DOI:
10.1039/D2RA01841A
(Paper)
RSC Adv., 2022,
12, 31046-31055
Understanding the role of 5d electrons in ferromagnetism and spin-based transport properties of K2W(Cl/Br)6 for spintronics and thermoelectric applications
Received
21st March 2022
, Accepted 2nd May 2022
First published on 31st October 2022
Abstract
In this article, we have systematically investigated the structural, electronic, magnetic, and spin-based thermoelectric properties of K2W(Cl/Br)6 by first-principles calculation. The obtained negative formation energy confirmed the thermodynamic stability of K2W(Cl/Br)6, while the tolerance factor calculation showed their cubic phase stability. In addition, we have estimated the elastic constants which confirmed the mechanical stability of K2W(Cl/Br)6. Further, the spin-polarized band structure and density of states calculations revealed the half-metallic nature with high Curie temperature (Tc) values of 613 K and 597 K for K2WCl6 and K2WBr6, respectively. Moreover, we have studied the temperature variation of thermoelectric properties such as kl, σ, ke, S, PF, and ZT. Such results showed that higher ZT values for spin-down channels are obtained from ultra-low ke, and high PF. Therefore, K2W(Cl/Br)6 are viable thermoelectric and spintronic materials.
1. Introduction
The advancement of spintronics and quantum computing technology has boosted the memory storage speed with multifunctional characteristics. This is a developing field which reduces magnetic chip size and enhances the memory speed by utilizing the electron spin and its charge.1,2 The advanced spintronic technology also has novel achievements in non-volatile magnetic random-access memory.3,4 These advancements can also be applied in electronic devices due to their low cost, faster data speed, and less power consumption.5–7 In addition, the recent quantum technology takes advantage of electronic spin states in the digital display rather than the charge states of a typical electronic operation. The development of giant magnetic resistance (GMR) in 1998 boosted this technology,8 where the electron spin can create an enormous difference in the resistance of alternative magnetic layers in the presence of external applied magnetic fields. Thus, the magnetic response of electronic charge and spin facilitate the low-powered, high-speed, non-volatile, and nano-size memory.9,10
The present scientific achievements of spintronics technology gave rise to the improvement of magneto-resistive random-access memory (MRAM), magnetic sensors, magnetic valves, read heads of magnetic hard drives, and giant magneto-resistive effect (GMR). Materials exhibiting the high spin polarization (SP) are suitable candidates for spintronics technology. Rather, for the half-metallic ferromagnetism (HMF) materials, one channel is metallic and the other one is insulating, which produces 100% SP, demonstrating that they are spintronics materials.11,12 The first HMF was observed by de Groot et al. in Heusler alloy PtMnSb and NiMnSb in 1983.13 After this, HMF was observed in many types of materials such as diluted magnetic semiconductors, perovskites, spinel chalcogenides, and double perovskites.14,15 But, the major confront of spintronic materials are their phase instability at elevated temperature and clustering of magnetic ions greatly influences the functionality of spin. In later research, the phase instability issue was resolved at higher temperature, but the problem of clustering remains to be addressed. Nonetheless, a lot of transition-metal doped alloys were formed at room temperature, still the issue of clustering limits their applications.16 To solve the problem of spin segregation, numerous procedures were adopted, where the doping of nonmagnetic elements into alloys is prominent. The FM behaviour has been reported in Be1−xCxS,17 Sn1−xMgxO2,18 etc. But these materials are expensive and have complicated production procurements.
Recently, Halide based double perovskites with chemical formula X2YZ6 (X = Cs, Rb, K Y = Os, Nb, Ta, W; Z = Cl, Br, I) have attracted considerable attention for spintronics applications due to their low cost, high Curie temperature (Tc), and good stability.19–23 In X2YZ6, the d-orbital electrons of Y atom contributes significantly to the magnetic moment. Furthermore, it has been noted that the halide based double perovskites have ferromagnetism at high Curie temperature. However, there is a lack of detailed description on the physical properties of X2YZ6. In this article, we have taken K2W(Cl/Br)6 compounds to investigate their magnetic and spin-based transport properties by first-principles calculation. These K2W(Cl/Br)6 compounds have been prepared by Xu et al. and Epperson et al. from a stoichiometric mixture of K(Cl/Br) and W(Cl/Br)4 and they have observed the cubic phase stability from the X-ray diffraction patterns.24,25 However, existing literatures about these compounds are only limited to their structural studies. To the best of our knowledge, there is no detailed report available on magnetic and spin-based transport properties of K2W(Cl/Br)6. Our spin-polarized band structure and density of states calculations revealed the presence of half-metallic character in these materials with high Curie temperature. Thus, K2W(Cl/Br)6 compounds are emerging spintronics materials.
Besides spintronics applications, the lead-free halide based double perovskites have attracted considerable attention for photovoltaic and thermoelectric technology. A good photovoltaic material should have high optical absorption coefficient and conductivity, with low reflectivity. On the other hand, a material is efficient for thermoelectric technology, if it has high Seebeck coefficient (S), good electrical conductivity (σ), and low thermal conductivity (k).26 Many lead-free halide based double perovskites are reported to have suitable photovoltaic and thermoelectric properties. For instance, Haq et al. predicted that Rb2XGaBr6 (X = Na, K) are promising photovoltaic and thermoelectric materials due to their optimum optical absorption coefficient and large ZT values, respectively.52 Later, Iqbal et al. have shown the emerging photovoltaic and thermoelectric properties of Rb2AlInX6 (X = Cl, Br, I) due to their narrow band gap.53 Also, Nawaz et al. reported that Rb2YInX6 (X = Cl, Br, I) are thermodynamically stable and they are suitable for photovoltaic and thermoelectric technology.54 Instead of low thermal conductivity arising from the occupation of cations in the octahedral structure, it is very surprising that these halide based double perovskites are mainly studied for photovoltaic purposes. Only very few experimental studies were performed to investigate their thermoelectric properties and interestingly the research in the thermoelectric response is now growing.27 In this article, we have carried out the spin-based thermoelectric properties of K2W(Cl/Br)6. We have computed the temperature variation of kl, σ, ke, S, PF, and ZT. The higher ZT values for spin-down channels have resulted from ultra-low ke, and high PF. Thus, K2W(Cl/Br)6 are potential thermoelectric and spintronic materials.
