DOI:
10.1039/D2RA01318E
(Review Article)
RSC Adv., 2022,
12, 11318-11344
Phenanthroline based rotaxanes: recent developments in syntheses and applications
Received
27th February 2022
, Accepted 31st March 2022
First published on 12th April 2022
Abstract
The advancements in the field of mechanically interlocked molecular systems (MIMs) has concurrently restructured the material chemistry frontiers and provided ample scope to explore new dimensions for applications and diversity creation. Among all these molecular entities, rotaxanes have a special locale and many research groups over the globe have contributed to their current niche in supramolecular chemistry. From refinements for better yielding synthetic strategies to their application oriented designs, this field has come a long way and is well inclined for further developments. In this review, we aim to document the contemporary developments in the synthesis of phenanthroline (phen) based rotaxanes. In addition to providing a comprehensive account of various subtypes of these rotaxanes and their stitching strategies, their applications, wherever discernible, will be duely highlighted.
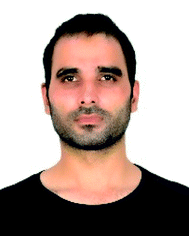 Showkat Rashid | Showkat Rashid received his M.Sc (2008–10) and M. Phil (2013) in chemistry from the University of Kashmir and his PhD in Dr Bilal A. Bhat's research group at CSIR-IIIM Jammu in a collaborative project between Dr Bhat and Prof. Goverdhan Mehta in the field of Natural product synthesis. He did his initial postdoctoral studies with Prof. Goverdhan Mehta at School of Chemistry, University of Hyderabad and presently he is working at Tokyo University of Science, Japan with Prof. Schinichi Saito as postdoctoral fellow. His current research interests are design and synthesis of aesthetically pleasing molecular constructs including molecular rotaxanes for various applications. |
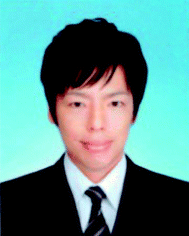 Yusuke Yoshigoe | Yusuke Yoshigoe, received his B.Sc. degree in 2011 from Tokyo gakugei university, and his PhD degree in 2016 from Titech (Prof. Kohtaro Osakada). He spent three years in Prof. Yoichiro Kuninobu's group at the Kyushu University, and one year in National Institute of Applied Industrial Science (AIST) and Technology, as post-doctoral fellow. He joined the Department of Chemistry, Faculty of Science, Tokyo University of Science as an assistant professor in Prof. Shinichi Saito's group in 2020. His current interests are organometallic chemistry and its synthetic application for π-conjugated molecules. |
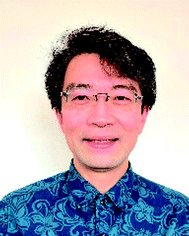 Shinichi Saito | Shinichi Saito obtained his PhD degree in 1995 from the University of Tokyo (Professor Koichi Shudo). In 1994, he stayed at the University of Utah (Professor Peter J. Stang) as a Nagai Foundation Fellow. After he worked in Tohoku University (Professor Yoshinori Yamamoto) and Toyama Medical and Pharmaceutical University (Professor Toru Koizumi), he became a RIKEN Special Researcher for Basic Science Program in Dr Wakatsuki's group. In 2002, he moved to Tokyo University of Science as a PI. His current research interests are the chemistry of interlocked compounds and the development of new reactions. |
Introduction
Rotaxanes represent a class of supramolecular architectures, comprising a macrocyclic component threaded to a linear axle component. This axle component bears bulky groups at both the terminals, avoiding the disintegration of the two components (Fig. 1).1,2
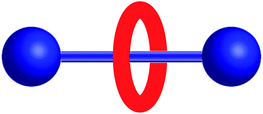 |
| Fig. 1 Pictorial representation of a typical [2]rotaxane. | |
Ever since the initial reports depicting the synthesis of rotaxanes in 1967 by Harrison, this field has seen tremendous growth and a large number of structurally as well as functionally different rotaxanes are being continuously added to this library of interesting molecular constructs. Pertinent to mention, initial attempts directed towards the synthesis of rotaxanes were faced with many practical problems like poor yields and reproducibility issues. In order to overcome these problems and facilitate a smooth access to these molecules, many refined synthetic approaches were developed over time. Some of these widely accepted approaches include threading, slipping, and clipping strategies. In addition to these, a more recent approach has evolved which is based upon the reaction catalysed by a transition metal inside the macrocyclic domain. Leigh3 and Saito's research groups4 have substantially contributed to this method for more than a decade. All these efforts culminated in generating a large number of rotaxanes having different structural and functional attributes. Depending upon the total sum of macrocycle and axle components, these rotaxanes can be classified as [2], [3], [4] or polyrotaxanes among which the higher order rotaxanes are very unique and interesting. In light of the voluminous literature being added continuously, there is a need for an inclusive documentation regarding the developments taking place in this field. In this review, we have comprehensively summarized the recent synthetic efforts (from 2001–2021) towards phenanthroline based rotaxanes. For the ease of presentation, we have classified these rotaxanes into different categories depending upon the mode of the assembling the cyclic and axle components. Applications of these rotaxanes will also be discussed to highlight their importance.
[A] Assembly of rotaxanes by utilizing the coordinating ability of the phen-Cu complex
1,10-Phenanthroline (Scheme 1) is a nitrogen-containing bidentate ligand with strong coordinating ability to metal ions. The strong binding between phenanthroline and the metal is retained even when bulky aryl or alkyl groups are introduced at 2- and 9- positions. 2,9-Disubstituted macrocyclic phenanthrolines could be accessed with ease due to the favourable alignment acquired by substituents at these positions. The metal interacting with the phenanthroline moiety of the macrocycle is located inside the ring and the propensity of this complex to affect various reactions has been utilized for the synthesis of mechanically interlocked molecular systems (MIMs). When two phenanthroline moieties interact with Cu ion, a tetrahedral complex results. This property was long back applied for the efficient synthesis of catenane by Dietrich-Buchecker and Sauvage (Scheme 1).5 In this study, a macrocyclic phenanthroline-Cu complex, 1 was treated with bisphenol 2 in presence of [Cu(CH3CN)4]BF4 to yield a tetrahedral Cu(I) complex 3. Diiodopolyether, 4 was reacted to install another ring to the intermediate 3 which eventually furnished the catenane 5.6 This seminal work provided a new and efficient approach for the synthesis of a large number of MIMs. For instance, the synthesis of a [2]rotaxane from a macrocyclic phenanthroline-Cu complex, was reported for the first time by Gibson wherein 3 was again utilized (Scheme 2).7 Introduction of blocking group through end capping reaction followed by final demetallation furnished the required rotaxane 6.
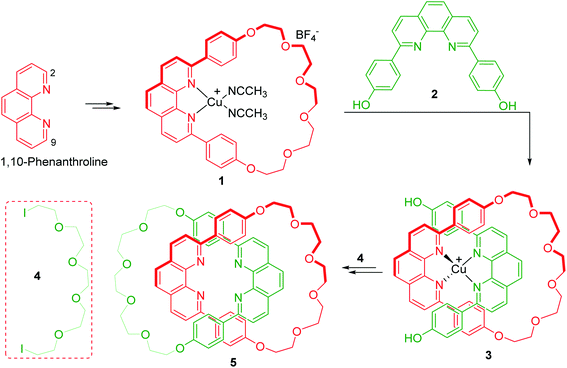 |
| Scheme 1 Synthesis of catenane from a tetrahedral phenanthroline-Cu(I) complex.6 | |
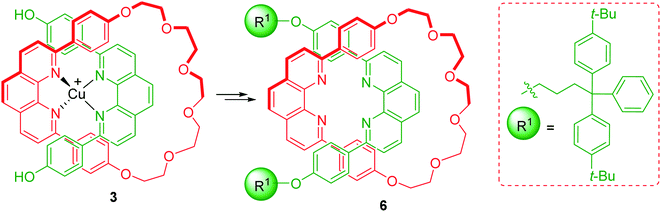 |
| Scheme 2 Synthesis of rotaxane from a tetrahedral phenanthroline-Cu complex.7 | |
Along the similar lines, Sauvage and co-workers shortly reported the synthesis of a novel rotaxane bearing two different porphyrin systems as chemical stoppers (Scheme 3).8 The role of these stoppers was not only to prevent de-threading but to induce novel electronic and photochemical properties to these molecular systems. Phenanthroline attached porphyrin, 7 was treated with the macrocycle 8 under standardized reaction conditions which resulted in intermediate 9. Second porphyrin stopper was introduced using the Lindsey's procedure which eventually furnished the rotaxane 10.
Saito group has estimated the relationship between the stability of the rotaxanes and the structure/size of the ring/axle components by using the same synthetic strategy, as mentioned above (Scheme 4).9 A series of phenanthroline based [2]rotaxanes 14a–d with difference in the size of macrocyclic component as well as in nature of blocking groups were synthesized. Rotaxane 14c, with small macrocycle 11a (n = 6) and having tris(4-cyclohexylbiphenyl)methyl group as blocking group was highly stable and the dissociation of the ring and axle components did not proceed under elevated temperature. However, rotaxane 14b with macrocycle 11b (n = 8) and tris(4-ethylbiphenyl)methyl functionality as blocking group was less stable, and the dissociation proceeded at 140 °C. No rotaxane formation was observed when larger macrocycle 11c (n = 10) was used.
