DOI:
10.1039/D2RA02864F
(Review Article)
RSC Adv., 2022,
12, 23808-23828
Recent update on electrospinning and electrospun nanofibers: current trends and their applications
Received
6th May 2022
, Accepted 13th July 2022
First published on 23rd August 2022
Abstract
Electrospinning is a versatile and viable technique for generating ultrathin fibers. Remarkable progress has been made in techniques for creating electro-spun and non-electro-spun nanofibers. Nanofibers were the center of attention for industries and researchers due to their simplicity in manufacture and setup. The review discusses a thorough overview of both electrospinning and non-electrospinning processes, including their setup, fabrication process, components, and applications. The review starts with an overview of the field of nanotechnology, the background of electrospinning, the surge in demand for nanofiber production, the materials needed to make nanofibers, and the critical process variables that determine the characteristics of nanofibers. Additionally, the diverse applications of electrospun nanofibers, such as smart mats, catalytic supports, filtration membranes, energy storage/heritage components, electrical devices (batteries), and biomedical scaffolds, are then covered. Further, the review concentrates on the most recent and pertinent developments in nanofibers that are connected to the use of nanofibers, focusing on the most illustrative cases. Finally, challenges and their possible solutions, marketing, and the future prospects of nanofiber development are discussed.
1. Introduction
Nanotechnology is one of the fastest growing fields of study and has entered the profession of medicine and other industries as well. Due to the wide variety of applications many researchers hailing from countries all over the world have shown their interest in the technology. With respect to the pharmaceutical industry, nanotechnology made a mark because of its efficacy and reduced adverse events.1–5 Various fields of nanotechnology are discussed by Adlakha-Hutcheon et al.6 and are diagrammatically shown in Fig. 1.
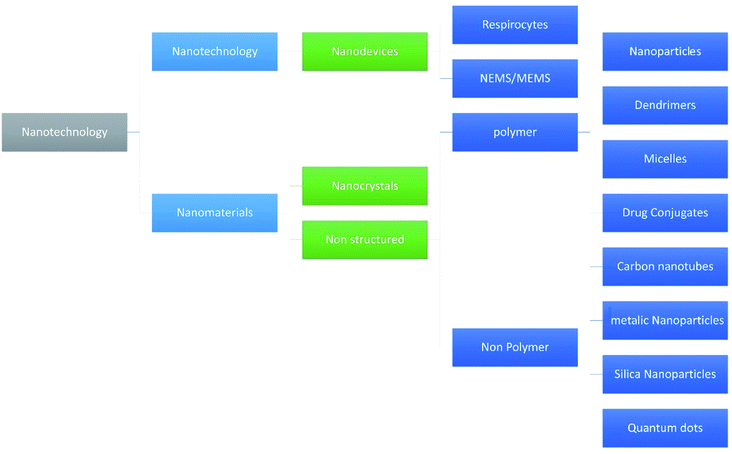 |
| Fig. 1 Summarize the fields of nanotechnology and their classification. | |
Nowadays a maximum number of drug targeting methods are based on nano-formulations because targeting therapy becomes easier when given in the form of a nano-formulation.7–16 In the category of nano-formulations nanofibers have vast application in the field of medicines,17–19,19–25 and amongst the nanofibers the magnetic nanofibers have different applications due to their characteristics and magnetic memory, as well as environmental treatment, catalysts, and biomedicine.26,27
Nanofibers can be simply defined as the fibers of the polymers having the size in the range of nanometer where the temperature of calcination and holding time affects the shape, diameter, fiber morphology, and crystallite size of the nanofiber structure. There are plethora of polymers that are being used for the manufacturing of nanofibers and other than above mentioned factors the diameter of the nanofiber heavily depends on the type of polymer used for the fabrication.28 When it comes the length of a unit nanofiber, it is almost around 60 nm, but the diameter does not vary much, it is found around 100 nm.
Evaluations of the nanofibers are done using vibrating sample magnetometer, X-ray diffraction, Fourier transform infrared spectroscopy, scanning electron.29
In the method of creation the electrospinning is very common and the basic principle of this method revolves around the static charges that are produced due to stretching of the polymer fiber repel each other which results in the formation of the fibers.30
Application of the nanofibers heavily depends upon the drug loaded in them as is the case with all the formulation in the pharmaceutical industry. Some of the applications include wound healing, targeted delivery of drugs, pH-sensitive drug therapy, gene delivery, photodynamic therapy, veterinary delivery, stent coating, theragnostic delivery of drugs, ophthalmic delivery, etc.31 Apart from this the general nanofibers have environmental applications also including, air filtration in which nanofiber beds/mats of mesh size 500 nm alone or in combination with any other filtration media used to filter the polluted air, dust particles. These filters work on the principle of absorption of dust particles over the individual nanofibers in the mesh which makes them highly efficacious. Also, these nanofiber filters are used widely in vehicle cabin filters and building filters.32 Although they have some biomedical applications in terms of tissue engineering, wound dressing, drug delivery and fabrication of face masks.
Now the fact is quiet known that the mortality rate is huge in the human population by the cancer, and thus nanofibers composed of anticancer drugs have caught the eye of crowd. As nanofibers provide a large surface area, high drug encapsulation efficiency due to their extreme porosity, good usability, low cost of fabrication with great benefits diverse research is carried out in cancer treatment via nanofiber delivery.33
There are a lot of fabrication processes are available for the manufacturing of the nanofibers, but electrospinning is the widely used method for the processing of the nanofibers. Electrospinning was used to create nanofiber composite membranes in which electrospun nanofiber coated with carbon, metal oxide, or carbon-coated metal oxide, which were subsequently modified with a thermally induced chemical cross-linking procedure. They have wideband applications (W/B) fields like environmental-desalination, oil/water adsorption, and separation, membrane separation, and waste water treatment. Although, they have biochemical applications and applications in the field of defense and chemistry which are further explained in the application part.34 Physically connected structures and chemically cross-linked networks between Polyvinyl Butyral (PVB) and Blocked Isocyanate Prepolymer (BIP) enhanced the mechanical and waterproof characteristics of the nanofibrous membranes.35 I. Alghoraibi et al. explained different techniques to fabricate the nanofibers which are illustrated in below Fig. 2.36
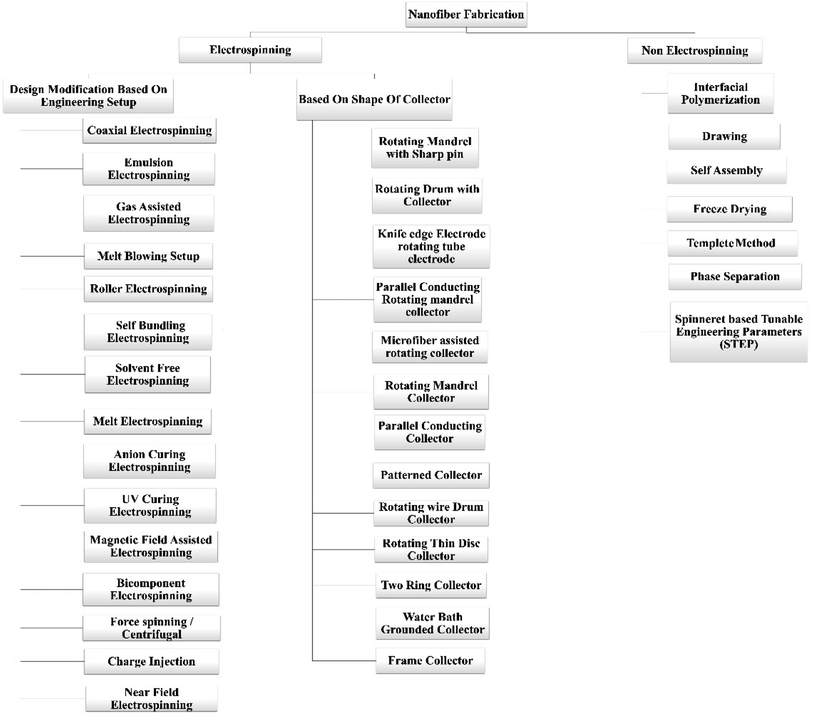 |
| Fig. 2 Illustrates the classification of different techniques used to fabricate the nanofibers. | |
Fig. 3 depicts the survey of several publications per year from 2002 to 2022. This quantitative data in the literary survey is supported by the Scopus database with the keyword ‘Electrospun Nanofibers’.
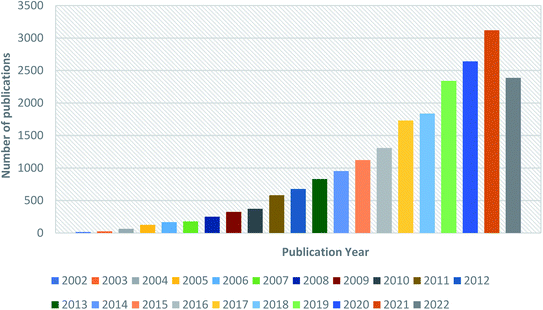 |
| Fig. 3 Graphical representation of publications per year from 2002 to 2022 obtained from the Scopus database with the keyword ‘Electrospun Nanofiber’. | |
The current review focuses on various fabrication techniques, applications, and challenges in the synthesis of electrospun nanofibers with compiled strategies to overcome challenges, additionally, it covers current available marketed products and future perspectives together which makes ease for readers to understand the concept.
2. Approaches to nanofibers fabrication
There are different methods of fabrication of nanofibers including electrospinning and non-electrospinning methods which are elaborated below.
2.1 Electrospinning techniques
Electrospinning is an effective nanofiber fabricating process that stretches unfragmented polymer fibers, from a polymer solution or polymer melt by electrostatic force using high voltage direct current, in the form of a liquid jet, simply, it is nothing but the application of high voltage current to the polymer in the syringe which forms a single thread of fiber of required dimension37 as shown in the Fig. 4 below:
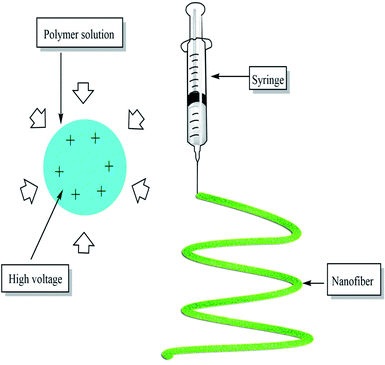 |
| Fig. 4 Pictorial representation of basic electrospinning setup. | |
Currently, there are 4 types of nanostructures which include 0D-zero-dimension (nanoparticles), 1D-one-dimension (involving nanowires, nanotubes, nanofibers) 2D two-dimension (films, layers), and 3D three-dimension structures (polycrystals).38
The concept of electrospinning was first invented by Formhals et al. in 1934.133 His innovation contains filament with the application of electric charge.39 In late 1987, the Hayati et al. studied the factors which have an impact on the atomization and the stability of the fibers. The results come out from him showing the liquid conduction capacity is the major factor in the electrostatic disruption of the surface of liquid while the broad with broad diameter were fabricated by using an unstable jet.40 The major processing parameters involved in the fabrication of the electrospinning technique include applied voltage, the distance between the tip of the nozzle and the collector, flow rate of polymer solution, the spinning environment, solution conductivity, solution viscosity, polymer concentration in solution, and solvent volatility.34
Some of the polymers that are used for the fabrication of the polymeric nanofibers are polyglycolide (PGA), poly lactide-co-glycolide (PLGA), poly-L-lactide (PLLA), poly-D,L-lactide (PDLLA), PEG, poly ε-caprolactone, montmorillonite, poly-L-lactide-co-ε-caprolactone, PVA, cellulose acetate, polystyrene, etc.41 Some of the electrospinning methods which depend upon the design of the electrospinning setup and the shape of the collector are explained below.