2. Computational details
The electronic structure, magnetic properties and transport properties of K2W(Cl/Br)6 were investigated by using Wien2k28 and BoltzTraP code.29 We have employed PBEsol approximation to optimize the crystal structure in FM and AFM states.30 However, PBEsol approximation underestimates the electronic bandgap on which magnetic and transport properties are dependent. Therefore, we have employed TB-mBJ formalism,31 which can accurately predict the bandgap. In addition, due to the presence of heavy elements, SOC coupling is significant, that was added with TB-mBJ. The energy cut-off for geometry optimization was selected to be 520 eV. On the other hand, the average forces per ions were optimized to 0.002 eV Å−1. Furthermore, we have considered a k mesh of 12 × 12 × 12 for electronic calculation. The product of maximum wave vector and muffin tin radii is kept as RMT × Kmax = 8, along with angular momentum vector l = 10 and Gaussian factor = 10. The level convergence is achieved to be 10−5 Ry self consistently by using the above-mentioned inputs. For transport properties calculation, we have considered a dense k-points of 150
000.
3. Results and discussion
3.1 Structural and mechanical stabilities
The halide based double perovskites K2W(Cl/Br)6 have a cubic phase with space group Fm
m (225).32 The perspective view of K2W(Cl/Br)6 is shown in Fig. 1. The vacancies between the octahedra W(Cl/Br)6 are occupied by K atoms whereas each octahedron is separated by the other octahedra through the 12-fold coordination system of (Cl/Br). In this structure, each K atom is surrounded by 12 (Cl/Br) atoms, whereas every W atom is coordinated with 6 (Cl/Br) atoms. Moreover, each W(Cl/Br)6 is located at the corner and face center of the cubic system. The K, W, and (Cl/Br) atoms in the unit cells of both systems are positioned at (0.25, 0.25, 0.25) (0, 0, 0) and (x, 0, 0), respectively. The atomic positions in the structure are corrected by minimizing strain throughout the optimization process.
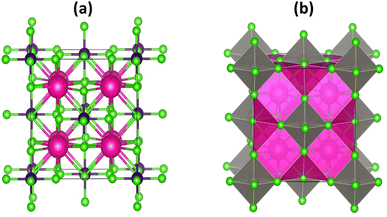 |
| Fig. 1 The crystal structure (a) atomic and (b) polyhedral forms of K2W(Cl/Br)6 with magenta, blue and green colors represent the K, W, and (Cl/Br) atoms respectively. | |
The optimized energy versus volume plot is shown in Fig. 2(a and b). It is noticeable from Fig. 2(a and b), that K2W(Cl/Br)6 compounds have positive energy difference between FM and AFM states indicates that the FM is more preferable because of more energy release in this process.
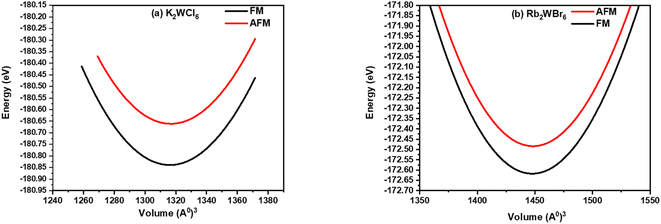 |
| Fig. 2 Volume optimization plot of (a) K2WCl6 and (b) K2WBr6 in FM (black line) and AFM (red line) calculated through PBEsol approximation. | |
Curie temperature (Tc) of K2W(Cl/Br)6 are predicted through the Heisenberg classical model. e., Tc = 2ΔE/3xKB, where x is the contribution of W atom and ΔE is the energy difference between ferromagnetic and antiferromagnetic ground states, i.e. ΔE = EAFM − EFM.33,34 The computed Tc values are 613 K and 597 K for K2WCl6 and K2WBr6, respectively. The high Tc values make these compounds suitable for spintronic applications.
The cubic phase stability of K2W(Cl/Br)6 are investigated from Goldsmith tolerance fact or calculation as follows.
|
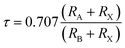 | (1) |
It is worthy to mention here that, the stable cubic phase should have τ value in the range of 0.9–1.02.35 Table 1 clearly shows that τ values of K2W(Cl/Br)6 are in this range demonstrating their cubic phase stability.
Table 1 The obtained lattice parameter (a), tolerance factor (τ), octahedral factor (μ), and formation energy (ΔHf) of K2W(Cl/Br)6
Compound |
a (Å) |
τ |
μ |
ΔHf (eV) |
K2WCl6 |
10.71 |
0.98 |
0.45 |
−31.12 |
K2WBr6 |
10.75 |
0.98 |
0.44 |
−27.12 |
To investigate the synthetic possibility of K2W(Cl/Br)6, we have calculated the enthalpy of formation by using the following equation
|
ΔHf = Etotal{K2W(Cl/Br)6)} − 2EbulkK − EbulkW − 6Ebulk(Cl/Br)
| (2) |
where,
Etotal{K
2W(Cl/Br)
6},
EbulkK,
EbulkW and
Ebulk(Cl/Br) represent the ground state energy of K
2WX
6, K, W, and (Cl/Br) in their bulk form. The predicted values of Δ
Hf are −31.12 eV and −27.12 eV for K
2WCl
6 and K
2WBr
6, respectively. This negative Δ
Hf revealed the thermodynamic stability of K
2W(Cl/Br)
6.