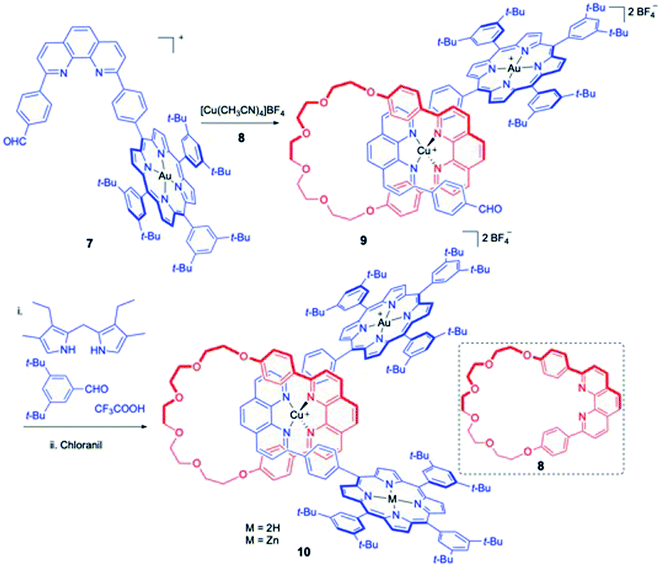 |
| Scheme 3 Synthesis of rotaxane bearing different porphyrin stoppers.8 | |
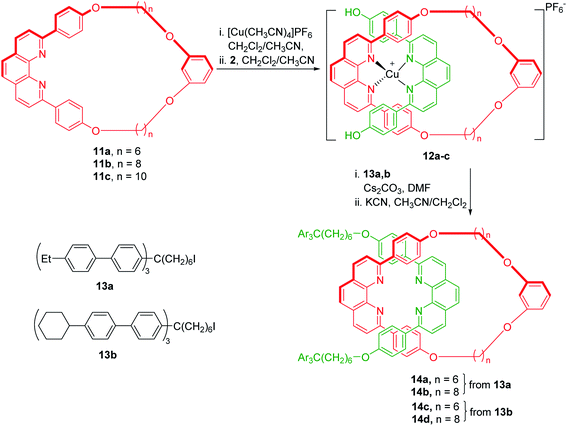 |
| Scheme 4 Synthesis of [2]rotaxanes by Williamson etherification.9 | |
In another advancement of this study, resorcinol-phen based macrocycles 11a,b were treated with bis(propargyl)phenanthroline moiety, 15 to result in stable tetrahedral Cu(I) complexes, 16a,b (Scheme 5).10 The introduction of the blocking groups was achieved by the copper catalyzed azide–alkyne Huisgen cycloaddition (CuAAC) reaction with azide 17. The removal of copper ion by KCN delivered the required [2]rotaxanes 18a (n = 6) and 18b (n = 8) in 63 and 76% yields respectively.
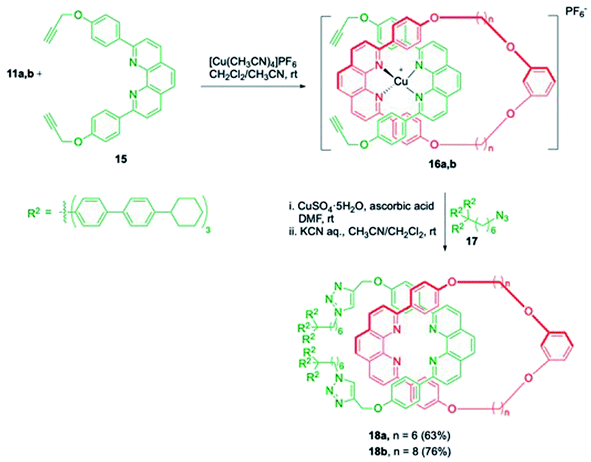 |
| Scheme 5 Synthesis of [2]rotaxanes by Azide–alkyne cycloaddition.10 | |
Tatay, Gaviña and co-workers reported the synthesis of a novel [2]rotaxane which harbours an elongated and unsymmetrical axle component (Scheme 6).11 Synthesis of this rotaxane ensued from mono-stoppered axle 19, having a terminal alkyne functionality at the free end for further manipulation. Treatment of 19 with macrocyclic component 8 in the presence of Cu(I) ion resulted in simultaneous threading of components to result in pseudorotaxane 20. Huisgen azide–alkyne stoppering reaction (with 21) in the presence of tris[2-(dimethylamino)ethyl]amine (Me6TREN)-copper complex generated the metal complexed rotaxane which under KCN mediated demetallation reaction furnished the final rotaxane. Remetallation was again performed to prepare metal complex 22 for electrochemical studies. Cyclic voltammetry depicted a reversible shuttling of the ring component along the axle.
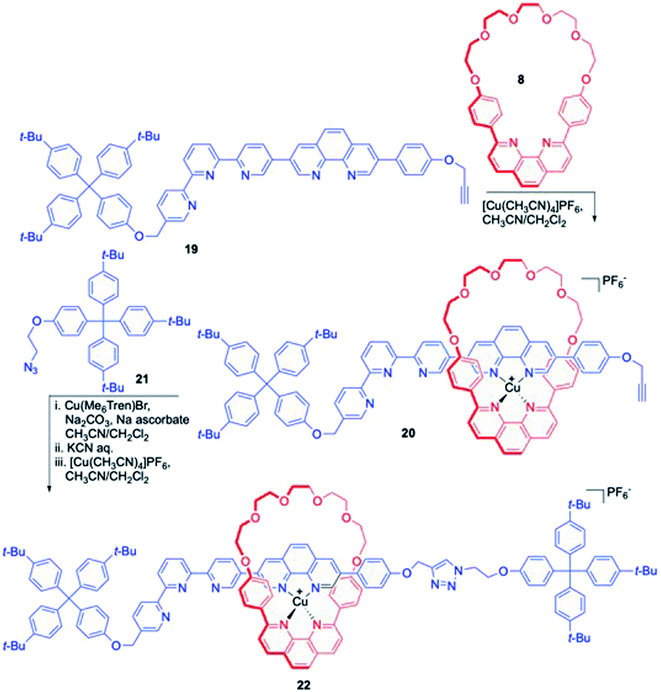 |
| Scheme 6 Synthesis of unsymmetrical [2]rotaxane using click reaction.11 | |
Stoddart, Sauvage and Yaghi in a collective effort reported the synthesis of a novel copper coordinated [2]pseudorotaxane and its successful incorporation into a MOF (Scheme 7).12 This strategy relies on Cu templated “gathering-and-threading” approach where a pseudorotaxane Cu complex 24 was synthesized by the reaction of a macrocyclic phenanthroline-Cu complex, 8-CuI with dicarboxylic acid 23. Treatment of 24 with Zn(NO3)2·6H2O in diethylformamide at 100 °C over 2 days yielded MOF-1040.
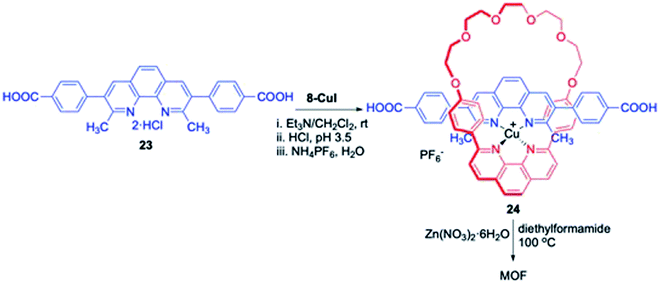 |
| Scheme 7 Synthesis of [2]pseudorotaxane and its MOF.12 | |
Diederich and co-workers reported the synthesis of a Cu coordinated bis-phenanthroline based [2]rotaxane wherein a [2 + 2] cycloaddition-retroelectrocyclization (CA-RE) reaction was implemented to install a bulky dicyanoquinodimethane (DCNQ) as second stopper (Scheme 8).13 Treatment of 25 with polyether macrocycle 8 in presence of copper salt furnished the pseudorotaxane 26 in 88% yield. Treatment of 26 with a stoichiometric quantity of 7,7,8,8-tetracyano-p-quinodimethane (TCNQ) triggered the CA-RE reaction to complete the synthesis of rotaxane 27 in 84% yield. The underlying photophysical properties of 27 were studied and it was found to exhibit complex excited-state dynamics and luminescence in the NIR region, which was detected as stimulated emission during transient absorption experimental investigations.
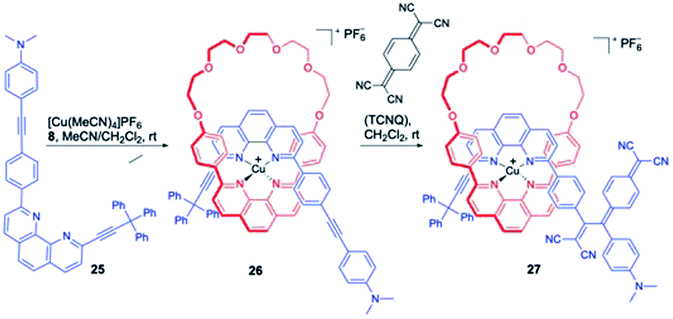 |
| Scheme 8 Synthesis of [2]rotaxane with DCNQ stopper.13 | |
Sauvage and co-workers reported the synthesis of a [3]rotaxane consisting of two copper coordinating macrocyclic rings threaded to an axle component at two co-ordinating sites (Scheme 9).14 The synthesis commenced from the reaction of the rigid axle precursor 28 having two coordinating sites with two equivalents of a Cu salt and a macrocyclic phenanthroline 8. The quantitative formation of 29 was observed, and the stoppering reaction with azide 30 by Huisgen cycloaddition delivered the Cu complexed rotaxane 31. The final [3]rotaxane 32 was synthesized by the removal of the copper from 31 upon treatment with KCN. The movement of the ring components was induced by the electrochemical redox process of the metal coordinated complex derived from 32.
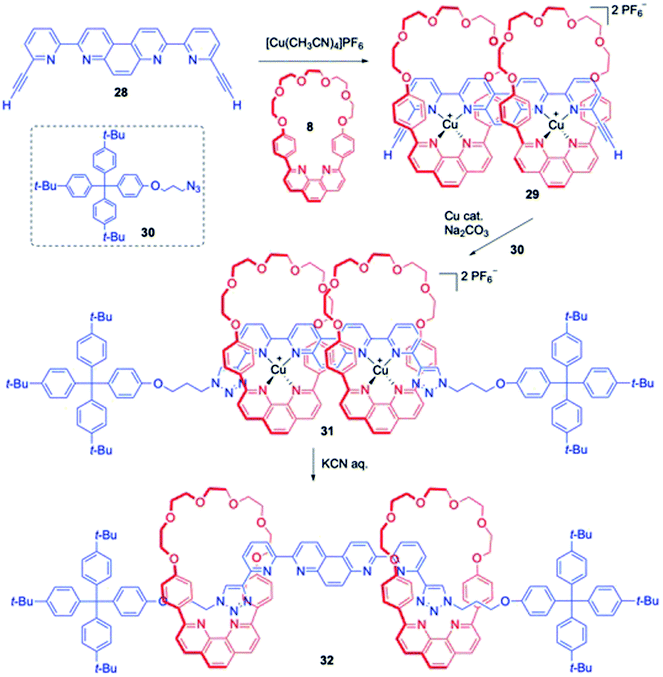 |
| Scheme 9 Synthesis of [3]rotaxane using click reaction for stopper installation.14 | |
Sauvage and co-workers reported the synthesis of a novel [3]rotaxane comprising of four phenanthroline-containing rings attached to the meso positions of a central zinc porphyrin system via C–C bonds (Scheme 10).15 The axles contain a central bis-3,8-(o-pyridyl)-4,7-phenanthroline rigid fragment and each axle is threaded through two adjacent phenanthroline rings of the porphyrin system. The phenanthroline based macrocyclic aldehyde 33 was used as a precursor and its condensation with pyrrole resulted in porphyrinic tetra-macrocycle 34. The rotaxane was synthesised from 34 through a two-step process. Initial treatment of 34 with acetylenic thread 35 in presence of [Cu(CH3CN)4](PF6) resulted in a tetrahedral complex with Cu(I) and delivered a [3]pseudorotaxane as an intermediate which upon further treatment with azide stopper 30 in presence of [Cu(CH3CN)4](PF6) and Na2CO3 underwent a Huisgen azide–alkyne cycloaddition to deliver the desired [3]rotaxane 36 in 44% yield over 2 steps.