2.1.1 Classification of electrospinning methods depending upon design of electrospinning setup.
2.1.1.1 Emulsion electrospinning. This method provides us a chance to make a single fibrous scaffold out of two immiscible solutions when they are electrospun, the method specifies that the immiscible solutions are to be made into an emulsion by mixing strenuously after which the resulting colloid is packed into a syringe made out of glass and fitted with a power supply of high voltage and a needle. Fibers which are made out of this are difficult to produce as it involves a plethora of variables that are to be accounted for to create the emulsion. Nanoparticles and surfactants like sod. Dodecyl sulfate has been used for interface stabilization effectively.42 In this method, the core–shell containing functional materials like peptides, proteins, enzymes, and flavonoids are incorporated into a nanofiber. The setup of emulsion electrospinning is shown in Fig. 5a. As far as emulsion material passes through the needle, due to the potential difference between the needle and collector plate results in the formation of nanofiber containing core–shells of emulsion droplets. The attenuation and deformation of this emulsion droplet occurred as a result of jet speed which drags the forces derived from its interaction with the surrounding air. Due to this, the solvent gets evaporated quickly and nanofiber with a core–shell containing fundamental material is formed.42
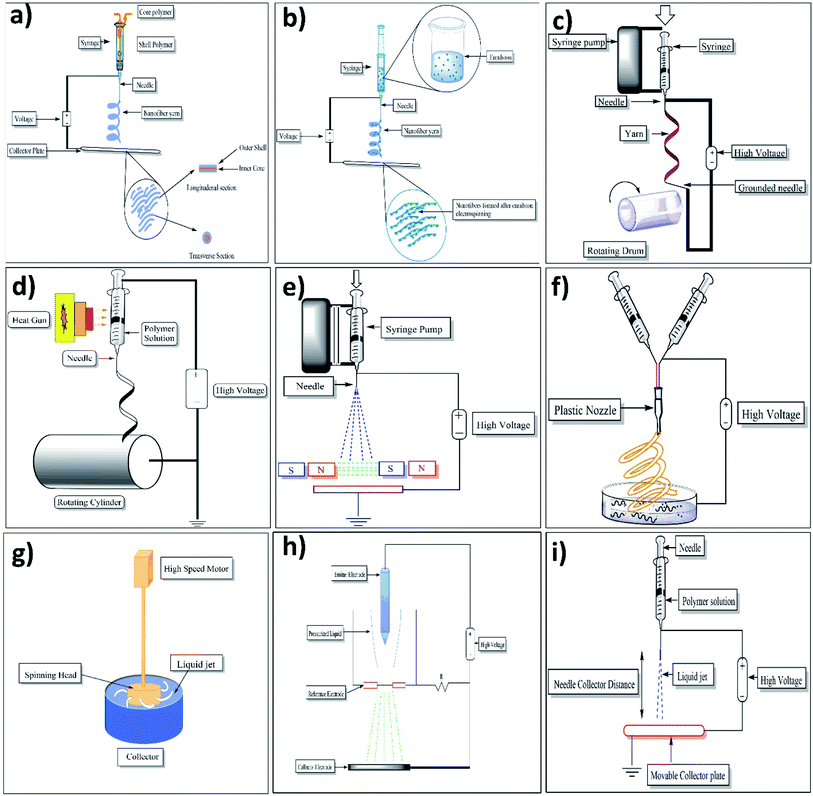 |
| Fig. 5 Pictorial representation of setup used for fabrication of nanofibers by (a) emulsion electrospinning, (b) coaxial electrospinning, (c) roller electrospinning, (d) melt electrospinning, (e) magnetic-field facilitated electrospinning with two parallel permanent magnets, (f) bicomponent electrospinning, (g) force spinning, (h) charge injection method and (i) near-field electrospinning. | |
2.1.1.2 Coaxial electrospinning. A technique that can be used to make core–shell (core–sheath) fibers by using spinneret in which there are two compartments (coaxial), where the shell/sheath (solution of polymer) and core (a solution of a compound) can be used as the precursors and co-spun, core–shell (2 different polymer solution can also be used). The fluids are independently driven using two compartments simultaneously in this method; the outer and inner solutions (precursors) reach the nozzle by passing from their capillaries and combining to produce droplets of composite. As both the solutions extrude through the jet, a nanofiber yarn is formed in which the core is surrounded by shell/sheath material as shown in Fig. 5b. Most of the time shell is acts as a physical barrier to avoid the evaporation of core material.43 Because of the electrostatic repulsive forces present in the middle of the surface, the extension was seen in the shell liquid of composite droplets, resulting in viscid tension then the resulting tension was transmitted to the core layer, which was quickly stretched.44,45
2.1.1.3 Melt-blowing setup. It is a setup of single-step to produce materials on a micrometer and small scale. Once the polymer was melted, multiple nozzles are used to eject the jet of polymer simultaneously. After that, jets were enervated using the hot air stream (high velocity), resulting in a drastic decrease in the diameter of jets. Ultimately, a web of fibers that are fine, have a random position and are non-woven gets deposited on the collector.46
2.1.1.4 Gas-assisted spinning. Also known as the spin blowing method gives a useful way to spin material that is considered poor material for electrospinning.47 Reyhaneh Aminyan et al. prepared fibers of 11% wt of aqueous a solution. The prepared fibers were kept under 20 kV voltage, followed by neutralization by 15 wt% NaOH solution, 20 rpm of the rotation speed of the collector with the fed rate of 20 μl min−1 (chosen conditions were rationalized by the previous group's experiences). Then they crosslinked prepared fibers using 1,4-butanediol diglyceryl ether at 0.5, 0.4, and 0.3% wt for 3–7 min at the temperature of 130–1600 °C. The prepared cross-linked specimens were used to obtain results by conducting the swelling ratio analysis.48Low fiber productivity is the primary problem associated with the typical electrospinning procedure. To bettering the productivity of fibers by introducing the gas jet with the application of increased driving force around the solution of the polymer. Integrated fibers with a narrower diameter dispersity can be achieved using gas-assisted electrospinning.48
2.1.1.5 Roller electrospinning (nano spider electrospinning). This particular device consists of a tank, a ground collector, a supporting material, and a power supply of high voltage.49 in this, to spin nanofibers a spinning roller electrode is half dipped into the polymer solution. An electrode (grounded) with non-woven material is fixed on the peak of the spine which makes a continuous nanofibers layer as illustrated in Fig. 5c.50
2.1.1.6 Self-bundling electrospinning. Self-bundling electrospinning is rather a straightforward mechanism for creating continuously aligned electrospun fiber yarn. It has many things like a needle tip, a syringe pump, a generator (high-voltage), and a ground collector (rotating).51 To start the formation of a bundle, a ground tip that is twisted around the collector is used and then the bundling continues. The solution's conductivity (electrical) is contemplated to be a criterion of a significant impact.52
2.1.1.7 Solvent-free electrospinning. Solvent-free electrospinning such as melt electrospinning, CO2-assisted electrospinning, and anion-curing electrospinning are techniques and never use conventional solvents. It is used to highly utilize precursors and avoid solvent emission.53
(a) Melt electrospinning.
The setup consists of a cylindrical collector, molten polymer, a needle, and a high-voltage power supply. For melting the polymer different techniques can be used such as electric heating, laser melting devices, heat guns, and heating ovens. Generally, the parameters are the same for molten polymer electrospinning as it is for the polymer solution electrospinning. Just like the latter, a large diameter is taken using a polymer with higher molecular wt and in the maximum cases, the melted one also has both nano and micrometer-scale fibers but there are some differences as well. The method for fabrication of nanofibers by electrospinning setup is illustrated in Fig. 5d.
In melt electrospinning, before initiating an uninterrupted heat supply to the reservoir, it had to be made sure that the solution of polymer is in the molten state. The gap between the tip and collector is roughly around 2 cm in melt electrospinning whereas it's around 10 cm in the conventional polymer solution electrospinning. A greater charge is required to start the jets in melt electrospinning because the viscosity of polymer melt is higher in the molten electrospinning when compared to the conventional polymer solution electrospinning.54
(b) UV-curing electrospinning.
UV-curing electrospinning method is used to develop fibers that are ultrathin nanofibres, modifications have to be done for creating an oxygen-free environment. Then only the precursors can be electrospun with the ultra-violet radiation and within the nitrogen then problems like solvent evaporation are not faced and fibers can be made ultrathin. The method utilizes an ultra-violet mendable material. An oxygen-free environment is created so that the precursors can be electro-spun under UV light and nitrogen into ultrathin fibers.53,55
(c) Anion-curing electrospinning.
Only two components are there in the solution, the cyanoacrylate (ca.) monomer and polymethyl methacrylate (PMMA). The latter is added to the former to increase the viscosity. Room temperature is enough for more than 90% of the precursor to be electrospun into ultrathin fibers. The acrylic resin (ca. monomer) may polymerize quickly and create strong chains in the presence of an anion. Just as the room temperature has a compelling impact on the diameter of the electrospun fibers created by the traditional method, it also affects the morphology of the anion-cured electro-spun fibers.47
(d) Supercritical CO2-assisted electrospinning.
In this method, electrostatic forces and supercritical CO2 solvents were used to make the polymer fibers. Carbon dioxide is the most commonly found supercritical solvent used in many processes and is known as a material that possesses both the temperature and pressure above the critical point because the supercritical stage is performed in a vessel with high pressure (14 mPa). So, it's difficult to employ a moving collector. The apparatus for this approach is far more sophisticated than any other method.53
2.1.1.8 Magnetic-field-assisted electrospinning. Fibers made using this technique don't split much like the ones without a magnetic field and are much more uniform. The advantage of the fibers which are made from this method is that they are more uniform and are with much less splitting than others. In this method, either 2 parallel permanent magnets should be used or Helmholtz coils, plus a syringe that contains the solutions that are compatible and can respond to magnets that are used. With this method, fibers with smaller diameters were attained because of high speed.56 Below mentioned Fig. 5e shows the setup of two parallel magnets with magnetic field-assisted electrospinning.