The mechanical stability was studied from elastic constant values (Cij), obtained by using the Cubic Elastic package.56 It is observed from Table 2 that the values of the various components of Cij obeyed the Born criteria of mechanical stability, i.e. C11 − C12 > 0, C44 > 0, C11 + 2C12 > 0, C12 < B < C11.37 Furthermore, we have estimated the Cauchy's pressure (CP = C12–C44). The positive value of CP demonstrated the ductile properties of K2W(Cl/Br)6. The overall elastic study confirmed that K2W(Cl/Br)6 are mechanically stable.36
Table 2 The calculated bulk modulus (B), elastic constants (Cij), and cauchy pressure (CP) of K2W(Cl/Br)6
Compound |
B (GPa) |
C11 |
C12 |
C44 |
CP |
K2WCl6 |
41.16 |
78.19 |
21.17 |
18.72 |
2.45 |
K2WBr6 |
39.33 |
74.89 |
20.76 |
18.41 |
2.35 |
3.2 Magnetic properties
The half-metallic ferromagnetic material having high spin polarization (P) is essential for spintronic applications. The spin polarization (P) can be obtained as |
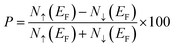 | (3) |
where (N↑) and (N↓) represent the density of states of up and down the channels at Fermi level (EF). The spin-polarized band structure is shown in Fig. 3. It is noticeable from Fig. 3 that, the quantum state of the spin-up channel overlapped with Fermi level (EF), which demonstrates the metallic nature. However, the spin-down channel shows the insulating nature because of the existence of finite separation between valence band maxima (VBM) and conduction band minima (CBM). Therefore, the combined spin up and spin down showed the half-metallic ferromagnetism nature with 100% spin polarization (P = 1). The detailed investigation revealed that the valence band maxima (VBM) and conduction band minima (CBM) of spin-down channels are located at the same k-point for both compounds, which indicates the direct band gap properties. We have obtained the band gap of 3.01 eV and 2.72 eV for K2WCl6 and K2WBr6, respectively in the spin-down channel. Also, the estimated total magnetic moment is calculated to be 2μB for both compounds. The integer value of total magnetic moment implies that K2W(Cl/Br)6 are half metallic ferromagnets. Thus, K2W(Cl/Br)6 are emerging spintronics materials.
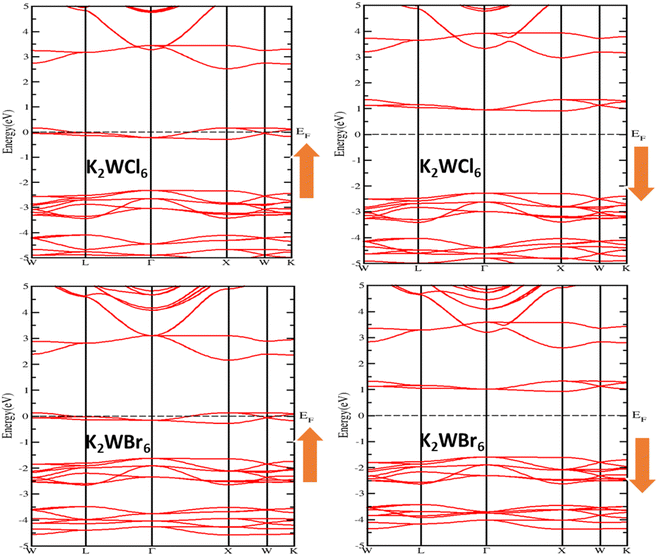 |
| Fig. 3 The spin-polarized band structure of K2W(Cl/Br)6 calculated through mBJ + SOC. | |
For the detailed investigation of band structure results, we have further calculated the total and partial density of states of K2W(Cl/Br)6 as shown in Fig. 4(a–d). It is noticeable from Fig. 4(a and b) that the total DOS in the up spin showed the metallic behavior and the down spin presented the insulating behavior. Therefore, K2W(Cl/Br)6 are half-metallic ferromagnetism (HMF) in nature. To investigate the origin of half-metallic ferromagnetism (HMF) in K2W(Cl/Br)6, we have further investigated the partial density of states (PDOS) which is shown in Fig. 4(c and d). It is evident from Fig. 4(c and d) that the d-t2g states of W atoms are responsible for introducing HMF in K2W(Cl/Br)6. In the spin-up case, d-t2g states of W atoms overlapped with Fermi level (EF), which produces the metallic nature in K2W(Cl/Br)6. In the spin-down case, d-t2g states move deep into the conduction band and thereby leaving a finite separation between the valence band and conduction band and therefore create insulating nature in K2W(Cl/Br)6.
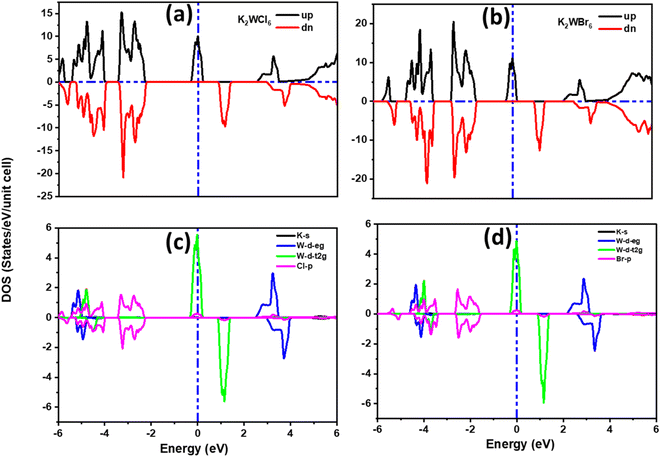 |
| Fig. 4 The spin polarized (a and b) total DOS, (c and d) partial DOS of K2W(Cl/Br)6 obtained with mBJ + SOC. | |
The magnetic behavior of K2W(Cl/Br)6 has also been addressed by an exchange mechanism. The crystal field due to the Coulomb interaction between W and (Cl/Br) atoms split the 5d states of W into t2g and eg. The splitting of W-5d states is schematically shown in Fig. 5. It is clearly noticeable from Fig. 5 that the t2g is triply degenerate (dxy, dyz, dzx), which has lower energy than the double degenerate eg (dz2, dx2 − y2) states. The splitting of eg states results in the upward and downward shifting of dx2 − y2 and dz2, respectively. At the same time, splitting of t2g state results in the increase of dxy state energy and decrease of dyz and dzx states energies. The major magnetic response originated from the t2g state of the W atom. This state is hybridized with the p state of (Cl/Br) via double exchange mechanism. Thus, we conclude that the double exchange mechanism between W atoms via (Cl/Br) atom is responsible for introducing HMF in K2W(Cl/Br)6.