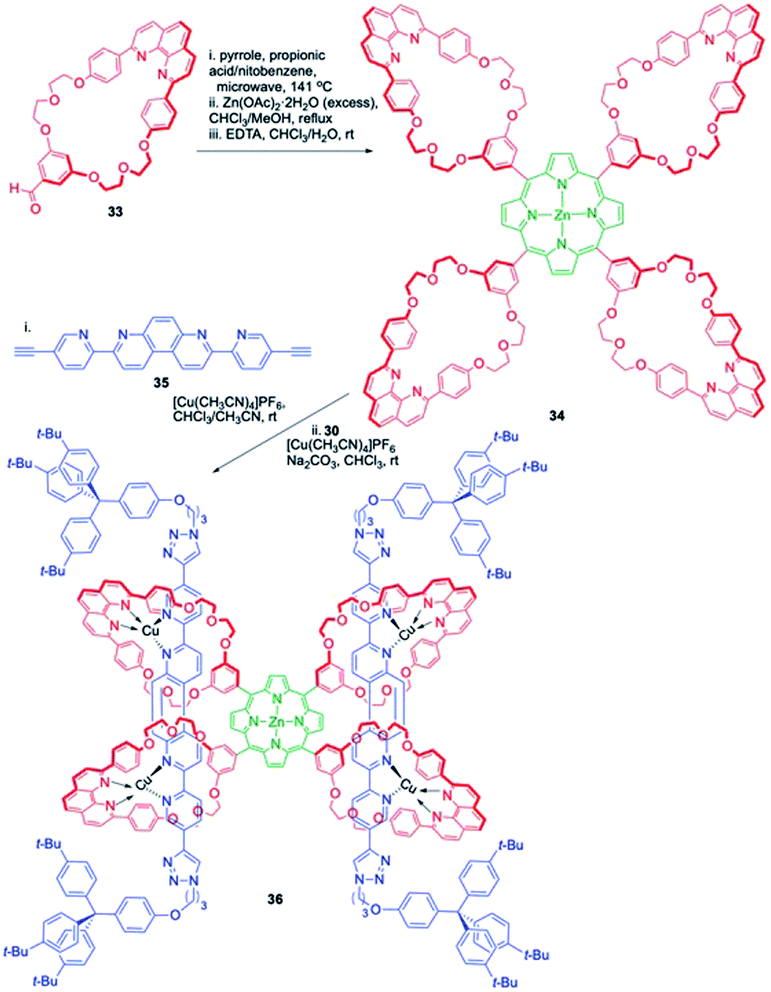 |
| Scheme 10 . Synthesis of porphyrin-based click rotaxane by π–π stacking.15 | |
Ghosh and co-workers reported the synthesis of a redox active click rotaxane, using π–π stacking interaction between the axle and macrocyclic components to assemble the target compound (Scheme 11).16 Treatment of macrocycle 37 with axle precursor 38 gave rise to interactions between the electron deficient pyridine units of 38 and electron rich phenyl units of 37 through π–π stacking interaction that ultimately resulted in the formation of 1
:
1 threaded complex, 39. Treatment of 39 with azide containing bulky stopper 40 in presence of copper catalyst completed the synthesis of rotaxane 41. Important functionalities of this rotaxane in the form of phenanthroline ester moiety, amide bond, triazolyl rings and viologen units render it a useful candidate for studying the associated anion binding and redox active properties.
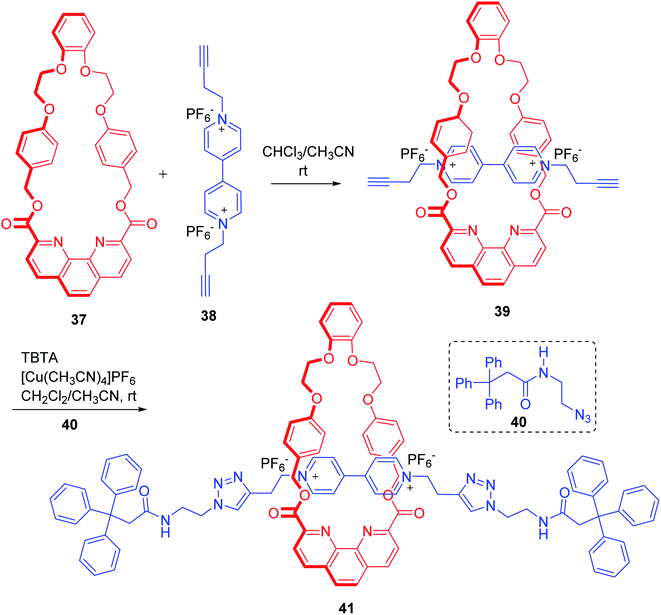 |
| Scheme 11 Synthesis of triazole containing rotaxane by π–π stacking.16 | |
[B] Assembly of rotaxanes by utilizing the catalytic activity of the phen-Cu complex
In addition to their peculiar coordinating ability for facilitating the synthesis of rotaxanes and catenanes, it was found that many of the phen-derived macrocycles, such as the ones discussed above acted as excellent catalysts for many coupling reactions. These coupling reactions (e.g. Glaser and Cadiot–Chodkiewicz coupling) were therefore utilized to stitch the appropriate axle fragments in presence of various Cu-derived macrocycles which culminated in the synthesis of different [2], [3] and polyrotaxanes. In this part of the review, we focus on the synthesis of rotaxanes from coupling potential of macrocyclic component and will be discussed under following two sub-sections.
1. Synthesis of diyne [2]rotaxanes. These rotaxanes in fact are reminiscent of a coupling reaction (Glaser coupling) between terminal alkyne axle precursor (specifically substituted by large terminal blocking groups) assisted by the catalytic activity of macrocyclic Cu-complex which results in final stitching of the two components. In this synthetic strategy, known as the catalytic threading strategy, a metal catalysed chemical reaction operates inside the ring fragment which assembles the two individual components before the final removal of metal and eventually delivers the required rotaxanes in an efficient manner (Fig. 2). Although many members of this subclass were earlier reviewed, few prototypical examples will still be discussed in order to be more comprehensive.17 Synthetic utility of the reaction catalysed by phenanthroline-Cu complex for [2]rotaxane synthesis was highlighted by Saito group (Scheme 12).4 A specifically designed phenanthroline complex, based upon the resorcinol backbone, 11a-CuI catalysed the oxidative dimerization (Glaser coupling) of axle precursors bearing bulky dumbbell moieties 42a,b for the synthesis of diyne based [2]rotaxanes 43a,b in good yields. This Cu assisted bond forming reaction was applied for the synthesis of a diverse range of rotaxanes. For instance, a phenanthroline-based macrocycle 44, which is devoid of phenyl group, reacted with the axle precursor 42b and yielded another analogue of [2]rotaxane 45 (Scheme 13).18
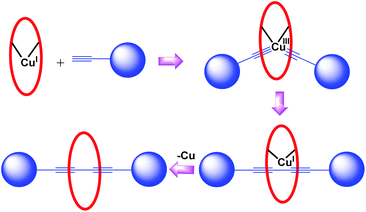 |
| Fig. 2 Glaser coupling for diyne rotaxanes. | |
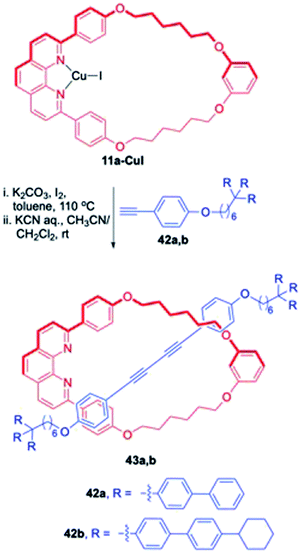 |
| Scheme 12 Synthesis of [2]rotaxanes using Glaser coupling.17 | |
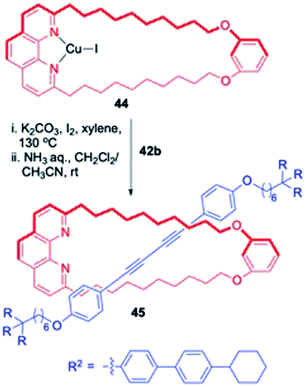 |
| Scheme 13 Synthesis of [2]rotaxane with modified macrocycle.18 | |
The use of Glaser coupling was further extended to achieve the synthesis of some chiral [2]rotaxanes (Scheme 14).19 In this approach, reaction of (R)-BINOL derived macrocycle (R)-46 with 42b delivered the chiral rotaxane (R)-47 in 78% yield. This homochiral rotaxane was further investigated for its optical properties which depicted intense CD signals arising from its axle component placed within a chiral environment.
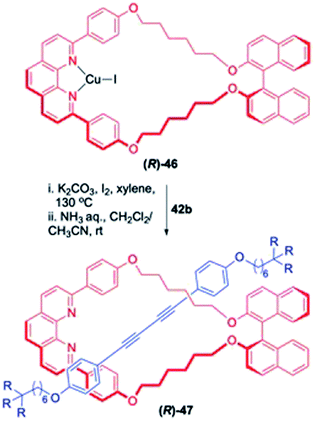 |
| Scheme 14 Synthesis of BINOL-derived chiral [2]rotaxane.19 | |
Another synthesis of a chiral [2]rotaxane was achieved by a totally different strategy (Scheme 15).20 The reaction of a less symmetric macrocycle 48 with 42b gave a rotaxane 49 with reduced symmetry. The introduction of a bulky pyrrole functionality into 49 upon treatment with 50 resulted the formation of a planar chiral [2]rotaxane, 51 with hampered shuttling of the ring component. The enantiomers were separated by chiral HPLC. Though the [2]rotaxane was optically stable at rt, the racemization of the [2]rotaxane proceeded at elevated temperature.
