DOI:
10.1039/D2RA00456A
(Paper)
RSC Adv., 2022,
12, 7040-7045
A solid-supported organocatalyst for asymmetric Mannich reaction to construct C2-quaternary indolin-3-ones†
Received
22nd January 2022
, Accepted 21st February 2022
First published on 2nd March 2022
Abstract
A simple and novel solid-supported organocatalyst from a 2-chlorotrityl chloride resin-immobilized 4-hydroxyproline was developed, and this organocatalyst has been used for the asymmetric Mannich reaction of 2-aryl-3H-indol-3-ones and aldehydes/ketones. A series of C2-quaternary indolin-3-ones were prepared in good yields (up to 83%) and with excellent diastereoselectivities (up to 20
:
1) and enantioselectivities (up to 99% ee). In addition, the organocatalyst can be recovered by simple filtration and also be reused for the asymmetric Mannich reaction without significant loss of catalytic efficiency.
Introduction
Organocatalysis has developed rapidly and become an important strategy in various asymmetric reactions in the past two decades, since organocatalysts are metal-free organic compounds of relatively low molecular weight and simple structure that are able to function as efficient and selective catalysts for a large variety of enantioselective transformations.1 In 2000, List et al. reported the direct asymmetric aldol reaction catalyzed by proline,2 which has become a research hotspot in the area of asymmetric catalysis because proline is a simple and cheap chiral catalyst. In this context, proline and its derivatives as powerful organocatalysts have been gradually and successfully applied to many asymmetric reactions, such as Robinson annulation,3 Mannich reactions,4 Michael reactions,5 aldol reactions, Diels–Alder reactions,6 etc. However, in order to achieve a high conversion rate within a reasonable reaction time, organocatalysis often requires up to 30 mol% as the loading amount of the catalyst.7 Moreover, it is difficult to separate the organic catalyst and the product, which is not conducive to recovery and reuse, and renders the isolation wasteful and tedious. To overcome this problem, the development of immobilized, easily recyclable, and reusable organocatalysts seems to be one of the most promising strategies. The significance of using organic catalysts will be even higher if the catalyst could be effectively solid-supported, recycled and reused. As sustainably and environmentally friendly concerns, catalyst recycling and reuse are extremely important to improve the greening process of organocatalysis. Although several solid-supported prolines have been developed for some asymmetric reactions such as aldol reactions,8 a simple and novel solid-supported proline is still highly needed to explore more asymmetric reactions.
On the other hand, C2-quaternary indolin-3-one skeletons are an important structural motif, which is widely presenting in a variety of natural products and biologically active molecules.9 Because of its importance in structure and biological activity, there has been extensively studied on the synthetic methods of these valuable C2-quaternary indolin-3-one skeletons.9e–i,10–15 Till now, some asymmetric synthesis of C2-quaternary indolin-3-ones have been developed from 2-substitued indoles15 or indolin-3-ones11–13 as substrates, including our recently developed the combinatorial catalysis of transition metal and organocatlysis to synthesize C2-quaternary indolin-3-ones from 2-substitued indoles,16 however, most of them require proline as an organocatalyst for this asymmetric Mannich reaction but none of them has been investigated in its solid-supported format to improve this asymmetric Mannich reaction yet (Scheme 1). Therefore, to construct diverse C2-quaternary indolin-3 ketone compounds, it is necessary to further develop environmentally friendly and sustainably solid-supported organocatalysts for this asymmetric Mannich reaction. Based on our previous work, we herein report a simple and cheap synthesis for preparing 2-chlorotrityl chloride resin immobilized 4-hydroxyproline as an organocatalyst, which displayed high catalytic activity and enantioselectivity in the Mannich reaction of 2-aryl-3H-indol-3-ones with aldehydes and ketones (Scheme 1). Furthermore, the solid-supported organocatalyst can be conveniently recycled and reused in a green Mannich reaction protocol.
