DOI:
10.1039/D2RA00378C
(Review Article)
RSC Adv., 2022,
12, 9139-9153
A comprehensive review on the sources, essentiality and toxicological profile of nickel
Received
18th January 2022
, Accepted 15th March 2022
First published on 23rd March 2022
Abstract
This review contains up-to-date knowledge and recent advancements on the essentiality, sources, and toxicological profile of nickel and its different compounds. Nickel is a recognized essential element for several important biological processes like the healthy growth of plants, animals, and soil/water microbes; though an excess amount of nickel intoxicates flora and fauna. Nickel is found to affect the photosynthetic function of higher plants; it can severely degrade soil fertility and causes many chronic diseases in humans. Due to the huge growth in the nickel industry and consumption of nickel-containing products, environmental pollution has become inevitable by the element nickel and also varieties of its by-products through all the phases of making, utilization and dumping. We have focused on the importance of agenda 2030 (UN 17 SDGs) during the preparation of the write-up and have highlighted goals 3, 6, 8, 9, 11, 12, 13, 14, and 15 by elaborately discussing associated points. The plausible molecular mechanism of nickel toxicity is presented in simple diagrams. The article elaborates on possible methods for remediation of nickel toxicity and the treatment of nickel dermatitis and nickel cancer. Recent advancements in the understanding of the dual aspects of nickel as beneficial and a carcinogen are the key subject of this article.
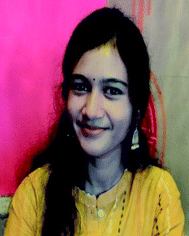 Wasefa Begum | Wasefa Begum was born in Burdwan, India. She pursued her M.Sc. from Presidency University, Kolkata, India and is currently working as a JRF at The department of chemistry, Burdwan University, WB, India. |
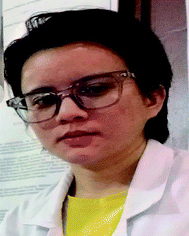 Summi Rai | Summi Rai was born in Bhojpur, Nepal. She is currently an M.Sc. student of Mahendra Morang Adarsh Multiple Campus, Tribhuvan University, Biratnagar, Nepal. She has published some international peer reviewed scientific articles on Surfactant chemistry. |
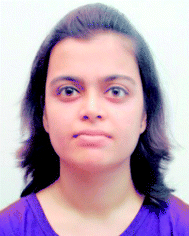 Soujanya Banerjee | Soujanya Banerjee was born in West Bengal, India. She perused her M.Sc. Degree from IIEST, Shibpur, India. She worked as a project student in the Bioremediation Laboratory under the supervision of Prof. Bidyut Saha. |
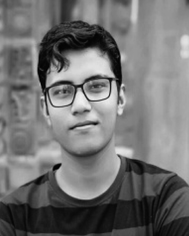 Sudip Bhattacharjee | Sudip Bhattacharjee was born in Birbhum, WB, India. He did his MSc from Bankura University India. He was a project student at Chemical Sciences Laboratory, GGDC-Singur under the supervision of Dr Monohar Hossain Mondal. |
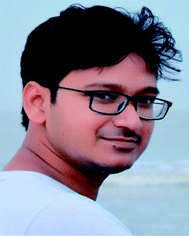 Monohar Hossain Mondal | Dr Monohar Hossain Mondal was born in West Bengal, India. He has completed his PhD degree from The University of Burdwan in 2017 from the Bioremediation Laboratory (The University of Burdwan). He is an Education Service Officer of the Government of West Bengal and is presently working as an Assistant Professor in Chemistry at Govt. General Degree College Singur, WB, India. His area of interest is bioremediation of toxic metals, surface studies and natural surfactants. He has published many international peer-reviewed scientific articles on Surfactant chemistry. |
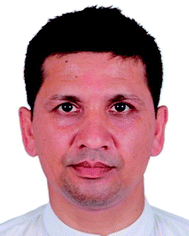 Ajaya Bhattarai | Dr Ajaya Bhattarai was born in Jhapa, Nepal in 1974. He obtained his PhD degree from the University of North Bengal, India in 2010. He was a post-doctoral fellow under Erasmus Mundus European Commission between 2012–2013 in the Department of Chemistry, University of Warsaw, Poland. He was also in Boston College, USA for Post-doctorate research under Fulbright fellowship between 2018–2019. Dr Bhattarai is presently working as a Professor in the Department of Chemistry, Mahendra Morang Adarsh Multiple Campus, Tribhuvan University, Biratnagar, Nepal. His area of interest is polymer chemistry, surfactant chemistry, nanomaterials, and crystallography. He has published more than 100 articles in reputed journals and book chapters. |
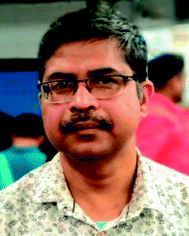 Bidyut Saha | Prof Bidyut Saha was born in Birbhum, WB, India in 1975. He obtained his PhD degree from Visva Bharati University, India in 2007. He was a visiting scientist between 2009–2010 in the Department of Chemistry, UBC, Vancouver, Canada. Dr Saha is presently working as the HoD and Professor in the Department of Chemistry, The University of Burdwan, India. His area of interest is the bioremediation of toxic metals, micellar catalysis and inorganic reaction mechanisms. He has published more than 140 articles and book chapters in international journals. |
1. Introduction
An imbalance in the relative abundance of a few metals in the ecosystem can transform those elements into potential threats to its flora and fauna.1,2 We have many works of literature about the toxicity of different metals; especially heavy metals (high-density elements) which can cause toxicity even at a lower concentration, making them one of the most significant environmental pollutants. Unlike the heavy metals, there are also some potential ‘light metal’ toxicants, which become a serious threat to the environment and eco-systems when exposed at elevated levels. Ni is one such metal. Ni and nickel-containing compounds are naturally distributed on the Earth's crust, ranking as the 24th most abundant element, and are constantly released into the atmosphere through various natural phenomena at modest levels.3 It is a recognized essential element for several important biological processes like the healthy growth of plants, animals, and soil/water microbes.4–6 With the increasing importance and demands of metallic Ni and its compounds, huge growth has been observed over the last few decades in commercial as well as industrial applications like electroplating, catalysis, electronics, pigments and coinage, batteries, ceramics, stainless steel, and other nickel alloys.7 Due to this high industrial demand, it is mined, extracted, and exploited at a higher rate. As a consequence, the disposal of industrial waste and fossil fuel combustion has resulted in anthropogenic emission of Ni into the different compartments (air, water, and soil) of the environment at elevated levels, leading to its unavoidable pollution.8,9
Nickel shows variable oxidation states with a span of −1 to +4, while the most stable oxidation state in nature is Ni2+.10 In natural sources, both soluble and insoluble Ni compounds are present. The insoluble forms, such as oxides, sulfides, and silicate minerals and soluble forms such as sulphates are common in the environment.11 Sulfides, oxides, silicates, and other soluble nickel compounds, as well as a small amount of metallic nickel, are released by the anthropogenic sources. Like many other metals, Ni is also required in trace amounts in several physiological and biochemical processes.12 It has been identified as one of the most essential micronutrients for the growth and development of plants such that its absence cannot be substituted by any other nutrient. However, its essentiality in human health is still in doubt, instead, its exposure to humans can cause severe and acute adverse effects on human health. Among the various speciation of Ni widely distributed in the biosphere, metallic Ni and some other specific speciation possess a potential threat to human health when exposed at an elevated level.13 Potential toxicity of Ni and its compound is thus dependent on its bioavailability which in turn depends on their physicochemical properties, as well as the amount, duration, and route of exposure. The primary routes of nickel exposure in humans occur via inhalation, ingestion, and dermal contact. A lifestyle including a high nickel content diet, prolonged occupational exposure to nickel deposits significant amounts of nickel species in the human body which indeed results in a variety of severe and chronic health effects. It is also known to impact non-occupationally exposed people, particularly those who work with stainless steel and nickel-plated everyday items. Among the workers of nickel mining, smelting, and refinery industries, severe skin allergy, cardiovascular diseases, kidney problems, lung fibrosis, lung, and nasal cancer have been diagnosed. Hepatic toxicity and nephrotoxicity have also been reported in various animal models and scientific studies. Besides, Ni exposure has also been reported to produce haematological defects in both humans and animals.10 With all such reports and evidence IARC (The International Agency for Research on Cancer) in 1990 has classified all nickel compounds except for metallic nickel to be carcinogenic to humans.13
The biosphere is constantly exposed to Ni at elevated concentrations due to its pervasive occurrence. Thus, Ni pollution will continue and will become a severe environmental hazard for future generations. We need to fix this problem immediately. However, we still are lacking proper knowledge on the speciation of Ni and its compounds, their physicochemical transformations, and reactions in the environment. Eventually, it is thus very much important to regularly monitor the environmental fate of nickel for a better understanding of its chemistry impacts on humans and the other stakeholders of the ecosystem. The current review aims to present a comprehensive and updated knowledge on the sources, essentiality, and toxicological profile of nickel to elicit inclusive ideas about the biochemistry of nickel to young readers as well as keen researchers all over the globe.