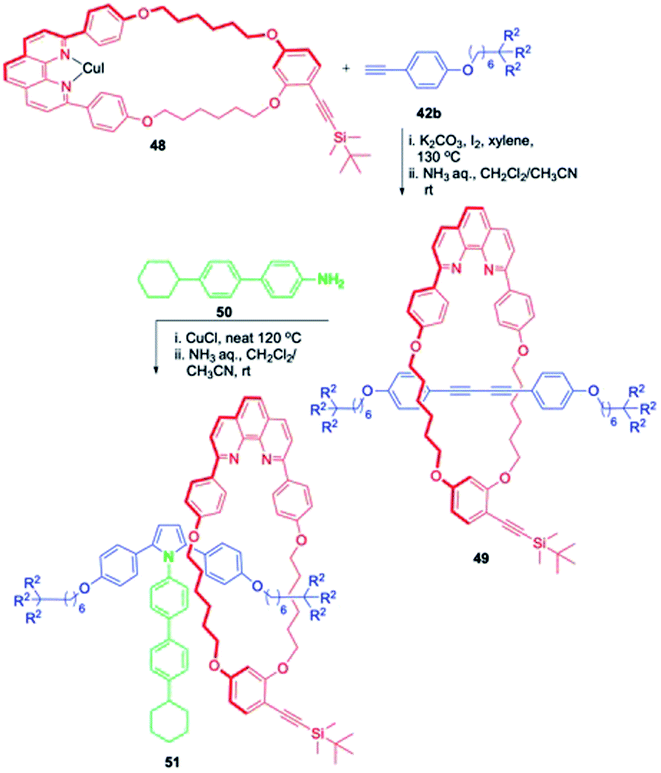 |
| Scheme 15 Synthesis of chiral [2]rotaxane by restricted shuttling.20 | |
Next, focus was shifted towards the synthesis of some structurally related [3]rotaxanes which represent interlocked compounds comprising of three components. The synthesis of [3]rotaxanes, especially those with one ring component and two axle components, has been a challenging issue. It was envisioned that these [3]rotaxanes could be prepared by utilizing the catalytic activity as well as the coordinating ability of the phenanthroline-Cu complex (Scheme 16).21 Initially, a series of [2]rotaxanes 52a–d were synthesised using the catalytic ability of the phen-Cu complexes, 11a,b-CuI in presence of axle components 42b,c. These [2]rotaxanes in turn were used as templates and their treatment with 15 in presence of copper catalyst generated the respective tetrahedral copper complexes, 53a–d bearing alkyne functionalities for Huisgen cycloaddition reaction. Treatment of 53a–d with azide 17 under standardized CuAAc conditions followed by Cu removal furnished the [3]rotaxanes 54a–d in very good yields.
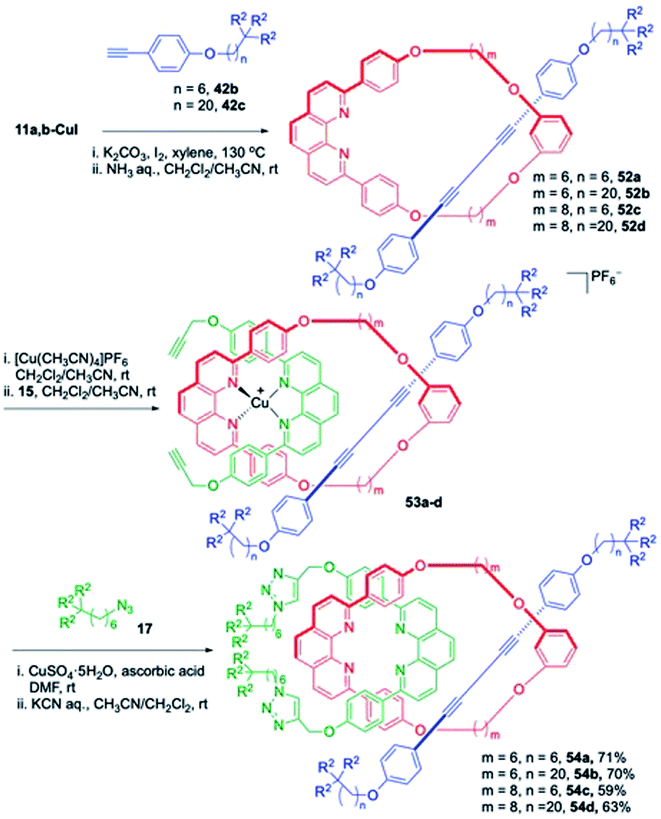 |
| Scheme 16 Synthesis of [3]rotaxanes using click chemistry.21 | |
In subsequent variations of this study, similar strategy was used to assemble many [3]rotaxane analogues with subtle variations in the end capping reaction using either azide–alkyne cycloaddition or Williamson ether synthesis.21
A sequence-selective synthesis of another class of interlocked compounds, known as rotacatenanes, which possess two macrocyclic components and one axle component was achieved efficiently (Scheme 17).22 In this synthetic approach, macrocyclic phenanthrolines 55a and 55b (synthesized by the Glaser coupling of acyclic compounds) were used as starting materials. The synthesis of [2]rotaxanes 56a and 56b was achieved by the reaction of 55a and 55b with 42b. Next, the coordinating ability of 56a and 56b was utilized to introduce the second ring component in each of these [2]rotaxanes to culminate in the synthesis of two rotacatenane isomers 57a and 57b.
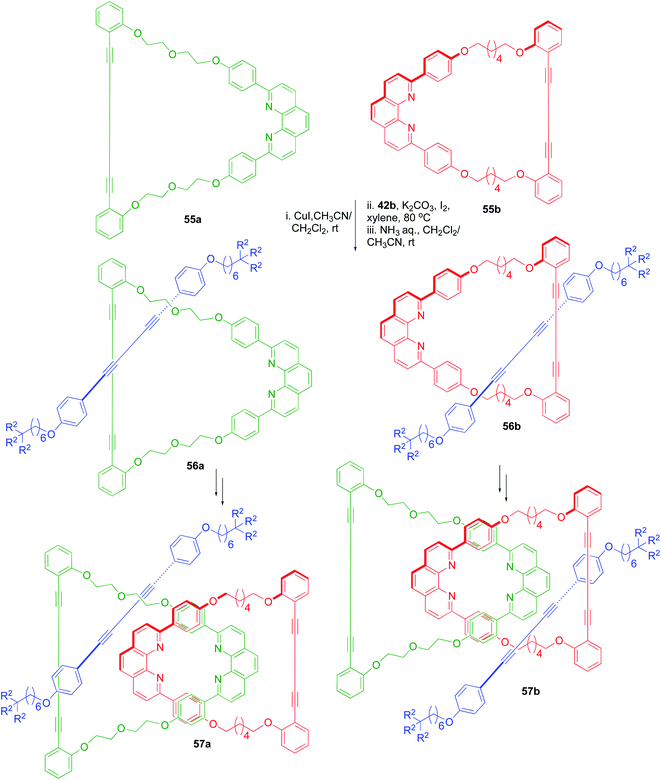 |
| Scheme 17 Sequence-selective synthesis of [2]rotaxanes and rotacatenanes.22 | |
A twofold threading reaction, either sequentially or simultaneously, was used to deliver the target [3]rotaxanes, 58a–c by Saito et al. (Scheme 18).23 First threading reaction between the 37 membered macrocyclic component, 11b-CuI and terminal alkynes 42b–d with various chain lengths resulted in the synthesis of [2]rotaxanes, as intermediates which underwent the second threading reaction operating inside tandemly to complete the synthesis of [3]rotaxanes 58a–c in acceptable yields along with smaller quantities of [2]rotaxane in some of the cases. As far as size of the macrocycle (methylene carbon chain) and the length of methylene units in the axle are concerned, they have an important role to play. With large macrocycle or an axle fragment with elongated methylene chain, synthesis of rotaxane was achieved nicely. However, with smaller macrocyclic complex, or a shorter methylene chain bearing axel fragment, the corresponding [3]rotaxane was not isolated which could be related to smaller cavity leading to increased steric bulk inside the macrocyclic cavity.
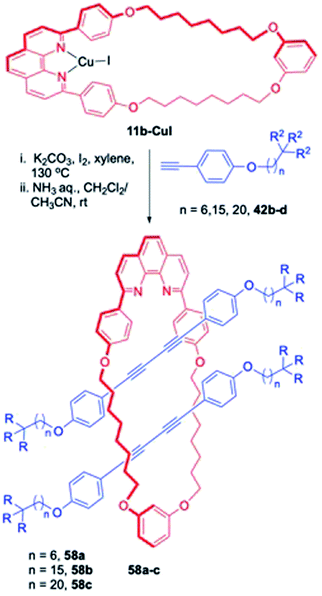 |
| Scheme 18 Sequential synthesis of [3]rotaxanes.23 | |
In addition to these above-mentioned examples, Weiss and Hayashi et. al. also reported a synthesis of a novel porphyrin based rotaxane using a “tandem active metal template” wherein a second metal ion, zinc(II) present in the porphyrin ring (along with the phenanthroline bound Cu) plays an important role in the Huisgen 1,3-dipolar cycloaddition reaction for assembling the final rotaxane (Scheme 19).24 In this endeavour, zinc porphyrin macrocycle 59 was in situ converted to corresponding copper complex, followed by treatment with azide 30 and alkyne 60 in the presence of diisopropylethylamine (DIPEA). A mixture of rotaxanes containing either copper, zinc, or both was encountered. Sequential removal of copper and zinc with ethylenediaminetetraacetic acid (EDTA) and trifluoroacetic acid (TFA) delivered the metal free rotaxane 61 whose nickel and zinc analogues were synthesized later by metalation with corresponding metal salts.
