DOI:
10.1039/D2RA02812C
(Paper)
RSC Adv., 2022,
12, 18441-18444
Direct phosphorylation of benzylic C–H bonds under transition metal-free conditions forming sp3C–P bonds†
Received
3rd May 2022
, Accepted 16th June 2022
First published on 24th June 2022
Abstract
Direct phosphorylation of benzylic C–H bonds was achieved in a biphasic system under transition metal-free conditions. A selective radical/radical sp3C–H/P(O)–H cross coupling was proposed, and various substituted toluenes were applicable. The transformation provided a promising method for constructing sp3C–P bonds.
To construct C–P bonds is of great significance in modern organic synthesis,1 because organophosphorus compounds play varied roles in medical,2,3 materials,4 and synthetic chemistry fields.5 Traditionally, the C–P bonds were formed from P–Cl species via nucleophilic substitutions with organometallic reagents,6 P–OR species via Michaelis–Arbusov reactions,7 or P–H species via the alkylation in the present of a base or a transition metal.8
Over the past decades, cross dehydrogenative coupling reactions (CDC reactions) have become a powerful and atom-economic methodology for constructing chemical bonds.9 By using this strategy, C–H bonds can couple with Z–H bonds without prefunctionalization and thus short-cut the synthetic procedures (Scheme 1a).
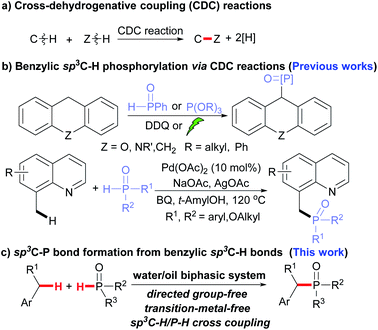 |
| Scheme 1 Cross dehydrogenative coupling reactions and direct phosphorylation of benzylic C–H bonds. | |
A similar construction of C–P bonds via CDC was also realized.10,11 Among these methods, the phosphorylation of sp3C–H having an adjacent N or O atom, or the carbonyl group was well-developed.11 Relatively, the formation of benzylic sp3C–P bond was less reported,11w which was mainly limited to the sp3C–H of xanthene or 8-methylquinoline (Scheme 1b).12 In these reported processes, transition metal catalysts or photo-, electro-catalysts were usually involved,11v and an excess of P(O)–H compounds was usually employed.11,13 To the best of our knowledge, the phosphorylation of non-active benzylic C–H bonds has scarcely been reported.
Considering both benzylic and phosphorus radicals could be generated by oxidation,14 which might subsequently couple, the phosphorylation of benzylic sp3C–H bonds would be achieved (Scheme 1c). Herein, we disclosed the construction of benzylic C–P bonds from toluene and P–H species. The reaction was carried out under transition metal-free reaction conditions,15 and exhibited high regio-selectivity. The aromatic C–H remained intact during the reaction.
We began our investigation by exploring the reaction of toluene 1a and diphenylphosphine oxide 2a in the presence of an oxidant (Table 1). When 2a was stirred in toluene at 120 °C for 3 h in the presence of 2 equiv. potassium persulfates, a trace amount of diphenylbenzylphosphine oxide 3a (<1%) was detected (entry 1). In a mixed solvent of toluene/water (1
:
1), the yield of 3a was increased to 12% (entry 2). It should be noted that the homocoupled product 1,2-diphenylethane 4 was formed in 16
:
84 ratio (3a/4). Further addition of 1 equiv. phase transfer reagent (sodium dodecyl sulfate, SDS) led to produce 3a in 37% yield (entry 3).16 Other persulfates could also be used as an oxidant albeit with decreasing yields (Table 1, entries 4 and 5). No desired products were detected when the reaction was carried out with oxone or in the absence of oxidants (entries 6–8). By using sodium 4-dodecylbenzenesulfonate (SDBS) instead of SDS as the phase transfer reagent, a higher yield was afforded (entry 9). A higher percent of water (toluene/water = 1
:
2) gave 3a in 46% yield. However, further increasing the percent of water led to a decrease of the yield (entries 10 and 11). The yield was not changed with a decreased load of SDBS to 50 mol%. Further reducing the amount of SDBS to 20 mol% resulted in a poorer yield (entries 12 and 13). At lower temperature, the yield of 3a was also decreased to 29% (entry 14). Worth noting is that the reaction could be complete within 15 min when 3 equiv. of K2S2O8 was used, producing 3a in 48% yield (entry 15).