2. Biological essentiality of nickel
Nickel plays a well-established role in biological systems; in humans, the deficiency of nickel offers a reduced growth in intra-uterine development and is also accompanied by reduced iron adsorption which indeed leads to anaemia.14 Urease, hydrogenase, s-methyl coenzyme-M (CoM) reductase, acetyl CoA synthase, CO dehydrogenase, Ni-superoxide dismutase, glyoxalase I, and cis–trans isomerase are all essential Ni-containing enzymes.15–17
In plants, it contributes to the production of viable seeds because it is essential for iron absorption and seed germination. Nickel is also proven to prevent diseases in crops and increase crop yields.18 Nickel also acts as a bio-control agent by promoting the production of secondary plant metabolites, which indeed grow resistance in plants against various diseases and pests.19–21
Nickel deficiency is rare in humans since nickel is abundant in nature. Additionally, nickel is present in almost all types of food, which helps to maintain a nickel sufficient diet. Nickel depletion in higher organisms is associated with increased perinatal mortality, changes in grooming behaviour, and decreased growth. The body can also struggle to absorb iron when it is deficient in nickel. In the liver of nickel-depleted animals, iron, copper, and zinc concentrations are also found to be lower. Nickel deficiency leads to abnormal cellular morphology, oxidative metabolism, and abnormal lipid levels (increased or decreased).14 Furthermore, a decrease in growth and haemoglobin concentrations and impaired glucose metabolism is found to be true.14 Nickel deficiency also lowers the specific activity of many enzymes involved in carbohydrates and amino acids metabolism. A moderate iron-deficient diet also alters serum and hepatic lipids in similar ways as nickel exposure.15–17 It is also believed that the CO2-fixation of propinyl-CoA to D-methylmalonyl-CoA may involve nickel.
As evidenced by its depletion in the diet, nickel may also have involvement in the metabolism of lipids, principally in phospholipid synthesis. It has been established that human and rabbit serum contain nickel in three different forms: nickel bound to ultrafilerable ligands, nickel bound to albumin, and nickel bound to macroglobulins. In humans, rats, and bovine serum, albumin is the main nickel transport protein. Nickeloplasmin, a metalloprotein, has been isolated from rabbit (α – 2 macroglobulin) and human (α-glycoprotein) serums.21
3. Sources and occurrence of nickel
Nickel is a naturally existing element widely located in the earth's crust as well as the core. Nickel is distributed in the environment, air, soil, aqua, sediments, etc. from plenty of natural sources and also by anthropogenic activities. In this segment, we have tried to accumulate all the possible sources of nickel concisely.
3.1. Natural sources and occurrence of nickel
Nickel being a widely distributed metal in natural flora and fauna, is found in animals, plants soil, and varied water bodies. In soil, nickel is present in a concentration of approximately 79 ppm, with different abundances between 4 and 80 ppm.22,23
3.1.1. In atmosphere. It has been reported that approximately 30
000 tons of nickel per year are emitted into the atmosphere from its natural sources. The natural release of nickel into the atmosphere arises from windblown sand, volcanic activity, wild forest fires, sea salt spray, continental particulates, marine, and continental volatiles, and aerosols from oceanic dust, although volcanoes and windblown dust from rocks and soil are of prime importance.24
3.1.2. In surface and groundwater. There are many natural sources of nickel in the aquatic environment. The naturally occurring nickel is produced through weathering, dissolution, and atmospheric evaporation of nickel-rich rocks and soils. It is also present on clay particles as soluble salts and organic matter (detritus, algae, and bacteria) in aquatic systems or as soluble salts of humic acid and fulvic acid. Surface water can become contaminated with it as a result of the dissolution of primary bedrock minerals in rainwater.25 Nickel is more mobile in the soil after acid rain, thereby increasing its concentration in groundwater.20 Both surface water and underground water contain active nickel concentrations depending on factors such as soil type, sampling depth, pH, etc. Nickel concentration levels seem to be correlated more with its naturally occurring abundance in soil, minerals, rock, and soil dust.26
3.1.3. In soil and rocks. Nickel is mainly released to the soil as a result of atmospheric emissions. Nickel concentration in soils varies widely depending on the parent rock, however, its presence in surface soils is also affected by soil-forming processes and pollution. Based on geology and anthropogenic input, nickel concentrations in soil can vary greatly, but they typically range from 3 to 1000 parts per million. The presence of nickel in soils can take a variety of forms, including inorganic minerals that are absorbed on organic cation surfaces or complexed on inorganic cation exchange surfaces.23 It has been suggested that in soil solution, humic and fulvic acids are significantly more mobile than divalent cations that are hydrated.16 Among these minerals are pentlandite, nickel–iron sulfide, garnierite, and nickel–magnesium silicate. It is found in the lowest concentrations in clays, limestones, sandstones, and shales, while it is found in the highest concentrations in igneous rocks.21
3.1.4. In food. Human beings are exposed to nickel regularly because of its high abundance in nature. In general, nickel deficiency in the human body is difficult to occur rather it is hard to maintain a diet deficient in nickel due to its high occurrence in food. There are plenty of nickel-containing foods like vegetables (spinach, cabbage, lettuce, peas, lentils, etc.), fruits (almonds, plums, dates, pineapples, etc.), grains (buckwheat, 2.0 μg g−1; oats, 2.3 μg g−1; oatmeal, 1.8 μg g−1) cocoa beans, 9.8 μg g−1; soya beans, 5.2 μg g−1; soya products, 5.1 μg g−1; peanuts, 2.8 μg g−1; walnuts, 3.6 μg g−1; hazelnuts, dark chocolate, seafood (crab, oysters, shrimps, salmon, etc.) and many more.27 The quantity of nickel in a particular food is dependent on the plant species as well as the level of nickel in soil.Nickel intake from food and beverages is dependent upon the type of food, the country, the age, and the gender of the person. According to the information from research conducted in the USA, the average daily intake for adults is 101–162 μg per day, for males, it is 136–140 μg per day, and for females, it is 107–109 μg per day. Nickel intakes were determined to be 9, 39, 82, and 99 μg per day for children aged 0–6 months, 7–12 months, 1–3 years, and 4–8 years. The average intakes for pregnant and lactating women are higher at 121 μg per day and 162 μg per day, respectively.
Additionally, cooking utensils made a significant contribution to nickel levels in cooked food (e.g. oven pans, roasting pans). Calogiuri et al. (2016)28 found that stainless-steel pans cause a rise in nickel concentrations in acidic foodstuffs.
3.2. Nickel from anthropogenic sources
Nickel is emitted in many forms from anthropogenic sources, including sulfides, oxides, silicates, soluble compounds, and metallic nickel to a lesser extent. Nickel is present in ambient air, drinking water, food, and tobacco products. One mainstream cigarette releases approximately 0.04–0.58 μg nickel. As a result, smoking 40 cigarettes per day may cause you to inhale 2–23 μg of nickel.29 Nickel is emitted from inefficient vehicles' engines during transportation due to their lubricants. Several other applications of nickel are related to oil refining, cryogenic containers, pollution abatement equipment, and plumbing materials. There is the possibility that nickel can leach into drinking water when pipes and other materials corrode, but these leaks are generally small.30 Other sources of nickel exposure are nickel alloys and nickel-plated items, such as steel, coins, and jewellery, and residual nickel can be found in soap, oils, and fats.
3.2.1. Nickel in air from anthropogenic sources. Primarily Nickel is released into the atmosphere as aerosols as a result of human activities.6,17 Approximately 1.4–1.8 times more emissions are generated by humans than by nature. During the 1980s, the combustion of fossil fuels accounted for 62% of anthropogenic emissions of atmospheric nickel. The combustion of fossil fuels released 570
000 tons of nickel worldwide in 1999. Among these, 326 tons were released from electric utilities, while municipal incineration accounted for 12%, nickel-metal refining accounted for 17%, steel production produced 3%, and other nickel-containing alloys 2%.31
3.2.2. Nickel in water from anthropogenic sources. In addition to the nickel emissions from industrial processes (viz. mining, processing, smelting), industrial wastewaters and effluents (e.g., tailing pile run-off), as well as domestic wastewaters, landfill leachate can also contain nickel. According to reports, approximately 0.5–2 ppb of nickel is present in seawater while rivers contain about 0.3 ppb of nickel species.32 Bureau of Indian standards (BIS 2012) have recommended the acceptable limit of nickel in drinking water is 20 μg L−1.33The concentration of dissolved nickel in groundwater with pH < 6.2 has been measured up to 980 μg L−1. Urban storm runoff water samples have been found to contain nickel levels ranging from <1–87 g L−1.28,29,34
3.2.3. Nickel in soil from anthropogenic sources. Nickel levels in the soil come from anthropogenic sources such as metal manufacturing waste, commercial waste, fallout, and sludge, coal fly ash, coal bottom ash, mining, and smelting. Around 5530 and 14
800 metric tonnes of nickel and its compounds respectively were estimated to have been released into the environment from US manufacturing and processing facilities in 2002 and the quantity is about 82% and 87% of the estimated level of nickel released to the environment.35 The problem related to nickel seems to be limited to urban areas, but it might also cause problems in agricultural soils due to reduced soil liming and acid rain for industrialization.31
4. Nickel exposure
Nickel exposure is an issue for both the general population as well as workers of the nickel industry. It enters the human body by various means viz. food, drinking water, dermal contact, inhalation, etc. Later in the next section, the details of its various exposure routes and consequences are discussed.