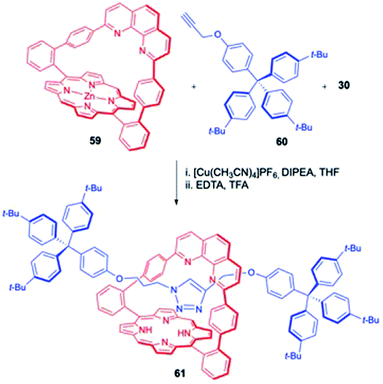 |
| Scheme 19 Synthesis porphyrin based rotaxane using click reaction.24 | |
2. Polyyne/cumulene derived rotaxanes. Banking on the advances in the synthetic strategies for rotaxanes, Anderson and co-workers have been actively engaged in the synthesis of rotaxanes in which a polyyne based axle component bearing bulky terminal groups, is threaded through a phenanthroline based macrocycle (Scheme 20).25 These rotaxanes with variable number of intervening sp carbon centers have been synthesized via slightly modified strategies as applied in case of the diynes. Sequential treatment of phenanthroline macrocycle, 11a with CuI, polyyne precursors, 62a–f and I2 yielded the polyyne rotaxanes, 63a–f. The coordinating ability of phenanthroline moiety was utilized to synthesize a [2] rotaxane–Re complex, 64b and the luminescence properties of this complex were studied.
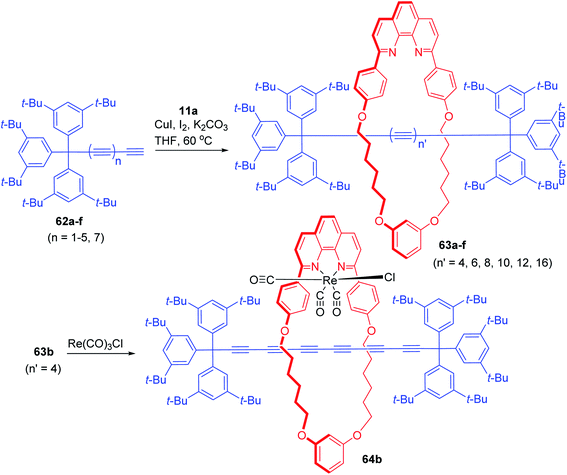 |
| Scheme 20 Synthesis of polyyne based [2]rotaxanes.25 | |
For stabilizing the polyynes, steric protection of sp carbon chain by terminal organometallic bulky end groups and subsequent tailoring into corresponding rotaxanes is one of the prominent strategies. In this direction, Gladysz and co-workers reported the synthesis of new rotaxane wherein the treatment of platinum butadiynyl complexes, 65a–c with the phenanthroline based macrocyclic copper complexes 11a or 11c gave a series of corresponding polyyne based rotaxanes, 66a–e (Scheme 21).26
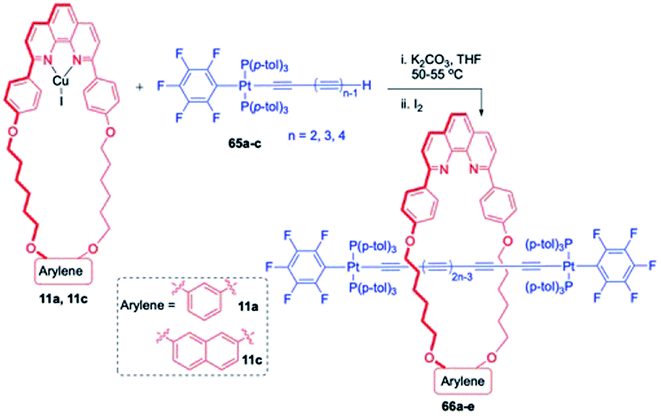 |
| Scheme 21 Synthesis of Pt thread based polyyne [2]rotaxanes.26 | |
After accomplishing the synthesis of polyyne based [2]rotaxanes, Anderson's group next conceptualized the synthesis of some interesting and highly stable cyclocarbons, a class of molecular entities that were studied earlier only in their gas phase. In light of this, synthesis of [5]rotaxane with p-phenylene spacers at the meso-positions and the corresponding porphyrin [3]rotaxanes was achieved (Scheme 22).27 The synthesis commenced from the phenanthroline based macrocyclic copper complexes bearing aldehyde functionality, 67a,b which upon Cadiot–Chodkiewicz coupling reaction between two axle components, 68 and 69 delivered the [2]rotaxane aldehydes, 70a,b. These rotaxanes were further treated with dipyrromethene 71, TFA, then 2,3-dichloro-5,6-dicyano-p-benzoquinone (DDQ) to deliver the porphyrin [3]rotaxane, 72a,b. In order to achieve the synthesis of [5]rotaxanes, macrocycle 70b was used which upon treatment with pyrrole furnished 73 (Scheme 23). All these porphyrin based [3]- and [5]rotaxanes were characterized as their respective zinc complexes. The photophysical interactions between the excited states of polyyne and porphyrin chromophores were investigated and the absorption spectra of Zn-rotaxane complexes showed that threading causes very little perturbation to the electronic structure of the polyyne component chromophores.
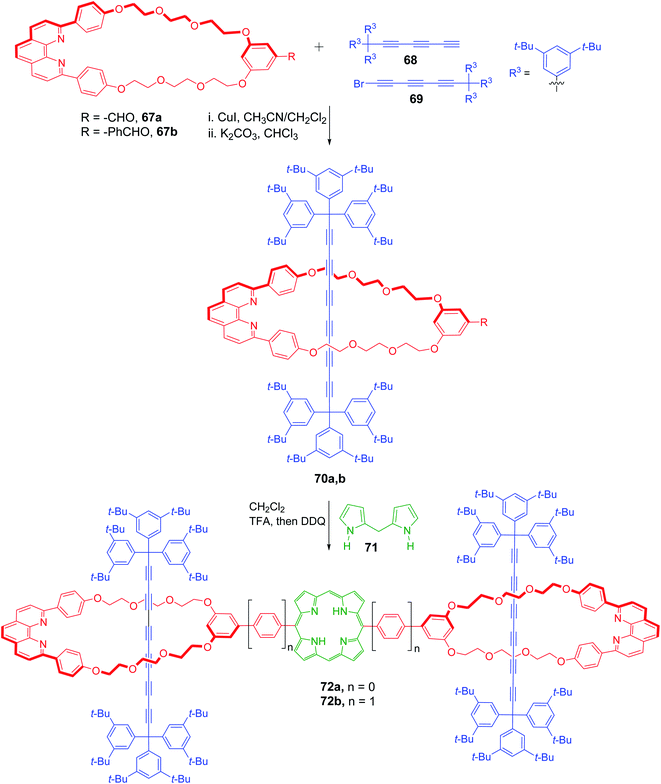 |
| Scheme 22 Synthesis of porphyrin based [3]rotaxanes.27 | |
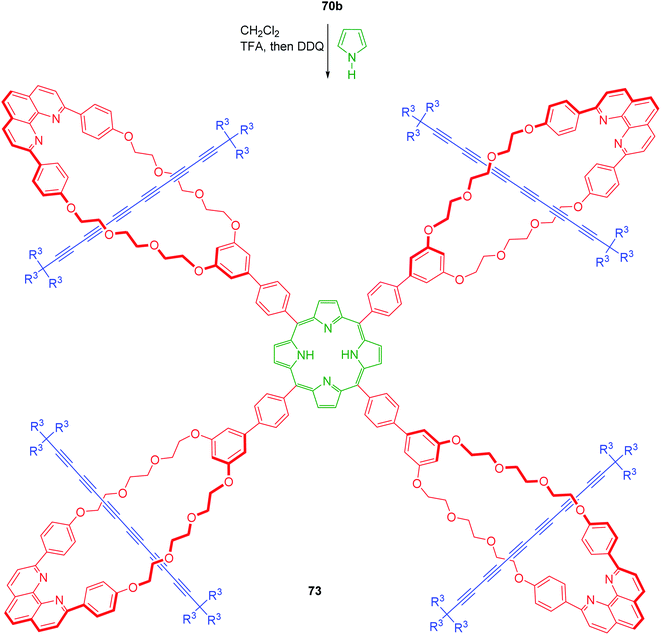 |
| Scheme 23 Synthesis of a porphyrin based [5]rotaxane.27 | |
Anderson's group reported an interesting strategy for the synthesis of a new class of polyyne based [2] and [3]rotaxanes bearing masked alkyne equivalents (MAE) as bulky stoppers which are large enough to prevent the dethreading of macrocyclic components (Scheme 24).28 An alkyne, 74 and a bromoalkyne, 75 with MAE were prepared from indane. Cadiot–Chodkiewicz coupling between 74 and 75 proceeded in presence of new macrocycle 76, CuI/K2CO3 and furnished the [2]rotaxane, 77. Subsequent deprotection with K2CO3/18-crown-6 resulted in the final [2]rotaxane, 78. This [2]rotaxane was the first example of an interlocked compound with masked alkyne as stopper.
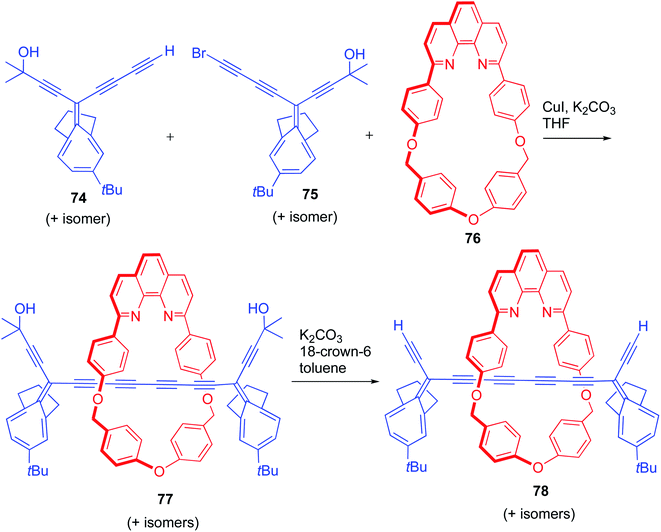 |
| Scheme 24 Synthesis of [2]rotaxane with MAEs.28 | |
A Similar strategy was applied for the synthesis of extended carbyne polyyne rotaxanes and in this direction initially an asymmetric [2]rotaxane, having a MAE stopper on one side and a bulky supertrityl stopper on the other side, was accessed using a Cadiot–Chodkiewicz coupling reaction between bromoalkyne 75 and supertrityl triyne 68 in presence of macrocycle 76 (Scheme 25).28 Removal of the acetonide protecting group under K2CO3/18-crown-6 reagent combination resulted in the deprotected [2]rotaxane, 79 having a modified axle component. The free acetylene functionality in 79 was efficiently harnessed and an oxidative Glaser homo-coupling, in presence of N,N,N′,N′-tetrametylethylenediamine (TMEDA) resulted in the MAE protected [3]rotaxane, 80. Subsequent photochemical unmasking of the functionalities relieved the long polyyne thread to furnish the corresponding [3]rotaxane which was detected in the final reaction mixture by ESI-MS and UV-visible spectroscopy.
