DOI:
10.1039/D2RA00868H
(Paper)
RSC Adv., 2022,
12, 8405-8413
MWCNT-modified MXene as cost-effective efficient bifunctional catalyst for overall water splitting
Received
10th February 2022
, Accepted 8th March 2022
First published on 16th March 2022
Abstract
Utilization of cost-effective, bifunctional, and efficient electrocatalysts for complete water splitting is desirable for sustainable clean hydrogen energy. In last decade, MXenes, a family of emerging two-dimensional (2D) materials with unique physiochemical properties, enticed scientists because of their use in different applications. However, insufficient electron transport, lower intrinsic chemical activity and limited active site densities are the factors inhibiting their use in electrocatalytic cells for hydrogen production. Here, we have presented material design to address this issue and introduced carbon nanotubes (CNTs) on V2CTx MXene sheets for conductive network channels that enhance the ion diffusion for enhanced electrochemical activity. The SEM reveals the uniform dispersion of the MWCNTs over the MXene surface that resulted in the formation of conductive network channels and enhances reaction kinetics. The as-synthesized electrocatalyst was subjected to linear sweep voltammetry (LSV) measurements for hydrogen evolution reaction (HER) and oxygen evolution reaction (OER). The hybrid catalyst M2 exhibited an enhanced HER activity with a lower over-potential of 27 mV which is comparable to commercially available Pt-based catalysts (32 mV). Similarly, an enhanced OER activity was observed with a lower over-potential of 469 mV as compared to pristine V2CTx MXene. The electrocatalyst was subjected to a durability test through chronoamperometry and was observed to be stable for 16 hours. Hence, this study opens a new avenue for future cost-effective efficient catalysts for overall water splitting as a solution to produce clean hydrogen.
Introduction
Global warming, increased carbon emission causing environmental hazards and shortage of renewable energy resources result in an ever growing demand for green energy production and conversion. Hydrogen fuel is considered to be the most green energy carrier to address the energy crises and environmental issues.1–3 Currently, hydrogen fuel is produced by the steam process via burning of fossil fuels. However, during this process, CO2 is produced causing environmental pollution.4–9 Electrochemical water splitting is considered as an advanced clean energy technology for hydrogen production.10,11 Hydrogen evolution reaction (HER) at the cathode and the oxygen evolution reaction (OER) at the anode are the two key reactions proceeding in an electrocatalytic cell for the generation of hydrogen (H2) and oxygen (O2), respectively. Precious metals (Pt, Ir, Ru) or metal oxides (Ru2O, IrO) are considered as efficient catalysts for electrochemical water splitting but their high cost and unavailability limit their commercialization and industrial use.12–14 Moreover, it is very difficult to achieve high HER and OER performance simultaneously using a single precious metal hence, there is a need to develop a cost effective, non-precious metal, bifunctional, durable catalyst for overall water splitting. In the past, transition metal chalcogenides (TMDC),15,16 oxides,17–21 phosphides,22–27 nitrides,28–31 oxy hydroxides,32,33 carbides13,34 and metal free hybrids35,36 have been thoroughly investigated for low-cost and effective electrocatalytic activity.
In recent years, a new family of 2D early transition metal carbides and nitrides known as MXenes have attracted much attention for their unique physical, optical, electronic, optoelectronic, thermal and magnetic properties.37 MXenes have general formula of MnXn+1Tx where M is early transition metal, X is carbon or nitrogen and Tx are the surface terminations like –OH, –F, –Cl or –O. This rich surface chemistry and high surface area enabled them as potential candidates for energy storage and conversion systems. MXenes are synthesized via wet chemical etching of A layer with hydrofluoric acid or salt soln. containing fluorine from the MAX phases. Where A is either Al or Si an element of group III A or IV A. M is early transition metal and X is carbon or nitrogen.38 MXenes have been widely explored for their use in lithium-ion-battery (LIBS)39 and supercapacitors.40–42 Due to the active surface functionality and chemical inertness in electrochemical potential, MXene is predicted to be a heterogeneous catalyst.43,44 However, very limited literature is available for the catalytic behavior of MXene. Only a few MXenes (Ti3C2Tx, Mo2TiC2Tx, Nb2CTx, V4C3Tx, Mo2CTx) out of the largest known 2D family have been explored for catalytic activities.45–51 Contrary to the theoretical calculation,43 experimental studies reveal that Ti3C2Tx and V4C3Tx have poor HER performance with an over-potential of 600 mV (ref. 46 and 49) than Mo-based MXene (Mo2CTx).46 Therefore, many research groups are attempting to improve the catalytic performance of MXene by modification of terminal groups so that it can be used as bifunctional catalyst for overall (for both HER and OER activities) water splitting. Due to high H-binding strength (high Gibbs free energy) of oxygen, vulnerable vanadium based MXene shows a poor HER performance.47,52 So, the use of V2CTx as an efficient catalyst is still a challenge.
Previous research shows that the introduction of the CNTs can effectively improve the conductivity of the Ti3C2Tx.57,62–67 Their unique hollow geometry offers a high specific area that makes them suitable support for heterogeneous catalysis. One more important feature of carbon nanotube is relatively high oxidation stability due to their chemically inert nature.67
In this study, a facile method was adopted for the modification of V2CTx MXene by introducing MWCNT for its effective use as an electrochemical catalyst. The MWCNT forms a uniform networking over the surface of the MXene sheets preventing oxidation as well as are intercalated between them that enhances the electrical conductivity and provides more active sites for the ion diffusion hence, resulting in an increased electrocatalytic activity. The modified MXene not only exhibits excellent HER activity comparable to precious metal industrial catalysts but also presented an enhanced OER hence, showed bifunctional catalyst for the overall water splitting.
Experimental section
Synthesis of V2CTx MXene and MWCNT@V2CTx MXene hybrid
Fig. 1 represents the schematic for the synthesis of MXene treating MAX and MWCNT decorated MXene. The V2CTx MXene was synthesized from the V2AlC MAX phase by wet chemical etching process. Typically, 1 g of MAX powder was added to the 15 ml of 50% Hydrofluoric Acid (HF) and kept on stirring at 200 rpm in a Teflon lined bottle for 90 hours at room-temperature. Then the mixture was centrifuged at 3500 rpm/5 min and washed several times with DI water until the PH reaches up to 6 followed by vacuum filtration to obtain multilayer (ML) V2CTx.