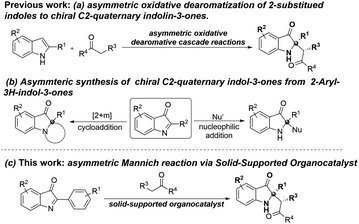 |
| Scheme 1 Strategies for enantioselective synthesis of C2-quaternary indolin-3-ones. | |
Result and discussion
Usually, a hydroxyproline or its derivative has been used as the solid-supported ligand to be immobilized onto resins by three different strategies (Fig. 1a): direct substitution using the free hydroxy group (A), or two possible combinations of azide (B) and alkyne (C) via “click chemistry”.8 However, the simplest method by direct substitution using free hydroxy group rendered the part of proline was too close to the solid phase, resulting in the adverse effect in the catalytic activity. Therefore, we have designed and synthesized four different solid-supported organocatalysts (6a, 6b, 8a and 8b) by starting from the esterification of cis-4-hydroxyl proline and trans-4-hydroxyl proline with succinic anhydride before direct substitution to 2-chlorotrityl chloride resin (Fig. 1b). The detail synthesis of these solid-supported organocatalysts were shown in ESI.† Trans-4-hydroxy proline methyl ester 2a was easily synthesized from the commercially available trans-N-Boc-4-hydroxy proline methyl ester 1a. Compound 2a was then reacted with N-(9 fluorenylmethoxycarbonyloxy)succinimide in THF at room temperature to give the compounds 3a. Then compound 3a was reacted with succinic anhydride in anhydrous CH2Cl2 at room temperature to give the compounds 4a, which was immobilized onto the 2-chlorotrityl chloride resin (f = 1.179 mmol g−1) to give solid-supported compound 5a. Solid-supported compounds 6a (f(N) = 0.193 mmol g−1) and 8a (f(N) = 0.497 mmol g−1) were obtained by removing Fmoc or removing Fmoc and methyl ester, respectively (Scheme 2). Using cis-N-Boc-4-hydroxy proline methyl ester as starting material, solid-supported compounds 6b (f(N) = 0.293 mmol g−1) and 8b (f(N) = 0.238 mmol g−1) were obtained according to the similar procedure (Scheme 2). The loading rate of these solid-supported compounds was determined by elemental analysis in ESI.†
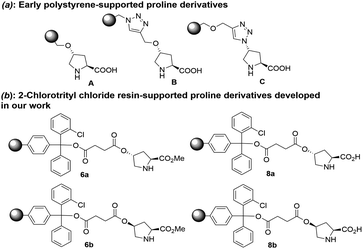 |
| Fig. 1 (a) Early polystyrene-supported proline derivatives. (b) 2-chlorotrityl chloride resin -supported proline derivatives developed in our work. | |
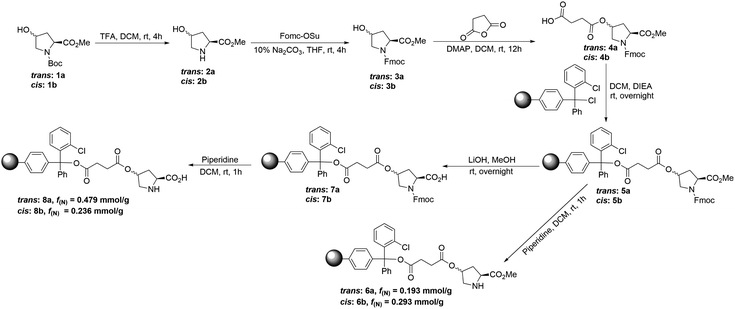 |
| Scheme 2 Synthesis of 2-chlorotrityl chloride resin-supported catalyst 6a, 6b, 8a and 8b. | |
The solid-supported organocatalysts 6a, 6b, 8a and 8b were tested in the Mannich reaction of 2-aryl-3H-indol-3-ones 9a and phenylpropionaldehyde 10a and followed by reduction of an aldehyde to give the desired product 11a (Table 1, entries 1–4). In presence of these solid-supported catalysts, all reactions gave good yields, and the solid-supported organocatalyst 8b proved to be the best catalyst with 78% yield, 1
:
10.1 dr (syn
:
anti) and 93% ee for the desired product 11a (syn). Although catalyst 8a led to similar yield, diastereoselectivity and ee value for 11a, catalyst 8b could give a higher ee value for the diastereomer of 11a′ (Table 1, entry 4 vs. entry 2). Therefore, we chose catalyst 8b to test different solvents to improve the diastereomer selectivity of desired product 11a (syn) under the above conditions (Table 1, entries 5–12). Some common solvents such as DMSO, dioxane, toluene, DMF, MeCN, EtOAc, CHCl3, CH2Cl2 and THF were used, and DMF was found to be the optimal choice and the desired product 11a was also obtained with 66% yield and 95% ee and the syn/anti ratio was increased to 1
:
1.7. Encouraged by these results, we tested different temperatures to further increase the diastereomer selectivity of desired product 11a (syn) (Table 1, entries 13–14). Experimental results show that the optimal temperature was 0 °C and the desired product 11a was obtained with up to 81% yield and 98% ee; and the syn/anti ratio was further increased to 6.6
:
1 (Table 1, entry 13). Finally, the amount of catalyst was also investigated (Table 1, entries 15–16), and the optimal conditions were achieved as: 9a (1.0 equiv.), 10a (2.0 equiv.), solid-supported organocatalyst 8b (10 mol%) and DMF as a solvent at 0 °C. Under the optimal conditions, the desired product 11a (syn) was obtained with up to 79% yield, 6.3
:
1 dr (syn
:
anti) and 98% ee (Table 1, entry 16).