4.1. Exposure of the general population
Nickel can be consumed orally, inhaled, or absorbed through the skin. Nickel is primarily ingested by nonsmokers through food and drinking water. Inhalation of ambient air and percutaneous absorption are other methods of exposure.31 Nickel is mainly excreted by the faeces after being swallowed orally. Nickel-containing endoprostheses, radiocontrast media, haemodialysis fluids, and/or albumin may also be related to nickel-related iatrogenic exposures, such as parenteral nickel exposure from nickel alloy jewellery, coins, or utensils, as well as cutaneous nickel absorption from nickel-plated items.36
4.2. Occupational exposure
Nickel, in its various alloy forms and many compounds, has been utilized commercially for ages.37 Nickel-producing firms such as mines, mills, smelters, and refineries, as well as nickel-consuming organizations and operating industries such as alloy and stainless-steel manufacturing; electroplating and electrowinning; welding, grinding, and cutting, are exposed to its toxicity through breathing, dermal contact, or accidental ingestion. The poisonous intermediate product, Ni(CO)4, was first assumed to be the sole carcinogen because the Mond process was used to purify nickel. However, refineries that did not use the Mond process had an elevated risk of respiratory malignancies.
The scientific reports figured from the studies on occupationally exposed clusters indicate that the incorporation of nickel and nickel-derivatives by breathing inhalation introduces many diseases, such as sinusitis, rhinitis, anosmia, etc. A prolonged inhalation exposure leads to the complete damage of the nasal septum. The high exposure and ingestion of nickel derivatives cause breathing shortness, headache, cough, nausea, vomiting, and diarrhoea. While in its severe form it becomes fatal and causes death in both animals and humans. As a result of analytic confusion about precisely which form of nickel was the causative agent resulting from co-exposure to different forms of nickel during processing, results were inconsistent and inconclusive at times. In the 1930s and 1970s, researchers had difficulty in distinguishing between the effects of metallic nickel and individuals exposed to it, since both processes occur jointly.38 Additionally, nickel particles and compounds that have varying charges can be generated during the melting of the nickel ores – workers can be exposed to Ni3S2, NiSO4, NiCl2, NiO, NiCO3, Ni0, Ni–Fe oxides, and Ni–Cu oxides during various smelting and refining processes, and determining bioactive species can be challenging. However, it was fairly clear there was a difference between nickel operations with “low” and “high” exposure, in line with the levels of respiratory cancer risk. In recent efforts, complex sampling techniques have been utilized to create “fingerprints” of workplaces that provide information about species of Ni and size categories.4 There is still a need to better understand workplace exposure limits today. Another puzzling factor was the possibility that Ni could increase the carcinogenicity of other toxins when used in conjunction. National Occupation Exposure Survey (NOES), which was conducted in 1981–1983 reported, 507
681 workers including 19
673 females, were severely exposed to Nickel toxicity (agent code: 50420) at work.39 Nearly 60% of exposed workers were employed in the following six industries: fabricated metal products (n = 69
984), equipment for transportation (n = 44
838), primary metal industries (n = 39
467), and auto repair service and garages (n = 27
686).
4.3. Other forms of exposure
Nickel allergies commonly cause contact dermatitis. Consumer products should be free of nickel, chromium, and cobalt levels exceeding 5 parts per million (ppm) and the ultimate goal should be 1 ppm. According to a contemporary survey, the following consumable products contained nickel (ppm): hand-wash powder, 0.9; heavy-duty powder, 0.5; laundry tablets, 0.5; liquid/powder cleaners, 0.4; heavy-duty liquids, 0.1; machine/hand-washing liquids, 0.1; hand-washing liquids, 0.1; fine wash liquids, 0.1; and dishwashing liquids, 0.1. Dialysis treatment, nickel leaching from nickel-containing alloys, and contaminated intravenous medications are all potential sources of nickel exposure.39
5. Inter-cellular transportation and uptake of nickel by cells
Integrating a toxin within a cell is often a crucial step in determining whether or not the toxin will produce mutagenic or epigenetic effects on the cell. The bioavailability of such toxic elements is dependent on the mechanism by which they are absorbed through cell membranes, distributes within cells, and bound to macromolecules therein. To understand how and why some Ni forms are taken up by the body and others are not, we must learn how and why some forms of Ni are toxic while others are not. Previously two main categories of Ni compounds viz. soluble and insoluble nickels were supposed to be the only toxins but in contemporary days four specific categories of Ni compounds viz. soluble nickel, sulfidic nickel, oxidic nickel, and metallic nickel are listed under observations.40
Several proposals have been made to classify the uptake routes of nickel compounds based on their size, shape, and surface characteristics. Max Costa and colleagues in their studies have used tissue culture cell systems and a variety of other fibroblast cell lines to demonstrate uptake and intracellular fates of crystalline nickel sulfides and nickel subsulfides compounds.26,41 The crystalline nickel particles were found to have phagocytized by the culture cells in presence of the internal acidic pH, which eventually facilitates nickel solubilization and indeed increases the concentrations of soluble nickel inside the cell.42 This Ni-filling then actually exercises their carcinogenic effects on the nuclear proteins and DNA inside the cell. Unlike the crystalline nickel particles, the poor absorption of amorphous nickel sulphide and water-soluble nickel salts result in lower nuclear and higher cytosolic nickel contents.26,41,42 Fig. 1 illustrates how surface charge influences phagocytosis. Nickel sulfide particles with a crystalline structure have an inclination towards a negative charge on their surfaces, while the amorphous nickels show a positive charge. Therefore, the degree of carcinogenic potency of these different nickel compounds seemed to be determined by their delivery into cells. After phagocytosis, the particles are captured in vacuoles that became highly acidic, allowing soluble nickel to be disintegrated from the particles, thus increasing the level of nickel in the cells. According to studies, nickel ions or complexes that result from the dissolution of the soluble nickel particles are promptly excreted through ciliary movement. The insoluble particles, on the other hand, can be phagocytosed by macrophages or epithelial cells. As these particles diffuse through the cell, they slowly dissolve (e.g., Ni3S2 dissolves by oxidation), providing a continuous flow of Ni(II) ions.43
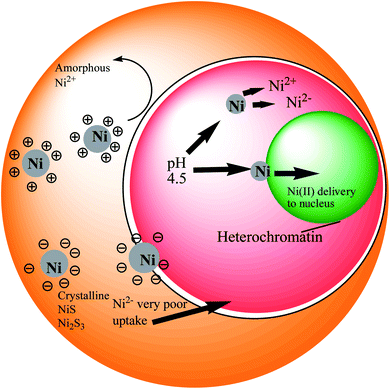 |
| Fig. 1 Nickel uptake model in a living cell. | |
The specific mechanisms through which nickel enters into interstitial areas proximal to capillaries are unknown after ingestion, although it passes through the intestinal epithelium. According to science, because the junctions of the intestinal epithelium are impermeable and tightly packed, a specific transport process could regulate the way nickel absorbs from the lumen and moves to the interstitial space.