2.1.1.9 Bicomponent spinning. This technique can be used to make mono and multi-component nanofibers. It's a 2-step process that involves producing nanofibers by splitting or removing one of the components when 2 polymers are spun through a spinning die. For us to produce a nanofiber there should be at least one component that has a cross-section in the nanoscale. Some of the common shapes for the bi-component fibers are segmented pie (hollow, ribbon, plain), sheath–core, side by side, etc. If shapes are to be explained, for instance, islands in the sea in which the sea is of one polymer need to be enclosed by other polymers known as islands (multiple individual fibrils) as shown in the below Fig. 5f.57
2.1.1.10 Force spinning (centrifugal spinning). This particular method is known to be well refined and is also called rotational/rotary spinning. The only dissemblance between the said method and the original one is the use of c-force in place of the electric field. In this method when the speed of rotation reaches a certain point then the c-force will be significant enough to subdue the surface tension then only the liquid jet will be excluded from the tip. Ultimately, the c-force will make the jet stretched and nanofibers will be deposited on the collector. The most significant drawback of centrifugal spinning is that material characteristics and spinneret design have a significant impact on fiber quality and production (as shown in Fig. 5g).5.8
2.1.1.11 Charge injection method. This technique was refined for electro-spraying still it can be used for electrospinning. This method is dissimilar from the ones used by other devices. This method doesn't involve the formation of Taylor cones and the splitting of the jet is done by an electrical charge. Out of many configurations for this technique, one of them involves three electrodes (power supply-high voltage, collector, and non-conducting pressurized fluid) and two out of those are submerged into the solution (as shown in Fig. 5h).5.9The “emitter electrode's” sharpened tip is situated above a grounded orifice i.e. blunt electrode. A strong e-field is created in the solution due to the narrow proximity between electrodes. As the fluid travels between the electrodes, it is continually pushed through the aperture at increased pressure and becomes highly charged, the extruded filaments from the orifice might be drawn towards the collector. Because of the proximity of the emitter electrode and aperture, this method has some certain disadvantages, like the lack of control over the size distribution of the fibers and that only fluids which have low conductivity/insulating can be used. As a matter of fact, the electro-spun mats are normally made of microfibers which are interconnected branches of nanoscale fibers.60
2.1.1.12 NFES (near-field electrospinning). Apart from the distance between the needle and the collector the system setup is the same as standard. The reduction in the distance (500 μm to 3 mm) is to avert the curving unsteadiness of the jet. The distance in the question employs a sturdy area of the electro-spun jet near the needle, which makes this system good for direct writing. But this closeness between the collector and the spinneret may create electric breakdown or splattering of the fibers because of insufficient evaporation of the solvent. As a result, an adjustment must be done with the polymer solution accurately. To initiate the process, a lower applied voltage (usually 100–600 V) is utilized. In contrast to traditional electrospinning, higher voltage has been observed to result in bigger diameter fibers in this method. With the volume of the collected fibers being limited, the alone droplet of the polymer is more than enough for the direct writing, negating the necessity for a consistent flow of polymer. To get an accurate control over orientation and deposition of the fibers the collector is normally put up on the XY-piezo stage.61 The setup of near-field electrospinning setup was well illustrated in Fig. 5i.
2.1.2 Classification of electrospinning methods depending upon the collector shape.
2.1.2.1 Electrospinning setup with rotating drum collector. In the said technique rotating drum is used as an electrode to collect the fibers (as shown in Fig. 6a). Quoc Pham Le et al. fabricated PVC nanofibers by this method. The parameters used for the fabrication of such PVC nanofibers are the speed of the drum (500–2500 rpm), the flow rate of polymer solution (5 ml h−1), applied voltage (20 kV), and the distance between the tip of the needle and surface of rotating drum (15 cm).62 The different diameter of nanofibers is fabricated at a different drum rotation speed, at the rotation speed of 0 rpm the nanofibers of diameter 313 nm are obtained, at 500 rpm NFS with a diameter of 280 nm, at 1000,1500, 2000, and 2500 rpm the diameter of 280, 272, 256 and 229 nm are obtained respectively.62
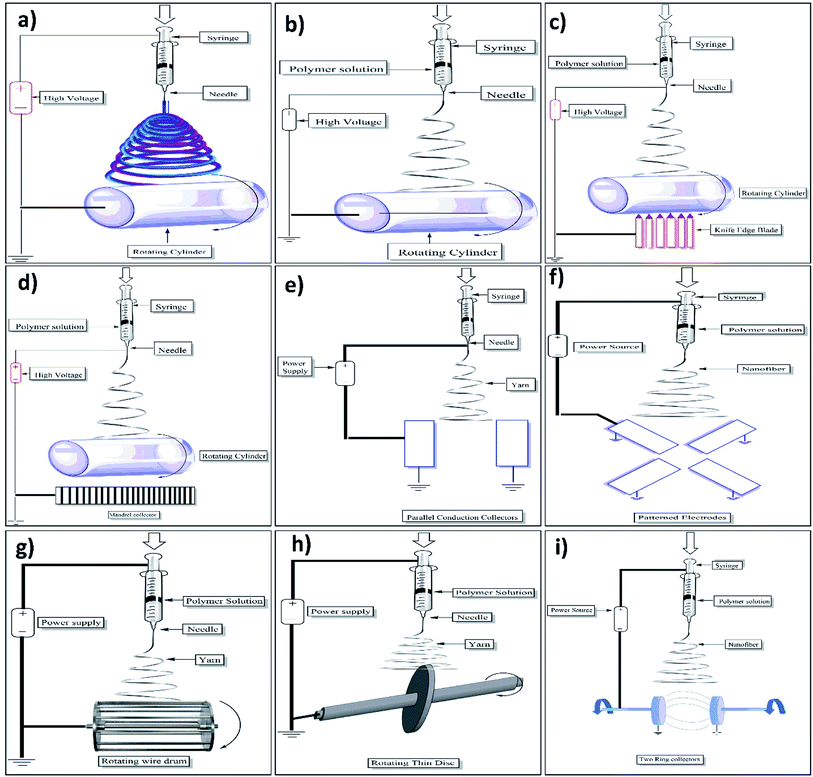 |
| Fig. 6 Setup used for fabrication of nanofibers by (a) electrospinning with rotating cylinder collector, (b) electrospinning with a revolving mandrel collector and a sharp pin, (c) electrospinning with knife-edge electrodes and a revolving tube collector, (d) electrospinning using a revolving parallel conducting mandrel collector, (e) parallel conducting receiver electrospinning, (f) patterned electrodes for electrospinning, (g) electrospinning using a revolving wire drum collector, (h) electrospinning with a narrow rotating disc collector with two rings, and (i) electrospinning using double-ring collector. | |
The fabrication of fibers depends on the rotation speed of the collector drum. With the increase in the speed of the collector above the specific limit, no continuous fibers are made because of the breaking of the fiber jet at a high rotation speed. While fibers with fair continuous diameter will be produced at a speed lower than alignment speed.63
2.1.2.2 Electrospinning setup having sharp pin with a rotating mandrel. Zhu, Ye, Pan, et al. fabricated micro-aligned nanofibers using the sharp pin having a negative charge with a diameter of 0.7 mm when positioned in the mandrel (rotating). Due to which the alignment of the fabricated fibers was improved by the application of an electric field of 15 to 20 kV. This applied electric field proceeds from the tip of the needle and is conveyed at the sharp pin positioned in the mandrel (rotating) (as shown in Fig. 6b). As compared to other methods this method is easier and simpler for the fabrication of nanofibers.64
2.1.2.3 Electrospinning setup with knife-edge electrodes and rotating tube collector. Z. Yang et al. used the edge electrode-rotating tube collector with a knife electrospinning setup for the nanofiber's fabrication. The disadvantage of the insulation effect of deposited fibers was overcome in this method. The thick layers of ordered nanofibers are deposited by the maintenance of an electrical field in between the deposited fiber yarns. They prepared poly(ε-caprolactone)/layered double hydroxide nanocomposites by applying negatively charged voltage to the knife-edge electrodes beneath an insulating mandrel (rotating). The fibers with different orientations and having multilayers scaffolds bearing customized mechanical properties can be deposited by just tilting the needle and the electrodes is the unique advantage of this method (as shown in Fig. 6c). Another advantage of this method is, that it makes hugely ordered electrospun meshes with dissimilar orientation angles.65
2.1.2.4 Electrospinning setup with parallel conducting stripes and rotating mandrel collector. A rotating mandrel collector with parallel conduction copper wire produces the fibers in the range of micron or nanometer range. The polymer solution for the electrospinning consisted of the polycaprolactone in methanol 10%
:
dichloromethane and dimethylformamide in the ratio of 6
:
3
:
1. By this method, the nanofibers having an average diameter of 1142 ± 391 nm at the rotation speed of 0 rpm are randomly oriented. While the aligned nanofibers are obtained at the speed of 200 rpm with an average diameter range of 663 ± 334 nm.66S. Liao et al. used the method in which, the needle was positively charged, and the auxiliary electrodes were negatively charged. To alter the electric field they placed a rotating mandrel which was nonconductor in nature in between the tip of the needle and an electrode (auxiliary) (parallel conducting strips) (as shown in Fig. 6d) which oriented the electric field to the auxiliary electrodes from the needle. As compared to the technique of rotating mandrel alone this setup produces better-aligned fibers at the lower rotation speed of around <1000 rpm. The reason was that the parallel conducting strips that focus the e-field along with the parallel strip orientation, lead to the fiber acceleration towards the electrode.67
2.1.2.5 Electrospinning setup with microfiber-assisted rotating collector. In this method the microfibers are coated by nanofibers this is done by depositing the nanofibers over the surface of microfibers. The coating of nanofibers around the microfibers is done by slowly moving the microfibers towards the small collector which rotates around the fibers that ultimately force nanofibers to twist around the microfibers to form a coat. The aligned and un-fragmented threads were formed due to this alteration in single or multi-polymer mixtures, which may then be interwoven to fabricate a woven nanofiber scaffold.68
2.1.2.6 Electrospinning setup with rotating frozen mandrel. This method was first introduced by Marc Simonet, Oliver D. Schneider and their coworkers. In this method, all the electrospinning parameters like rotation speed of the drum, relative humidity, and polymer flow rate are kept constant but the only variation is made in the temperature of the rotating drum collector. The temperature variations are made ranging between 300 and 200 K. Cooling of the collector tube is done by placing dry ice in the tube.69 The porosity of the formed nanofibers depends on the temperature and humidity. The results of PLGA nanofibers obtained were found that at room temperature of about 30 °C (300 K) the porosity was found around 79% at 30% of RH. But these results are varied by changing the humidity and the temperature. At temperature of 200–220 K and RH of 15, 30, 50, 85 the porosity was found to be 66%, 90%, 91%, 88% respectively.70
2.1.2.7 Electrospinning setup with parallel conducting collector. In this method, two conducting plates are placed in the gap available below the needle. These plates are substrate-free and negatively charged. Due to the static charge present on the electrospun nanofiber and the negative charge on the plates attracts the fibers which are positively charged towards the plates. Moving the jet forward and backward in the gap decides the length of the nanofiber be fabricated as shown in Fig. 6e.71 The same charges present on the nanofiber surface create the repulsive force and enhance their parallel distribution. As the aligned fiber collection is achieved within the air gap it is used in tissue engineering without any contamination from the grounded electrodes which is the major source of contamination.72