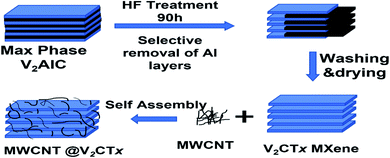 |
| Fig. 1 Systematic synthesis of MXene and MWCNT decorated V2CTx MXene. | |
Co-precipitation route was opted for the synthesis of MWCNT/MXene hybrid. Cetyl Trimethyl Ammonium Bromide (CTAB) grafted MWCNT was prepared by dissolving 2 mg of CTAB in 20 ml DI water via magnetic stirring to achieve a uniform aqueous solution. After that, MWCNTs were dispersed in CTAB solution via probe sonication to a concentration of 0.5 mg ml−1. Briefly, 20 ml of CTAB grafted MWCNT solution was added dropwise to 50 ml (0.4 mg ml−1) of V2CTx solution. The resultant mixture was probe sonicated for 10 minutes followed by vacuum filtration and vacuum drying at 50 °C overnight to get a hybrid material. In a similar fashion, four ratios 1
:
1, 1
:
2, 0.5
:
2, and 0.25
:
2 of MWCNT
:
MXene samples were prepared labelled as M1, M2, M3, and M4.
Material characterization
The crystal structure and phase identification were carried out using powder X-ray diffraction (DRON-8) diffractometer equipped with Cu K-α (λ = 0.154 nm) source in the 2θ range of 3–70°. VEGA3/TESCAN 51-ADD007 scanning electron microscope (SEM) was used for the study of surface morphology. Transmission electron microscopy (TEM) analysis was carried out to further investigate the morphology. The high-resolution images were acquired on Titan 60-300 from Thermo Fischer Scientific equipped with an imaging Cs-corrector and working at 300 kV.
Electrochemical measurements. Gamry 1010B potentiostat workstation was used for the electrochemical testing in a three-electrode configuration using Pt wire as counter electrode and Ag/AgCl as a reference electrode in 3.5 M KCl. The working electrode was fabricated on Ni foam by dispersing 10 mg of as-synthesized MWCNT@V2CTx hybrid catalyst in 500 ml DI water, 450 ml isopropyl alcohol and 50 ml Nafion (wt 5%) by ultrasonication for 35 min. Then 100 ml ink was spread on cleaned NF (1 cm × 1 cm) surface with a loading of 2–3 mg cm−2. The prepared electrodes were overnight vacuum-dried at room-temperature. The dried electrodes were pressed under 5–10 MPa pressure for 10 seconds. Linear Sweep Voltammetry (LSV) towards HER and OER activity was performed at constant scan rate of 10 mV s−1 in 1 molar KOH. All the measured potentials against Ag/AgCl were converted to RHE based on the formula of Nernst equation: ERHE = EAg/AgCl + 0.059pH + 0.1976. The CV voltammograms were recorded in the voltage range of −0.4 to 0.7 V under a scan rate range of 2 mV s−1 to 200 mV s−1. The EIS was acquired at open circuit potential (OCP) in a frequency range of 20 kHz to 10 MHz using a sinusoidal signal of 10 mV. The long term durability was tested through chronoamperometry at 0.6 V.
Results and discussions
Analysis of crystallographic structure of V2CTx MXene and MWCNTs/V2CTx MXene hybrid are shown in Fig. 2a and b, respectively. The most intense diffraction peak at 2θ = 41.3° is reduced after the HF treatment confirming the successful removal of Al layers. In addition, the shifting and broadening of (002) peak of MAX at 2θ = 13.4°to 2θ = 8.9° indicates the high c-axis orientation and an increased interlayer spacing (JCPDS no. 29-0101).53 It is obvious that the two significant peaks of MWCNTs at 2θ = 25.3° and 2θ = 42.6° (ref. 54 and 55) and the significant diffraction peaks of MXene remain intact during the hybrid fabrication, indicating its successful fabrication. The (002) plane of V2CTx MXene further shifts towards a lower angle of 2θ = 8.24° with introduction of the carbon nanotubes. To investigate whether the MWCNT were successfully grafted on the V2CTx MXene, the scanning electron microscopy (SEM) was carried out. Fig. 3a shows the SEM of MAX phase revealing the multilayer crystalline structure. While in Fig. 3b, the layers are separated forming an accordion-like structure that given an evidence of selective etching of Al after the acidic treatment.56 The MWCNTs forms a uniform network over the MXene sheets as can be observed from Fig. 3d; the inset represents a closer look of networking.57
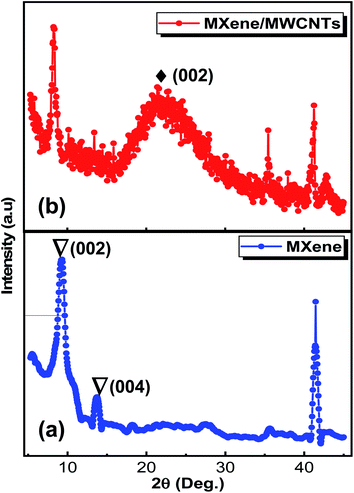 |
| Fig. 2 XRD patterns of (a) V2CTx MXene (b) MWCNT decorated V2CTx MXene. | |
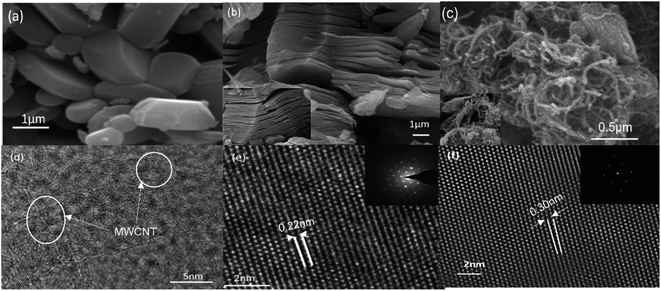 |
| Fig. 3 SEM images of (a) MAX phase (b) multilayer V2CTx MXene (c) MWCNT@V2CTx MXene. TEM images of (d) MWCNT (e) MXene (f) MWCNT@V2CTx MXene. | |
The high-resolution transmission electron microscopy (HRTEM) is in good agreement of SEM micrographs revealing the separated basal planes of MXene (Fig. 3e). The sheets are more separated out confirming the intercalation of MWCNTs in hybrid structure (Fig. 3f). The selective area electron diffraction (SAED) (inset of Fig. 3e and f) reveals that the basal planes maintained the primitive hexagonal structure of parent MAX phase after the acidic treatment as well the hybrid formation.