Table 1 Optimization of reaction conditionsa
With the optimal reaction conditions in hand, we then investigated the reactions of a variety of 2-aryl-3H-indol-3-ones 9 and aldehydes 10 catalyzed by the solid-supported organocatalyst 8b in DMF at 0 °C (Table 2 and 3). In general, the desired C2-quaternary indolin-3-one products 11a–11o and 12a–12d were obtained with up to 83% yields, >20
:
1 dr and 99% ee, the absolute configuration and structure of 12d was unambiguously confirmed by single-crystal X-ray crystallography (Table 3).16 The reactions of 9a with aldehydes proceeded smoothly, affording the corresponding 2,2-disubstituted indolin-3-ones (11a–11e) in 48–82% yield with 6.3
:
1–11
:
1 dr and 77–98% ee. However, the slightly lower yield and poor ee was obtained for products 11e may be due to the steric interaction of isobutyraldehyde. We next investigated the effect of different R1 or R2 substituent of 2-aryl-3H-indol-3-ones 9 on this reaction. 2-aryl-3H-indol-3-ones 9 with electron-donating and withdrawing substituents on the aromatic ring of indole were employed to give indolin-3-ones (11f–11l) in moderate to good yields (61–83%) with moderate diastereo- (3
:
1–7.3
:
1 dr) and moderate to good enantio- (51–87% ee) selectivities. Compared with electron donating groups (11i–11j), substrates with halide groups (11f–11h) have slightly higher yield and enantioselectivity. Different R2 substituents (Cl or Me) on the aromatic ring can give 11m–11o with 38–63% yield, 2
:
1–5
:
1 dr and 82–89% ee. The electronic effects of the R2 substituents may have some impact on the experimental results since the yield of the substrate with an electron donating group is higher than that of the one with electron withdrawing group (11m vs. 11n). Encouraged by the results of aldehydes, we also investigated different ketones as substrates on this Mannich reaction. Gratifyingly, the reaction of 2-aryl-3H-indol-3-ones 9 and ketones 10 catalyzed by the solid-supported organocatalyst 8b in DMF at 0 °C also can give the desired compounds 12a–12d in yields 46–64% yield with >20
:
1 dr and 69–99% ee (Table 3). These results indicated that our solid-supported organocatalyst 8b was suitable for the application in this asymmetric Mannich reaction to construct C2-quaternary indolin-3-ones.