2.1.2.8 Electrospinning setup with patterned electrodes. Electrospinning setup with patterned electrodes is the advancement of the previous method (parallel conducting electrodes) difference being that the additional two conducting electrodes are added to create the multilayered mat having a controlled hierarchical structure of the fabricated nanofibers. The spatial orientation of the fibers obtained in this method is due to the uniaxial direct deposition of the polymer solution in the gap. Also, it is possible to deposit a multilayered mat of the prepared nanofibers by controlling the applied voltage which produces nanofibers having different directions. The only disadvantage of this method is that the nanofibers formed by this method are having less thickness (as shown in Fig. 6f).73
2.1.2.9 Electrospinning setup with drum collector and rotating wire. In this technique, the nanofibers are collected on the drum which is made up of parallel copper wires of a diameter of 8 cm and are separated from each other by a distance of 0.8 cm (as shown in Fig. 6g). the syringe containing electrospinning solution is mounted upside of the drum at the distance of 12 cm and the mixture of polymer is forced through the needle with the flow rate of around 50 μm min−1 having applied voltage of 15 kV. The fabricated nanofibers are then placed into the vacuum oven and dried at 50 °C to remove the residual solvent.74Through necked eyes, the single nanofiber cannot be seen, but the sheath of nanofiber created perpendicular to the symmetry axis can be viewed.75 The collector's constant rotation overcomes the constraint of short-length fragment fiber deposition of a few millimeters. However, with this design, problems with fiber separation are always a worry.76
2.1.2.10 Electrospinning setup with a rotating thin disk. The rotating disk method consists of a thin metal disc of diameter 1 mm which is used as a fiber generator. The rotating disc is immersed in a solution of polymer underneath (as shown in Fig. 6h).73 The assembly consists of a collector which is also made up of metal placed just above the rotating disc having applied voltage to avoid electrical interferences. The formed nanofibers bear a sufficient electric charge which helps to repel the two nanofibers from each other.77The only disadvantage associated with this method is that this method produces a small surface area for the deposition of the fibers as well as loss of alignment due to the deposition of fibers in a 3D direction.72
2.1.2.11 Electrospinning setup with double-ring collector. The two rings are made up of aluminum SEM stubs with a diameter of approximately 12.5 mm covered with carbon tape. Grounding is done for both the rings with the copper wire. The distance between the two ring collectors is around 60 nm and is shielded with rubber septa. The potential of 15 kV is applied to the rings. Amongst the rings, one is rotated at the speed of 2500 rpm for up to 60 seconds to form the nanofiber between two collector rings.78 Bellow mentioned Fig. 6i shows the electrospinning setup with a double ring collector.
2.1.2.12 Electrospinning setup with water bath grounded collector. E. Smit et al. (2005) used an electrospinning setup with a water bath grounded collector. They used polyvinyl acetate (PVA), polyvinylidene difluoride, and polyacrylonitrile polymers for nanofiber production.79 Electrospinning setup with water bath grounded collector incorporates a water surface that is open to amass nanofibers. Depending on the objective of the deposition, this bath may contain a variety of solvents.67 This method consists of a Petri plate (150 × 15 mm) containing a water bath and the collector electrode (140 × 0.42 mm) immersed in distilled water in the Petri plate at the bottom of the plate. This immersed collector plate is then grounded through thin copper wire. The electrospinning solution is filled in the glass micropipette having an opening of 0.5 mm and forced according to gravity flow with the flow rate of 1 ml h−1. The formed nanofibers in the Petri plate are then pulled throughout the water bath with the help of a revolving collector drum as shown in Fig. 7a.79
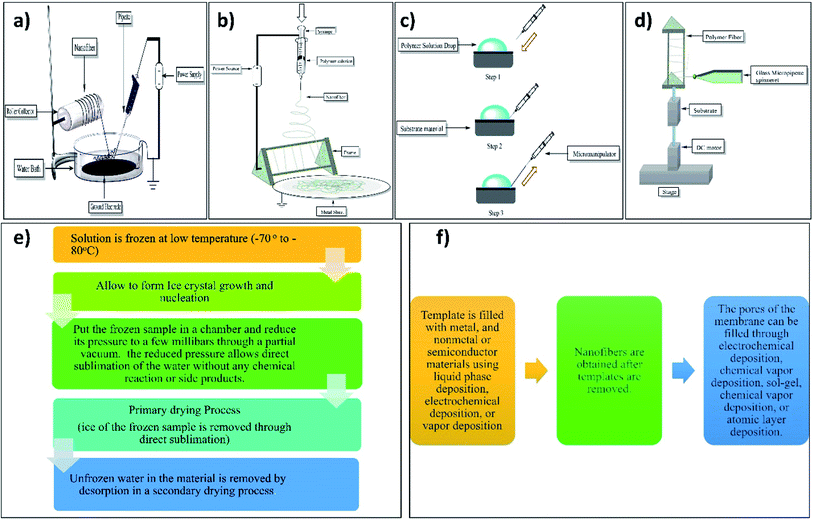 |
| Fig. 7 Illustration of setup used for fabrication of nanofibers by (a) water bath grounded collector electrospinning, (b) electrospinning with a frame collector, (c) drawing method of nanofiber fabrication, (d) spinneret-based tunable engineered parameters (continuous technique), (e) steps involved in the freeze-drying and (f) general preparatory procedures of the template method. | |
2.1.2.13 Electrospinning setup with frame collector. This method consists of the spinneret having a metal frame which may be of aluminum with an applied voltage of 0–60 kV. As shown in Fig. 7b the electrospinning solution is forced on the spinneret with the flow rate (polymer solution) of 2 ml h−1 for the formation of the nanofiber yarn.80 By this method, one can fabricate a single nanofiber for characterization.81
2.2 Non-electrospinning methods
2.2.1 Interfacial polymerization method of nanofiber fabrication. This method contains the mixing of two solvents one containing 1 M HCl (20 ml) which contains 4 mmol of ammonium persulfate and another solution containing 20 ml of chloroform which contains 4 mmol of aniline. These two solutions are mixed on magnetic stirrers for 1 hour.After the mixing of the two solutions, the oxidant-based solution is added to the aniline solution. The reaction took place after a while and at the interface of the two solutions of polymer the polyaniline was formed; which increased over the period.82
2.2.2 Drawing method of nanofiber fabrication. Nanofibers are created using a device known as a micromanipulator in this process. The micropipette is used to fabricate nanofibers. A millimeter drop of polymer solution is deposited on the substrate material, as illustrated in Fig. 7c. Depending on the kind of polymer employed, the micropipette tip is touched at the drop's edge and returned at a certain pace. The diameter of the generated nanofibers is mostly determined by the material composition, drawing speed, and solvent evaporation rate. As the electrospinning methods could not provide the single fiber; the drawing method has the major advantage in that it provides the single nanofiber which makes it easy to determine the properties of a single nanofiber.83
2.2.3 Self-assembly method of nanofiber fabrication. The theory of the self-assembly process is that non-covalent forces such as hydrophobic forces, hydrogen bonding, and electrostatic interactions organize and arrange molecules into certain patterns or structures.84The method employs electrostatic repulsion forces in the conductive fibers with the applied field to produce fine nanofibers, similar to the principle of conventional electrospinning. The only difference in the proposed method is it uses electrostatic repulsion forces in the conductive fibers with the applied field, with only the tips of the formed nanofibers remaining separated. These tips are then mechanically linked to the freshly produced nanofiber by electrospinning's mechanical whipping action, which twists the charged nanofiber into a bundle along its longitudinal axis to form the yarn.85
The supramolecular hydrogels were constructed by self-assembling the small molecules using weak interaction forces including hydrophobic and hydrogen bonding interactions. The sole drawback of this technology is that it can only tiny active molecules to fabricate nanofibers that can self-assemble or respond to an external interaction.86 For the fabrication of tiny nanofibers <100 nm up to micrometer in length, this method is excellent in terms of fabrication of such.41
2.2.4 Freeze-drying method of nanofiber fabrication. This approach, also known as solid–liquid phase separation/ice segregation-induced self-assembly, is used to create fibers. Freeze drying gives an upper hand over the conventional drying method. As a result, the production of nanofibers has gained a lot of interest.87,88 The freeze-drying procedure produced ultrafine precursors, which were crucial in porous nanofibers synthesis with smaller diameters ranging from 20–60 nanometers and having high aspect ratios. The pyrolysis parameters were used to modify the pore volume and specific surface area of the ultrafine nanofibers that were produced. All of these properties make porous nanofibers intriguing for hydrogen storage, water treatment, catalyst support, and many other applications.89 The steps involved in the freeze-drying method of fabrication of nanofibers are illustrated in the below Fig. 7e.
2.2.5 Template method of nanofiber fabrication. One of the most often used methods for preparing MNFs is the template technique. The needed material is synthesized within the holes of a template membrane with evenly sized cylindrical pores in this method.90 The template method's general preparatory procedures are as shown in Fig. 7f.By this approach, the diameter of the nanofibers may be modified by adjusting the specifications of the templates, and the preparation conditions aren't as stringent. The carbon nanotube (CNT), molecular hoof, macromolecule compound, microporous glass, alumina template, surfactant, and porous polymer template are all common templates used for fabrication.91,92
2.2.6 Phase separation method of nanofiber fabrication. Thermal phase separation at low temperatures is more probable than liquid–solid phase separation to create porous nanofiber membranes. It is a method of dissolving a polymer in mixed solvents and generating a homogenous mixture in a film-forming mixture at a temperature below the melting point of the polymer. To achieve the phase separation the above film-forming mixture is cooled. In the freeze-drying technique, this low-temperature phase separation technique can also be initiated for the building of porous nanofibers.93 As per the kind of solvent employed, the phase separation methods can be divided into EFD (emulsion freeze-drying), HFD (hydrogel freeze-drying), and SFD (solution freeze-drying) methods.94 The o/w proportion, the energy of polymer mixture, and MW of polymer are the major processing variables in this technique.95
2.2.7 STEP (spinneret-based tunable engineered parameters) method of nanofiber fabrication. The number of surface characteristics that impact cell activity including the size of nanofiber, geometrical spacing, and architecture of fiber, can be investigated using the step technology. In a bottom-up assembly context, the suggested spinneret-based tunable engineered parameters (step) approach allows flexibility in creating nanofiber scaffolds. With respect to template and phase separation method having limitation fiber deposition with different hierarchical levels. This technique produces nanofibers with controlled dimensions with tunable spacing and aligned configuration. The step technique is further divided into two techniques such as point-to-point custom technique and continuous technique for fabrication of nanofiber scaffold.