Electrochemical measurements
The electrocatalytic performance was estimated via HER and OER studies in 1 molar KOH using 3-electrode system. Where the as-synthesized pristine V2CTx and MWCNT@V2CTx catalysts were used as working electrode, platinum wire and Ag/AgCl were used as counter and reference electrode, respectively. To minimize the interference of the capacitive current iR, corrected linear sweep voltammetry was acquired at a low scan rate of 10 mV s−1.
OER activity
Fig. 4a represents the polarization curves for OER catalytic activity of V2CTx MXene and MWCNT@V2CTx hybrid. The hybrid material possesses lower onset potential and over-potential as compared to the pristine MXene at a current density of 10 mA cm−2 (η10). From Fig. 4c, it can be observed that the over-potentials for M1, M2, M3 and M4 are 560 mV, 469 mV, 562 mV and 570 mV, respectively are lower than the pristine MXene (652 mV @ η10). The higher OER activity of the hybrid is attributed to the enhanced surface area of carbon nanotubes along with the formation of uniform conductive channels for the fast ion transportation through electrode/electrolyte interface, resulting in an improved electrochemical performance. With the increasing concentration of MWCNTs, the OER activity decreases which could be attributed to the overloading and blocking of active sites. Furthermore, the Tafel plot, log(|j|) versus over-potential (η) was employed to verify the electrocatalytic kinetics of OER. The small Tafel slope (77 mV dec−1) of MWCNT@V2CTx hybrid sample M2 is lower than the pristine MXene (116 mV dec−1) representing the synergetic effects between V2CTx MXene and MWCNT (Fig. 4b).68 The 103 mV dec−1, 92 mV dec−1, and 75 mV dec−1 are the Tafel slope values for M1, M3 and M4, respectively.
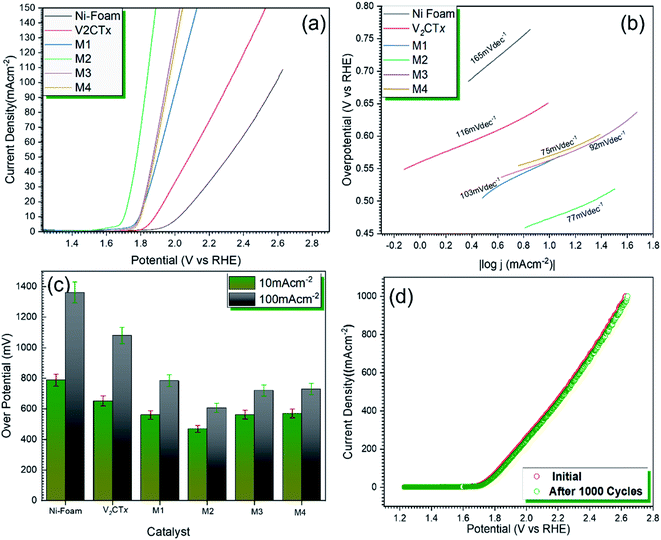 |
| Fig. 4 (a) OER polarization curves against RHE (b) Tafel slopes of pristine MXene and MWCNT decorated V2CTx MXene (c) comparison of overpotential @ η10 and η100 mA cm−2 (d) durability test of MWCNT decorated MXene for 1000 CV cycles. | |
The durability of the electrode is a key parameter to evaluate the performance of electrocatalyst. The catalyst was subjected to continuous cyclic voltammetry for stability test and the polarization curves were plotted after 1000 CV cycles. There was no significant change in the initial and final over-potentials after 1000 cycles confirming high durability of hybrid catalyst.
HER activity
Fig. 5a represents the LSV polarization curves for HER activity of pristine V2CTx MXene and MWCNT@V2CTx. It can be observed from the graphs that V2CTx shows an average HER activity with an over-potential of 77 mV while the hybrid posses 27 mV that is comparable to commercial Pt catalysis i.e., 32 mV.
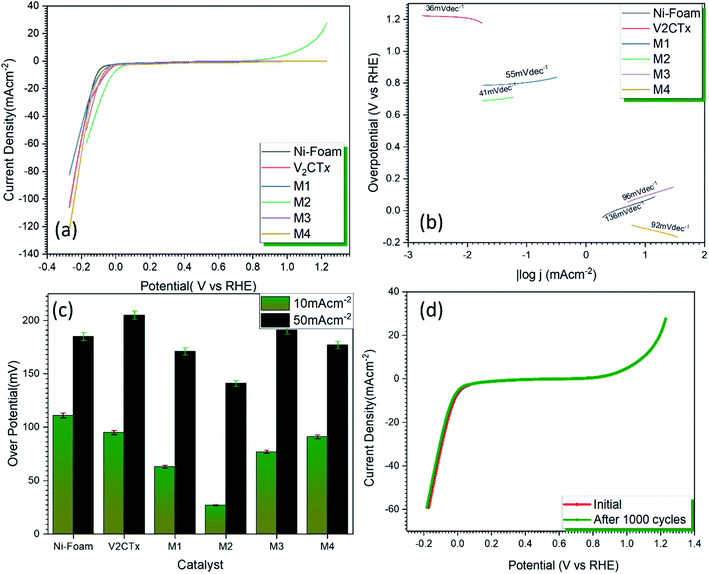 |
| Fig. 5 (a) HER polarization curves (b) Tafel slopes against overpotential of RHE of pristine MXene and MWCNT decorated V2CTx MXene (c) comparison of over potential @ η10 and η50 mA cm−2 (d) durability test of MWCNT modified MXene electrode for 1000 CV cycles. | |
The lower Tafel slope of 44 mV dec−1 (Fig. 5b) shows an outstanding HER kinetics obeying Volmer–Heyrovsky mechanism.
Volmer step:
H2O + M + e− → M–Hads + OH− |
Heyrovsky step:
where, M is the active metal site, during the Volmer process in an alkaline solution M–H
ads intermediate is formed when hydrogen is released from the catalyst surface followed by the desorption process resulted in hydrogen gas evolution (Heyrovsky process).