Table 2 Scope of aldehydes and 2-aryl-3H-indol-3-onesa
Unless otherwise specified, the reactions were carried out with 9 (0.10 mmol), 10 (0.20 mmol), 8b (10 mol%) in DMF (1.0 mL) at 0 °C. Then the aldehyde was reduced by NaBH4 was needed to give compounds 11. Combined yields of all diastereomers after flash column chromatography. The dr values were determined by 1H NMR analysis of the crude products. The ee values were determined by chiral HPLC. |
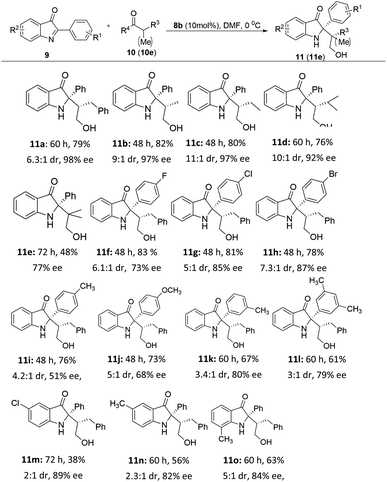 |
Table 3 Scope of ketonesa
Unless otherwise specified, the reactions were carried out with 9 (0.10 mmol), 10 (0.20 mmol), 8b (10 mol%) in DMF (1.0 mL) at 0 °C. Combined yields of all diastereomers after flash column chromatography. The dr values were determined by 1H NMR analysis of the crude products. The ee values were determined by chiral HPLC. |
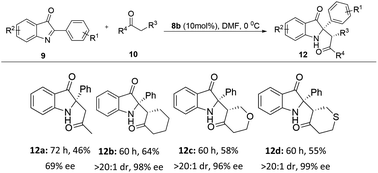 |
To further investigate the potential application of the asymmetric Mannich reaction, the large-scale synthesis of chiral C2-quaternary indolin-3-ones 11a was performed. Under the optimized conditions, the gram scale synthesis of chiral product 11a can be obtained in 68% yield, 4.3
:
1 dr and 99% ee (Scheme 3). In the four cycles, the recovery rate was between 92–95%. More importantly, the ee value for each cycle rate was between 92–95%. More importantly, the ee value for each cycle was consistent as ∼98% ee although the yield and dr value was slightly dropped (Table 4).
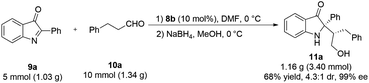 |
| Scheme 3 The large-scale synthesis of product 11a. | |
Table 4 Recycling and reusinga
Conclusions
In summary, we have developed a simple synthesis method for preparing 2-chlorotrityl chloride resin-immobilized hydroxyproline catalyst, which has been used for the reaction of 2-aryl-3H-indol-3-ones with aldehydes or ketones asymmetric Mannich reaction. A series of chiral C2-quaternary indolin-3-ones were obtained via this strategy. In addition, the catalyst can be recovered by simple filtration, and it can also be reused at least 3 times without significant loss of catalytic efficiency. To the best of our knowledge, this is the first application of solid-supported catalyst synthesized from 2-chlorotrityl chloride resin and 4-hydroxyl proline for the construction of C2-quaternary indolin-3-one skeleton.
Conflicts of interest
There are no conflicts to declare.
Acknowledgements
We thank the following for the financial support to this work: National Natural Science Foundation of China (No. 81960628, U1812403-05 and 82160655), Guizhou Science and Technology Department of China (No. [2020]1Y395 and [2020]4Y206), Guizhou Medical University (No. J[2021]047).
Notes and references
-
(a) G. Guillena, C. Najera and D. J. Ramon, Tetrahedron: Asymmetry, 2007, 18, 2249 CrossRef CAS;
(b) H. Pellissier, Tetrahedron, 2007, 63, 9267 CrossRef CAS;
(c) G. Guillena and D. J. Ramon, Tetrahedron: Asymmetry, 2006, 17, 1465 CrossRef CAS;
(d) P. I. Dalko and L. Moisan, Angew. Chem., Int. Ed., 2004, 43, 5138 CrossRef CAS PubMed;
(e) B. List, Chem. Rev., 2007, 107, 5413 CrossRef CAS;
(f) R. O. Duthaler, Angew. Chem., Int. Ed., 2003, 42, 975 CrossRef CAS PubMed;
(g) E. R. Jarvo and S. J. Miller, Tetrahedron, 2002, 58, 2481 CrossRef CAS.
- B. List, R. A. Lerner and C. F. Barbas III, J. Am. Chem. Soc., 2000, 122, 2395 CrossRef CAS.
-
(a) U. Eder, G. Sauer and R. Wiechert, Angew. Chem., Int. Ed. Engl., 1971, 10, 496 CrossRef CAS;
(b) Z. G. Hajos and D. R. Parrish, J. Org. Chem., 1974, 39, 161 Search PubMed;
(c) T. Bui and F. Barbas III, Tetrahedron Lett., 2000, 41, 6951 CrossRef CAS.