2.2.7.1 Point to point custom STEP technique. The polymer drop is deposited on the substrate's surface while the pipette is positioned perpendicularly on the nanopositioner in the point-to-point custom procedure. Each time the pipette is contacted to the surface of the polymer droplet by lifting the substrate to the tip of the pipette and stretching horizontally creating nanofiber, the pipette is touched to the surface of the substrate forming dual attachment. The glass micropipette after pulling the fiber is brought back into contact with the substrate, generating a suspended nanofiber.96
2.2.7.2 Continuous STEP technique. The substrate is attached to the dc motor, which is installed on the nano positioner, in this continuous process for fabricating nanofiber scaffolds. The spinneret of the glass micropipette is set perpendicular to the substrate. The glass micropipette draws fibers constantly on the revolving substrate. The vertical speed of the nano positioner is controlled to achieve the appropriate geometrical separation between the manufactured nanofibers. Multilayers are synthesized over the preceding layer once a single layer of nanofibers has been completed. Below mentioned Fig. 7d shows the continuous technique of spinneret-based tunable engineered parameters.96
3. Application of electrospun nanofibers
Nanofibers have wide applications in pharmaceuticals as well as in others. Some of the applications are listed below.
3.1 Biomedical applications
3.1.1 Drug delivery. Various types of drugs are extensively delivered with the use of electrospun nanofibers because nanofibers impart distinctive favor in the field of drug delivery by acting as; biodegradability, easily fabricating at a large scale, attain control release of drugs, extensive surface-area-to-volume ratio, etc. Various forms of controlled release drugs are integrated into electrospun nanofibers such as; Avandia, eprosartan, carvedilol, hydrochlorothiazide, aspirin, naproxen, nifedipine, indomethacin, ketoprofen, etc.97Drug delivery with the help of nanofibers is conveniently classified into drug loading and drug release mechanisms. Drug loading normally means the entrapment of a drug into the nanofibers to control the release of that drug and to provide site-specificity to the drug; whereas, the drug release mechanism is known about the: demagnetization from fiber surface, fibers diffusion through solid-state, and in vivo fiber degradation (Fig. 8).98
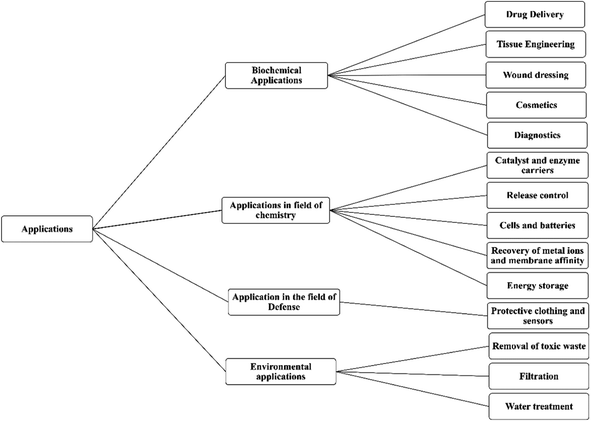 |
| Fig. 8 Summarizes the numerous applications of nanofiber/nanofiber mats. | |
In a recent study it is shown that the nanofibers made up of carbon nanotubes (CNTs) make a hybrid of nanofibers which are used widely for delivery of doxorubicin (dox); which was incorporated in the CNTs nanofibers chemically, and its encapsulation efficiency is very good, i.e., 83.7% and the PLGA polymer solution were mixed in 3 different ratios with dox particles to form an appropriate nanofiber by electrospinning. The encapsulation of CNTs in PLGA does not affect the structure of the PLGA nanofibers but it increases the mechanical strength of the nanofibers. The CNTs nanofibers avoided the burst release of dox, but it also provides controlled delivery of dox over 42 days.99
Various drugs found in modified form including protein and peptides, antibodies, and tiny molecular drugs were incorporated into the nanofibers to deliver them at their target site and get more desirable outcome with effective therapeutic actions. For incorporating hydrophobic drug into the nanofibers, they primarily dissolves into the organic solvent along with the polymer. It is also feasible to deliver multi-drug systems along with time programmed release.100
3.1.2 Tissue engineering. Tissue engineering took a great area of interest and a dramatic and versatile research area in recent days. The purpose of review about tissue engineering scaffolds is to restore, replace, support, or intensify the function of a certain tissue or organ.99It has various applications nowadays, e.g., bio mimic ECM, enzyme, blood vessel, gene, and medicine to restore skin, cartilage, and nerve. And it is excellent in numerous procedures including; biocompatibility, mechanical property, penetrability, and degenerating-ability in the human body.97
Tissue engineering is an amalgamation of cell biology, chemistry, and biomaterials to assemble 3D tissues that impersonate the structure of a biological membrane or ECM (extracellular matrix) that embrace different nanofibrous matrices. Generally, this technique is used for the formation of nanofiber matrices which includes polymer self-build, phase segregation, and electrospinning. We have to incorporate various additives into nanofibers to provide special properties and modify them to get them properly working as ECM to use in tissue.101
Nanofibers expand its application in the field of bone tissue engineering, cartilage tissue engineering, tendon and ligament tissue engineering, neural tissue engineering, cardiovascular tissue engineering etc. which reveals its wide application over the medical surgical field.102
3.1.3 Wound dressing/ligature. The wound healing process is a deliberate process that is the combination of inflammation, proliferation, remodeling phase, and epithelization. Wound dressing normally requires a pore size of 500 to 1000 nm range which is enough to protect the wound from the outer environment or microorganisms.99Normally our body is unable to recover wound that occurs in the deep layer of dermal tissue because the cells which are involved in treating or proliferating wounds get damaged by injury, which results in complete re-epithelization takes place which requires a long duration. A test was done for the open wound healing process i.e. if collagen is used for nanofibrous mat material for covering the wound then the wound is recovered faster than the normal cotton gauze.99,103 Some of the currently available marketed products containing nanofibers are illustrated in Table 1.
Table 1 Enlists currently available nanofiber beds/mats for wound healing
Sr. no. |
Product name |
Company name |
Description |
Website |
1 |
Aeos |
Zeus Industrial Products, Inc. |
Composed of nonwoven fibrous material for sutures |
https://www.zeusinc.com/ |
2 |
HealSmart |
Poly Remedy |
Nanofiber wound dressing contained a drug product to improve wound healing |
https://polyremedy.com/ |
3 |
ResQFoam |
Arsenal Medical |
Core–shell fiber containing foam for the treatment of non-compressible hemorrhage |
https://arsenalmedical.com/ |
4 |
SNC Best |
Stellenbosch Nanofiber Company |
Nonwoven nanofiber mat for medical applications |
https://sncfibers.com/ |
5 |
ReThink |
Stellenbosch Nanofiber Company |
Surgical mask |
https://sncfibers.com/ |
6 |
SpinCare |
NanoMedic |
Skincare is a portable electrospinning system that directly prints temporary skin over the wound |
|
There is a polysaccharide coated nanofibers, kindly made up of a specific polymer i.e. poly caprolactone is used for preparing the modified form of nanofiber termed as multilayered. This kind of nanofibers was formulated by combining electrospinning to layer-by-layer deposition technique. It is a kind of charged fibers which is prepared by deposition of positively charged chitosan along with the accumulation of negatively charged polyelectrolyte over it. This kind of nanofibers is preferable used in the designing biomaterials for wound dressing.104
3.1.4 Cosmetics. There are many cosmetic preparations of nanofibers that have been marketed recently as facemasks, they are also used in treating skin defects or healing various types of skin problems and also used as skin cleansers with adding different ingredients imparts different medicinal properties.97 The nanofiber mat fabricated after incorporation of ingredients like retinoic acid, ascorbic acid, and collagen in the electrospinning solution is used in the treatment of certain skin problems including wrinkles. Although these face masks made from nanofibers containing active ingredients have antioxidant activity, they are wetted before application to the skin, which application on the skin provides a healthy appearance on the skin. Some skin problems like overaccumulation of oil are treated by these nanofiber mats and are also used for the removal of cosmetic ingredients from the skin.105The silver nanoparticle-loaded nanofibers are widely used for the prevention and treatment of bacterial infection, and fungi. Thus, they accelerate the wound healing process. Also, nanofibers containing silk fibroin loaded with human epidermal growth factors accelerate wound healing.106
3.1.5 Diagnosis. Nanofibers have revealed their utilization in the field of detection or recognition as well as cure or treatment. Nanofibers have served as various devices such as ultrasonic biosensors for detection of cancer, diagnosis of malaria, recognition of circulating tumor cells in patients of cancer, and detection of glucose cholesterol, urea, bacteria, etc.31 Biosensors are more effective due to their various benefits such as low-cost, fast detection, and good portability.There are various sensors used in diagnosis such as electrochemical biosensors also known as DNA biosensors use in the detection of proteins, glucose, genes, etc.; gas sensors use for the diagnosis of lung cancer; fluorescent chemo-sensor is used in the diagnosis of peptides, molecular biologics, etc.; immune-sensor use in antigen and antibody recognition, etc.31
In an experiment, the nanofibers are worked to reveal its potential in the detection of leakage of H2S gas. For detecting this poisonous gas, nanofibers are precisely introduced with lead acetate at the time of nanofiber production and the produced nanofiber having higher thermal stability and contrary to aggregation of particles, numerous reaction sites and increase the diffusion of H2S in the nanofibrous 3D network where the H2S reacts with lead acetate and form brownish colored precipitates of lead sulfide. This kind of nanofibers can help in detecting the poisonous H2S gas in human body and indicates the peoples for going to overcome with such stressful situation.107
3.2 Applications in the field of chemistry
3.2.1 Catalyst & enzyme carriers. Nanofibers play an exhaustive role to increase the catalytic activity of enzymes by increasing the surface area of catalysts. They can load a higher number of catalysts and provide good solid support for the enzymes.99Nanofibers are also used as biological catalysts because of their high loading capability and large specific surface area. To incorporate enzymes in electrospun nanofibers there are various ways together; including enzyme grafting on the surface of fibers, physical adsorption, and incorporating enzymes into nanofiber through the electrospinning process succeeded by the crosslinking reaction. Biological catalysts or enzymes should only be incorporated into nanofibers if nanofibers contain at least one reactive polymer to bind with the enzyme. 27.4% monolayer of the surface of nanofiber is supposed to be filled with enzyme and most it is supposed that 65% of enzyme was loaded in native enzyme and shows its hydrolytic activity, whereas; there is an increment of three times in enzyme activity by non-aqueous solution under same condition.97
3.2.2 Release control. Nanofibers provide a very useful tool to control the delivery of many drugs, reduce side effects, and increase patient compliance. It makes great achievement to delivery of drugs in the human body which is induced in nanofibers. It makes the drug release in control and continuous form and avoids burst release of drug.99Various types of nanofibers are used to control the delivery of diverse types of drugs like stimuli-responsive nanofibers fabricated by electrospinning which control the drug delivery based on signals captured by the body under which thermal, pH, light, magnetic field, electric field, and other multiple stimuli-responsive electrospun nanofibers are included.57,108
3.2.3 In the field of cells & batteries. A porous or mesh-like material between two electrodes of a battery provides various benefits to run the batteries smoothly, e.g. preventing short circuits in batteries and making an easy path to move ions freely from one electrode to another, they have high discharge current capacity.99,109 There are various or different types of cells or batteries are which have nanofibers to improve their working efficiency, e.g. fuel cells, discs electrodes, dye-sensitized solar batteries or cells, solar batteries, energy retention devices, etc.110,111
3.2.4 Recovery of metal ions & membranes affinity. In the current scenario water pollution is one of the major drawbacks in our living world. Mainly the pollutants of water pollution are heavy metals or soluble inorganic traces in water bodies which cause early aging or eutrophication in lakes rivers ponds etc. So to overcome these problems as soon as possible through different ways which include non-adsorbents, including magnetic, nanocomposites, and nanofibrous array for abolishing massive metal/metalloids.112Along with all these treatments nanofibers also get the possibility to treat or protect water bodies with these hazardous pollutants since it contains extreme surface-to-volume ratio, good adsorption capacity, high gas permeability, etc. There is recent research about the preparation of polyvinylidene fluoride (PVDF) nanofiber coatings made up of electrospinning techniques that help to provide a microporous separator membrane. With the help of this PVDF nanofibrous coating on the polyolefin membrane, there is an enhancement in the capacity of performance and properties of the Celgard microporous battery separator membrane. They use the force spinning method to fabricate this type of nanofibers which is more beneficial than the electrospinning technique of nanofiber production.