Several studies have proposed that trans-membrane diffusion is responsible for the absorption and secretion of nickel by the rats' jejunum.44 Nickel ions can also be transported via Ca2+ channels. In human kidney epithelial cells, surface binding and uptake of Ni2+ were investigated, and Ca 2+ channels are important for nickel transport, as the calcium ionophore potentiated nickel uptake.44
6. Environmental chemistry and speciation of nickel
Various geogenic processes constantly distribute Ni between land, water, and air. In the atmosphere, Ni occurs at relatively low concentrations. Nickel released into the atmosphere mostly exists in particulate form with varying diameters depending on the source.45 Ni particulates with anthropogenic origin have a certain range of diameters lies between 0.1 μm to 2 μm, while those from natural sources have diameters ranging between 2 μm to 10 μm. Fine Ni particulate (<10 μm) have a longer residence time in the atmosphere (5–8 days) and can be transported over longer distances as compared to larger Ni particulates. Dispersion of Ni particulates occurs by the wind and deposition from the atmosphere occurs by gravitational settling (>5 μm) or dry and wet depositions (<5 μm).46 The Ni particulates however do not absorb infrared radiation and hence might not contribute to global warming or the stratospheric ozone depletion.47
However, there is limited knowledge about the chemical forms, chemical and physical transformations it undergoes throughout the atmosphere. Because from analytical methods, information about the metal content is obtained rather than its specific or generic compounds. As a consequence of such limited knowledge about the specific information of varied compounds, some assumptions are made regarding this variation. It is generally assumed that in the case of Ni from anthropogenic sources, specifically those obtained from the combustion process are present in the form of oxide of nickel (nickel oxide). While the windblown dust particles contain mineral sulfide species of Ni. Nickel sulfate is probably produced in the atmosphere from the oxidation of Ni in the presence of sulfur dioxide.48
Most of the nickel released into the aquatic system exists as soluble salts associated with particulate matter, suspended solids, or in combination with organic matter originating from biological sources.46 The fate and bioavailability of Ni in the aquatic system are governed by its partition between particulate solid phases and soluble phases by phenomena such as adsorption, precipitation, coprecipitation, and complexation which depends on the redox potential, pH, the ionic strength of the water, the concentration of metal and ligand and presence of solid surfaces for adsorption (in particular, hydrous iron and manganese oxides). For example, under anaerobic conditions (underground water), nickel concentration remains low because of the precipitation of nickel sulfide. Relatively insoluble nickel sulphide is produced in some sediments under reducing conditions and in the presence of sulphur. The compounds formed by the nickel with hydroxide, sulphate, carbonate, and naturally existing organic ligands under aerobic conditions and pH < 9, are considerably soluble to maintain concentrations of aqueous Ni2+ above 60 μg L−1.47,48
The divalent ion is the dominant form in natural waters at pH values ranging between 5–9, existing as the octahedral, hexahydrate ion (Ni (H2O)6)2+. The presence, existence, and stability of other nickel compounds depend upon the pH and their inorganic or organic binding partners.13 Solubility property of Nickel chloride hexahydrate and nickel sulfate hexahydrate is extremely high in water about 2400–2500 g L−1. Nickel compounds having lower aqueous solubility include nickel nitrate and nickel hydroxide with solubilities of 45 g L−1 and 0.13 g L−1 respectively, and the most inferior one is nickel carbonate having aqueous solubility of 0.09 g L−1. Nickel produces strong and soluble complexes with SO42−, OH− and HCO3−; nevertheless, these species are minor in comparison to hydrated Ni2+ in groundwater and surface water.45
Most of the Ni released to the environment gets accumulated in the soil where it is strongly adsorbed by various adsorbing species, most importantly amorphous oxides of Fe and Mn including other soil minerals. In maximum soils, Ni is bound to ion exchange sites and is specifically adsorbed or coprecipitated with aluminium and iron oxyhydroxides. The extent of Ni adsorption by soil depends on the properties of the soil such as bulk density, pH, texture, organic matter, the type and amount of clay minerals, and certain hydroxides, additionally the extent of groundwater flow, influence the retention and release of metals by soil. So, the adsorption of Ni by soil is site-specific. In acidic soil, the mobility of Ni is more while in alkaline soil adsorption is irreversible which limits both availability and mobility of Ni in soil.48,49 In acidic soil solution, the most common forms of Ni that have been identified are Ni2+, NiSO4, and NiHPO4 and in the alkaline medium are Ni2+ and Ni(OH)+.45 In loessial soil, Ni slowly transforms from exchangeable and carbonate fractions into the reducible oxides and the organic and easily reducible oxides fractions in sandy soil.50 H. B. Xue and co-workers in their study reported fantastic data about nickel speciation in lake, river, and groundwater samples.51 They employed the ligand exchange technique with DMG and DPCSV. They observed pseudo-first-order kinetics of the ligand exchange reactions and concluded in their article that, the dissolved concentration of nickel in their water samples ranged from 4 nM (in lake water) to 30 nM (in river water) affected by the sewage and agriculture effluents. The study also revealed that 99.9% of dissolved nickel was bound by organic ligands with high binding constant values. Another study by Mandal and co-workers also supported similar findings in different water samples of their study.52
In a recent study by Yebra-Biurrun et al.,53 they have tried to find out the amount of labile Ni (the actual toxic nickel) in sea-water and river water samples. Their simple and effective instrumentation was the combined and continuous sono-digestion and solid-phase extraction of Ni by the use of chelating resin. They were able to determine both the labile (free) nickel as well as organic-bound nickel complexes in various water samples. The study also claimed similar findings with Xue and co-workers51 and Mondal and co-workers.52 These studies on nickel chemistry and real-time determination of nickel species in the environment suggested the presence of dissolved Ni-organic bound species and also 0.01% of free elemental Ni in different water bodies (Fig. 2).
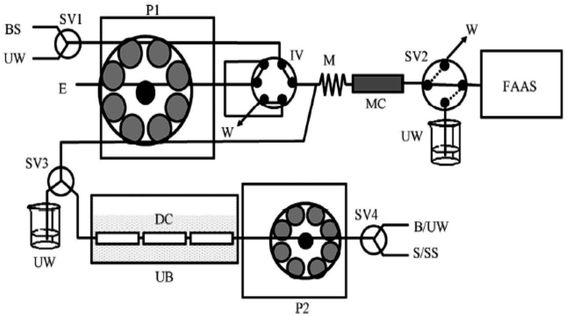 |
| Fig. 2 A schematic representation of the flow injection model used for the determination of total and dissolved labile Ni(II) in various environmental water samples. P1 and P2: peristaltic pumps; B: blank; E: eluent; BS: buffer solution; M: mixing coil; DC: digestion chamber; FAAS: flame atomic absorption spectrometer; IV: injection valve; MC: microcolumn containing the resin Serdolit Chelite Che; S/SS: sample or standard solution; SV1, SV2, SV3 and SV4: selecting valves; UB: ultrasonic bath; UW: ultrapure water; W: waste. This scheme is represented with permission from reference no. 53. Copyright SciRes 2011 (http://www.SciRP.org/journal/ajac). | |
7. Nickel toxicity in plants
As micronutrients, nickel is necessary for cellular redox reactions and essential for plant growth and development. But the presence of Ni above the allowed limit, however, alters the functions of many cellular components, which can ultimately result in cellular damage and death of the plant.54 There are so many toxic effects of nickel on the plant if the concentration exceeds the optimum intake level. Excessive accumulation of nickel in plant cause disruption of iron (Fe) uptake and metabolism which results in chlorosis and necrosis in plants.55,56
Ni also inhibits, modifies, or enhances the structure/activity of a large number of target molecules. Ochiai57 in his book claimed that three events generate toxicity in transition metals, including Ni. As a result, the metal can [a] remove essential components from the biomolecules, [b] block the function of an essential biological group in the molecules, and [c] change the structure or function of enzyme/proteins, plasma membranes, and membrane transporters. Besides the oxidative effects of Ni, Ni also inhibits root and shoot growth as well as decreases leaf area by interrupting cell division (Scheme 1).58
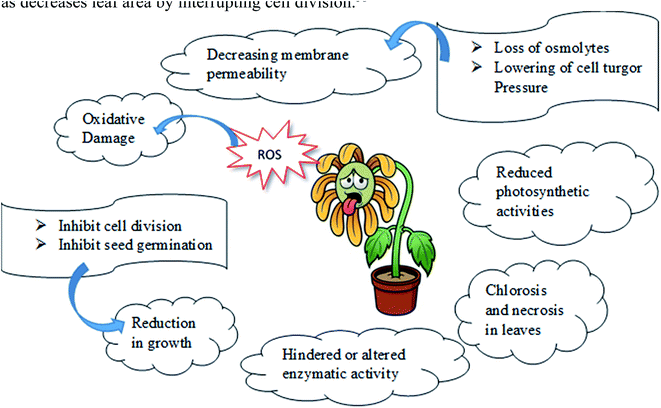 |
| Scheme 1 Schematic representation of nickel toxicity in plants. | |
Besides hampering the growth of plants, it also disturbs the plant anatomy and morphology by decreasing the size of the vascular bundle, the thickness of mesophyll, plasticity of a cell wall, chlorophyll content and many more activities resulting in hampering in seed germination and other metabolic processes.59 Additionally as an obvious consequence, the photosynthesis mechanism is disturbed in plants. Nickel hampers photosynthesis in plants in both direct and indirect ways among which the prime activity is to alter or displacing the essential metal ions like Mg by itself resulting in hindering the activity of chlorophyll. Other ways include disruption in chloroplast structure, interference in electron transport chain reaction, hindering the enzymatic activities, decreasing photosynthetic pigments, and many more.60 Because of nickel stress, the rate of transpiration and stomatal conductance are found to decrease which results in reduced water intake by plants.61