Table 1 Optimization of the reaction conditionsa
Based on the above results, we can easily find that a serious amount of 1,2-diphenylethane 4 was formed. These results suggest that the homocoupling rate of 1a was very quick. Thus, the choice of the phase transfer reagent and oxidant is the key to cross-coupling of toluene and diphenylphosphine oxide in this biphasic solvent system.
Excessive P–H species were usually employed in reported CDC reactions to form C–P bonds, because of their facile oxidation.13 In our procedure, toluene was excessive, thus the yields were calculated based on 2a. The yields looked like low, which did not indicate the poorer conversion rate of P–H species. With the optimized conditions in hand, the substrate scope of the CDC reactions was explored (Table 2). Toluene and variously substituted toluene reacted with secondary phosphine oxides to afford corresponding 3. The phosphorylation of sp2C–H was not detected, and only mono-phosphorylation product was formed, which exhibited excellent chemo- and regioselectivity.
Table 2 Transition-metal-free benzylic C–H phosphorylationa
Reaction conditions: 1 (0.8 mL), 2 (0.2 mmol), K2S2O8 (3 equiv.), SDBS (50%) and H2O (1.6 mL), 120 °C, under N2, the reactions were monitored by TLC and/or GC until 2 work out. 1 mmol scale, 30 min. 130 °C. 100 °C. |
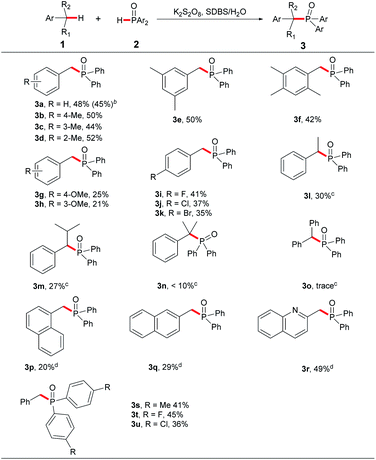 |
In addition to toluene, o-xylene, m-xylene, p-xylene, mesitylene, and 1,2,4,5-tetramethylbenzene all coupled with 2a to give the expected 3b–f in moderate yields. Methoxy substituted toluene gave relatively lower yields of 3g and 3h. para-Halo substituted toluene exhibited good reactivity, furnishing the coupling products 3i and 3j in moderate yields. Comparing to toluene, a decreasing order of reactivity was observed for ethyl benzene (3l, 30% yield), isobutyl benzene (3m, 27% yield), isopropyl benzene (3n, <10% yield), and diphenyl methane (3o, trace). The order was probably controlled by the steric hindrance around the benzylic carbon. 1-Methylnaphthalene and 2-methylnaphthalene also gave low yields (3p and 3q). However, 2-methylquinoline served well and coupled with 2a, affording the product 3r in 49% yield, which could be ascribed to the activation of the nitrogen atom. Besides of 2a, diaryl phosphine oxides having methyl, F, and Cl substituents could also be employed as the substrate, producing 3s–3u in moderate yields under similar reaction conditions.