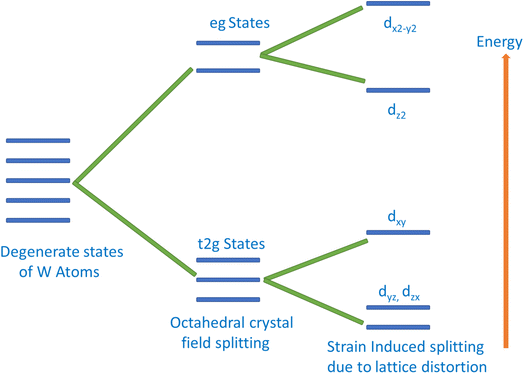 |
| Fig. 5 Schematic representation of crystal-field splitting of W-5d orbital. | |
3.3 Thermoelectric properties
Solid-state thermoelectric materials are gaining considerable attraction as novel materials for converting thermal to electrical energy.38–42 Many mechanical and electronic devices release a huge amount of heat as waste. To take the advantage of wasted heat, efficient thermoelectric materials are needed which can directly convert the wasted heat into electricity. The thermoelectric performance of a material is given by a dimensionless parameter known as a figure of merit (ZT) given by;
, where σ, S, T, ke, and kl represent the electrical conductivity, Seebeck coefficient, temperature, electrical thermal conductivity, and lattice thermal conductivity, respectively. The material with a large ZT value is considered to be a potential candidate for thermoelectric technology. But, obtaining a large ZT value is challenging due to the strong correlation of these physical properties. According to a recent report, magnetic interaction is one of the suitable approaches to enhance ZT.43–47 The interaction of charge carriers with local magnetic moments, can enhance the carrier effective mass (m*), consequently thermoelectric power (S2σ) and corresponding figure of merit (ZT). It is worthy to mention here that such magnetic interaction is present in K2W(Cl/Br)6. Due to the presence of magnetic interaction in K2W(Cl/Br)6, it is expected that these systems will have large ZT values. However, there are no detailed studies on thermoelectric properties of K2W(Cl/Br)6.
In this article, we have calculated the temperature variation of thermoelectric properties in K2W(Cl/Br)6. For this, we have employed BoltzTrap code.24 During all calculations, the relaxation time (τ) is fixed by BoltzTraP code as τ = 10−14 s. We have separately calculated the thermoelectric parameters for spin-up and spindown configurations.
For thermoelectric calculation, the temperature was varied from 200 K to 600 K. The total thermal conductivity (k) is the sum of electronic (ke) and lattice (kl) thermal conductivities. A good thermoelectric material should have low k. The temperature variation of lattice thermal conductivity (kl) was calculated by using the Slack equation.55 The temperature variation of kl is shown in Fig. 6. It can be seen from Fig. 6 that kl has an inverse relationship with temperature, which is a typical feature of half-metallic ferromagnetic materials.48 It is worthy to mention here that K2W(Cl/Br)6 compounds have low kl values.
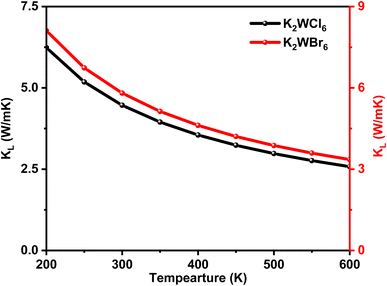 |
| Fig. 6 The temperature-dependent lattice thermal conductivity (Kl) of K2W(Cl/Br)6 calculated through the Slack equation. | |
The amount of charge flow per unit time inside of a compound can be understood from its electrical conductivity (σ). The materials are categorized into insulator, semiconductor, and metal-based on their ability of charge flow.49 A good thermoelectric material should have a large σ value.50 The temperature variation of σ is calculated for K2W(Cl/Br)6 as shown in Fig. (a) and Fig. 8(a), respectively. The detailed analyses revealed that the σ value for spin-up configurations in K2W(Cl/Br)6 decreases with temperature until achieving the lowest value of 1.97 × 105 Ω−1 m−1 (K2WCl6) and 2.25 × 105 Ω−1 m−1 (K2WBr6) at 600 K. On the other hand, the values of σ for spin-up states in K2W(Cl/Br)6 are almost constant in the entire temperature up to 400 K. However, there was a gradual increase of σ is observed above 400 K. This trend of σ for up and down channels is typical feature in HMF.51 The detailed investigation reveals that spin-up channel σ is dominant in both cases.