Excess of nickel level also causes nickel stress resulting in loss of osmolytes as well as a decrease in cell turgor pressure. As a consequence, lowering of membrane permeability occurs causing leakage of many essential ions through the cell membrane in plants.62,63 Formation of reactive oxygen species like superoxide, peroxides, alkoxy or hydroxyl radicals in plant body occurs due to excessive accumulation of nickel in growing medium resulting in oxidative damage of plant organelles.64
8. Nickel toxicity in humans and animals
The toxicity of any compound is determined by its physicochemical properties, concentration, and its exposure pathway. As indicated by occupational exposure studies on humans and animals, the principal target of toxic effects appears to be the respiratory tract following oral ingestion, the skin and inhalation exposure and the reproductive system in humans following oral exposure. Almost all nickel compounds, except for nickel carbonyl, are nontoxic after ingestion because of their low absorption from the gastrointestinal tract. Nickel salt bioavailability is significantly affected by parallel consumption of food. It appears to be excreted rapidly through the urine and the elimination seems to follow first-order kinetics without evidence of dose-dependent elimination. In addition to its embryotoxic and teratogenic properties, nickel can cross the placenta. A study in hens revealed that nickel exposure decreased the magnesium, manganese, and zinc levels in various tissues.53
According to studies, nickel and zinc had antagonistic effects on animals and humans.36 In addition to its cancer-causing properties, nickel can also cause sensitization reactions in humans (Scheme 2).6,17
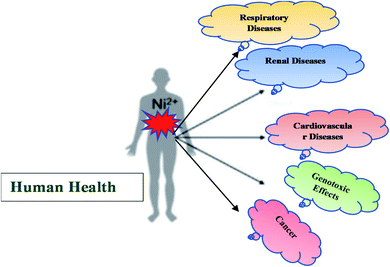 |
| Scheme 2 Schematic representation of nickel toxicity in humans. | |
8.1. Nickel carbonyl toxicity
Inhalation of Ni(CO)4 by workers usually causes non-specific, immediate symptoms such as nausea, vertigo, headache, dyspnea, and chest pain. In a person exposed to nickel carbonyl, two stages of symptoms occur: immediate and delayed (Toxicology Department CRCE 2009).65 Initial symptoms typically disappear within a few hours, but in case of prolonged and high exposure between 12 and 36 hours and in some cases up to 5 days, severe pulmonary symptoms appear, including coughing, dyspnea, tachycardia, cyanosis, and profound weakness. The delayed pulmonary symptoms appear following an asymptomatic period. The most severe cases of pneumonitis can lead to pulmonary haemorrhage, cerebral oedema, toxic myocarditis, pulmonary oedema and neurasthenic syndrome. It has also been reported that deaths have occurred 4 to 13 days after high exposure to Ni(CO)4. Most of the time, the cause of death has been diffuse interstitial pneumonia and cerebral haemorrhage.66 According to the EU, nickel carbonyl is a reproductive toxicant (Risk Phrase R61—“may harm an unborn child”). Animals exposed to nickel carbonyl have been shown to produce malformations in their offspring.65 A table is given below containing the acute toxicity, subchronic toxicity, and chronic toxicity due to nickel exposure in humans and rats (Table 1).67
Table 1 Extreme health effects: acute toxicity, subchronic toxicity, and chronic toxicity due to nickel exposure in humans and rats67
Toxicity |
Acute toxicity |
Subchronic toxicity |
Chronic toxicity |
Exposure period |
01 day |
10–100 days |
>100 days |
Route of exposure |
Exposure to nickel fumes and nickel dust, nickel polluted water, and direct consumption or inhalation of nickel compounds |
Exposure to nickel fumes and soluble nickel compound |
Occupational exposure to nickel dust |
Extreme health effects |
On human |
Respiratory distress syndrome, cardiac arrest |
Tubular dysfunction, visual dysfunction |
Asthma, bronchitis, pulmonary and nasal cancer, disruption in oxidative phosphorylation |
On rats |
Renal damage, frank hematuria |
Liver and kidney failure, hyperglycemia, ataxia, hypothermia, diarrhoea, lung fibrosis |
Loss of kidney weight with significant albuminuria, emphysema |
8.2. Toxicity due to other forms of nickel
Nickel compounds with more soluble forms are more toxic than nickel compounds with less soluble forms. The highest toxicity has been reported for soluble nickel sulphate and the lowest toxicity for insoluble nickel oxide.28 The acute oral LD50 values of nickel sulphate were 46 mg kg−1 in male rats and 39 mg kg−1 in female rats.
It has been shown that the LD50 values in the case of nickel oxide, less soluble nickel compounds, and sub sulphide for rats were >3930 and >3665 mg kg−1, respectively. For nickel acetate, the oral LD50 is 350 mg kg−1 for rats and 410 mg kg−1 for mice. Two to three hours after exposure, diarrhoea, distress, and lethargy were noted in animals that ultimately died.31,57,65 Nickel oxide was also reported to increase lung tumours both in females and males, as well as in the adrenal medulla (malignant and benign pheochromocytomas) of rats exposed at low doses.31 Male rats exposed to metallic nickel had an increased incidence of benign adrenal pheochromocytomas and a higher incidence of benign and malignant adrenal tumours. Female rats exposed to metallic nickel had an increased incidence of adrenal cortex tumours. Adrenal tumours of both types showed dose-related responses. Despite elevated blood levels of nickel after inhalation of metallic nickel, lung tumour formation was not significantly increased. Metallic nickel dissolves in the body and releases ionic nickel, which indeed acts as the geno-toxicant and carcinogen. Nickel sub sulphide was also reported to cause local sarcomas in mice in several studies after multiple injections at multiple sites.31,57,65 Nickel sub sulphide also caused respiratory, liver, and spleen metastases in rats.35
9. Specific health effects of nickel toxicity in humans
9.1. Effects on the respiratory system
The acute effects of nickel inhalation on humans are not well studied. Exposure to nickel for 90 minutes at a dose of 382 mg m−3 was reported to fatally affect the respiratory system of adults.65 Workers in the nickel industry are reported to have perforations of the nasal septum, chronic rhinitis, and sinusitis. There are also reports of pulmonary fibrosis and decreased pulmonary residual capacity for those working with nickel dust or fumes. Radiological evidence of nickel pneumoconiosis in those workers exposed to nickel dust over long periods has also been reported. Twelve steelworkers who worked as distempers for up to 16 years of storage of steel ingots were studied for their respiratory symptoms, chest radiographs, and lung ventilation capacities.68 Typical workplace fume concentrations were in the range from 1.3 to 294.1 mg m−3, with all three oxides are iron, chromium, and nickel in a composition of 6
:
1
:
1 (fumes from stainless steel) and 98.8% iron oxide (fumes of special steel). From these values, 0.15–34 mg m−3 concentrations of nickel oxide were calculated. The study reported a measurable loss of lung function in two workers, but the radiographic signs of pneumoconiosis were evident in five men.68
9.2. Effects on the renal system
A male worker who ingested contaminated water containing nickel sulphate and chloride experienced nephrotoxic effects including edema, hyperaemia, and parenchyma degeneration. On the fifth day after exposure, the urine-albumin concentrations returned to normal (68, 40, and 27 mg g−1 creatinine). The results suggest that nephron-toxication was mild and transient. Several enzymes involved in the metabolism of ascorbate cholesterol and maker enzymes are altered by nickel.69
9.3. Effects on the cardiovascular system
In a report of Sunderman, Jr, et al.66 it was suggested that a high serum nickel level can cause coronary vasoconstriction if a patient has angina pectoris and myocardial infarction. There is no known mechanism or source of nickel release, however, some recommend limiting intravenous nickel content to 5 μg kg−1 per day. The study revealed increased lung and nasal cancer rates in nickel refinery workers who have spent 5 years in the process area. 16 cases of cerebrovascular death were reported compared with 8.5 expected from the study.66
9.4. Effects on skin
Unlike the absorption of nickel via inhalation and ingestion, nickel ions cannot penetrate through intact skin. Contact dermatitis can be caused by the absorption of nickel-metal dust, nickel alloys, and nickel salts on wounded skin.13 Nickel is the most prevalent cause of contact dermatitis among the general public. Jewellery, buttons, and zippers are responsible for prolonged dermal exposure in people. Studies reported that approximately 10% of females are nickel sensitive and the condition is associated with ear piercings in particular.70 A nickel-sensitive person's T-lymphocytes get stimulated to proliferate and secrete cytokines in vitro by nickel. Major histocompatibility complex-encoded molecules may not be required for this stimulation, nickel-induced proliferation remains unaffected by antibodies to HLA class I and II molecules. Nickel stimulates the immune response in humans in vivo as well as in nonallergic and Ni-sensitized individuals. Mobile phones have been identified as a new source of contact dermatitis among children and adolescents.71
9.5. Genotoxic effects
The workers of nickel industries working in crushing, roasting, and smelting procedures are highly exposed to nickel sulphides and nickel oxides. Workers in the nickel-electrolysis department are generally exposed to nickel chloride and nickel sulphate. Cytogenetic studies on both types of workers revealed dangerously increased stages of chromosome aberrations, mainly gaps. Shockingly, the same report was found in retired nickel workers, too. The report established a 2 μg L−1 plasma nickel level in those retired workers.71
As a result of nickel compositions, which are weakly clastogenic, specific chromosomal damage has been shown to occur, particularly in the heterochromatic regions. There is a pronounced effect in the heterochromatic long arm of the X chromosome of the Chinese hamster, which is frequently decondensed and aberrations are formed as a result of insoluble Ni3S2 and soluble NiCl2.