-
(a) B. List, J. Am. Chem. Soc., 2000, 122, 9336 CrossRef CAS;
(b) A. Cordova, W. Notz, G. Zhong, J. M. Betancort and C. F. Barbas III, J. Am. Chem. Soc., 2002, 124, 1842 CrossRef CAS PubMed;
(c) B. List, P. Pojarliev, W. T. Biller and H. J. Martin, J. Am. Chem. Soc., 2002, 124, 827 CrossRef CAS PubMed;
(d) W. Notz, F. Tanaka, S. Watanabe, N. S. Chowdari, J. M. Turner, R. Thayumanavan and C. F. Barbas III, J. Org. Chem., 2003, 68, 9624 CrossRef CAS PubMed;
(e) N. S. Chowdari, J. T. Suri and C. F. Barbas III, Org. Lett., 2004, 6, 2507 CrossRef CAS PubMed;
(f) Y. Hayashi, W. Tsuboi, I. Ashimine, T. Urushima, M. Shoji and K. Sakai, Angew. Chem., Int. Ed., 2003, 42, 3677 CrossRef CAS PubMed;
(g) N. S. Chowdari, D. B. Ramachary and C. F. Barbas III, Synlett, 2003, 12, 1906 Search PubMed.
-
(a) B. List, P. Pojarliev and H. J. Martin, Org. Lett., 2001, 3, 2423 CrossRef CAS PubMed;
(b) J. M. Betancort and C. F. Barbas III, Org. Lett., 2001, 3, 3737 CrossRef CAS PubMed;
(c) D. Enders and A. Seki, Synlett, 2002, 1, 26 CrossRef;
(d) D. Gryko, Tetrahedron: Asymmetry, 2005, 16, 1377 CrossRef CAS;
(e) I. K. Mangion and D. W. C. MacMillan, J. Am. Chem. Soc., 2005, 127, 3696 CrossRef CAS PubMed.
-
(a) G. Sabitha, N. Fatima, E. V. Reddy and J. S. Yadav, Adv. Synth. Catal., 2005, 347, 1353 CrossRef CAS;
(b) R. Thayumanavan, B. Dhevalapally, K. Sakthivel, F. Tanaka and C. F. Barbas III, Tetrahedron Lett., 2002, 43, 3817 CrossRef CAS;
(c) D. B. Ramachary, N. S. Chowdari and C. F. Barbas III, Tetrahedron Lett., 2002, 43, 6743 CrossRef CAS.
- R. Martin-Rapffln, X. Fan, S. Sayalero, M. Bahramnejad, F. Cuevas and M. A. Pericas, Eur. J. Chem., 2011, 17, 8780 CrossRef PubMed.
-
(a) M. Benaglia, A. Puglisi and F. Cozzi, Chem. Rev., 2003, 103, 3401 CrossRef CAS PubMed;
(b) F. Cozzi, Adv. Synth. Catal., 2006, 348, 1367 CrossRef CAS;
(c) M. Gruttadauria, F. Giacalone and R. Noto, Chem. Soc. Rev., 2008, 37, 1666 RSC;
(d) M. Benaglia, New J. Chem., 2006, 30, 1525 RSC;
(e) E. Alza, C. Rodríguez-Escrich, S. Sayalero, A. Bastero and M. A. Pericàs, Eur. J. Chem., 2009, 15, 10167 CrossRef CAS PubMed;
(f) C. Rodríguez-Escrich and M. A. Pericàs, Chem. Rec., 2019, 19(9), 1872 CrossRef PubMed.
-
(a) W. Wu, M. Xiao, J. Wang, Y. Li and Z. Xie, Org. Lett., 2012, 14, 1624 CrossRef CAS PubMed;
(b) D. S. Bhakuni, M. Silvan, S. A. Matlin and P. G. Sammes, Phytochemistry, 1976, 15, 574 CrossRef CAS;
(c) D. Stoermer and C. H. Heathcock, J. Org. Chem., 1993, 58, 564 CrossRef CAS;
(d) P. S. Baran and E. J. Corey, J. Am. Chem. Soc., 2002, 124, 7904 CrossRef CAS PubMed;
(e) C. Shu, L. Li, X.-Y. Xiao, Y.-F. Yu, Y.-F. Ping, J.-M. Zhou and L.-W. Ye, Chem. Commun., 2014, 50, 8689 RSC;
(f) Y.-Q. Zhang, D.-Y. Zhu, Z.-W. Jiao, B.-S. Li, F.-M. Zhang, Y.-Q. Tu and Z. Bi, Org. Lett., 2011, 13, 3458 CrossRef CAS PubMed;
(g) Y. Goriya and C. V. Ramana, Chem. Commun., 2013, 49, 6376 RSC;
(h) Z. Xia, J. Hu, Y.-Q. Gao, Q. Yao and W. Xie, Chem. Commun., 2017, 53, 7485 RSC;
(i) Y.-J. Li, N. Yan, C.-H. Liu, Y. Yu and Y.-L. Zhao, Org. Lett., 2017, 19, 1160 CrossRef CAS PubMed.