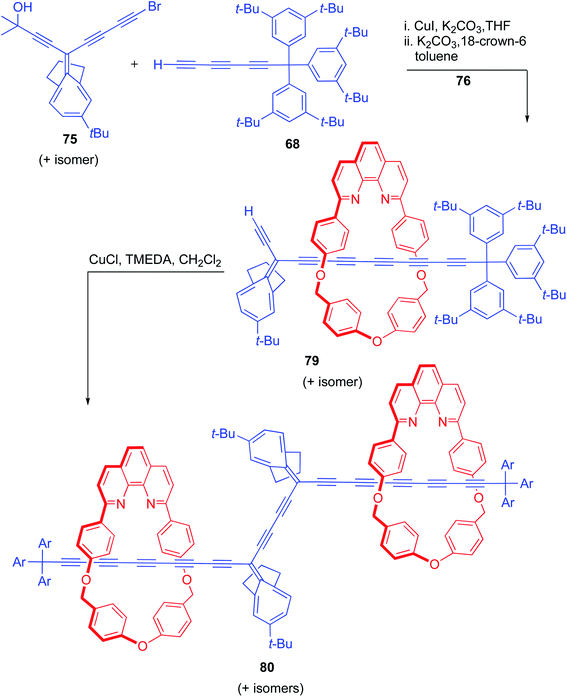 |
| Scheme 25 Synthesis of polyyne based [3]rotaxane with protecting groups.28 | |
Anderson and Tykwinski reported the synthesis of a new class of porphyrin–polyyne hybrid rotaxanes using copper mediated Cadiot–Chodkiewicz cross coupling (Scheme 26).29 Reaction of substituted porphyrin, 81 with supertrityl bromotriyne, 69 in presence of phenanthroline copper complex 11a furnished the [2]rotaxane 82. This rotaxane was in turn used as a template for its higher analogue, a [3]rotaxane 83. The triisopropylsilyl (TIPS) group removal by tetra-n-butylammonium fluoride (TBAF) released the terminal alkyne functionality which under similar reaction conditions as those for the first coupling reaction, yielded the porphyrin templated [3]rotaxane 83.
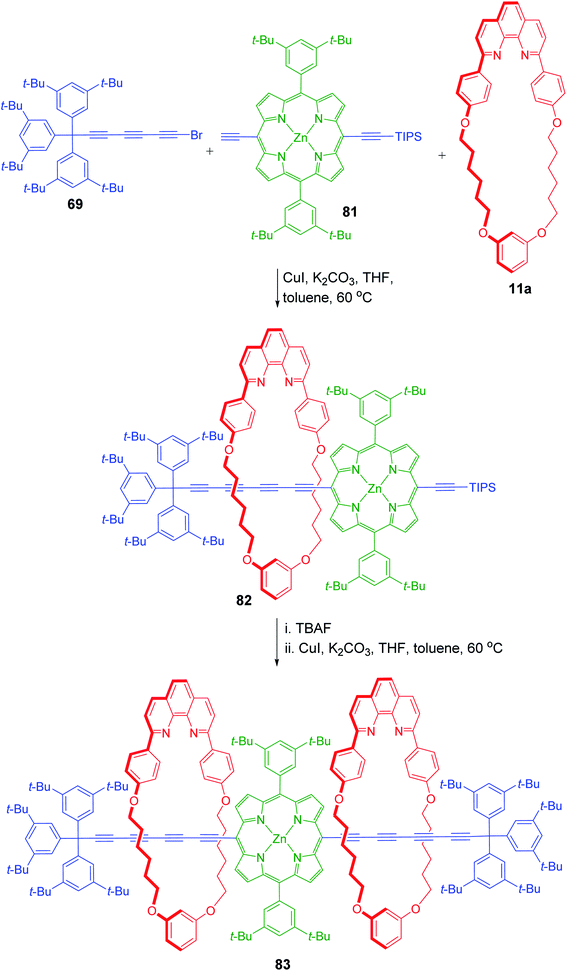 |
| Scheme 26 Synthesis of complex porphyrin based [3]rotaxane.29 | |
In another attempt, Anderson and co-workers used masked oligoynes for assembling polyyne threads in a protected form for rotaxane synthesis (Scheme 27).29 Polyyne precursors in the form of gem-dibromoolefins with supertrityl blocking groups (84 and 85) were treated with the macrocyclic copper complex, 11a which resulted in the formation of required rotaxane 86. The base mediated conversion of the protected rotaxane 86 to final target polyyne rotaxane under Fritsch–Buttenberg–Wiechell rearrangement did not work and therefore settlement was made for 86.
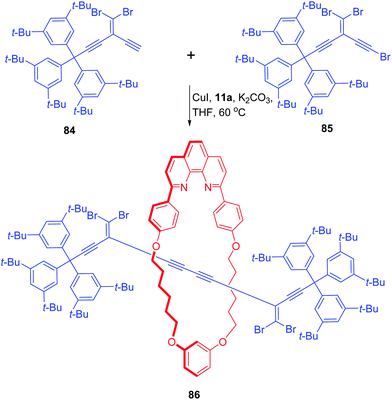 |
| Scheme 27 Synthesis of a new polyyne [2]rotaxane.29 | |
Another study was directed to synthesize a series of polyyne rotaxanes bearing different types of macrocyclic components (11a–g, Scheme 28).29 Triyne axle fragment with supertrityl end groups and bromotriyne derivatives were used as axle precursors. Phenanthroline based macrocycles were subjected to the threading reaction with triynes 68 and 69 under the standardized cross coupling conditions that yielded a series of hexyne rotaxanes, 63b, 87a–f in variable yields.
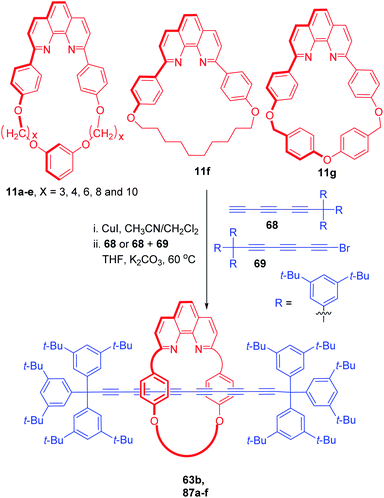 |
| Scheme 28 Synthesis of a series of polyyne [2]rotaxanes.29 | |
Hexyne rotaxanes (63b and 87b) with large alkyl bridges were further used as advanced templates for the synthesis of [3]rotaxanes, 88a,b (Scheme 29).29 For this purpose, [2]rotaxanes, 63b and 87b were treated again with the axle precursor under similar reaction conditions which resulted in the synthesis of [3]rotaxane 88a and 88b, although in low yield.
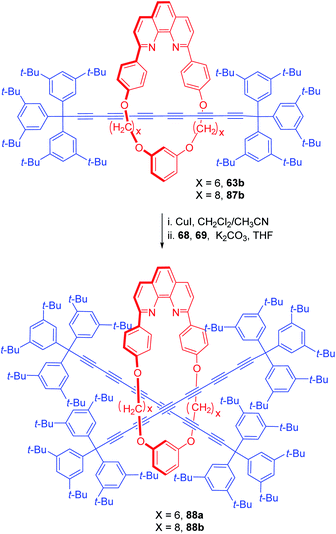 |
| Scheme 29 Synthesis of novel double polyyne [3]rotaxanes.29 | |
Tykwinski and co-workers delineated an excellent strategy for assembling [2] and [3]rotaxanes with polyyne threads (Scheme 30).30 This synthetic strategy commenced with the synthesis of macrocyclic metal complexes 89a,b followed by their treatment with acetylene bearing axle precursors (68 and 69) under Cadiot–Chodkiewicz reaction conditions to yield rotaxanes, 90a,b bearing a protected acetylene or iodine functionality for further derivatization.
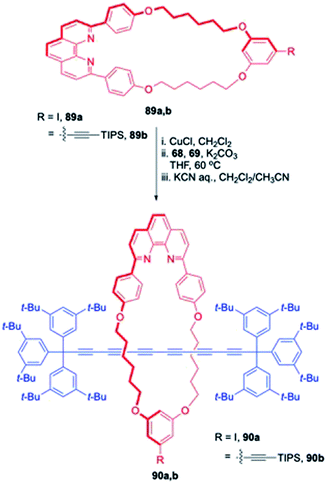 |
| Scheme 30 Synthesis of substituted polyyne [2]rotaxane.30 | |
TBAF mediated silyl deprotection in 90b exposed the terminal acetylene and yielded 91 (Scheme 31).30 Hay coupling of this [2]rotaxane, 91 in presence of TMEDA and CuCl finally furnished the required [3]rotaxane, 92 with two similar axle components threaded through a conjoined macrocyclic component.
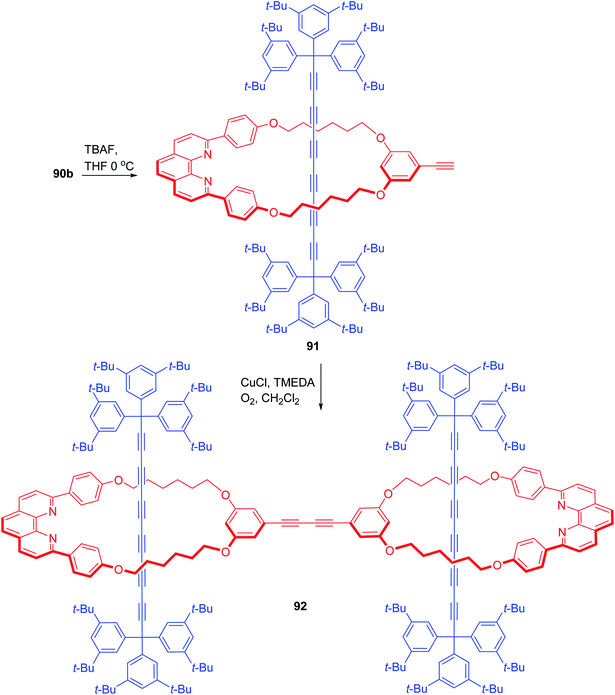 |
| Scheme 31 Synthesis of doubly polyyne based [3]rotaxane.30 | |
Next, it was envisioned to target the synthesis of [3]rotaxanes with mixed axels, one cumulene and another polyyne axel component (Scheme 32).30 In this direction, the known macrocycle with TIPS protected acetylene, 89b was subjected to Cadiot–Chodkiewicz reaction with acetylene fragments (93, 94) followed by silyl deprotection that delivered the [2]rotaxane, 95 bearing a shorter axel with four contagious acetylene units.