9.6. Carcinogenicity of nickel (nickel cancer)
A shameful name was given to nickel in 2008: “Allergen of the Year”. Previously, only the water-insoluble nickel compounds or dust such as Ni3S2, NiO, etc. were considered to be carcinogenic but epidemiological data from recent studies have shown that nickel aerosols (e.g., Ni(II) sulphate) created in nickel electrorefining plants are also carcinogenic to the human respiratory system. For nickel particles injected intramuscularly, the order of carcinogenic activity towards rats has been determined as Ni3S2∼β NiS (nickel(II) sulphide) > NiO (nickel(II) oxide) ≫ Ni0 ⋙ NiS (amorphous).6,36,70,71 In the nickel refining industry the effluent by-products like nickel sulphate, sulfides, and oxides are carcinogenic when exposed to humans during nickel refining. A case study was conducted on the 100 sino-nasal cancer cases in nickel refinery workers. Squamous cell carcinomas accounted for 48%, anaplastic and undifferentiated carcinomas for 39%, adenocarcinomas for 6% and transitional cell carcinomas for 3%, anaplastic small cell and oat cell carcinomas for 15%, large cell carcinomas for 3%, other malignant tumours for 1% and unspecified cancers for 6%. This study indeed provides us with strong evidence of occupational nickel inhalation causing squamous cell carcinomas.36 Other than occupational exposures, nickel contained in endoprostheses, bone fixing plates, screws, and other medical devices has been suspected, though not proven, to be the main reason behind sporadic local tumours in humans.36
10. General mechanism of nickel carcinogenesis
The carcinogenicity of nickel is not well understood yet in terms of its molecular mechanisms, various possible ways for the development of cancer have been suggested by scientists. The interaction of metals with cellular bodies inside a living cell is a complex phenomenon to even properly understand. Extensive research and studies have reported three major mechanisms that might be satisfactory for understanding carcinogenic interactions of any metal. These mechanisms are applicable for most of the carcinogenic metal compounds as well as for several reactions regarding specific metal compounds. These are [i] oxidative stress, [ii] DNA modulation, and [iii] disturbances of signal transduction pathways.4,26,44 In the coming segments we have tried to explain all the three interactive mechanisms in terms of nickel in cellular conditions (Fig. 3).
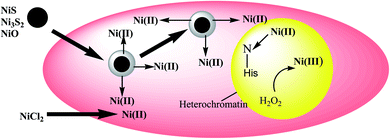 |
| Fig. 3 Generalmechanism of nickel carcinogenesis. | |
10.1. Nickel induced oxidative stress
Mutagenic and carcinogenic effects of any metal can be explained by their inducing oxidative stress. It has been reported that metal ions being capable to perform redox reactions in biological systems, such as antimony, arsenic, nickel, etc., can induce the production of reactive oxygen and nitrogen species in mammalian cells in both vivo and vitro. The produced oxygen radicals then induce oxidative damage to proteins, lipids, and DNA of the cells (Fig. 4). These oxygen radicals are generally produced following the Fenton and Haber–Weiss-type reactions inside mammalian cells.72,73
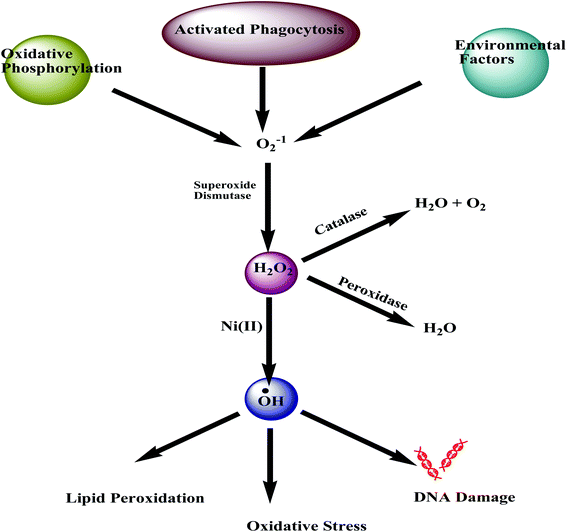 |
| Fig. 4 Nickel induced oxidative stress. | |
The spectrofluorometric DCF methods are applied to understand the possible reactions of nickel inside a cell by measuring ‘ROS’. The dichlorofluorescein (DCF) fluorescent method is one of the best and most popular tools for measuring the cellular reactive oxidant species called the ‘ROS’. For a nickel, it has been reported that it produces low levels of free radicals in cells. The soluble NiCl2 and insoluble Ni3S2 both improves the formation of intracellular oxidant after 6 hours of incubation inside the cell.72,73 Cells that are exposed to crystalline Ni3S2 are found to produce more free radicals after 18 hours of ingestion.72 The oxidative mechanisms associated with the genotoxicity of nickel thus are directly linked to the speciation of nickel and the type of cells it is interacting with. The release of reactive oxygen species (ROS) by nickel carbonate hydroxide causes sister chromatid exchanges to occur in human lymphocytes even at low concentrations, although it has been found that NiCl2 in hela cells causes DNA oxidative damage only at a very high concentration.72
In addition to the counting of free radicals directly, an indirect way of measuring oxidative stress is also marked by the depletion of the antioxidant glutathione (GSH). ROS like free radicals, superoxides, peroxides, lipid peroxides, and heavy metals can extensively damage important cellular components of GSH present.13 Metabolic biochemical reactions like DNA synthesis and repair, actually utilize the enzyme GSH. In nickel contaminated cells, a significant diminishing of GSH levels is reported.13
According to the data reported by Denkhaus and Salnikow,13 nickel can specifically damage genetically inactive heterochromatin through oxidative DNA damage. As a result, nickel might be mutagenic in some mutational assays. Nickel reportedly oxidized bovine serum albumin in a dose-dependent manner, where the formation of carbonyls is detected in vitro in intact nickel-containing cells (CHO).72
The activation of some transcription factors, including NF-kB, AP-1 and hypoxia-inducible factor-1 (HIF-1), may also be influenced by reactive oxygen species. Reactive oxygen species or the GSH depletion in cells exposed to nickel could be responsible for setting off the activation of these transcription factors.
10.2. Interference with DNA repair
Several DNA repair pathways, partly overlapping in mammalian cells, operate at the same time, which includes excision, mismatches, nucleotide excision, and recombination. Inheritance or acquisition of deficiencies in such pathways can lead to malignant growth.17
NiO and Ni(II) compounds, despite their weak DNA-damaging properties, are capable of sufficiently reducing the synthesis of DNA adducts in response to benzopyrene, a well-known environmental contaminant. The presence of Ni(II) also inhibits cell cycle progression and cell cycle control after exposure to ultraviolet radiation.74
DNA damage checkpoints in a healthy cell regulate the cell cycle as a result of DNA repair activities. A cell's ability to freeze the cell cycle in response to DNA degradation is controlled by these checkpoints. In some cases, they also control the DNA repair pathways, the movement of DNA repair components to mutilated DNA, and the initiation of transcriptional programs. Throughout the cell cycle, these control mechanisms remain active. Flow cytometry studies of carcinogenic metal compounds revealed that Ni(II) inhibited the progression of cell division in human lung tumour cells.74
At subtoxic concentrations of NiCl2, DNA repair, O6-alkyl-guanine repair, and oxidative DNA damage repair were all found to get prevented. Recent studies also showed that nickel sulphide silencing of the O6-methyl guanine-DNA methyltransferase gene could lead to lung cancer. In addition, nickel(II) inhibits the degradation of a specific DNA GTPase which breaks down 8-oxo-dGTP.74 In particular, DNA damage-response pathways have been observed to be sensitive towards toxic metal ions in particular and this is due to inhibitions at low noncytotoxic metal compound concentrations, and thus DNA repair and cell cycle control may be altered.