Although the mechanism of the direct phosphorylation of benzyl C–H bond in aqueous solution is not quite clear, some aspects could be grasped based on experimental results. Firstly, the desired product 3a was not detected when the 2,2,6,6-tetramethyl-1-piperidinyloxy (TEMPO) was added, implying that a radical pathway might be possible in this reaction (entry 16). Secondly, the reaction only occurred in aqueous phase, and relied on the presence of PTC (phase transfer catalyst), as seen in that 3a was difficultly formed in entries 1 and 2 of Table 1. In most cases, 1,2-diphenylethane 4 was detected, which showed benzyl radical 5 should be involved.17
We supposed 1 is converted to benzyl radical 5 by SO4− radical that is generated via hemolytic cleavage of potassium persulfates.18 Meanwhile, phosphorus radical 6 is similarly formed from 2. Because potassium persulfate was water soluble, both 1 and 2 had to be transferred into aqueous solution to react with potassium persulfates.19 This proposal is also in accord with the experimental results that no products of sp2C–H phosphorylation are detected, which are the main products in the previous radical systems.20 Finally, cross couplings between 5 and 6 produce 3 (Scheme 2).
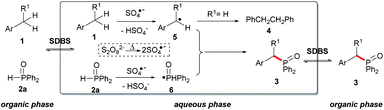 |
| Scheme 2 Proposed mechanism for the direct phosphorylation of benzylic C–H bonds under transition metal-free reaction conditions. | |
Conclusions
In summary, we have achieved the first direct phosphorylation of benzylic C–H bonds with secondary phosphine oxides in a biphasic system under the transition metal-free reaction conditions via radical/radical sp3C–H/P(O)–H cross coupling. This transformation is applicable to various toluene derivatives, affording a new atom-economic protocol for sp3C–P bond formation. Compared with the previous reports, this novel process based on a readily available reaction system exhibits exclusive chemo- and regioselectivity.
Conflicts of interest
There are no conflicts to declare.
Acknowledgements
The authors acknowledge the financial support of the Natural Science Foundation of China (grant no. 21802062, 21573064).
Notes and references
-
(a) C. Baillie and J. Xiao, Curr. Org. Chem., 2003, 7, 477 CrossRef CAS; for reviews of C–P bonds formation, see:
(b) F. M. J. Tappe, V. T. Trepohl and M. Oestreich, Synthesis, 2010, 3037 CAS;
(c) C. S. Demmer, N. Krogsgaard-Larsen and L. Bunch, Chem. Rev., 2011, 111, 7981 CrossRef CAS PubMed;
(d) S. Wendels, T. Chavez, M. Bonnet, K. A. Salmeia and S. Gaan, Materials, 2017, 10, 784 CrossRef PubMed;
(e) J. Yang, J. Xiao, Y.-B. Zhou, T. Chen, S.-F. Yin and L.-B. Han, Chin. J. Org. Chem., 2017, 37, 1055 CrossRef CAS;
(f) Y.-T. Huang and Q. Chen, Chin. J. Org. Chem., 2021, 41, 4138 CrossRef.
-
(a) M. Sawa, T. Kiyoi, K. Kurokawa, H. Kumihara, M. Yamamoto, T. Miyasaka, Y. Ito, R. Hirayama, T. Inoue, Y. Kirii, E. Nishiwaki, H. Ohmoto, Y. Maeda, E. Ishibushi, Y. Inoue, K. Yoshino and H. Kondo, J. Med. Chem., 2002, 45, 919 CrossRef CAS PubMed;
(b) X. Chen, D. J. Kopecky, J. Mihalic, S. Jeffries, X. Min, J. Heath, J. Deignan, S. Lai, Z. Fu, C. Guimaraes, S. Shen, S. Li, S. Johnstone, S. Thibault, H. Xu, M. Cardozo, W. Shen, N. Walker, F. Kayser and Z. Wang, J. Med. Chem., 2012, 55, 3837 CrossRef CAS PubMed.