This outstanding catalytic performance is the result of synergetic effects between V2CTx and multiwall carbon nanotubes along with the conductive support provided by Ni foam. The porous Ni foam provides the smaller pathways for the movement of ions.58,59 The MWCNT forms a uniform conductive network that facilitates the ion diffusion and provide large surface area that results in the high catalytic activity. The durability is the key for the industrial scale considerations. The catalyst was subjected to 1000 cycles, and it is observed that the catalyst is stable and there is a very minor change in the catalytic activity (Fig. 5d). An over-potential of 28 mV was achieved which shows high durability of MWCNT@V2CTx as shown in Fig. 5c.
Stability test
From Fig. 6a, it can be observed from the Nyquist plots that the hybrid material has the lowest charge transfer resistance of 245.4 Ω as compared to pristine MXene (1.502 × 103). This result confirms that layered V2CTx MXene with high conductivity and with active sites facilitates the fast charge transfer resulting in an efficient catalytic activity because of its strong uniform interfacial linkage with MWCNTS thus, the charge transfer behavior is immensely enhanced.60,61 The cyclic voltammograms of the hybrid at scan rates of 10 mV s−1, 100 mV s−1 and 200 mV s−1 are shown in inset of Fig. 6b. It can be seen from the shape of the curve that the total capacitance is the sum of EDLC capacitance of MWCNTs and pseudo-capacitance of V2CTx MXene. As MWCNT provides conductive channels for the fast ion diffusion and their increased surface are enhances the active site availability hence an increased electrochemical activity. The redox peaks are visible in the potential range of 2–3.1 V. The slight shifting of the peaks can be observed due to faradaic activity while the shape of the curve is maintained even at higher scan rates showing the symmetry and confirming the reversibility of the electrode material. The current density increases with an increase in the scan rate due to small diffusion resistance. An increase in the redox peak at higher scan rate is attributed to surface confined faradaic process that contributes to the stability of the electrode. The electron impedance spectroscopy EIS was carried out within the frequency range of 0.1 Hz to 20 kHz at a constant AC potential of 10 mV. Since, the stability of electrochemical device is a key parameter for the commercialization of the catalyst, for this purpose, the chronoamperometry was carried out at 0.6 V for 16 h which shows a very small change in current for MWCNT@V2CTx confirming high stability of the catalyst.
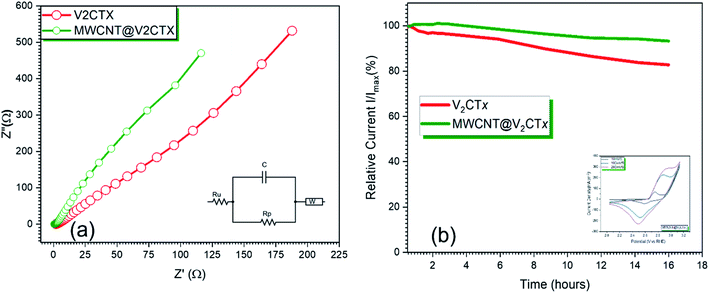 |
| Fig. 6 (a) EIS curves of pristine and MWCNT modified V2CTx MXene with equivalent circuit model (b) chronoamperometric curves of MWCNT modified V2CTx MXene for 16 h @ 0.6 V, inset: cyclic voltammograms of MWCNT decorated V2CTx MXene at different scan rates. | |
The EIS data was subject to Randle's model fitting with additional Warburg element for fitting purposes. Table 1 shows a comprehensive analysis for the different components of equivalent circuit confirming the low charge transfer resistance of MWCNT modified V2CTx i.e. 245.4 Ω as compared to pristine MXene with 1.502 × 103 Ω thus responsible for fast kinetics of the reaction.
Table 1 Fitting parameter values of the EIS data of V2CTx MXene and MWCNT@V2CTx
Sample |
Rs (Ω) |
Rct (Ω) |
C (F) |
W (Ω s−(1/2)) |
V2CTx |
3.30 |
1.502 × 103 |
4.6 × 10−3 |
16.08 × 10−3 |
MWCNT@V2CTx |
1.6 |
245.4 |
10.74 × 10−3 |
41.59 × 10−3 |
A comparison of this research study to MXene-based electrocatalysts is given in Table 2.
Table 2 Summary of electrocatalytic (HER & OER) activities of MXene-based electrocatalysts
Catalyst |
Electrolyte |
Activity |
Over-potential @ 10 mA cm−2 (η10) (mV) |
References |
VS2/V2CTx |
1 M KOH |
HER |
164 |
66 |
MWCNT/V2CTx |
1 M KOH |
HER |
27 |
This work |
Co3+–V2CTx |
1 M KOH |
OER |
420 |
69 |
MWCNT/V2CTx |
1 M KOH |
OER |
469 |
This work |
Co/N-CNTs@Ti3C2Tx |
0.1 M KOH |
OER |
411 |
65 |
V4C3Tx |
0.5 M H2SO4 |
HER |
200 |
47 |
Mo2CTx |
1 M H2SO4 |
HER |
230 |
70 |
Ti3C2Tx flakes |
0.5 M H2SO4 |
HER |
385 |
71 |
Co3+–Ti2CTx |
1 M KOH |
OER |
420 |
69 |
Co3+–Cr2CTx |
1 M KOH |
OER |
420 |
69 |
MoS2/Ti3C2-MXene@C |
0.5 M H2SO4 |
HER |
135 |
72 |
Co–Bi/Ti3C2Tx |
1 M KOH |
HER |
250 |
73 |
FeNi-LDH/Ti3C2Tx |
1 M KOH |
OER |
298 |
74 |
N–Ti2CTx |
0.5 M H2SO4 |
HER |
215 |
75 |
NiS2/V2CTx |
1 M KOH |
HER |
179 |
76 |
CoNi-ZIF-67@Ti3C2Tx |
1 M KOH |
OER |
323 |
77 |
MoS2⊥Ti3C2 |
0.5 M H2SO4 |
HER |
110 |
78 |
Co–MoS2@Mo2CTx |
1 M KOH |
HER |
112 |
79 |
Ti3C2Tx nanofibers |
0.5 M H2SO4 |
HER |
169 |
71 |
Summary
In summary, a novel MWCNT–MXene heterostructure was constructed for efficient bifunctional electrochemical catalyst for an overall water splitting. For comparison, pristine MXene catalyst was also synthesized and characterized. MWCNT@V2CTx showed a remarkable HER activity and an OER performance superior to pristine MXene. This enhanced performance may be attributed to the high conductivity of MXene sheets holding many active sites and uniform networking of MWCNTS over the surface, enhancing charge transfer ability and aggregation prevention. This work reveals that MWCNT@V2CTx has a potential to replace commercial noble metal electrocatalysts. Moreover, this study opens the doors for other construction of MWCNT hybrids of MXene based materials for efficient bifunctional electrocatalysis for an overall water splitting.