In addition to toxic metal ions, some zinc finger proteins can bind zinc to sulphydryl groups. There are four cysteine or histidine residues in the zinc finger domain, which are crucial in interacting not just with DNA, but also with other proteins as well.17 Although most zinc finger structures are DNA repair enzymes. The results concerning zinc finger DNA repair enzymes and carcinogenic metal compositions have been summarized recently: Ni(II) inhibited XPA's DNA binding. The poly(ADP-ribose) polymerase (PARP)-1 is another zinc finger protein activated by DNA damage, after induction of DNA strand breaks, poly(ADP-ribosyl)ation of various proteins occurs.75
Despite nickel's infrequent interaction with DNA, it has a significant effect on proteins. Depending on the type of amino acid, the degree of affinity for histidine residue is different. Heterochromatic DNA is known to interact with Ni(II), probably due to Ni(II) having an extremely high affinity constant for amino acids (such as histidine and cysteine). Other amino acids had affinity constants in the order of 105, while Ni(II) had an affinity constant of 102 for DNA. Therefore, Ni interacted with DNA through molecules associated with it and not directly through DNA.75 In addition to possessing a unique compact DNA–protein structure that has a superior affinity for DNA–protein interactions, heterochromatin is also found inside the interphase nucleus, which makes it an ideal location for the initial interaction with any geno-toxicant. A second reason for the selective interaction of Ni with magnesium binding sites is that Mg(II) is an important component of the maintenance of heterochromatin.76 It has been shown that Ni can cause heterochromatic decondensation when it interacts with heterochromatin.76 In Ni-induced transformation, many of these heterochromatic regions were found to have major chromosomal deletions that were originally thought to be caused by Ni treatment.76 Ni bound to the imidazole nitrogen of histidine, for example, was oxidized by hydrogen peroxide. In addition to catalyzing the covalent cross-linking of amino acids with DNA, Ni was also involved in the cross-linking of proteins with DNA. Tyrosine and histidine are therefore amino acids that can be incorporated into DNA through covalent-type linkages occurring after oxygen radical-mediated crosslinking (for example, X-irradiation) rather than through direct Ni(II) participation.77 Ni carcinogenesis may be mediated by DNA–protein crosslinks. The lesions caused by DNA–protein crosslinks are difficult to repair and the DNA sequences within these lesions are likely to be deleted (Fig. 5).78
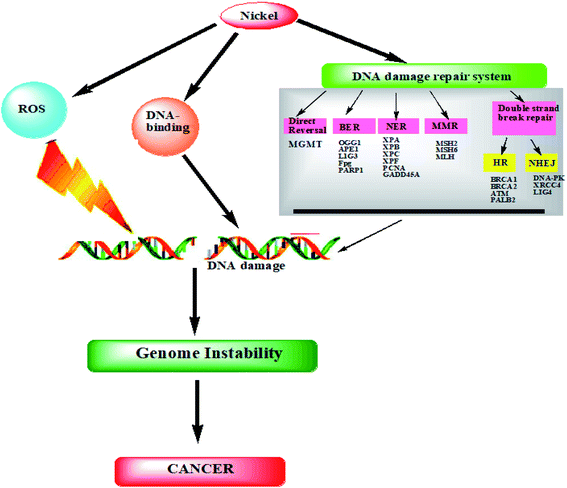 |
| Fig. 5 Nickel carcinogenesis: DNA damage (cancer). | |
10.3. Deregulation of cellular proliferation
In tumours, cell growth and differentiation are deregulated. Several epigenetic mechanisms regulate nickel-induced cell growth.77 As a result of nickel chloride's effect on methylating cytosine bases and reducing tumour suppressor gene expression, mammalian cells grew more quickly. Nickel compounds have been found to increase DNA methylation and inactivate gene expression. A possible mechanism for nickel's ability to induce hypermethylation of DNA is that it substitutes for magnesium, accelerates condensation of chromatin and initiates de novo DNA methylation.79 Furthermore, nickel has been shown to suppress histone H4 acetylation in yeast and mammalian cells in vitro, in addition to silencing genes by methylation. Nickel compounds are weakly mutagenic, despite their potency as carcinogens in humans and rodents. In order to organize the genome into active and inactive transcriptional regions, DNA methylation, as well as histone acetylation, play a key role. Nickel's effects on tumour suppressors and senescence genes are a major factor in its carcinogenicity.80
11. Conclusions
Nickel-mediated toxicity and carcinogenicity among humans and plants have been highlighted in this comprehensive review. Since nickel is ubiquitous in the environment, it is unavoidable to be exposed to harmless doses of nickel. Despite being biologically essential for plants, its importance to higher animals and especially humans are still debated. Due to phenomenal growth in consumption of nickel and nickel-containing products in contemporary times, nickel and its derivatives have become inevitable sources of environmental pollution through all stages of its production, disposal, and recycling. Various geogenic processes constantly distribute Ni between land, water, and air. In the atmosphere, Ni occurs at relatively low concentrations. Nickel released into the atmosphere mostly exists in particulate form with varying diameters depending on the source. Inhalation of workplace air may expose nickel-producing or nickel industry workers more prone to acute nickel toxicity concerning the general population. The scientific reports figured from the studies on occupationally exposed clusters indicate that the incorporation of nickel and nickel-derivatives by breathing inhalation introduces many diseases, such as sinusitis, rhinitis, anosmia, etc. The high exposure and ingestion of nickel derivatives cause breathing shortness, headache, cough, nausea, vomiting, and diarrhoea. While in its severe form it becomes fatal and causes death in both animals and humans.
In this paper, one of the major topics discussed is nickel carcinogenesis and its molecular mechanism. Nickel compounds exhibit different levels of carcinogenicity depending on their solubility in water.
In this article, we have explained several proposed cellular pathways that explain the phenomenon of nickel-induced carcinogenesis. It has been explained that nickel binding to the nucleus, as well as nickel inhibiting DNA repair pathways, can all alter gene expression in chromatin. The contentious environmental degradation, uncontrollable climate changes, and the outbreaks of diseases as pandemics are the evidence of increasing environmental degradation as well as decreasing human immunity. The devastating impact of the very recent COVID-19, a sharp increase in cases of cancer, pulmonary failure, heart health has triggered questions for the sustainable development of modern sciences. In the search for inclusive and effective strategies to meet today's demand, The UN has proposed ‘Agenda 2030’ (UN 17 SDGs). Improvised methodologies, alternative remediation processes, more application of green chemistry, and course updating existing knowledge will help us to find a way out. This extensive review thus advocates for a better and inclusive understanding of nickel, its chemistry, and toxicological profile for not only researchers but also for all those people concerned about the environment, ecology, and human health.
Author contributions
The manuscript was written and edited by Wasefa Begum, Monohar Hossain Mondal, Summi Rai, Ajaya Bhattarai, Sudip Bhattacharjee, and Bidyut Saha. It was revised several times by Monohar Hossain Mondal, Bidyut Saha and Ajaya Bhattarai.
Conflicts of interest
There are no conflicts to declare.
References
- K. K. Das, R. C. Reddy, I. B. Bagoji, S. Das, S. Bagali, L. Mullur, J. P. Khodnapur and M. S. Biradar, J. Basic Clin. Physiol. Pharmacol., 2019, 30, 141–152 CAS.
- T. Amari, T. Ghnaya and C. Abdelly, S. Afr. J. Bot., 2017, 111, 99–110 CrossRef CAS.
- Y. A. Iyaka, Sci. Res. Essays, 2011, 6, 6774–6777 Search PubMed.
- A. Muñoz and M. Costa, Toxicol. Appl. Pharmacol., 2012, 260, 1–16 CrossRef PubMed.
- F. W. Sr. Sunderman, Ann. Clin. Lab. Sci., 1990, 20, 12–21 CAS.
- R. L. Prueitt, W. Li, Y. C. Chang, P. Boffetta and J. E. Goodman, Crit. Rev. Toxicol., 2020, 50, 605–639 CrossRef CAS PubMed.
- M. Janas, A. Zawadzka and R. Cichowicz, Environ. Sci. Pollut. Res., 2018, 25, 33240–33248 CrossRef CAS PubMed.
- D. Schaumlöffel, J. Trace Elem. Med. Biol., 2012, 26, 1–6 CrossRef PubMed.
- K. S. Kasprzak, F. W. Sunderman and K. Salnikow, Mutat. Res., Fundam. Mol. Mech. Mutagen., 2003, 533, 67–97, DOI:10.1016/j.mrfmmm.2003.08.021.
- A. Tammaro, A. Narcisi, S. Persechino, C. Caperchi and A. Gaspari, Dermatitis, 2011, 22, 251–255 CrossRef CAS PubMed.
- S. Buxton, E. Garman, K. E. Heim, T. Lyons-Darden, C. E. Schlekat, M. D. Taylor and A. R. Oller, Inorganics, 2019, 7, 89, DOI:10.3390/inorganics7070089.
- G. Tewari, L. Tewari, P. C. Srivastava and B. Ram, Chem. Special. Bioavailab., 2010, 22, 141–155, DOI:10.3184/095422910X12826770835261.
- E. Denkhaus and K. Salnikow, Crit. Rev. Oncol. Hematol., 2002, 42, 35–56, DOI:10.1016/S1040-8428(01)00214-1.
- S. Kumar and A. V. Trivedi, Int. J. Curr. Microbiol. Appl. Sci., 2016, 5(3), 719–727, DOI:10.20546/ijcmas.2016.503.084.
- E. Y. Min, Y. J. Cha and J. C. Kang, Environ. Sci. Pollut. Res., 2015, 22, 13546–13555 CrossRef CAS PubMed.
- K. Kubicka, A. Samecka-Cymerman, K. Kolon, P. Kosiba and A. J. Kempers, Environ. Sci. Pollut. Res., 2015, 22, 527–534 CrossRef CAS PubMed.
- H. Guo, H. Liu, H. Wu, H. Cui, J. Fang, Z. Zuo, J. Deng, Y. Li, X. Wang and L. Zhao, Int. J. Mol. Sci., 2019, 20, 4690, DOI:10.3390/ijms20194690.
- A. Fargašova, Ecotoxicology, 2012, 21, 1476–1483 CrossRef PubMed.
- B. R. Glick, Biotechnol. Adv., 2003, 21, 383–393 CrossRef CAS PubMed.
- ICP Vegetation, Heavy metals in European mosses: Survey (2005) Monitoring manual, UNECE ICP Vegetation Coordination Centre, 2005/2006, 2006, CEH Bangor, UK Search PubMed.
- A. G. Kachenko, B. Singh and N. P. Bhatia, Aust. J. Bot., 2007, 55, 63–73 CrossRef CAS.
- UKTAG, UK Technical Advisory Group, Updated Recommendations on Environmental Standards, 2012 Search PubMed.
- M. Gardner, S. Comber, M. Scrimshaw, E. Cartmell, J. Lester and B. Ellor, Sci. Total Environ., 2012, 437, 363–372 CrossRef CAS PubMed.
- V. D. J. Keller, M. J. Whelan and H. G. Rees, A global assessment of chemical effluent dilution capacities from a macro-scale hydrological model, in Climate variability and change-hydrological impacts, ed. S. Demuth, A. Gustard, E. Planos, F. Scatena and E. Servat, International Association of Hydrological Sciences, Wallingford, UK, 2006, pp. 586–590 Search PubMed.
- J. N. Rauch and J. M. Pacyna, Global Biogeochem. Cycles, 2009, 23, 1–16 CrossRef.
- M. Costa, Toxicol. Environ. Chem., 1995, 49, 145–148 CrossRef CAS.