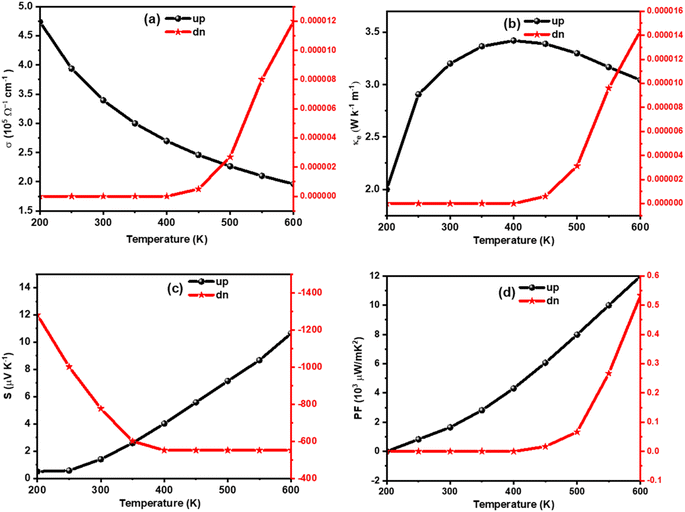 |
| Fig. 7 Calculated (a) σ, (b) k, (c) S, and (d) PF of K2WCl6 as a function of temperature. | |
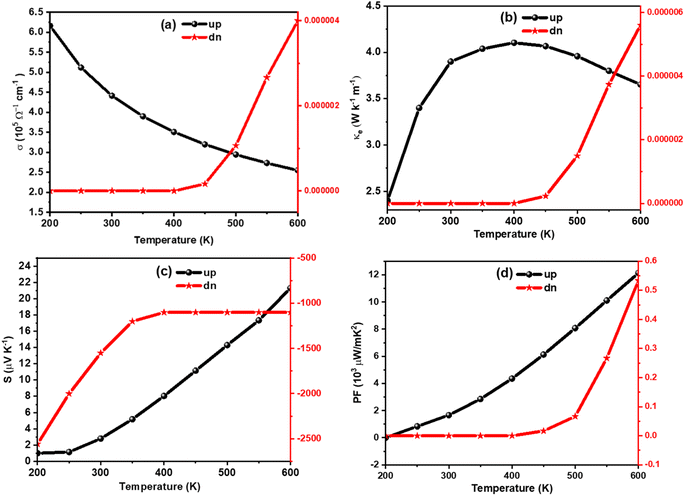 |
| Fig. 8 Calculated (a) σ, (b) k, (c) S, and (d) PF of K2WBr6 as a function of temperature. | |
We have also examined the temperature variation of electronic thermal conductivity (ke) as shown in Fig. 7(b) and 8(b) for K2WCl6 and K2WBr6, respectively. It is noticeable that ke for spin-up configuration has a direct relation with temperature up to a certain limit where ke increases to 3.41 W K−1 m−1 and 4.1 2 W K−1 m−1 respectively for K2WCl6 and K2WBr6, at 400 K. Above this temperature, ke decreases gradually for both systems. On the other hand, the values ke in spin-down states of K2W(Cl/Br)6 are 0.0 in the entire temperature up to 400 K. Above this temperature, ke increases abruptly in both cases. It is worthy to mention here that ke values for spin-down channels are very less compared to spin-up configuration.
The Seebeck coefficient (S) plays an important role to describe the thermoelectric performance. The S is defined by the ratio of a voltage difference to that of a temperature difference. It can also show the capability of a material to generate the thermo-electromotive force from a given temperature gradient. The S can be calculated by the following relation
|
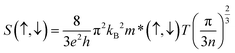 | (4) |
where
h,
kB,
e, m*(↑,↓),
T, and
n represent the Planck constant, Boltzmann constant, electronic charge, spin-dependent carrier effective mass, absolute temperature, and carrier concentration respectively. A good thermoelectric material should have a large Seebeck coefficient.
Fig. 7(c) and
8(c) presented the Seebeck coefficients for both compounds in both spin configurations as a function of temperature. It is noticeable from
Fig. 7(c) and
Fig. 8(c) that the obtained values of Seebeck coefficients are positive in the entire temperature range of spin up channels, demonstrating the presence of p-type charge carriers (hole), whereas negative values Seebeck coefficients for a spin up channel suggest the presence n-type charge carriers (electron). The absolute value of S for spin up configuration in K
2WCl
6 (K
2WBr
6) increases from 0.67 μV K
−1 (1.01 μV K
−1) at 200 K linearly up to 600 K. This increasing trend in up channel is due to its metallic nature. For metal, there is huge number of free electrons and hence applying a temperature gradient should lead to diffusion of more charge carriers towards cold end. As a result, the potential difference between two ends will increase and therefore
S will increase. In the spin-down channel, there is an abrupt decrease of
S in K
2WCl
6 (K
2WBr
6) from a very high value of 1267 μV K
−1 (1397 μV K
−1) at 200 K beyond this temperature
S decreases gradually. This, decrease trend in spin-down channel can be described from
eqn (4). The increasing of temperature can enhance the carrier concentration and thereby decreasing the value of
S. The detailed investigation depicts that spin down channel Seebeck coefficient is dominant in both cases.
In addition, we have calculated the thermoelectric power factor (PF) as shown in Fig. 7(d) and Fig. 8(d). It is noticeable that the PF of spin-up configuration in K2WCl6 (K2WBr6) increases from 0.02 × 109 W mK−1 (0.01 × 109 W mK−1) at 200 K linearly up to 600 K. On the other hand, the PF of spin-down state is found to be 0.0 μW mK−2 for both systems in the entire temperature up to 400 K. Beyond this temperature, the PF increases gradually.
Motivated by large S, σ, and PF with low ke, we have calculated the temperature variation of thermoelectric figure of merit (ZT) as Fig. 9(a and b). The detailed analysis revealed that the ZT for spin upstate in K2WCl6 (K2WBr6) increases from 0.001 (0.001) at 200 K to 0.05 (0.041) at 600 K. On the other hand, ZT values of a spin-down channel in K2WCl6 (K2WBr6) gradually decrease from 0.99 (0.884) at 200 K to 0.95 (0.845) at 600 K. It is noticeable that spin down channel ZT value is dominant in both cases. The higher ZT value of the spin-down channel is due to its very low thermal conductivity. Also, the large ZT values demonstrated that K2W(Cl/Br)6 are potential thermoelectric materials.