There is a study about the ion exchange nanofibers (IES-NFS), which help to induce a charge on nanofibers and increase its adsorption capacity from oppositely charge-induced atoms or metals ions; thus, improving its recovery of metal and increasing the membrane affinity by inducing a charge on IEX-NFS. With the help of IEX-NFS there is easy recycling or recovery of heavy or expensive metals, catalysts, and enzymes.113
3.2.5 Energy storage. Electrospinning is extensively used in energy storage devices with great interest and have various applications including various type of batteries such as lithium-ion batteries, redox flow batteries, metal–air batteries, and supercapacitors.114Significantly, the use of nanofiber for energy storage devices in many ways such as; lignin can combine with nanofibers to produce carbon nanofibers network which had a very large surface area that produces great potential to nanofibers by which they will be used in energy storage applications. Till now there is very limited research was done on lignin efficiency to know its capacity to store energy. Once, lignin carbon nanofiber is used as anode materials in lignin-containing batteries. According to some studies, the lens is greatly influenced by its carbonization capacity which affects its electrochemical properties.115
3.3 Application in the field of defense
3.3.1 Protective clothing. For many years normally, humans use protective clothing to protect our bodies from different types of unusual or unbearable environmental conditions such as heat, cold, rain, etc.; and such clothing must have good breathable properties. There are maximal manufacturing of bio-degradable protecting clothing has been done to keep our environment healthy and clean and there must be used advanced materials in such types of clothing to get the exigency of effective clothing for outdoor activities, including for business and industrial clothing and having nanofibers pore size of 50 nm.116Protective clothing is also used to define the requirement of our defense system, sports, or industrial applications through which our textile industries used to manufacture bulletproof clothing, cloths that must protect humans from external chemical or biological agents, fire, cold, heat, etc. Generally, the most basic thing that must be kept in mind during the manufacturing of protective clothing is that the clothes must have low weight, low cost, and comfortable clothing systems to provide superior performance.117 Some of the currently available marketed products in the textile and clothing industry containing nanofibers are illustrated in Table 2.
Table 2 Tabulates the marketed nanofiber used in the textile and clothing industry
Sr. no. |
Name of product |
Company name |
Description |
Website |
1 |
Nexture |
Lime Nano |
Nanofiber membrane (10−9 m) laminated clothing for water and wind protection |
https://limenano.com/ |
2 |
Wetlaid Fabrics |
Hirose Paper Mfg. Co. |
Nonwoven nanofiber fabrics |
https://www.hirose-paper-mfg.co.jp/ |
3 |
NanoDream |
NanoLayr |
Pillow lining made of nanofiber clothing |
https://www.nanolayr.com/ |
3.3.2 Sensors. In the defensive field nanofibers are used with the purpose of providing protection and survivability of the peoples who are living in stressful situations and dangerous areas to cope up with them. For such need in the field of defense nanofibers are incorporated with some sensors which are used to detect various kind of danger in case of metallic, electrolytic, or in chemical form. This kind of sensors can also sense some kind of hazardous and poisonous gases.118,119According to a recent research, a sensor incorporated nanofibers was made by using carbon nanotubes, which having super strong, tough, and flexible fabrics nanofiber with ballistic property is fabricated. This kind of sensors is introduced into the wearing material which is very light weighted generally made up of cottony material and proved to be bullet proof as carbon nanotubes are light, tough, and extensively strong.120
Nanofibers also used as shielded material by incorporating various polymers which were used to protect the body from electromagnetic rations and UV exposure of sun. The photopolymerized thin coating of graphene nanoparticles and iron incorporated nanoparticles were introduced into the nanofibers which were fabricated by electrospinning technique. According to the authors knowledge this is the first simultaneous coating achieved for protecting the defense peoples by mitigating radiation pollution, strain sensing and environmental heating.121
3.4 Environmental applications
3.4.1 Removal of toxic wastes. There is the fabrication of alumina containing nanofibers which help to adsorb heavy metals from their immediate environment. These nanofibers were formulated by using the electrospinning method in which PVP solution (10% w/v) was prepared by using ethanol in which PVP polymer powder was dissolved in it under constant stirring, then aluminum acetate solution was mixed with that solution, in which aluminum acetate is used as aluminum precursor; the ratio of precursor to PVP solution is 3
:
1. The resulting alumina nanofibers were used to adsorb chromium(IV) as well as fluoride ions from an aqueous environment.122,123Textile wastes are the greatest environmental issue in these days which reveals one-fifth of industrial water pollutant all over the world. In textile industries the use of hazardous chemicals, heavy metals like formaldehyde as well as toxic gases like sulphur dioxide were on increasing demand and their remnants were disposed off in water on daily basis which decreased the quality of water and soil regularly as a result our environment get polluted and getting damaged by this kind of applications. Therefore, the nanofibers introduced with pollutants repellants were widely used in textile industries to recover these heavy losses.124
3.4.2 Filtrations. Filters are extensively used in a wide range of household works, health care, industries, etc. For filtering various types of unwanted particles or to protect the environment from various particles such as pollutants present in air or water.99A study showed the differentiation among a nylon-6 electrospun membrane (with a thickness of 100 μm, a pore size of 0.24 μm) and a commercial HEPA filter with a thickness of 500 μm and pore size of 1.7 μm utilizing 300 nm test particles indicated that the thin nanofiber membrane had a bit higher filtration efficiency of 99.993% than the HEPA filter (99.97%). There are nanofibers of 16 nm or one-tenth of the range of common nanofibers mesh, which are normally used in filters.97,125
Nanofibers is widely applicable in filter the media present in cabin air filtration of mining vehicles. In cleaning the cabin dust concentration there is a gadget present in nanofibers which helps to circulate the fresh air in and contaminated air out of the cabin. Primarily the cellulosic filters were used for this kind of cleaning which is recently replaced by the cellulose + nanofibrous filters which reduce the dust concentration in respirable gadgets as well as in submicron. Whereas reduction in dust percentage is increased from 68% by the use of cellulosic filter to 92% by using nanofiber + cellulose filter.126
Some of the currently available marketed products containing nanofibers used for air filtration are illustrated in Table 3.
Table 3 Summarizes the marketed products consisting nanofibers for air filtration purposes
Sr. no. |
Product name |
Company name |
Description |
Website |
1 |
BreaSAFE |
Nano4Fibers |
Respirators are made of nanofiber beds that protect from microbes, dust toxic gases, and odors |
https://www.nano4fibers.com/ |
2 |
Exceed |
Espin Tech. |
Air filter made of nanofiber membrane |
https://www.espintechnologies.com/ |
3 |
FilterLayr |
Nanolayr |
Air filtration unit |
https://www.nanolayr.com/ |
3.4.3 Water treatment. In today's era membranes made up of nanofibers are extensively used in various applications, such as desalination, water, and wastewater treatment, membrane separation, oil/water separation, adsorption, etc. In this special type of technology, the selectivity and flux of membrane provide high-performance capacity to nanofibers for separation or water treatment. The nanofibers sheets are either used alone or in association with other materials to provide hybrid performance to the nanofiber membrane for providing membrane-based processes such as membrane distillation, ultrafiltration, microfiltration, nanofiltration, and reverse osmosis.127,128 Some of the currently available marketed products containing nanofibers used for water filtration are illustrated in Table 4.