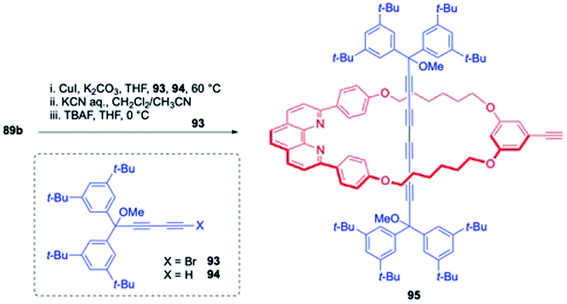 |
| Scheme 32 Synthesis of symmetrical polyyne based [2]rotaxane.30 | |
Sonogashira coupling of 95 with previously accessed [2]rotaxane 90a bearing iodo functionality resulted in a mixture of cross coupled (96) and homocoupled (97) products with homocoupled rotaxane as the dominant product (55%, Scheme 33).30 Finally, treatment of 96 with SnCl2/HCl completed the synthesis of mixed [3]rotaxane 98. Similarly, treatment of 97 also furnished the pure cumulene based [3]rotaxane 99 (Scheme 34).30
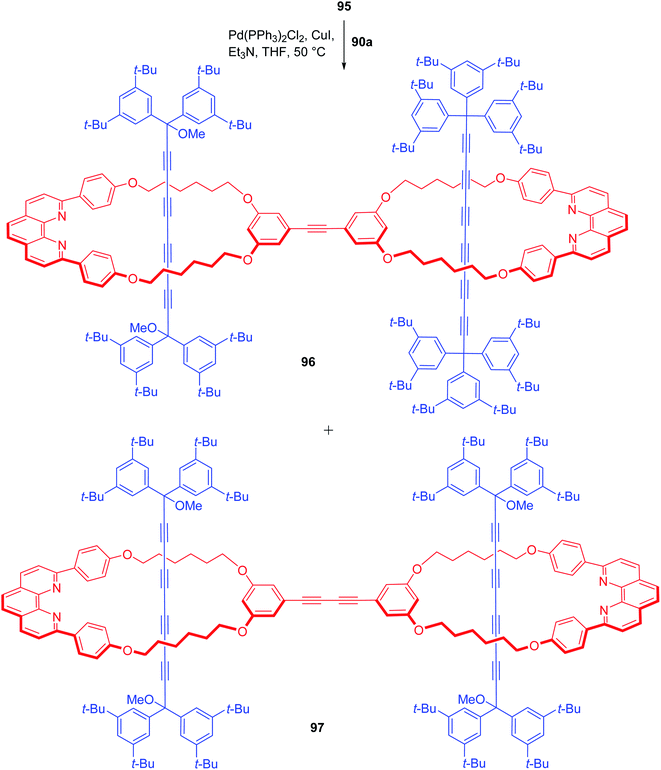 |
| Scheme 33 Synthesis of symmetrical/unsymmetrical polyyne based [3]rotaxanes.30 | |
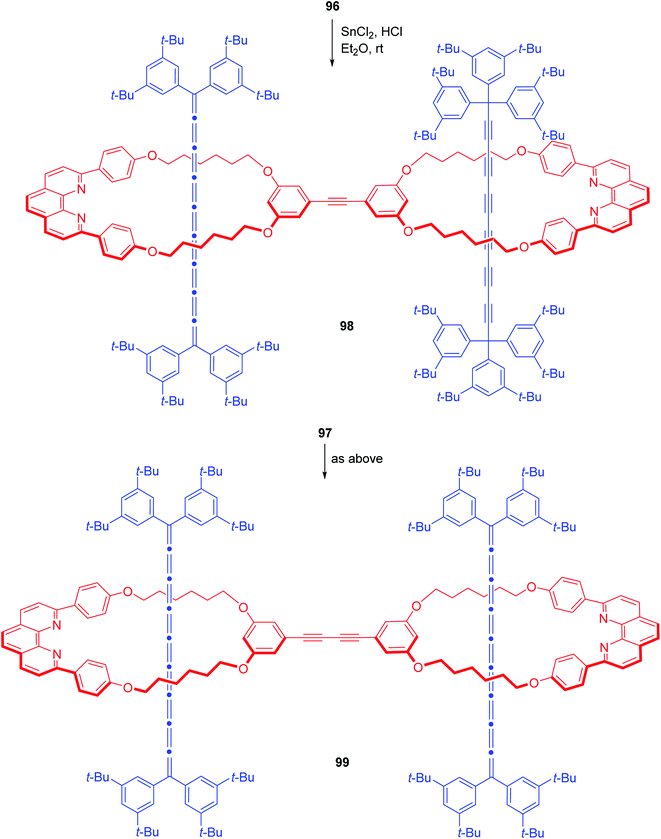 |
| Scheme 34 Synthesis of polyyne-cumulene hybrid [3]rotaxane.30 | |
[C] Synthesis of rotaxanes with miscellaneous axle moieties
In addition to above discussed Glaser and Cadiot–Chodkiewicz coupling reactions for the synthesis of diyne and polyyne rotaxanes, the catalytic activity of the macrocyclic phenanthroline copper(I) complexes has been utilized to synthesize rotaxanes with different structures of axle components. The Cu-mediated coupling reaction (Sonogashira type reaction) between aryl iodide, 100 and alkyne 45b in presence of macrocycle 11a proceeded at elevated temperature and rotaxane 101 was isolated (Scheme 35).31
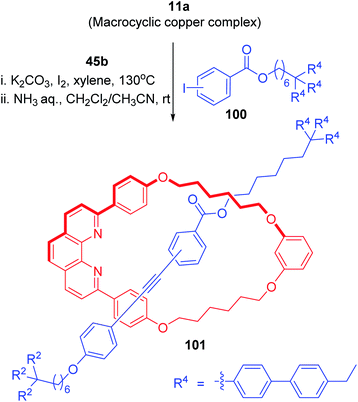 |
| Scheme 35 Synthesis of a [2]rotaxane by Sonogashira type coupling.31 | |
Similarly, a C–S bond forming reaction was utilized to synthesize [2]rotaxane with thiophenol axle (Scheme 36).4 The important bond-forming reaction between 102 and 103 proceeded selectively inside the macrocyclic complex 11a to deliver the required rotaxane 104 in robust manner.
 |
| Scheme 36 Synthesis of a [2]rotaxane with thiol axle component.4 | |
Ghosh et al. reported the synthesis of a triazole containing [2]rotaxane with a novel phenanthroline based heteroditopic macrocycle bearing ester and ether functionalities (Scheme 37).32 Treatment of this macrocyclic Cu-complex 40 with the azide and alkyne fragments (43, 105) which act as precursors for the axel component, under metal catalysis triggered the azide–alkyne click reaction resulting in the rotaxane-metal complex. Demetallation with EDTA solution finally delivered the rotaxane 106 in 27% yield.
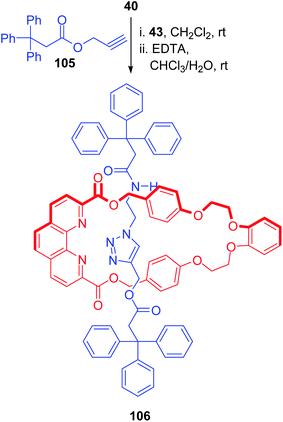 |
| Scheme 37 Synthesis of triazole rotaxane.32 | |
Muraoka and co-workers reported an efficient synthesis of a phenanthroline based [2]rotaxane by simultaneous induction of hydrogen bonding and π-interaction between the components (Scheme 38).33 This rotaxane, which incorporates the phenanthroline moiety in its axle component and an isophthalamide derived non-ionic macrocycle, was found to act as a reversible pH-controllable molecular switch. Synthesis of this rotaxane is straightforward and involves the treatment of the phenanthroline derivative with benzaldehyde moieties, 107 with the macrocyclic component 108 in CDCl3. This resulted in a pseudorotaxane intermediate which upon introduction of the stopper, tritylaniline 109 furnished the final [2]rotaxane 110 in 60% yield. Under the influence of excess TFA, protonation of the phenanthroline moiety takes place to deliver 111. This results in the migration of macrocycle component to protonated aniline site that renders it a potential molecular switch.
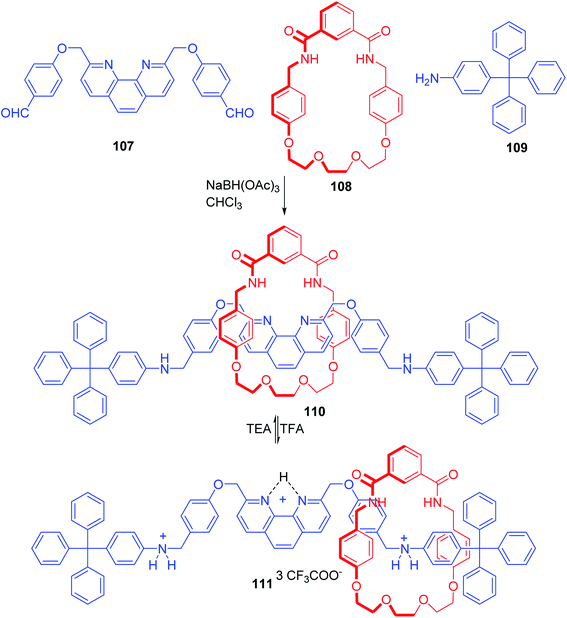 |
| Scheme 38 Synthesis of [2]rotaxane involving π–π stacking and hydrogen bond.33 | |
Summary and outlook
Rapid transition from attractive synthetic curiosities to potentially applicable molecular machines, the field of rotaxanes has witnessed extensive growth from last few decades. Many research groups have made quintessential strides that have opened new horizons for further advancements. Variable synthetic approaches for assembling different structural make up and hunt for application oriented designs is what has added diversity to this ever growing domain of material chemistry. Out of many sublasses, phenanthroline based rotaxanes have not only been central to the evolution of this field but are also expected to contribute substantially in attaining new horizons in near future. We hope this review will attract further research interests in this area of phenanthroline derived molecular rotaxanes.