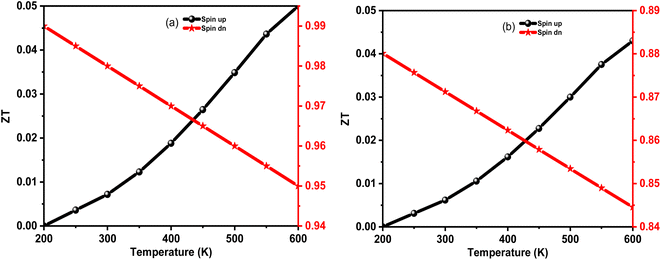 |
| Fig. 9 The calculated ZT value of (a) K2WCl6 and (b) K2WBr6 as a function of temperature. | |
4. Conclusion
To summarize this article, we have systematically investigated the structural, electronic, and thermoelectric properties of K2W(Cl/Br)6. The negative formation energy leads to the thermodynamic stability of K2W(Cl/Br)6. In addition, the spin-polarized band structure and density of states calculations revealed the presence of half-metallic character with higher Tc values, which are 613 K and 597 K for K2WCl6 and K2WBr6, respectively. Thus, K2W(Cl/Br)6 are potential candidates for spintronics application. Furthermore, the origin of half-metallic ferromagnetism is discussed with the double-exchange mechanism. Finally, we have computed the temperature variation of kl,σ, ke, S, PF, and ZT. The higher ZT values for spin-down channels have resulted from ultra-low ke, and high PF. In short, K2W(Cl/Br)6 are potential thermoelectric and spintronic materials.
Data availability
The datasets produced for current study would be available from Mr Mukaddar Sk on reasonable request.
Conflicts of interest
The authors have no conflict of interest.
Acknowledgements
Mr Mukaddar Sk would like acknowledge CSIR-HRDG for fellowship grant 09/1045(0041)/2019-EMR-I.
References
- T. Dietl, H. Ohno, F. Matsukura, J. Cibert and E. D. Ferrand, Zener model description of ferromagnetism in zinc-blende magnetic semiconductors, Science, 2000, 287, 1019 CrossRef CAS PubMed.
- Y. Huai, F. Albert, P. Nguyen, M. Pakala and T. Valet, Observation of spin-transfer switching in deep submicron-sized and low-resistance magnetic tunnel junctions, Appl. Phys. Lett., 2004, 84, 3118 CrossRef CAS.
- C. Chappert C, A. Fert, F. Nguyen and V. Dau, The emergence of spin electronics in data storage, Nat. Mater., 2007, 6, 813 CrossRef.
- M. C. Prestgard, G. P. Siegel and A. Tiwari, Oxides For Spintronics: A Review Of Engineered Materials For Spin Injection, Adv. Mater. Lett., 2014, 5, 242 CrossRef.
- X. Li and J. Yang, First-principles design of spintronics materials, Natl. Sci. Rev., 2016, 3, 365 CrossRef CAS.
- S. Assefa, J. Nowak, J. Sun, E. O'Sullivan, S. Kanakasabapathy, W. Gallagher, Y. Nagamine, K. Tsunekawa, D. Djayaprawira and N. Watanabe, Fabrication, and characterization of MgO-based magnetic tunnel junctions for spin momentum transfer switching, J. Appl. Phys., 2007, 102, 063901 CrossRef.
- K. L. Wang, J. G. Alzate and P. K. Amiri, Low-power non-volatile spintronic memory: STT-RAM and beyond, J. Phys. D: Appl. Phys., 2013, 46, 074003 CrossRef CAS.
- M. N. Baibich, J. M. Broto, A. Fert, F. N. van Dau, F. Petro, P. Eitenne, G. Creuzet, A. Friederich and J. Chazelas, Giant magnetoresistance of (001) Fe/(001)Cr magnetic superlattices, Phys. Rev. Lett., 1988, 61, 2472 CrossRef CAS PubMed.
- S. A. Wolf, D. D. Awschalom, R. A. Buhrman, J. M. Daughton, S. V. Molnár, M. L. Roukes, A. Y. Chtchelkanova and D. M. Treger, Spintronics: a spin-based electronics vision for the future, Science, 2001, 294, 1488 CrossRef CAS PubMed.
- J. Puebla, J. Kim, K. Kondou and Y. Otani, Spintronic devices for energy-efficient data storage and energy harvesting, Commun. Mater., 2020, 1, 24 CrossRef.
- W. E. Pickett and D. J. Singh, Electronic structure and half-metallic transport in the La1−x CaxMnO3 system, Phys. Rev. B: Condens. Matter Mater. Phys., 1996, 53, 1146 CrossRef CAS.
- D. J. Singh and W. Pickett, Pseudogaps Jahn–Teller distortions, and magnetic order in manganite perovskites, Phys. Rev. B: Condens. Matter Mater. Phys., 1998, 57, 88 CrossRef CAS.
- R. De Groot, F. Mueller, P. Van Engen and K. Buschow, New class of materials: half-metallic ferromagnets, Phys. Rev. Lett., 1983, 50, 2024 CrossRef CAS.
- Q. Lu, B. Wang, X. R. Chen and W. M. Liu, Robust large-gap quantum spin Hall insulators in methyl functionalized III-Bi buckled honeycombs, Phys. Rev. Mater., 2018, 2, 014005 CrossRef CAS.
- X. L. Zhang, L. F. Liu and W. M. Liu, Quantum anomalous Hall effect and tunable topological states in 3d transition metals doped silicene, Sci. Rep., 2013, 3, 2908 CrossRef PubMed.
- T. Dietl, Origin and control of ferromagnetism in dilute magnetic semiconductors and oxides, J. Appl. Phys., 2008, 103, 07D111 CrossRef.