Table 4 Contains marketed products consisting nanofibers used for water treatment
Sr. no. |
Product name |
Company name |
Description |
Website |
1 |
ProTura |
Parker |
Cellulose nanofiber filtration assembly in cartilage filtration unit |
https://www.parker.com/ |
2 |
Nanofiber Filter |
Astral Pool |
Self-cleansing cartridge filter |
https://www.astralpool.com/en/ |
3 |
Return Focus Pod |
IQ Commercials |
Aquatic sound proofing |
https://www.iqcommercial.co.nz/ |
4. Challenges and future perspective
Nanofibers are not much used in the field of cosmetics in spite it has wide applications due to the availability of larger surface area and ease of fabrication. Most nanofibers nowadays are available in the drug delivery and biomedical field but only limited companies are fabricating them for cosmetic purposes.129,130 Stability is the prime challenge in the case of nanofibers. Most of the nanofiber mats are wetted before their application as a cosmetic face mask due to their stability issue. The appropriate additive materials are required along with biopolymers to improve the processing also post-processing is required without disturbing bioactivity and intrinsic biocompatibility for enhancement of stability and mechanical strength. The use of cyclodextrins in the nanofibers may improve the stability, solubility and drug release as cyclodextrins encapsulate drug conjugates and forms a non-covalent linkage between cyclodextrin and drug conjugate. Also, complexing cyclodextrins with the biopolymers may improve the biocompatibility, digestibility, biodegradability and improved biological response of nanofiber mat/bed.131
Despite the wide advantages of electrospun nanofibers, they have some limitations including less cellular infiltration inside and smaller pore size. Several types of research were made to tackle these challenges like multilayering, blending by polymers having different degradation behavior, and inclusion of Hypersil in the nanofiber mat.132
5. Conclusion
Over the past few decades electrospinning is grown rapidly and also nowadays it is the major attraction of many industrial and academic researchers for the therapeutic delivery system. The efforts of many researchers towards eliminating the drawbacks of the electrospinning process and inventing an advanced setup of electrospinning are appreciable. Major modifications have been made to neglect the problems in industrial fiber production and enhance fiber quality. Electrospinning is now capable to provide the controlled fiber diameter by using various polymer grades. Some major advancements are also made in the electrospinning setup to provide hollow nanofibers. The applications of nanofibers in various fields are increasing constantly due to their advantages over other nanotechnological therapies also the fact of consideration that the nanofibers are efficacious over microfibers. It is considered that besides their applications the potential of nanofibers is still to be explored and researchers continuously modify the electrospinning setup and the electrospinning parameters to assess the controlled unit fiber morphology of interest. The area of electrospun nanofiber is grabbed majorly by the polymer industries and textile engineering. In the future, electrospinning is supposed to be the bigger area for the fabrication of nanomaterials with the enumorus applications.
Conflicts of interest
There is no conflict of interest and disclosures associated with the manuscript.
References
- S. E. McNeil, Nanotechnology for the biologist, J. Leukocyte Biol., 2005, 78, 585–594, DOI:10.1189/jlb.0205074.
- N. Hasan, M. Imran, P. Kesharwani, K. Khanna, R. Karwasra, N. Sharma, S. Rawat, D. Sharma, F. J. Ahmad, G. K. Jain, A. Bhatnagar and S. Talegaonkar, Intranasal delivery of Naloxone-loaded solid lipid nanoparticles as a promising simple and non-invasive approach for the management of opioid overdose, Int. J. Pharm., 2021, 599 DOI:10.1016/j.ijpharm.2021.120428.
- A. Sheikh, S. Md and P. Kesharwani, Aptamer grafted nanoparticle as targeted therapeutic tool for the treatment of breast cancer, Biomed. Pharmacother., 2022, 146, 112530, DOI:10.1016/J.BIOPHA.2021.112530.
- V. Singh, S. Md, N. A. Alhakamy and P. Kesharwani, Taxanes loaded polymersomes as an emerging polymeric nanocarrier for cancer therapy, Eur. Polym. J., 2022, 162, 110883, DOI:10.1016/J.EURPOLYMJ.2021.110883.
- A. Sheikh, S. Md and P. Kesharwani, RGD engineered dendrimer nanotherapeutic as an emerging targeted approach in cancer therapy, J. Controlled Release, 2021, 340, 221–242, DOI:10.1016/J.JCONREL.2021.10.028.
- G. Adlakha-Hutcheon, R. Khaydarov, R. Korenstein, R. Varma, A. Vaseashta, H. Stamm and M. Abdel-Mottaleb, Nanomaterials, Nanotechnology Applications, Consumer Products, and Benefits, Nanomater. Risks Benefits, 2009, p. 195 Search PubMed.
- K. Khanna, N. Sharma, S. Rawat, N. Khan, R. Karwasra, N. Hasan, A. Kumar, G. K. Jain, D. K. Nishad, S. Khanna, H. Popli and A. Bhatnagar, Intranasal solid lipid nanoparticles for management of pain: a full factorial design approach, characterization & Gamma Scintigraphy, Chem. Phys. Lipids, 2021, 236, 105060, DOI:10.1016/j.chemphyslip.2021.105060.
- R. Chadar, O. Afzal, S. M. Alqahtani and P. Kesharwani, Carbon nanotubes as an emerging nanocarrier for the delivery of doxorubicin for improved chemotherapy, Colloids Surf., B, 2021, 208, 112044, DOI:10.1016/J.COLSURFB.2021.112044.
- Y. Nitheesh, R. Pradhan, S. Hejmady, R. Taliyan, G. Singhvi, A. Alexander, P. Kesharwani and S. K. Dubey, Surface engineered nanocarriers for the management of breast cancer, Mater. Sci. Eng., C, 2021, 130, 112441, DOI:10.1016/J.MSEC.2021.112441.
- V. Singh and P. Kesharwani, Dendrimer as a promising nanocarrier for the delivery of doxorubicin as an anticancer therapeutics, J. Biomater. Sci., Polym. Ed., 2021, 1–29, DOI:10.1080/09205063.2021.1938859.
- H. Kaur and P. Kesharwani, Advanced nanomedicine approaches applied for treatment of skin carcinoma, J. Controlled Release, 2021, 337, 589–611, DOI:10.1016/J.JCONREL.2021.08.003.
- V. Singh, A. Sahebkar and P. Kesharwani, Poly (propylene imine) dendrimer as an emerging polymeric nanocarrier for anticancer drug and gene delivery, Eur. Polym. J., 2021, 158, 110683, DOI:10.1016/J.EURPOLYMJ.2021.110683.
- R. Chadar, A. Sheikh and P. Kesharwani, Nanotechnology-based siRNA delivery strategies for treatment of triple negative breast cancer, Int. J. Pharm., 2021, 605, 120835, DOI:10.1016/J.IJPHARM.2021.120835.
- B. Surekha, N. S. Kommana, S. K. Dubey, A. V. P. Kumar, R. Shukla and P. Kesharwani, PAMAM dendrimer as a talented multifunctional biomimetic nanocarrier for cancer diagnosis and therapy, Colloids Surf., B, 2021, 204, 111837, DOI:10.1016/j.colsurfb.2021.111837.
- A. R. Singh, P. K. Desu, R. K. Nakkala, V. Kondi, S. Devi, M. S. Alam, H. Hamid, R. B. Athawale and P. Kesharwani, Nanotechnology-based approaches applied to nutraceuticals, Drug Delivery Transl. Res., 2021, 1–15, DOI:10.1007/s13346-021-00960-3.
- D. Modi, J. Nirmal, M. H. Warsi, M. Bhatia, N. Hasan, P. Kesharwani and G. K. Jain, Formulation and development of tacrolimus-gellan gum nanoformulation for treatment of dry eye disease, Colloids Surf., B, 2022, 211, 112255, DOI:10.1016/J.COLSURFB.2021.112255.
- M. Z. Ahmed, A. Gupta, M. H. Warsi, A. M. A. Ali, N. Hasan, F. J. Ahmad, A. Zafar and G. K. Jain, Nano Matrix Soft Confectionary for Oral Supplementation of Vitamin D: Stability and Sensory Analysis, Gels, 2022, 8, 250, DOI:10.3390/GELS8050250.
- N. Hasan, M. Imran, D. Jain, A. Shamim, S. Beg, P. Kesharwani, G. Jain and F. J. Ahmad, Rapid analytical method development and validation for the simultaneous estimation of 5-Fluorouracil and Cannabidiol in plasma and lipid-based nanoformulations, Curr. Anal. Chem., 2022, 18 DOI:10.2174/1573411018666220304085236.
- M. Fatima, A. Sheikh, N. Hasan, A. Sahebkar, Y. Riadi and P. Kesharwani, Folic acid conjugated poly(amidoamine) dendrimer as a smart nanocarriers for tracing, imaging, and treating cancers over-expressing folate receptors, Eur. Polym. J., 2022, 170, 111156, DOI:10.1016/J.EURPOLYMJ.2022.111156.
- N. Hasan, M. Imran, D. Jain, A. Shamim, S. Beg, P. Kesharwani, G. Jain and F. J. Ahmad, Rapid analytical method development and validation for the simultaneous estimation of 5-Fluorouracil and Cannabidiol in plasma and lipid-based nanoformulations, Curr. Anal. Chem., 2022, 18 DOI:10.2174/1573411018666220304085236.
- A. Nikhat, N. Hasan, Z. Iqbal, P. Kesharwani and S. Talegaonkar, Enhanced transdermal delivery of lutein via nanoethosomal gel: formulation optimization, in vitro evaluation, and in vivo assessment, J. Drug Delivery Sci. Technol., 2022, 103447, DOI:10.1016/J.JDDST.2022.103447.
- U. A. Khan, U. Parveen, N. Hasan, M. Z. Ahmed, S. Saad, F. J. Ahmad and G. K. Jain, Parenteral sustained release lipid phase-transition system of ziprasidone: fabrication and evaluation for schizophrenia therapy, Drug Des., Dev. Ther., 2020 DOI:10.2147/DDDT.S247196.
- N. Hasan, M. Imran, A. Sheikh, S. Saad, G. Chaudhary, G. K. Jain, P. Kesharwani and F. J. Ahmad, Cannabis as a potential compound against various malignancies, legal aspects, advancement by exploiting nanotechnology and clinical trials, J. Drug Targeting, 2022, 30(7), 709–725, DOI:10.1080/1061186X.2022.2056188.
- N. Hasan, M. Imran, A. Sheikh, S. Saad, G. Chaudhary, G. K. Jain, P. Kesharwani and F. J. Ahmad, Cannabis as a potential compound against various malignancies, legal aspects, advancement by exploiting nanotechnology and clinical trials, J. Drug Targeting, 2022, 30(7), 709–725, DOI:10.1080/1061186X.2022.2056188.
- G. Chaudhary, N. Hasan, A. K. Malik, V. Singh, K. Raza, F. J. Ahmad, P. Kesharwani and G. K. Jain, Recent update of 3D printing technology in pharmaceutical formulation development, J. Biomater. Sci., Polym. Ed., 2021, 32, 2306–2330, DOI:10.1080/09205063.2021.1967702.
- X. Chen, L. Cheng, H. Li, A. Barhoum, Y. Zhang, X. He, W. Yang, M. M. Bubakir and H. Chen, Magnetic Nanofibers: Unique Properties, Fabrication Techniques, and Emerging Applications, ChemistrySelect, 2018, 3, 9127–9143, DOI:10.1002/slct.201702480.
- H. Shaghaleh, X. Xu and S. Wang, Current progress in production of biopolymeric materials based on cellulose, cellulose nanofibers, and cellulose derivatives, RSC Adv., 2018, 8, 825–842, 10.1039/C7RA11157F.
- R. H. Müller and C. M. Keck, Drug delivery to the brain-realization by novel drug carriers, J. Nanosci. Nanotechnol., 2004, 4, 471–483, DOI:10.1166/jnn.2004.078.