Author contributions
Syedah Afsheen Zahra performed experimentation and paper writing; and Syed Rizwan conceived the research concept, helped in paper writing, and supervised the complete project.
Conflicts of interest
There are no conflicts to declare.
Acknowledgements
The authors thank the Higher Education Commission (HEC) of Pakistan for providing research funding under the Project No.: 20-14784/NRPU/R&D/HEC/2021.
References
- Y. Li, H. Wang, L. Xie, Y. Liang, G. Hong and H. Dai, MoS2 nanoparticles grown on graphene: an advanced catalyst for the hydrogen evolution reaction, J. Am. Chem. Soc., 2011, 133(19), 7296–7299 CrossRef CAS PubMed.
- L. Liao, et al., A nanoporous molybdenum carbide nanowire as an electrocatalyst for hydrogen evolution reaction, Energy Environ. Sci., 2014, 7(1), 387–392 RSC.
- Z. Zhang, Y. Zhu, X. Chen, H. Zhang and J. Wang, A full-spectrum metal-free porphyrin supramolecular photocatalyst for dual functions of highly efficient hydrogen and oxygen evolution, Adv. Mater., 2019, 31(7), 1806626 CrossRef PubMed.
- B. Zhang, J. Hou, Y. Wu, S. Cao, Z. Li, X. Nie and L. Sun, Tailoring Active Sites in Mesoporous Defect-Rich NC/Vo-WON Heterostructure Array for Superior Electrocatalytic Hydrogen Evolution, Adv. Energy Mater., 2019, 9(12), 1803693 CrossRef.
- H. Jiang, J. Gu, X. Zheng, M. Liu, X. Qiu, L. Wang and J. Li, Defect-rich and ultrathin N doped carbon nanosheets as advanced trifunctional metal-free electrocatalysts for the ORR, OER and HER, Energy Environ. Sci., 2019, 12(1), 322–333 RSC.
- B. You, M. T. Tang, C. Tsai, F. Abild-Pedersen, X. Zheng and H. Li, Enhancing electrocatalytic water splitting by strain engineering, Adv. Mater., 2019, 31(17), 1807001 CrossRef PubMed.
- J. Staszak-Jirkovský, C. D. Malliakas, P. P. Lopes, N. Danilovic, S. S. Kota, K. C. Chang and N. M. Markovic, Design of active and stable Co–Mo–Sx chalcogels as pH-universal catalysts for the hydrogen evolution reaction, Nat. Mater., 2016, 15(2), 197–203 CrossRef PubMed.
- P. D. Vaidya, Y. J. Wu and A. E. Rodrigues, Kinetics of ethanol steam reforming for hydrogen production, in Ethanol, Elsevier, 2019, pp. 341–354 Search PubMed.
- A. Ersoz, H. Olgun and S. Ozdogan, Reforming options for hydrogen production from fossil fuels for PEM fuel cells, J. Power Sources, 2006, 154(1), 67–73 CrossRef CAS.
- P. Kuang, T. Tong, K. Fan and J. Yu, In situ fabrication of Ni–Mo bimetal sulfide hybrid as an efficient electrocatalyst for hydrogen evolution over a wide pH range, ACS Catal., 2017, 7(9), 6179–6187 CrossRef CAS.
- P. Kuang, M. He, H. Zou, J. Yu and K. Fan, 0D/3D MoS2-NiS2/N-doped graphene foam composite for efficient overall water splitting, Appl. Catal., B, 2019, 254, 15–25 CrossRef CAS.
- T. Maiyalagan, K. A. Jarvis, S. Therese, P. J. Ferreira and A. Manthiram, Spinel-type lithium cobalt oxide as a bifunctional electrocatalyst for the oxygen evolution and oxygen reduction reactions, Nat. Commun., 2014, 5(1), 1–8 Search PubMed.
- Y. Zheng, Y. Jiao, Y. Zhu, L. H. Li, Y. Han, Y. Chen and S. Z. Qiao, Hydrogen evolution by a metal-free electrocatalyst, Nat. Commun., 2014, 5(1), 1–8 Search PubMed.
- Q. Lu, G. S. Hutchings, W. Yu, Y. Zhou, R. V. Forest, R. Tao and J. G. Chen, Highly porous non-precious bimetallic electrocatalysts for efficient hydrogen evolution, Nat. Commun., 2015, 6(1), 1–8 Search PubMed.
- F. Wang, Y. Li, T. A. Shifa, K. Liu, F. Wang, Z. Wang and J. He, Selenium-enriched nickel selenide nanosheets as a robust electrocatalyst for hydrogen generation, Angew. Chem., Int. Ed., 2016, 55(24), 6919–6924 CrossRef CAS PubMed.
- J. X. Feng, J. Q. Wu, Y. X. Tong and G. R. Li, Efficient hydrogen evolution on Cu nanodots-decorated Ni3S2 nanotubes by optimizing atomic hydrogen adsorption
and desorption, J. Am. Chem. Soc., 2018, 140(2), 610–617 CrossRef CAS PubMed.
- H. Hu, B. Guan, B. Xia and X. W. Lou, Designed formation of Co3O4/NiCo2O4 double-shelled nanocages with enhanced pseudocapacitive and electrocatalytic properties, J. Am. Chem. Soc., 2015, 137(16), 5590–5595 CrossRef CAS PubMed.