- W. Akbar and S. Nazir, Origin of p-type half-metallic ferromagnetism in carbon-doped BeS: first-principles characterization, J. Alloys Compd., 2018, 743, 83 CrossRef CAS.
- C. W. Zhang and S. S. Yan, First-principles study on ferromagnetism in Mg-doped SnO2, Appl. Phys. Lett., 2009, 95, 232108 CrossRef.
- R. Ullah, M. A. Ali, G. Murtaza, A. Khan and A. Mahmood, Ab initio study for the structural, electronic, magnetic, optical, and thermoelectric properties of K2OsX6 (X Cl, Br) compounds, Int. J. Energy Res., 2020, 44, 9035 CrossRef CAS.
- M. A. Ali, G. Murtaza, A. Khan, E. Algrafy, A. Mahmood and S. M. Ramay, Magnetoelectronic properties of ferromagnetic compounds Rb2TaZ6 (Z Cl, Br) for possible spintronic applications, Int. J. Quantum Chem., 2020, 120, e26357 CAS.
- M. Faizan, S. H. Khan, G. Murtaza, A. Khana and A. Laref, Electronic and magnetic properties of alkali metal chlorides A2MCl6 (A = K, Rb, Cs; M = Mn, Mo): A density functional theory study, Int. J. Mod. Phys. B, 2019, 33, 1950072 CrossRef CAS.
- M. Faizan, S. H. Khan, A. Khan, A. Laref and G. Murtaza, Ab-initio prediction of structural, electronic and magnetic properties of Hexafluoromanganete(IV) complexes, Int. J. Mod. Phys. B, 2018, 32, 1850270 CrossRef CAS.
- M. A. Ali, G. Murtaza and A. Laref, Exploring ferromagnetic half-metallic nature of Cs2NpBr6 via spin polarized density functional theory, Chin. Phys. B, 2020, 29, 066102 CrossRef CAS.
- M. G. Brik and I. V. Kityk, Modeling of lattice constant and their relations with ionic radii and electronegativity of constituting ions of A2XY6 cubic crystals (A = K, Cs, Rb, Tl; X = tetravalent cation, Y = F, Cl, Br, I), J. Phys. Chem. Solids, 2011, 72(11), 1256–1260, DOI:10.1016/j.jpcs.2011.07.016.
- E. R. Epperson, The Binary Halides Of Molybdenum(IV) And Tungsten(IV) and The Oxochlorides Of Tungsten(VI), 1965, https://scholarlycommons.pacific.edu/cgi/viewcontent.cgi?article=3864%26context=uop_etds Search PubMed.
- S. R. Bhandari, D. K. Yadav, B. P. Belbase, M. Zeeshan, B. Sadhukhan, D. P. Rai, R. K. Thapa, G. C. Kaphle and M. P. Ghimire, Electronic, magnetic, optical and thermoelectric properties of Ca2Cr1−xNixOsO6 double perovskites, RSC Adv., 2020, 10, 16179 RSC.
- M. Nabi and D. C. Gupta, Potential lead-free small bandgap halide double perovskites Cs2CuMCl6 (M = Sb, Bi) green technology, Sci. Rep., 2021, 11, 12945 CrossRef CAS.
- P. Blaha, K. Schwarz, G. K. H. Madsen, D. Kvasnicka, and J. Luitz, WIEN2k:An Augmented Plane Wave Plus Local Orbitals Program for Calculating Crystal Properties, Vienna University of Technology, Austria, 2001, https://www.researchgate.net/publication/237132866_WIEN2k_An_Augmented_Plane_Wave_plus_Local_Orbitals_Program_for_Calculating_Crystal_Properties Search PubMed.
- G. K. H. Madsen and D. J. Singh, BoltzTraP. A code for calculating band-structure-dependent quantities, Comput. Phys. Commun., 2006, 175, 67 CrossRef CAS.
- J. P. Perdew, K. Burke and M. Ernzerhof, Generalized Gradient Approximation Made Simple, Phys. Rev. Lett., 1996, 77, 3865 CrossRef CAS PubMed.
- F. Tran and P. Blaha, Accurate band gaps of semiconductors and insulators with a semilocal exchange-correlation potential, Phys. Rev. Lett., 2009, 102, 226401 CrossRef.
- I. V. Fernandez, S. Mariotti, O. S. Hutter, M. Birkett, T. D. Veal, T. D. C. Hobson, L. J. Phillips, L. Danos, P. K. Nayak, H. J. Snaith, W. Xie, M. P. Sherburne, M. Asta and K. Durose, Vacancy-Ordered Double Perovskite Cs2TeI6 Thin Films for Optoelectronics, Chem. Mater., 2020, 32, 6676 CrossRef.
- Q. Mahmood, M. Hassan and N. A. Noor, Systematic study of room temperature ferromagnetism and optical response of Zn1-xTMxS/Se (TM Mn, Fe Co, Ni) ferromagnets: First principle approach, J. Phys.: Condens. Matter, 2016, 28, 506001 CrossRef CAS.
- C. W. Zhang and S. S. Yan, First-principles prediction of half-metallic ferromagnetism in Cu-doped ZnS, J. Appl. Phys., 2010, 107, 043913, DOI:10.1063/1.3309771.
- C. Li, X. Lu, W. Ding, L. Feng, Y. Gao and Z. Guo, Formability of ABX3 (X = F, Cl, Br, I) halide perovskites, Acta Crystallogr., Sect. B: Struct. Sci., 2008, 64, 702 CrossRef CAS.
- M. Jamal, S. J. Asadabadi, I. Ahmad and H. A. R. Aliabad, Elastic constants of cubic crystals, Comput. Mater. Sci., 2014, 95, 592 CrossRef CAS.
- J. Wang, S. Yip, S. R. Phillpot and D. Wolf, Crystal instabilities at finite strain, Phys. Rev. Lett., 1993, 71, 4182 CrossRef CAS.