-
(a) L. Bialy and H. Waldmann, Angew. Chem., Int. Ed., 2005, 44, 3814 CrossRef CAS PubMed;
(b) A. George and A. Veis, Chem. Rev., 2008, 108, 4670 CrossRef CAS PubMed;
(c) K. C. Nicolaou, P. Maligres, J. Shin, E. De Leon and D. Rideout, J. Am. Chem. Soc., 1990, 112, 1826 Search PubMed;
(d) R. K. Haynes, W. A. Loughlin and T. W. Hambley, J. Org. Chem., 1991, 56, 5785 CrossRef CAS;
(e) R. K. Haynes, S. C. Vonwiller and T. W. Hambley, J. Org. Chem., 1989, 54, 5162 CrossRef CAS.
-
(a) H. R. Allcock, M. A. Hofmann, C. M. Ambler and R. V. Morford, Macromolecules, 2002, 35, 3484 CrossRef CAS;
(b) H. Onouchi, T. Miyagawa, A. Furuko, K. Maeda and E. Yashima, J. Am. Chem. Soc., 2005, 127, 2960 CrossRef CAS PubMed;
(c) C. Queffélec, M. Petit, P. Janvier, D. A. Knight and B. Bujoli, Chem. Rev., 2012, 112, 3777 CrossRef PubMed.
-
(a) J. Boutagy and R. Thomas, Chem. Rev., 1974, 74, 87 CrossRef CAS;
(b) B. E. Maryanoff and A. B. Reitz, Chem. Rev., 1989, 89, 863 CrossRef CAS;
(c) M. Kitamura, M. Tokunaga and R. Noyori, J. Am. Chem. Soc., 1995, 117, 2931 CrossRef CAS.
- B. Zhang, C. G. Daniliuc and A. Studer, Org. Lett., 2014, 16, 250 CrossRef CAS PubMed.
-
(a) P.-Y. Renard, P. Vayron, E. Leclerc, A. Valleix and C. Mioskowski, Angew. Chem., Int. Ed., 2003, 42, 2389 CrossRef CAS PubMed;
(b) A. K. Bhattacharya and G. Thyagarajan, Chem. Rev., 1981, 81, 415 CrossRef CAS;
(c) B. A. Arbuzov, Pure Appl. Chem., 1964, 9, 307 CrossRef CAS;
(d) X. Ma, Q. Xu, H. Li, C. Su, L. Yu, X. Zhang, H. Cao and L.-B. Han, Green Chem., 2018, 20, 3408 RSC.
-
(a) T. Hirao, T. Masunaga, Y. Ohshiro and T. Agawa, Synthesis, 1981, 56 CrossRef CAS;
(b) A. L. Schwan, Chem. Soc. Rev., 2004, 33, 218 RSC;
(c) H. Rao, Y. Jin, H. Fu and Y. Zhao, Chem.–Eur. J., 2006, 12, 3636 CrossRef CAS PubMed;
(d) F. M. J. Tappe, V. T. Trepohl and M. Oestreich, Synthesis, 2010, 3037 CAS;
(e) I. P. Beletskaya and M. A. Kazankova, Russ. J. Org. Chem., 2002, 38, 1391 CrossRef CAS;
(f) M. Andaloussi, J. Lindh, J. Sävmarker, P. J. R. Sjöberg and M. Larhed, Chem. –Eur. J., 2009, 15, 13069 CrossRef CAS PubMed;
(g) R. Zhuang, J. Xu, Z. Cai, G. Tang, M. Fang and Y. Zhao, Org. Lett., 2011, 13, 2110 CrossRef CAS PubMed;
(h) H.-Y. Zhang, M. Sun, Y.-N. Ma, Q.-P. Tian and S.-D. Yang, Org. Biomol. Chem., 2012, 10, 9627 RSC;
(i) Z.-Y. Wang, Q. Guo, S. Xu and K.-K. Wang, Synthesis, 2021, 53, 3683 CrossRef CAS.
