosakoonkriang, Tetrahedron, 2017, 73, 6564–6572 CrossRef CAS.
- X. Xiong, F. Tan and Y. Y. Yeung, Org. Lett., 2017, 19, 4243–4246 CrossRef CAS PubMed.
- W. Zhang, H. Xu, H. Xu and W. Tang, J. Am. Chem. Soc., 2009, 131, 3832–3833 CrossRef CAS PubMed.
- L. S. Pimenta, E. V. Gusevskaya and E. E. Alberto, Adv. Synth. Catal., 2017, 359, 2297–2303 CrossRef CAS.
- S. E. Denmark and G. L. Beutner, Angew. Chem., Int. Ed., 2008, 47, 1560–1638 CrossRef CAS PubMed.
- M. Li, Y. Li, B. Zhao, F. Liang and L.-Y. Jin, RSC Adv., 2014, 4, 30046–30049 RSC.
- S. Guha, I. Kazi, A. Nandy and G. Sekar, Eur. J. Org. Chem., 2017, 5497–5518 CrossRef CAS.
Footnote |
† Electronic supplementary information (ESI) available. See DOI: 10.1039/d2ra00197g |
|
This journal is © The Royal Society of Chemistry 2022 |
Click here to see how this site uses Cookies. View our privacy policy here.