- M. Blasco-Ahicart, J. Soriano-López, J. J. Carbó, J. M. Poblet and J. R. Galan-Mascaros, Polyoxometalate electrocatalysts based on earth-abundant metals for efficient water oxidation in acidic media, Nat. Chem., 2018, 10(1), 24–30 CrossRef CAS PubMed.
- L. Trotochaud, J. K. Ranney, K. N. Williams and S. W. Boettcher, Solution-cast metal oxide thin film electrocatalysts for oxygen evolution, J. Am. Chem. Soc., 2012, 134(41), 17253–17261 CrossRef CAS PubMed.
- L. Han, S. Dong and E. Wang, Transition-metal (Co, Ni, and Fe)-based electrocatalysts for the water oxidation reaction, Adv. Mater., 2016, 28(42), 9266–9291 CrossRef CAS PubMed.
- A. Vojvodic and J. K. Nørskov, Optimizing perovskites for the water-splitting reaction, Science, 2011, 334(6061), 1355–1356 CrossRef CAS PubMed.
- E. J. Popczun, J. R. McKone, C. G. Read, A. J. Biacchi, A. M. Wiltrout, N. S. Lewis and R. E. Schaak, Nanostructured nickel phosphide as an electrocatalyst for the hydrogen evolution reaction, J. Am. Chem. Soc., 2013, 135(25), 9267–9270 CrossRef CAS PubMed.
- X. Y. Yu, Y. Feng, B. Guan, X. W. D. Lou and U. Paik, Carbon coated porous nickel phosphides nanoplates for highly efficient oxygen evolution reaction, Energy Environ. Sci., 2016, 9(4), 1246–1250 RSC.
- L. Yan, L. Cao, P. Dai, X. Gu, D. Liu, L. Li and X. Zhao, Metal-organic frameworks derived nanotube of nickel–cobalt bimetal phosphides as highly efficient electrocatalysts for overall water splitting, Adv. Funct. Mater., 2017, 27(40), 1703455 CrossRef.
- Z. Pu, J. Zhao, I. S. Amiinu, W. Li, M. Wang, D. He and S. Mu, A universal synthesis strategy for P-rich noble metal diphosphide-based electrocatalysts for the hydrogen evolution reaction, Energy Environ. Sci., 2019, 12(3), 952–957 RSC.
- B. Chang, Z. Ai, D. Shi, Y. Zhong, K. Zhang, Y. Shao and X. Hao, p–n tungsten oxide homojunctions for Vis-NIR light-enhanced electrocatalytic hydrogen evolution, J. Mater. Chem. A, 2019, 7(33), 19573–19580 RSC.
- Z. Ai, B. Chang, C. Xu, B. Huang, Y. Wu, X. Hao and Y. Shao, Interface engineering in the BNNS@Ti3C2 intercalation structure for enhanced electrocatalytic hydrogen evolution, New J. Chem., 2019, 43(22), 8613–8619 RSC.
- J. Jia, M. Zhai, J. Lv, B. Zhao, H. Du and J. Zhu, Nickel molybdenum nitride nanorods grown on Ni foam as efficient and stable bifunctional electrocatalysts for overall water splitting, ACS Appl. Mater. Interfaces, 2018, 10(36), 30400–30408 CrossRef CAS PubMed.
- Y. Gu, S. Chen, J. Ren, Y. A. Jia, C. Chen, S. Komarneni and X. Yao, Electronic structure tuning in Ni3FeN/r-GO aerogel toward bifunctional electrocatalyst for overall water splitting, ACS Nano, 2018, 12(1), 245–253 CrossRef CAS PubMed.
- I. Ashraf, S. Rizwan and M. Iqbal, A comprehensive review on the synthesis and energy applications of nano-structured metal nitrides, Front. Mater., 2020, 7, 181 CrossRef.
- J. Lin, Y. Yu, Z. Xu, F. Gao, Z. Zhang, F. Zeng and G. Li, Electronic engineering of transition metal Zn-doped InGaN nanorods arrays for photoelectrochemical water splitting, J. Power Sources, 2020, 450, 227578 CrossRef CAS.
- H. Liang, F. Meng, M. Cabán-Acevedo, L. Li, A. Forticaux, L. Xiu and S. Jin, Hydrothermal continuous flow synthesis and exfoliation of NiCo layered double hydroxide nanosheets for enhanced oxygen evolution catalysis, Nano Lett., 2015, 15(2), 1421–1427 CrossRef CAS PubMed.
- F. Song and X. Hu, Ultrathin cobalt–manganese layered double hydroxide is an efficient oxygen evolution catalyst, J. Am. Chem. Soc., 2014, 136(47), 16481–16484 CrossRef CAS PubMed.
- F. X. Ma, H. B. Wu, B. Y. Xia, C. Y. Xu and X. W. Lou, Hierarchical β-Mo2C nanotubes organized by ultrathin nanosheets as a highly efficient electrocatalyst for hydrogen production, Angew. Chem., 2015, 127(51), 15615–15619 CrossRef.
- Y. P. Zhu, C. Guo, Y. Zheng and S. Z. Qiao, Surface and interface engineering of noble-metal-free electrocatalysts for efficient energy conversion processes, Acc. Chem. Res., 2017, 50(4), 915–923 CrossRef CAS PubMed.
- W. Zhang, W. Lai and R. Cao, Energy-related small molecule activation reactions: oxygen reduction and hydrogen and oxygen evolution reactions catalyzed by porphyrin-and corrole-based systems, Chem. Rev., 2017, 117(4), 3717–3797 CrossRef CAS PubMed.
- B. Anasori, M. R. Lukatskaya and Y. Gogotsi, Nat. Rev. Mater., 2017, 2(2), 16098 CrossRef CAS.
- M. Naguib, R. R. Unocic, B. L. Armstrong and J. Nanda, Large-scale delamination of multi-layers transition metal carbides and carbonitrides “MXenes”, Dalton Trans., 2015, 44(20), 9353–9358 RSC.
- M. Naguib, J. Come, B. Dyatkin, V. Presser, P. L. Taberna, P. Simon and Y. Gogotsi, MXene: a promising transition metal carbide anode for lithium-ion batteries, Electrochem. Commun., 2012, 16(1), 61–64 CrossRef CAS.