-
(a) C.-J. Li, From C-H to C-C bonds: cross dehydrogenative coupling, Royal Society of Chemistry, 2014 RSC;
(b) C.-J. Li, Acc. Chem. Rev., 2009, 42, 335 CrossRef CAS PubMed;
(c) C. S. Yeung and V. M. Dong, Chem. Rev., 2011, 111, 1215 CrossRef CAS PubMed;
(d) Z. Zhao and F. Peng, Angew. Chem., Int. Ed., 2010, 49, 9566 CrossRef PubMed;
(e) R. Waterman, Chem. Soc. Rev., 2013, 42, 5629 RSC;
(f) S. A. Girard, T. Knauber and C.-J. Li, Angew. Chem., Int. Ed., 2014, 53, 74 CrossRef CAS PubMed;
(g) T. Tian, Z. Li and C.-J. Li, Green Chem, 2021, 23, 6789 RSC.
-
(a) T. Chen, J.-S. Zhang and L.-B. Han, Dalton Trans., 2016, 1843 RSC;
(b) T. Chen and L.-B. Han, Synlett, 2015, 26, 1153 CrossRef CAS;
(c) O. Berger and J.-L. Montchamp, Chem.–Eur. J., 2014, 20, 12385 CrossRef CAS PubMed;
(d) X. Mao, X. Ma, S. Zhang, H. Hu, C. Zhu and Y. Cheng, Eur. J. Org. Chem., 2013, 4245 CrossRef CAS;
(e) X.-J. Mu, J.-P. Zou, Q.-F. Qian and W. Zhang, Org. Lett., 2006, 8, 5291 CrossRef CAS PubMed;
(f) T. Kagayama, A. Nakano, S. Sakaguchi and Y. Ishii, Org. Lett., 2006, 8, 407 CrossRef CAS PubMed. For reviews of sp2C–P bonds formation, see:
(g) H. B. Yu and O. G. Sinyashin, Russ. Chem. Rev., 2015, 84, 917 CrossRef;
(h) A. Włodarczyk, Tetrahedron, 2022, 106–107, 132550 CrossRef , and references cited therein..
-
(a) O. Baslé and C.-J. Li, Chem. Commun., 2009, 27, 4124 RSC;
(b) W. Han and A. R. Ofial, Chem. Commun., 2009, 40, 6023 RSC;
(c) W. Han, P. Mayer and A. R. Ofial, Adv. Synth. Catal., 2010, 352, 1667 CrossRef CAS;
(d) J. Xie, H. Li, Q. Xue, Y. Cheng and C. Zhu, Adv. Synth. Catal., 2012, 354, 1646 CrossRef CAS;
(e) M. Rueping, S. Zhu and R. M. Koenigs, Chem. Commun., 2011, 47, 8679 RSC;
(f) W.-P. To, Y. Liu, T.-C. Lau and C.-M. Che, Chem.–Eur. J., 2013, 19, 5654 CrossRef CAS PubMed;
(g) W.-J. Yoo and S. Kobayashi, Green Chem., 2014, 16, 2438 RSC;
(h) D. P. Hari and B. König, Org. Lett., 2011, 13, 3852 CrossRef CAS PubMed;
(i) K. Alagiri, P. Devadig and K. R. Prabhu, Tetrahedron Lett., 2012, 53, 1456 CrossRef CAS;
(j) J. Dhineshkumar, M. Lamani, K. Alagiri and K. R. Prabhu, Org. Lett., 2013, 15, 1092 CrossRef CAS PubMed;
(k) A. Tanoue, W.-J. Yoo and S. Kobayashi, Org. Lett., 2014, 16, 2346 CrossRef CAS PubMed;