- R. O. Torres-Ochoa, T. Buyck, Q. Wang and J. P. Zhu, Angew. Chem., Int. Ed., 2018, 57, 5679 CrossRef CAS PubMed.
- R.-R. Liu, S.-C. Ye, C.-J. Lu, G.-L. Zhuang, J.-R. Gao and Y.-X. Jia, Angew. Chem., Int. Ed., 2015, 54, 11205 CrossRef CAS PubMed.
-
(a) Q. Ni, X. Song, G. Raabe and D. N. Enders, Chem. - Asian J., 2014, 9, 1535 CrossRef CAS PubMed;
(b) J. Guo, A.-H. Lin, K.-B. Chen, Y. Xie, A. S. C. Chan, J. Weng and G. Lu, Org. Chem. Front., 2017, 4, 1400 RSC;
(c) S. Yarlagadda, B. Ramesh, C. Ravikumar Reddy, L. Srinivas, B. Sridhar and B. V. Subba Reddy, Org. Lett., 2017, 19, 170 CrossRef CAS PubMed;
(d) L.-J. Zhang, Y. Wang, X.-Q. Hu and P.-F. Xu, Chem.–Asian J., 2016, 11, 834 CrossRef CAS PubMed;
(e) S. Yarlagadda, G. S. Sankaram, S. Balasubramanian and B. V. Subba Reddy, Org. Lett., 2018, 20, 4195 CrossRef CAS PubMed;
(f) R. Dalpozzo, Adv. Synth. Catal., 2017, 359, 1772 CrossRef CAS;
(g) H. Kazahiro, M. Kouhei, K. Tamami, H. Masahiro, S. Masanori and K. Tomomi, Heterocyles, 2007, 73, 641 CrossRef;
(h) C. Y. Jin, Y. Wang, Y. Z. Liu, C. Shen and P. F. Xu, J. Org. Chem., 2012, 77, 11307 CrossRef CAS PubMed;
(i) T. G. Chen, P. Fang, X. L. Hou and L. X. Dai, Synthesis, 2015, 47, 134 CrossRef CAS;
(j) V. P. R. Gajulapallia, E. Jafaria, D. S. Kundua, S. Mahajana, A. Peuronenb, K. Rissanenb and D. Enders, Synthesis, 2017, 49, 4986 CrossRef;
(k) C. Guo, M. Schedler, C.-G. Daniliuc and F. Glorius, Angew. Chem., Int. Ed., 2014, 53, 10232 CrossRef CAS PubMed;
(l) S. Yarlagadda, B. Sridhar and B.-V. Subba Reddy, Chem. - Asian J., 2018, 13, 1327 CrossRef CAS PubMed;
(m) S. Yarlagadda, C.-R. Reddy, B. Ramesh, G. Ravi kumar, B. Sridhar and B.-V.-S. Reddy, Eur. J. Org. Chem., 2018, 2018, 1364 CrossRef CAS;
(n) Y.-L. Zhao, W. Yao, J. Cao, Y.-M. Liang and P.-F. Xu, Org. Lett., 2014, 9, 2438 CrossRef PubMed;
(o) Y.-L. Zhao, X.-H. Fei, Y.-Q. Tang, P.-F. Xu, F.-F. Yang, B. He, X.-Z. Fu, Y.-Y. Yang, M. Zhou, Y.-H. Mao, Y.-X. Dong and C. Li, J. Org. Chem., 2019, 84, 8168 CrossRef CAS PubMed.