- Y. Yoon, M. Lee, S. K. Kim, G. Bae, W. Song, S. Myung and K. S. An, A Strategy for Synthesis of Carbon Nitride Induced Chemically Doped 2D MXene for High-Performance Supercapacitor Electrodes, Adv. Energy Mater., 2018, 8(15), 1703173 CrossRef.
- M. Fatima, J. Fatheema, N. B. Monir, A. H. Siddique, B. Khan, A. Islam and S. Rizwan, Nb-doped MXene with enhanced energy storage capacity and stability, Front. Chem., 2020, 8, 168 CrossRef CAS PubMed.
- M. Fatima, S. A. Zahra, S. A. Khan, D. Akinwande, J. Minár and S. Rizwan, Experimental and Computational Analysis of MnO2@V2C-MXene for Enhanced Energy Storage, Nanomaterials, 2021, 11(7), 1707 CrossRef CAS PubMed.
- G. Gao, A. P. O'Mullane and A. Du, 2D MXenes: a new family of promising catalysts for the hydrogen evolution reaction, ACS Catal., 2017, 7(1), 494–500 CrossRef CAS.
- J. Zhang, Y. Zhao, X. Guo, C. Chen, C. L. Dong, R. S. Liu and G. Wang, Single platinum atoms immobilized on an MXene as an efficient catalyst for the hydrogen evolution reaction, Nat. Catal., 2018, 1(12), 985–992 CrossRef CAS.
- Z. W. Seh, K. D. Fredrickson, B. Anasori, J. Kibsgaard, A. L. Strickler, M. R. Lukatskaya and A. Vojvodic, Two-dimensional molybdenum carbide (MXene) as an efficient electrocatalyst for hydrogen evolution, ACS Energy Lett., 2016, 1(3), 589–594 CrossRef CAS.
- S. Li, P. Tuo, J. Xie, X. Zhang, J. Xu, J. Bao and Y. Xie, Ultrathin MXene nanosheets with rich fluorine termination groups realizing efficient electrocatalytic hydrogen evolution, Nano Energy, 2018, 47, 512–518 CrossRef CAS.
- M. H. Tran, T. Schäfer, A. Shahraei, M. Dürrschnabel, L. Molina-Luna, U. I. Kramm and C. S. Birkel, Adding
a new member to the MXene family: synthesis, structure, and electrocatalytic activity for the hydrogen evolution reaction of V4C3Tx, ACS Appl. Energy Mater., 2018, 1(8), 3908–3914 CrossRef CAS.
- A. Djire, H. Zhang, J. Liu, E. M. Miller and N. R. Neale, Electrocatalytic and optoelectronic characteristics of the two-dimensional titanium nitride Ti4N3Tx MXene, ACS Appl. Mater. Interfaces, 2019, 11(12), 11812–11823 CrossRef CAS PubMed.
- A. D. Handoko, K. D. Fredrickson, B. Anasori, K. W. Convey, L. R. Johnson, Y. Gogotsi and Z. W. Seh, Tuning the basal plane functionalization of two-dimensional metal carbides (MXenes) to control hydrogen evolution activity, ACS Appl. Energy Mater., 2017, 1(1), 173–180 CrossRef.
- L. Xiu, Z. Wang, M. Yu, X. Wu and J. Qiu, Aggregation-resistant 3D MXene-based architecture as efficient bifunctional electrocatalyst for overall water splitting, ACS Nano, 2018, 12(8), 8017–8028 CrossRef CAS PubMed.
- K. Li, M. Liang, H. Wang, X. Wang, Y. Huang, J. Coelho and Y. Xu, 3D MXene architectures for efficient energy storage and conversion, Adv. Funct. Mater., 2020, 30(47), 2000842 CrossRef CAS.
- C. Ling, L. Shi, Y. Ouyang and J. Wang, Searching for highly active catalysts for hydrogen evolution reaction based on O-terminated MXenes through a simple descriptor, Chem. Mater., 2016, 28(24), 9026–9032 CrossRef CAS.
- A. VahidMohammadi, A. Hadjikhani, S. Shahbazmohamadi and M. Beidaghi, Two-dimensional vanadium carbide (MXene) as a high-capacity cathode material for rechargeable aluminum batteries, ACS Nano, 2017, 11(11), 11135–11144 CrossRef CAS PubMed.
- W. Zhang, H. Jin, G. Chen and J. Zhang, Sandwich-like N-doped carbon nanotube@ Nb2C MXene composite for high performance alkali ion batteries, Ceram. Int., 2021, 47(14), 20610–20616 CrossRef CAS.
- P. Yan, R. Zhang, J. Jia, C. Wu, A. Zhou, J. Xu and X. Zhang, Enhanced super capacitive performance of delaminated two-dimensional titanium carbide/carbon nanotube composites in alkaline electrolyte, J. Power Sources, 2015, 284, 38–43 CrossRef CAS.
- F. Liu, Y. Liu, X. Zhao, K. Liu, H. Yin and L. Z. Fan, Prelithiated V2C MXene: A High-Performance Electrode for Hybrid Magnesium/Lithium-Ion Batteries by Ion Cointercalation, Small, 2020, 16(8), 1906076 CrossRef CAS PubMed.
- M. Q. Zhao, C. E. Ren, Z. Ling, M. R. Lukatskaya, C. Zhang, K. L. Van Aken and Y. Gogotsi, Flexible MXene/carbon nanotube composite paper with high volumetric capacitance, Adv. Mater., 2015, 27(2), 339–345 CrossRef CAS PubMed.
- X. Yang, Q. Jia, F. Duan, B. Hu, M. Wang, L. He and Z. Zhang, Multiwall carbon nanotubes loaded with MoS2 quantum dots and MXene quantum dots: Non–Pt bifunctional catalyst for the methanol oxidation and oxygen reduction reactions in alkaline solution, Appl. Surf. Sci., 2019, 464, 78–87 CrossRef CAS.
- M. M. Baig, I. H. Gul, M. Z. Khan, M. T. Mehran and M. S. Akhtar, Binder-free heterostructured MWCNTs/Al2S3 decorated on NiCo foam as highly reversible cathode material for high-performance supercapacitors, Electrochim. Acta, 2020, 340, 135955 CrossRef CAS.
- L. Huang, L. Ai, M. Wang, J. Jiang and S. Wang, Hierarchical MoS2 nanosheets integrated Ti3C2 MXenes for electrocatalytic hydrogen evolution, Int. J. Hydrogen Energy, 2019, 44(2), 965–976 CrossRef CAS.