(l) C. Huo, C. Wang, M. Wu, X. Jia, X. Wang, Y. Yuan and H. Xie, Org. Biomol. Chem., 2014, 12, 3123 RSC;
(m) H. Wang, X. Li, F. Wu and B. Wan, Tetrahedron Lett., 2012, 53, 681 CrossRef CAS;
(n) K. Alagiri, P. Devadig and K. R. Prabhu, Chem.–Eur. J., 2012, 18, 5160 CrossRef CAS PubMed;
(o) C. Huo, H. Xie, M. Wu, X. Jia, X. Wang, F. Chen and J. Tang, Chem.–Eur. J., 2015, 21, 5723 CrossRef CAS PubMed;
(p) M. N. Gandy, C. L. Raston and K. A. Stubbs, Chem. Commun., 2015, 51, 11041 RSC;
(q) X.-Z. Wang, Q.-Y. Meng, J.-J. Zhong, X.-W. Gao, T. Lei, L.-M. Zhao, Z.-J. Li, B. Chen, C.-H. Tung and L.-Z. Wu, Chem. Commun., 2015, 51, 11256 RSC;
(r) H. E. Ho, Y. Ishikawa, N. Asao, Y. Yamamoto and T. Jin, Chem. Commun., 2015, 51, 12764 RSC;
(s) H. Zhi, S. P.-M. Ung, Y. Liu, Y. Zhao and C.-J. Li, Adv. Synth. Catal., 2016, 358, 2553 CrossRef CAS;
(t) Y. Liu, C. Wang, D. Xue, M. Xiao, C. Li and J. Xiao, Chem.–Eur. J., 2017, 23, 3051 CrossRef CAS PubMed;
(u) J. Ke, Y. Tang, H. Yin, Y. Li, Y. Cheng, C. Liu and A. Lei, Angew. Chem., Int. Ed., 2015, 54, 6604 CrossRef CAS PubMed;
(v) L. Chen, X.-Y. Liu and Y.-X. Zou, Adv. Synth. Catal., 2020, 362, 1724 CrossRef CAS;
(w) S. Hore and R. P. Singh, Org. Biomol. Chem., 2022, 20, 498 RSC , and references cited therein..
-
(a) Q. Chen, X. Wang, G. Yu, C. Wen and Y. Huo, Org. Chem. Front., 2018, 5, 2652 RSC;
(b) Y. Yuan, J. Qiao, Y. Cao, J. Tang, M. Wang, G. Ke, Y. Lu, X. Liu and A. Lei, Chem. Commun., 2019, 55, 4230 RSC;
(c) K.-J. Li, Y.-Y. Jiang, K. Xu, C.-C. Zeng and B.-G. Sun, Green Chem., 2019, 21, 4412 RSC;
(d) L. Chen, Z. Zhou, S. Zhang, X. Li, X. Ma and J. Dong, Chem. Commun., 2019, 55, 13693 RSC;
(e) C. Wen, G. Yu, Y. Ou, X. Wang, K. Zhang and Q. Chen, Tetrahedron Lett., 2019, 60, 1345 CrossRef CAS.
-
(a) N. V. Dubroviba and A. Börner, Angew. Chem., Int. Ed., 2004, 43, 5883 CrossRef PubMed;
(b) W. B. Beaulieu, T. B. Rauchfuss and D. M. Roundhill, Inorg. Chem., 1975, 14, 1732 CrossRef CAS;
(c) L. Ackermann, R. Born, J. H. Spatz, A. Althammer and G. J. Gschrei, Pure Appl. Chem., 2006, 78, 209 CrossRef CAS;
(d) J. Xu, P. Zhang, X. Li, Y. Gao, J. Wu, G. Tang and Y. Zhao, Adv. Synth. Catal., 2014, 356, 3331 CrossRef CAS.