Conflicts of interest
There are no conflicts to declare.
Acknowledgements
This work was supported by JSPS KAKENHI Grant Number JP19K05442 and The Science Research Promotion Fund.
Notes and references
- For selected reviews on interlocked compounds, see:
(a) C. O. Dietrich-Buchecker and J.-P. Sauvage, Chem. Rev., 1987, 87, 795 CrossRef CAS;
(b) J.-P. Sauvage, Acc. Chem. Res., 1990, 23, 319 CrossRef CAS;
(c) R. Hoss and F. Vögtle, Angew. Chem., Int. Ed., 1994, 33, 375 CrossRef;
(d) D. B. Amabilino and J. F. Stoddart, Chem. Rev., 1995, 95, 2725 CrossRef CAS;
(e) R. Jager and F. Vögtle, Angew. Chem., Int. Ed., 1997, 36, 930 CrossRef CAS;
(f) S. A. Nepogodiev and J. F. Stoddart, Chem. Rev., 1998, 98, 1959 CrossRef CAS PubMed;
(g) J.-P. Sauvage, Acc. Chem. Res., 1998, 31, 611 CrossRef CAS;
(h) F. M. Raymo and J. F. Stoddart, Chem. Rev., 1999, 99, 1643 CrossRef CAS PubMed;
(i) C. A. Schalley, K. Beizai and F. Vögtle, Acc. Chem. Res., 2001, 34, 465 CrossRef CAS PubMed;
(j) H. Tian and Q.-C. Wang, Chem. Soc. Rev., 2006, 35, 361 RSC;
(k) J. D. Crowley, S. M. Goldup, A.-L. Lee, D. A. Leigh and R. T. McBurney, Chem. Soc. Rev., 2009, 38, 1530 RSC;
(l) K. D. Hänni and D. A. Leigh, Chem. Soc. Rev., 2010, 39, 1240 RSC;
(m) J. E. Beves, B. A. Blight, C. J. Campbell, D. A. Leigh and R. T. McBurney, Angew. Chem., Int. Ed., 2011, 50, 9260 CrossRef CAS PubMed;
(n) E. A. Neal and S. M. Goldup, Chem. Commun., 2014, 50, 5128 RSC;
(o) M. Xue, Y. Yang, X. Chi, X. Yan and F. Huang, Chem. Rev., 2015, 115, 7398 CrossRef CAS PubMed;
(p) J. E. M. Lewis, M. Galli and S. M. Goldup, Chem. Commun., 2017, 53, 298 RSC;
(q) F. Niess, V. Duplan and J.-P. Sauvage, Chem. Lett., 2014, 43, 964 CrossRef CAS;
(r) M. Denis and S. M. Goldup, Nat. Rev. Chem., 2017, 01, 0061 CrossRef CAS;
(s) P. R. McGonigal, Supramol. Chem., 2018, 30, 782 CrossRef CAS;
(t) H.-Y. Zhou, Q.-S. Zong, Y. Han and C.-F. Chen, Chem. Commun., 2020, 56, 9916 RSC.
- For selected books on interlocked compounds, see:
(a) G. Schill, Catenanes, Rotaxanes and Knots; Academic Press, New York, 1971 Search PubMed;
(b) Molecular Catenanes, Rotaxanes and Knots, ed. J. P. Sauvage and C. Dietrich-Buchecker, Wiley-VCH, New York, 1999 Search PubMed;
(c) C. J. Bruns and J. F. Stoddart, The Nature of the Mechanical Bond: From Molecules to Machines, John Wiley & Sons, 2016 CrossRef.
- V. Aucagne, K. D. Hänni, D. A. Leigh, P. J. Lusby and D. B. Walker, J. Am. Chem. Soc., 2006, 128, 2186 CrossRef CAS PubMed.
- S. Saito, E. Takahashi and K. Nakazono, Org. Lett., 2006, 8, 5133 CrossRef CAS PubMed.
- C. Dietrich-Buchecker and J.-P. Sauvage, J. Am. Chem. Soc., 1984, 106, 3043 (Angew. Chem., Int. Ed., 1989, 28, 189) CrossRef CAS.
- C. O. Dietrich-Buchecker and J.-P. Sauvage, Tetrahedron Lett., 1983, 24, 5095 CrossRef CAS.
- C. Wu, P. R. Lecavalier, Y. X. Shen and H. W. Gibson, Chem. Mater., 1991, 3, 569 CrossRef CAS.
- J.-P. Chambron, V. Heitz and J.-P. Sauvage, J. Chem. Soc., Chem. Commun., 1992, 1131 RSC.
- S. Saito, E. Takahashi, K. Wakatsuki, K. Inoue, T. Orikasa, K. Sakai, R. Yamasaki, Y. Mutoh and T. Kasama, J. Org. Chem., 2013, 78, 3553 CrossRef CAS PubMed.
- R. Hayashi, Y. Mutoh, T. Kasama and S. Saito, J. Org. Chem., 2015, 80, 7536 CrossRef CAS PubMed.
- E. Coronado, P. Gaviña, J. Ponce and S. Tatay, Org. Biomol. Chem., 2014, 12, 7572 RSC.
- A. Coskun, M. Hmadeh, G. Barin, F. Gμndara, Q. Li, E. Choi, N. L. Strutt, D. B. Cordes, A. M. Z. Slawin, J. F. Stoddart, J.-P. Sauvage and O. M. Yaghi, Angew. Chem., Int. Ed., 2012, 51, 2160 CrossRef CAS PubMed.
- Y. Trolez, A. D. Finke, F. Silvestri, F. Monti, B. Ventura, C. Boudon, J.-P. Gisselbrecht, W. B. Schweizer, J.-P. Sauvage, N. Armaroli and F. Diederich, Chem.–Eur. J., 2018, 24, 10422 CrossRef CAS PubMed.
- A. Joosten, Y. Trolez, J.-P. Collin, V. Heitz and J.-P. Sauvage, J. Am. Chem. Soc., 2012, 134, 1802 CrossRef CAS PubMed.
- C. Roche, J.-P. Sauvage, A. Sour and N. L. Strutt, New J. Chem., 2011, 35, 2820 RSC.
- M. Nandi, S. Bej, S. Bhunia and P. Ghosh, ChemElectroChem, 2020, 7, 1038 CrossRef CAS.
-
(a) S. Saito, J. Inclusion Phenom. Macrocyclic Chem., 2015, 82, 437 CrossRef CAS;
(b) Y. Yamazaki, Y. Mutoh and S. Saito, Chem. Lett., 2017, 46, 904 CrossRef CAS.
- S. Saito, T. Ohkubo, Y. Yamazaki, T. Yokoyama, Y. Mutoh, R. Yamasaki and T. Kasama, Bull. Chem. Soc. Jpn., 2015, 88, 1323 CrossRef CAS.
- S. Saito, Y. Hirano, Y. Mutoh and T. Kasama, Chem. Lett., 2015, 44, 1509 CrossRef CAS.
-
(a) Y. Matsuoka, Y. Mutoh, I. Azumaya, S. Kikkawa, T. Kasama and S. Saito, J. Org. Chem., 2016, 81, 3479 CrossRef CAS PubMed;
(b) Y. Mochizuki, K. Ikeyatsu, Y. Mutoh, S. Hosoya and S. Saito, Org. Lett., 2017, 19, 4347 CrossRef CAS.
- R. Hayashi, Y. Mutoh, T. Kasama and S. Saito, J. Org. Chem., 2015, 81, 7536 CrossRef PubMed.
- R. Hayashi, P. Slavík, Y. Mutoh, T. Kasama and S. Saito, J. Org. Chem., 2016, 81, 1175 CrossRef CAS PubMed.
- Y. Yamashita, Y. Mutoh, R. Yamasaki, T. Kasama and S. Saito, Chem. - Eur. J., 2015, 21, 2139 CrossRef CAS PubMed.
- Y. Miyazaki, C. Kahlfuss, A. Ogawa, T. Matsumoto, J. A. Wytko, K. Oohora, T. Hayashi and J. Weiss, Chem.–Eur. J., 2017, 23, 13579 CrossRef CAS PubMed.
- L. D. Movsisyan, D. V. Kondratuk, M. Franz, A. L. Thompson, R. R. Tykwinski and H. L. Anderson, Org. Lett., 2012, 14, 3424 CrossRef CAS PubMed.
-
(a) N. Weisbach, Z. Baranová, S. Gauthier, J. H. Reibenspies and J. A. Gladysz, Chem. Commun., 2012, 48, 7562 RSC;
(b) H. Amini, Z. Baranova, N. Weisbach, S. Gauthier, N. Bhuvanesh, J. H. Reibenspies and J. A. Gladysz, Chem.–Eur. J., 2019, 25, 15896 CrossRef CAS PubMed.
- D. R. Kohn, L. D. Movsisyan, A. L. Thompson and H. L. Anderson, Org. Lett., 2017, 19, 348 CrossRef CAS PubMed.
- P. Gawel, S. L. Woltering, Y. Xiong, K. E. Christensen and H. L. Anderson, Angew. Chem., Int. Ed., 2021, 60, 5941 CrossRef CAS PubMed.
- L. D. Movsisyan, M. Franz, F. Hampel, A. L. Thompson, R. R. Tykwinski and H. L. Anderson, J. Am. Chem. Soc., 2016, 138, 1366 CrossRef CAS PubMed.
- M. Franz, J. A. Januszewski, F. Hampel and R. R. Tykwinski, Eur. J. Org. Chem., 2019, 3503 CrossRef CAS.
- K. Ugajin, E. Takahashi, R. Yamasaki, Y. Mutoh, T. Kasama and S. Saito, Org. Lett., 2013, 15, 2684 CrossRef CAS PubMed.
- M. Nandi, S. Bej, T. K. Ghosh and P. Ghosh, Chem. Commun., 2019, 55, 3085 RSC.
- M. Muraoka, K. Aoyama, S. Fujihara, R. Yamane, I. Hisaki, M. Miyata, M. Murata and Y. Nakatsuji, Symmetry, 2019, 11, 1137 CrossRef CAS.
|
This journal is © The Royal Society of Chemistry 2022 |
Click here to see how this site uses Cookies. View our privacy policy here.