- L. E. Bell, Cooling, heating, generating power, and recovering waste heat with thermoelectric systems, Science, 2008, 321, 1457 CrossRef CAS.
- I. Petsagkourakis, K. Tybrandt, X. Crispin, I. Ohkubo, N. Satoh and T. Mori, Thermoelectric materials and applications for energy harvesting power generation, Sci. Technol. Adv. Mater., 2018, 19, 836–862 CrossRef CAS.
- N. Nandihalli, C. J. Liu and T. Mori, Polymer based thermoelectric nanocomposite materials and devices: Fabrication and characteristics, Nano Energy, 2020, 78, 105186 CrossRef CAS.
- B. Cai, H. L. Zhuang, J. Pei, B. Su, J. W. Li, H. Hu, Y. Jiang and J. F. Li, Spark plasma sintered Bi-Sb-Te alloys derived from ingot scrap: Maximizing thermoelectric performance by tailoring their composition and optimizing sintering time, Nano Energy, 2021, 85, 106040 CrossRef CAS.
- Z. Liu, N. Sato, W. Gao, K. Yubuta, N. Kawamoto, M. Mitome, K. Kurashima, Y. Owada, K. Nagase, C. Ho Lee, J. Yi, K. Tsuchiya and T. Mori, Demonstration of ultrahigh thermoelectric efficiency of ∼7.3% in Mg3Sb2/MgAgSb module for low-temperature energy harvesting, Joule, 2021, 5, 1196 CrossRef CAS.
- R. Ang, A. U. Khan, N. Tsujii, K. Takai, R. Nakamura and T. Mori, Thermoelectricity Generation and Electron-Magnon Scattering in a Natural Chalcopyrite Mineral from a Deep-Sea Hydrothermal Vent, Angew. Chem., Int. Ed., 2015, 54, 12909 CrossRef CAS PubMed.
- F. Ahmed, N. Tsujii and T. Mori, Thermoelectric properties of CuGa1−xMnxTe2: power factor enhancement by incorporation of magnetic ions, J. Mater. Chem. A, 2017, 5, 7545 RSC.
- Y. Zheng, T. Lu, M. M. H. Polash, M. Rasoulianboroujeni, N. Liu, M. E. Manley, Y. Deng, P. J. Sun, X. L. Chen, R. P. Hermann, D. Vashaee, J. P. Heremans and H. Zhao, Paramagnon drag in high thermoelectric figure of merit Li-doped MnTe, Sci. Adv., 2019, 5, eaat9461, DOI:10.1126/sciadv.aat9461.
- J. B. Vaney, S. A. Yamini, H. Takaki, K. Kobayashi, N. Kobayashi and T. Mori, Magnetism-mediated thermoelectric performance of the Cr-doped bismuth telluride tetradymite, Mater. Today Phys., 2019, 9, 100090 CrossRef.
- B. He, C. Sahin, S. R. Boona, B. C. Sales, Y. Pan, C. Felser, M. E. Flatte and J. P. Heremans, Large magnon-induced anomalous Nernst conductivity in single-crystal MnBi, Joule, 2021, 5, 3057 CrossRef CAS PubMed.
- R. Ullah, M. A. Ali, G. Murtaza, A. Mahmood and S. M. Ramay, The significance of anti-fluorite Cs2NbI6 via its structural, electronic, magnetic, optical and thermoelectric properties, Int. J. Energy Res., 2020, 44, 10179 CrossRef CAS.
- M. Ullah, S. A. Khan, G. Murtaza, R. Khenata, N. Ullah and S. B. Omran, Electronic, thermoelectric and magnetic properties of La2NiMnO6 and La2CoMnO6, J. Magn. Magn. Mater., 2015, 377, 197 CrossRef CAS.
- O. Rabin, Y. M. Lin and M. S. Dresselhaus, Anomalously high thermoelectric figure of merit in Bi1−xSbxBi1−xSbx nanowires by carrier pocket alignment, Appl. Phys. Lett., 2001, 79, 81 CrossRef.
- R. Ullah, M. Ali, G. Murtaza, A. Mahmmod and S. M. Ramay, An investigation of structural, elastic, mechanical, electronic, magnetic and thermoelectric properties of ferromagnetic
half metallic EuCrO3, Mater. Sci. Semicond. Process., 2020, 122, 105487 CrossRef.
- A. U. Haq, G. M. Mustafa, M. Amin, S. M. Ramay and A. Mahmood, Ab-initio study of opto-electronic and thermoelectric properties of direct bandgap double perovskites Rb2XGaBr6 (X=Na, K), Int. J. Energy Res., 2021, 45, 9241 CrossRef CAS.
- S. Iqbal, G. M. Mustafa, M. Asghar, N. A. Noor, M. W. Iqbal, A. Mahmood and Y.-H. Shin, Tuning the optoelectronic and thermoelectric characteristics of narrow bandgap Rb2AlInX6(X= Cl, Br, I) double perovskites: A DFT study, Mater. Sci. Semicond. Process, 2022, 143, 106551 CrossRef CAS.
- P. A. Nawaz, G. M. Mustafa, S. Sagar, N. A. Noor, T. S. Ahmad, A. Mahmood and R. Neffati, Theoretical investigations of optoelectronic and transport properties of Rb2YInX6 (X = Cl, Br, I) double perovskite materials for solar cell applications, Sol. Energy, 2022, 231, 586 CrossRef.
- G. A. Slack, Nonmetallic crystals with high thermal conductivity, J. Phys. Chem. Solids, 1973, 34, 321 CrossRef CAS.
- M. Jamal, S. J. Asadabadi, I. Ahmad and H. A. R. Aliabad, Elastic constants of cubic crystals, Comput. Mater. Sci., 2014, 95, 592 CrossRef CAS.
|
This journal is © The Royal Society of Chemistry 2022 |
Click here to see how this site uses Cookies. View our privacy policy here.