- Q. Xue, Z. Pei, Y. Huang, M. Zhu, Z. Tang, H. Li and C. Zhi, Mn3O4 nanoparticles on layer-structured Ti3C2 MXene towards the oxygen reduction reaction and zinc–air batteries, J. Mater. Chem. A, 2017, 5(39), 20818–20823 RSC.
- Y. Cai, J. Shen, G. Ge, Y. Zhang, W. Jin, W. Huang and X. Dong, Stretchable Ti3C2Tx MXene/carbon nanotube composite based strain sensor with ultrahigh sensitivity and tunable sensing range, ACS Nano, 2018, 12(1), 56–62 CrossRef CAS PubMed.
- P. Yan, R. Zhang, J. Jia, C. Wu, A. Zhou, J. Xu and X. Zhang, Enhanced supercapacitive performance of delaminated two-dimensional titanium carbide/carbon nanotube composites in alkaline electrolyte, J. Power Sources, 2015, 284, 38–43 CrossRef CAS.
- X. Xie, M. Q. Zhao, B. Anasori, K. Maleski, C. E. Ren, J. Li, B. W. Byles, E. Pomerantseva, G. Wang and Y. Gogotsi, Porous heterostructured MXene/carbon nanotube composite paper with high volumetric capacity for sodium-based energy storage devices, Nano Energy, 2016, 26, 513–523 CrossRef CAS.
- Y. Zhang, H. Jiang, Y. Lin, H. Liu, Q. He, C. Wu and L. Song, In situ growth of cobalt nanoparticles encapsulated nitrogen-doped carbon nanotubes among Ti3C2Tx (MXene) matrix for oxygen reduction and evolution, Adv. Mater. Interfaces, 2018, 5(16), 1800392 CrossRef.
- S. Koh and P. Strasser, Electrocatalysis on bimetallic surfaces: modifying catalytic reactivity for oxygen reduction by voltammetric surface dealloying, J. Am. Chem. Soc., 2007, 129(42), 12624–12625 CrossRef CAS PubMed.
- W. Xia, X. Yin, S. Kundu, M. Sánchez, A. Birkner, C. Wöll and M. Muhler, Visualization and functions of surface defects on carbon nanotubes created by catalytic etching, Carbon, 2011, 49(1), 299–305 CrossRef CAS.
- Z. Wang, W. Xu, K. Yu, Y. Feng and Z. Zhu, 2D heterogeneous vanadium compound interfacial modulation enhanced synergistic catalytic hydrogen evolution for full pH range seawater splitting, Nanoscale, 2020, 12(10), 6176–6187 RSC.
- S. Y. Pang, Y. T. Wong, S. Yuan, Y. Liu, M. K. Tsang, Z. Yang and J. Hao, Universal strategy for HF-free facile and rapid synthesis of two-dimensional MXenes as multifunctional energy materials, J. Am. Chem. Soc., 2019, 141(24), 9610–9616 CrossRef CAS PubMed.
- D. A. Kuznetsov, Z. Chen, P. V. Kumar, A. Tsoukalou, A. Kierzkowska, P. M. Abdala and C. R. Müller, Single site cobalt substitution in 2D molybdenum carbide (MXene) enhances catalytic activity in the hydrogen evolution reaction, J. Am. Chem. Soc., 2019, 141(44), 17809–17816 CrossRef CAS PubMed.
- W. Yuan, L. Cheng, Y. An, H. Wu, N. Yao, X. Fan and X. Guo, MXene nanofibers as highly active catalysts for hydrogen evolution reaction, ACS Sustainable Chem. Eng., 2018, 6(7), 8976–8982 CrossRef CAS.
- X. Wu, Z. Wang, M. Yu, L. Xiu and J. Qiu, Nanohybrids: Stabilizing the MXenes by carbon nanoplating for developing hierarchical nanohybrids with efficient lithium storage and hydrogen evolution capability, Adv. Mater., 2017, 29, 1607017 CrossRef PubMed.
- J. Liu, T. Chen, P. Juan, W. Peng, Y. Li, F. Zhang and X. Fan, Hierarchical cobalt borate/MXenes hybrid with extraordinary electrocatalytic performance in oxygen evolution reaction, ChemSusChem, 2018, 11(21), 3758–3765 CrossRef CAS PubMed.
- M. Yu, S. Zhou, Z. Wang, J. Zhao and J. Qiu, Boosting electrocatalytic oxygen evolution by synergistically coupling layered double hydroxide with MXene, Nano Energy, 2018, 44, 181–190 CrossRef CAS.
- Y. Yoon, A. P. Tiwari, M. Lee, M. Choi, W. Song, J. Im and K. S. An, Enhanced electrocatalytic activity by chemical nitridation of two-dimensional titanium carbide MXene for hydrogen evolution, J. Mater. Chem. A, 2018, 6(42), 20869–20877 RSC.
- P. Kuang, M. He, B. Zhu, J. Yu, K. Fan and M. Jaroniec, 0D/2D NiS2/V-MXene composite for electrocatalytic H2 evolution, J. Catal., 2019, 375, 8–20 CrossRef CAS.
- Y. Wen, Z. Wei, C. Ma, X. Xing, Z. Li and D. Luo, MXene boosted CoNi-ZIF-67 as highly efficient electrocatalysts for oxygen evolution, Nanomaterials, 2019, 9(5), 775 CrossRef CAS PubMed.
- N. H. Attanayake, S. C. Abeyweera, A. C. Thenuwara, B. Anasori, Y. Gogotsi, Y. Sun and D. R. Strongin, Vertically aligned MoS 2 on Ti3C2 (MXene) as an improved HER catalyst, J. Mater. Chem. A, 2018, 6(35), 16882–16889 RSC.
- J. Liang, C. Ding, J. Liu, T. Chen, W. Peng, Y. Li and X. Fan, Heterostructure engineering of Co-doped MoS2 coupled with Mo2CTx MXene for enhanced hydrogen evolution in alkaline media, Nanoscale, 2019, 11(22), 10992–11000 RSC.
|
This journal is © The Royal Society of Chemistry 2022 |
Click here to see how this site uses Cookies. View our privacy policy here.