- G. Genchi, A. Carocci, G. Lauria, M. S. Sinicropi and A. Catalano, Int. J. Environ. Res. Public Health, 2020, 17(3), 679, DOI:10.3390/ijerph17030679.
- G. F. Calogiuri, D. Bonamonte, C. Foti and S. Al-Sowaidi, J. Allergy Ther., 2016, 7, 243, DOI:10.4172/2155-6121.1000243.
- M. Poonkothai and B. S. Vijayavathi, Int. J. Environ. Sci., 2012, 1, 285–288 Search PubMed.
- ATSDR, Toxicological Profile for Nickel, Atlanta, GA, US Public Health Service, 2005 Search PubMed.
- M. Cempel and G. Nikel, Pol. J. Environ. Stud., 2005, 15, 375–382 Search PubMed.
- Nickel and Water: Reaction Mechanisms, Environmental Impact and Health Effects, Lenntech, Netherlands, https://www.lenntech.com/periodic/water/nickel/nickel-and-water.htm#ixzz7HpwHAQWG, last accessed on 13. 01. 2022 Search PubMed.
- Central Water Commission, Ministry of Water Resources, River Development and Ganga Rejuvnation, Government of India (CWC, GOI), Status of Trace and Toxic Metals in Indian Rivers, River Data Compilation, 2, 2018 Search PubMed.
- C. Oze, C. Skinner, A. W. Schroth and R. G. Coleman, Appl. Geochem., 2008, 23, 3391–3403 CrossRef CAS.
- K. Straif, The IARC Monographs: A Review and Update on Occupational Carcinogens, vol. 100C, 2010 Search PubMed.
- V. Ilic, V. Bojanic and B. Jovic, Acta Medaca. Medianae., 2007, 46, 37–44 Search PubMed.
- M. H. Mondal, M. A. Ali, A. Pal and B. Saha, Tenside, Surfactants, Deterg., 2019, 56, 516–525 CrossRef CAS.
- D. H. Wegman and L. J. Fine, Annu. Rev. Public Health, 1990, 11, 89–103 CrossRef CAS PubMed.
- V. Göhre and U. Paszkowski, Planta, 2006, 223, 1115–1122 CrossRef PubMed.
- J. E. Goodman, R. L. Prueitt, D. G. Dodge and S. Thakali, Crit. Rev. Toxicol., 2009, 39, 365–417 CrossRef CAS PubMed.
- M. Costa and H. H. Mollenhauer, Cancer Res., 1980, 40, 2688–2694 CAS.
- A. Sigel, H. Sigel and R. K. O. Sigel, Met. Ions Life Sci., 2013, 13, 321–357, DOI:10.1007/978-94-007-7500-8_10.
- W. Bal, H. Kozłowski and S. K. Kasprzak, J. Inorg. Biochem., 2000, 79, 213–218 CrossRef CAS PubMed.
- E. C. Foulkes and D. M. McMullen, Toxicology, 1986, 38, 35–42 CrossRef CAS PubMed.
- R. Eisler, Biol. Sci. Rep., 1998, 34, 95 Search PubMed.
- A. B. Mukherjee, Environ. Rev., 1998, 6, 173–187, DOI:10.1139/er-6-3-4-173.
- D. Treissman, S. Guigard, W. Kindzierski, J. Schulz and E. Guigard, Assessment Report on Nickel For Developing Ambient Air Quality Objectives, Science and Standards Branch, WBK & Associates Inc., Alberta Environment, 2004, ISBN No. 0-7785-3951-2 (Printed Edition), 0-7785-3953-9 (On-line Edition), Web Site: https://www3.gov.ab.ca/env/info/infocentre/publist.cfm Search PubMed.
- U.S. Department of Health and Human Services, Nickel: Potential for Human Exposure. Toxicol. Profile Nickel, 2005, pp. 205–263 Search PubMed.
- P. Harasim and T. Filipek, J. Elem., 2015, 20, 525–534, DOI:10.5601/jelem.2014.19.3.651.
- M. Rajaie, N. Karimian and J. Yasrebi, Geoderma, 2008, 144, 344–351, DOI:10.1016/j.geoderma.2007.12.001.
- H. B. Xue, S. Jansen, A. Prasch and L. Sigg, Environ. Sci. Technol., 2001, 35, 539–546 CrossRef CAS PubMed.
- R. Mandal, N. M. Hassan, J. Murimboh, C. L. Chakrabarti and M. H. Back, Environ. Sci. Technol., 2002, 36, 1477–1484 CrossRef CAS PubMed.
- M. C. Yebra-Biurrun and J. M. Castro-Romero, Am. J. Anal. Chem., 2011, 2, 116–125, DOI:10.4236/ajac.2011.22013.
- V. Göhre and U. Paszkowski, Planta, 2006, 223, 1115–1122 CrossRef PubMed.
- S. G. Aschmann and R. J. Zasoski, Physiol. Plant., 1987, 71, 191–196 CrossRef CAS.
- P. C. De kock, Ann. Bot., 1956, 20, 133–141 CrossRef CAS.
- E. I. Ochiai, Bioinorganic Chemistry: An Introduction, Allyn and Bacon chemistry series, Allyn and Bacon, Boston, 1977, ISBN no.: 0205054439, 9780205054435 Search PubMed.
- A. I. Robertson and M. E. R. Meakin, J. Bot. Zimb., 1980, 12, 115–125 Search PubMed.
- I. V. Seregin and A. D. Kozhevnikova, Fiziol. Rast., 2006, 53, 285–308 Search PubMed.
- S. A. Bhalerao, A. S. Sharma and A. C. Poojari, Int. j. pure appl. Biosci., 2015, 3, 345–355 Search PubMed.
- N. Bazihizina, M. Redwan, C. Taiti, C. Giordano, E. Monetti, E. Masi, E. Azzarello and S. Mancuso, J. Plant Physiol., 2015, 174, 137–146 CrossRef CAS PubMed.
- A. Llamas, C. I. Ullrich and A. Sanz, J. Plant Biol., 2008, 46, 905–910 CAS.
- I. V. Seregin and A. D. Kozhevnikova, Russ. J. Plant Physiol., 2006, 53, 257–277 CrossRef CAS.
- E. Gajewska and M. Skłodowska, BioMetals, 2007, 20, 27–36 CrossRef CAS PubMed.
- Nickel Toxicological Overview, The Toxicology Department, CRCE, PHE, England, version 1, 2009, https://assets.publishing.service.gov.uk/government/uploads/system/uploads/attachment_data/file/337433/Nickel_Toxicological_Overview_phe_v1.pdf Search PubMed.
- F. W. Sunderman, Jr, Ann. Clin. Lab. Sci., 1997, 7, 377–398 Search PubMed.
- K. K. Das, S. N. Das and S. A. Dhundasi, Nickel: Molecular Diversity, Application, Essentiality And Toxicity In Human Health, in Biometals: Molecular Structures, Binding Properties…ed. Gaillard Blanc and Damien Moreau, Nova Science Publishers, Inc., 2009, ISBN: 978-1-60876-852-3 Search PubMed.
- J. G. Jones and C. G. Warner, Br. J. Ind. Med., 1972, 29, 168–177 Search PubMed.
- K. Das A, Al Ameen J. Med. Sci., 2009, 2, 43–50 Search PubMed.
- R. Kornik and A. K. Zug, Nickel, Dermatitis, 2008, 19, 3–8 CrossRef PubMed.
- S. Wöhrl, T. Jandl, G. Stingl and T. Kinaciyan, Contact Dermatitis, 2007, 56, 113 CrossRef PubMed.
- D. Beyersmann and A. Hartwig, Arch. Toxicol., 2008, 82, 493–512 CrossRef CAS PubMed.
- X. Huang, C. B. Klein and M. Costa, Carcinogenesis, 1994, 15, 545–548 CrossRef CAS PubMed.
- H. Dally and A. Hartwig, Carcinogenesis, 1997, 18, 1021–1026 CrossRef CAS PubMed.
- A. Hartwig, M. Asmussn, I. Ehleben, U. Herzer, D. Kostelac, A. Pelzer, A. Schwerdtle and T. Bürkle, Environ. Health Perspect., 2002, 110, 797–799 CrossRef CAS PubMed.
- M. Costa, Annu. Rev. Pharmacol. Toxicol., 1991, 31, 321–337 CrossRef CAS PubMed.
- M. Costa, Z. Zhuang, X. Huang, S. Cosentino, C. Klein and K. Salnikow, Sci. Total Environ., 1994, 148, 191–199 CrossRef CAS PubMed.
- M. Costa, J. Cell. Biochem., 1990, 44, 127–135 CrossRef CAS PubMed.
- Y. W. Lee, C. B. Klein, B. Kargacin, K. Salnikow, J. Kitahara, K. Dowjat, A. Zhitkovich, N. T. Christie and M. Costa, Mol. Cell. Biol., 1995, 15, 2547–2557 CrossRef CAS PubMed.
- F. W. Sunderman, Nickel in Disorders of Mineral Metabolism, ed. F. Bronner and J. W. Coburn, Academic Press, New York, 1981, vol. 1, pp. 201–232 Search PubMed.
|
This journal is © The Royal Society of Chemistry 2022 |
Click here to see how this site uses Cookies. View our privacy policy here.