-
(a) L. Li, M. Han, M. Xiao and Z. Xie, Synlett, 2011, 12, 1727 Search PubMed;
(b) Q. Yin and S.-L. You, Chem. Sci., 2011, 2, 1344 RSC;
(c) A. Parra, R. Alfaro, L. Marzo, A. Moreno-Carrasco, J. L. GarcíaRuano and J. Aleman, Chem. Commun., 2012, 48, 9759 RSC;
(d) J.-X. Liu, Q.-Q. Zhou, J.-G. Deng and Y.-C. Chen, Org. Biomol. Chem., 2013, 11, 8175 RSC;
(e) S. Nakamura, N. Matsuda and M. Ohara, Eur. J. Chem., 2016, 22, 9478 CrossRef CAS PubMed;
(f) J.-S. Li, Y.-J. Liu, G.-W. Zhang and J.-A. Ma, Org. Lett., 2017, 19, 6364 CrossRef CAS PubMed;
(g) J.-S. Li, Y.-J. Liu, S. Li and J.-A. Ma, Chem. Commun., 2018, 54, 9151 RSC;
(h) S.-S. Fang, S.-Y. Jin, R. Ma, T. Lu and D. Du, Org. Lett., 2019, 21, 5211 CrossRef CAS PubMed;
(i) M. Rueping, S. Raja and A. Nunez, Adv. Synth. Catal., 2011, 353, 563 CrossRef CAS.
-
(a) C. I. Altinis Kiraz, T. J. Emge and L. S. Jimenez, J. Org. Chem., 2004, 69, 2200 CrossRef CAS PubMed;
(b) F. Lin, Y. Chen, B. Wang, W. Qin and L. Liu, RSC Adv., 2015, 5, 37018 RSC;
(c) C. Zhang, S. Li, F. Bures, R. Lee, X. Ye and Z. Jiang, ACS Catal., 2016, 6, 6853 CrossRef CAS;
(d) Z. Deng, X. Peng, P. Huang, L. Jiang, D. Ye and L. Liu, Org. Biomol. Chem., 2017, 15, 442 RSC;
(e) Y. Kong, J. Zhu, Z. Chen and L. Liu, Can. J. Chem., 2014, 92, 269 CrossRef CAS;
(f) H. Huang, J. Cai, X. Ji, F. Xiao, Y. Chen and G. Deng, Angew. Chem., Int. Ed., 2016, 55, 307 CrossRef CAS PubMed;
(g) L. Kong, M. Wang, F. Zhang, M. Xu and Y. Li, Org. Lett., 2016, 18, 6124 CrossRef CAS PubMed;
(h) X. Jiang, B. Zhu, K. Lin, G. Wang, W. Su and C. Yu, Org. Biomol. Chem., 2019, 17, 2199 RSC;
(i) M. J. Buller, T. G. Cook and Y. Kobayashi, Heterocycles, 2007, 72, 163 CrossRef CAS;
(j) W. Ding, Q.-Q. Zhou, J. Xuan, T.-R. Li, L.-Q. Lu and W.-J. Xiao, Tetrahedron Lett., 2014, 55, 4648 CrossRef CAS.
-
(a) X. Ding, C.-L. Dong, Z. Guan and Y.-H. He, Angew. Chem., Int. Ed., 2019, 58, 118 CrossRef CAS PubMed;
(b) X. Liu, X. Yan, J.-H. Yan, Y.-D. Tang, K. Wang and H. Zhang, Org. Lett., 2019, 21, 5626 CrossRef CAS PubMed;
(c) F.-Y. Lu, Y.-J. Chen, Y. Chen, X. Ding, Z. Guan and Y.-H. He, Chem. Commun., 2020, 56, 623 RSC;
(d) C.-L. Dong, X. Ding, L.-Q. Huang, Y.-H. He and Z. Guan, Org. Lett., 2020, 22, 1076 CrossRef CAS PubMed;
(e) L. Bu, J. Li, Y. Yin, B. Qiao, G. Chai, X. Zhao and Z. Jiang, Chem.–Asian J., 2018, 13, 2382 CrossRef CAS PubMed;
(f) S. Lerch, L.-N. Unkel and M. Brasholz, Angew. Chem., Int. Ed., 2014, 53, 6558 CrossRef CAS PubMed.
- Y.-L. Zhao, J.-X. An, F.-F. Yang, X. Guan, X.-Z. Fu, Z.-Q. Li, D.-P. Wang, M. Zhou, Y.-Y. Yang and B. He, Adv. Synth. Catal., 2022 DOI:10.1002/adsc.202101498.
Footnote |
† Electronic supplementary information (ESI) available. See DOI: 10.1039/d2ra00456a |
|
This journal is © The Royal Society of Chemistry 2022 |
Click here to see how this site uses Cookies. View our privacy policy here.