-
(a) Y.-M. Li, M. Sun, H.-L. Wang, Q.-P. Tian and S.-D. Yang, Angew. Chem., Int. Ed., 2013, 52, 3972 CrossRef CAS PubMed;
(b) Y.-R. Chen and W.-L. Duan, J. Am. Chem. Soc., 2013, 135, 16754 CrossRef CAS PubMed;
(c) X.-Q. Pan, L. Wang, J.-P. Zou and W. Zhang, Chem. Commun., 2011, 47, 7875 RSC;
(d) D. Leca, L. Fensterbank, E. Lacôte and M. Malacria, Chem. Soc. Rev., 2005, 34, 858 RSC;
(e) L. Zhu, H. Yu, Q. Guo, Q. Chen, Z. Xu and R. Wang, Org. Lett., 2015, 17, 1978 CrossRef CAS PubMed;
(f) X.-Q. Pan, J.-P. Zou, W.-B. Yi and W. Zhang, Tetrahedron, 2015, 71, 7481 CrossRef CAS;
(g) D. Leifert and A. Studer, Angew. Chem., Int. Ed., 2020, 59, 74 CrossRef CAS PubMed;
(h) I. Bosque, R. Chinchilla, J. C. Gonzalez-Gomez, D. Guijarro and F. Alonso, Org. Chem. Front., 2020, 7, 1717 RSC.
-
(a) R. Samanta, K. Matcha and A. P. Antonchick, Eur. J. Org. Chem., 2013, 26, 5769 CrossRef;
(b) C.-L. Sun and Z.-J. Shi, Chem. Rev., 2014, 114, 9219 CrossRef CAS PubMed;
(c) R. Vanjari and K. N. Singh, Chem. Soc. Rev., 2015, 44, 8062 RSC.
-
(a) P. A. Lovell and M. S. El-Aasser, Emulsion Polymerization and Emulsion Polymers, Wiley, Chichester, 1997 Search PubMed;
(b) C.-S. Chern, Principle and Applications of Emulsion Polymerization, Wiley, Hoboken, 2008 CrossRef.
-
(a) J.-j. Ma, W.-b. Yi, G.-p. Lu and C. Cai, Org. Biomol. Chem., 2015, 13, 2890 RSC;
(b) P. Kumar, T. Guntreddi, R. Singh and K. N. Singh, Org. Chem. Front., 2017, 4, 147 RSC.
-
(a) X. S. Liu, Z. T. Wang and C. Z. Li, J. Am. Chem. Soc., 2012, 134, 14330 CrossRef CAS PubMed;
(b) F. Yin and X. S. Wang, Org. Lett., 2014, 16, 1128 CrossRef CAS PubMed;
(c) N. Basickes, T. E. Hogan and A. Sen, J. Am. Chem. Soc., 1996, 118, 13111 CrossRef CAS;
(d) W. P. Mai, J. T. Wang and L. B. Qu, Org. Lett., 2014, 16, 204 CrossRef CAS PubMed;
(e) N. R. Patel and R. A. Flowers II, J. Am. Chem. Soc., 2013, 135, 4672 CrossRef CAS PubMed;
(f) W. P. Mai, G. C. Sun and L. B. Qu, J. Org. Chem., 2014, 79, 8094 CrossRef CAS PubMed.
- For similar reports, see:
(a) R. D. Baxter, Y. Liang, X. Hong, T. A. Brown, R. N. Zare, K. N. Houk, P. S. Baran and D. G. Blackmond, ACS Cent. Sci., 2015, 1, 456 CrossRef CAS PubMed;
(b) L.-M. Altmann, M. C. D. Fürst, E. I. Gans, V. Zantop, G. Pratsch and M. R. Heinrich, Org. Lett., 2020, 22, 479 CrossRef CAS PubMed.
- Those reactions proceed via a process involving electrophilic addition of phosphorus radical to aromatic cycles, single-electron transfer and subsequent deprotonation, see ref. 10c–h.
Footnote |
† Electronic supplementary information (ESI) available: Experimental information, characterization data for the products and copies of 1H, 13C, and 31P NMR spectroscopies. See https://doi.org/10.1039/d2ra02812c |
|
This journal is © The Royal Society of Chemistry 2022 |
Click here to see how this site uses Cookies. View our privacy policy here.