- X. Shen, M. Liu, F. Song and X. Meng, Structural evolution and magnetic properties of SrFe12O19 nanofibers by electrospinning, J. Sol-Gel Sci. Technol., 2010, 53, 448–453, DOI:10.1007/s10971-009-2119-7.
- H. Wu, W. Pan, D. Lin and H. Li, Electrospinning of ceramic nanofibers: fabrication, assembly and applications, J. Adv. Ceram., 2012, 1, 2–23, DOI:10.1007/s40145-012-0002-4.
- P. Prabhu, Nanofibers for Medical Diagnosis and Therapy, 2019, DOI:10.1007/978-3-319-53655-2_48.
- V. Thavasi, G. Singh and S. Ramakrishna, Electrospun nanofibers in energy and environmental applications, Energy Environ. Sci., 2008, 1, 205–221, 10.1039/B809074M.
- Z. Chan, Z. Chen, A. Zhang, J. Hu, X. Wang and Z. Yang, Electrospun nanofibers for cancer diagnosis and therapy, Biomater. Sci., 2016, 4, 922–932, 10.1039/c6bm00070c.
- T. Subbiah, G. S. Bhat, R. W. Tock, S. Parameswaran and S. S. Ramkumar, Electrospinning of nanofibers, J. Appl. Polym. Sci., 2005, 96, 557–569, DOI:10.1002/app.21481.
- J. Sheng, M. Zhang, W. Luo, J. Yu and B. Ding, Thermally induced chemical cross-linking reinforced fluorinated polyurethane/polyacrylonitrile/polyvinyl butyral nanofibers for waterproof-breathable application, RSC Adv., 2016, 6, 29629–29637, 10.1039/C5RA27913E.
- I. Alghoraibi and S. Alomari, Handbook of Nanofibers, 2020, DOI:10.1007/978-3-319-42789-8.
- D. W. Hutmacher and P. D. Dalton, Melt electrospinning, Chem.–Asian J., 2011, 6, 44–56, DOI:10.1002/asia.201000436.
- S. I. Abd Razak, I. F. Wahab, F. Fadil, F. N. Dahli, A. Z. Md Khudzari and H. Adeli, A review of electrospun conductive polyaniline based nanofiber composites and blends: processing features, applications, and future directions, Adv. Mater. Sci. Eng., 2015, 2015, 356286, DOI:10.1155/2015/356286.
- J. Yuan, Y. Xu and A. H. E. Müller, One-dimensional magnetic inorganic–organic hybrid nanomaterials, Chem. Soc. Rev., 2011, 40, 640–655, 10.1039/c0cs00087f.
- I. Hayati, A. Bailey and T. F. Tadros, Investigations into the mechanism of electrohydrodynamic spraying of liquids: II. Mechanism of stable jet formation and electrical forces acting on a liquid cone, J. Colloid Interface Sci., 1987, 117, 222–230, DOI:10.1016/0021-9797(87)90186-X.
- W.-J. Li, R. M. Shanti and R. S. Tuan, Electrospinning Technology for Nanofibrous Scaffolds in Tissue Engineering, 2007, DOI:10.1002/9783527610419.ntls0097.
- C. Zhang, F. Feng and H. Zhang, Emulsion Electrospinning: Fundamentals, Food Applications and Prospects, Elsevier Ltd, 2018, DOI:10.1016/j.tifs.2018.08.005.
- H. Qu, S. Wei and Z. Guo, Coaxial electrospun nanostructures and their applications, J. Mater. Chem. A, 2013, 1, 11513–11528, 10.1039/C3TA12390A.
- O. O. Dosunmu, G. G. Chase, W. Kataphinan and D. H. Reneker, Electrospinning of polymer nanofibres from multiple jets on a porous tubular surface, Nanotechnology, 2006, 17, 1123–1127, DOI:10.1088/0957-4484/17/4/046.
- A. L. Yarin, Coaxial electrospinning and emulsion electrospinning of core-shell fibers, Polym. Adv. Technol., 2011, 22, 310–317, DOI:10.1002/pat.1781.
- J. Song, Z. Li and H. Wu, Blowspinning: A New Choice for Nanofibers, ACS Appl. Mater. Interfaces, 2020, 12, 33447–33464, DOI:10.1021/acsami.0c05740.
- A. Barhoum, K. Pal, H. Rahier, H. Uludag, I. S. Kim and M. Bechelany, Nanofibers as new-generation materials: from spinning and nano-spinning fabrication techniques to emerging applications, Appl. Mater. Today, 2019, 17, 1–35, DOI:10.1016/j.apmt.2019.06.015.
- R. Aminyan and S. Bazgir, Fabrication and characterization of
nanofibrous polyacrylic acid superabsorbent using gas-assisted electrospinning technique, React. Funct. Polym., 2019, 141, 133–144, DOI:10.1016/j.reactfunctpolym.2019.05.012.
- F. E. Ahmed, B. S. Lalia and R. Hashaikeh, A review on electrospinning for membrane fabrication: challenges and applications, Desalination, 2015, 356, 15–30, DOI:10.1016/j.desal.2014.09.033.
- Y. Liu, X. Zhang, Y. Xia and H. Yang, Magnetic-field-assisted electrospinning of aligned straight and wavy polymeric nanofibers, Adv. Mater., 2010, 22, 2454–2457, DOI:10.1002/adma.200903870.
- M. Abbasipour and R. Khajavi, Nanofiber bundles and yarns production by electrospinning: a review, Adv. Polym. Technol., 2013, 32, 1–9, DOI:10.1002/adv.21363.
- W. E. Teo, R. Inai and S. Ramakrishna, Technological advances in electrospinning of nanofibers, Sci. Technol. Adv. Mater., 2011, 12, 013002, DOI:10.1088/1468-6996/12/1/013002.
- D. Lv, M. Zhu, Z. Jiang, S. Jiang, Q. Zhang, R. Xiong and C. Huang, Green Electrospun Nanofibers and Their Application in Air Filtration, Macromol. Mater. Eng., 2018, 303, 1–18, DOI:10.1002/mame.201800336.
- J. Lyons and F. Ko, Melt electrospinning of polymers: a review, Polym. News, 2005, 30, 170–178, DOI:10.1080/00323910500458666.
- B. Zhang, X. Yan, H. W. He, M. Yu, X. Ning and Y. Z. Long, Solvent-free electrospinning: opportunities and challenges, Polym. Chem., 2017, 8, 333–352, 10.1039/c6py01898j.
- H. Yuan, Q. Zhou and Y. Zhang, Improving fiber alignment during electrospinning, Elsevier Ltd., 2017, DOI:10.1016/B978-0-08-100907-9.00006-4.
- B. Zaarour, L. Zhu, C. Huang and X. Jin, A mini review on the generation of crimped ultrathin fibers via electrospinning: materials, strategies, and applications, Polym. Adv. Technol., 2020, 31, 1449–1462, DOI:10.1002/pat.4876.
- H. Peng, Y. Liu and S. Ramakrishna, Recent development of centrifugal electrospinning, J. Appl. Polym. Sci., 2017, 134, 1–10, DOI:10.1002/app.44578.
- T. Minari, T. Miyadera, K. Tsukagoshi, Y. Aoyagi and H. Ito, Charge injection process in organic field-effect transistors, Appl. Phys. Lett., 2007, 91, 2005–2008, DOI:10.1063/1.2759987.
- D. R. Salem, Electrospinning of nanofibers and the charge injection method, Nanofibers Nanotechnol. Text., 2007, 3–21, DOI:10.1533/9781845693732.1.3.
- H. Liu, S. Mukherjee, Y. Liu and S. Ramakrishna, Recent studies on electrospinning preparation of patterned, core–shell, and aligned scaffolds, J. Appl. Polym. Sci., 2018, 135, 1–11, DOI:10.1002/app.46570.
- Q. P. Le, M. V. Uspenskaya, R. O. Olekhnovich and M. A. Baranov, The mechanical properties of PVC nanofiber mats obtained by electrospinning, Fibers, 2021, 9, 1–12, DOI:10.3390/fib9010002.
- W. E. Teo and S. Ramakrishna, A review on electrospinning design and nanofibre assemblies, Nanotechnology, 2006, 17(14), R89, DOI:10.1088/0957-4484/17/14/R01.
- Y. Zhu, Y. Cao, J. Pan and Y. Liu, Macro-alignment of electrospun fibers for vascular tissue engineering, J. Biomed. Mater. Res., Part B, 2010, 92, 508–516, DOI:10.1002/jbm.b.31544.
- Z. Yang, H. Peng, W. Wang and T. Liu, Crystallization behavior of poly(ε-caprolactone)/layered double hydroxide nanocomposites, J. Appl. Polym. Sci., 2010, 116, 2658–2667, DOI:10.1002/app.
- M. A. Alfaro De Prá, R. M. Ribeiro-do-Valle, M. Maraschin and B. Veleirinho, Effect of collector design on the morphological properties of polycaprolactone electrospun fibers, Mater. Lett., 2017, 193, 154–157, DOI:10.1016/j.matlet.2017.01.102.
- S. Liao, B. Li, Z. Ma, H. Wei, C. Chan and S. Ramakrishna, Biomimetic electrospun nanofibers for tissue regeneration, Biomed. Mater., 2006, 1(3), R45, DOI:10.1088/1748-6041/1/3/R01.
- L. Baldino, S. Cardea and E. Reverchon, A supercritical CO2 assisted electrohydrodynamic process used to produce microparticles and microfibers of a model polymer, J. CO2 Util., 2019, 33, 532–540, DOI:10.1016/j.jcou.2019.08.013.
- J. Diani and K. Gall, Finite Strain 3D Thermoviscoelastic Constitutive Model, Society, 2006, pp. 1–10, DOI:10.1002/pen.20497.
- I. Alghoraibi and S. Alomari, Different methods for nanofiber design and fabrication, Handbook of nanofibers, 2018, pp. 1–46, DOI:10.1007/978-3-319-42789-8_11-2.
- T. Lei, Z. Xu, X. Cai, L. Xu and D. Sun, New Insight into Gap Electrospinning: Toward Meter-long Aligned Nanofibers, Langmuir, 2018, 34, 13788–13793, DOI:10.1021/acs.langmuir.8b03114.
- Y. Maung Maung, Z. M. Myat, T. T. Win and K. K. K. S. Kyaw Soe, Growth and Characterization of Electrospun LaMnO3 Nanofibers by Electrospinning Technique, Am. J. Mater. Sci. Technol., 2013, 24–28, DOI:10.7726/ajmst.2013.1003.
- D. Li, Y. Wang and Y. Xia, Electrospinning Nanofibers as Uniaxially Aligned Arrays and Layer-by-Layer Stacked Films, Adv. Mater., 2004, 16, 361–366, DOI:10.1002/adma.200306226.
- S. Xu, J. Zhang, A. He, J. Li, H. Zhang and C. C. Han, Electrospinning of native cellulose from nonvolatile solvent system, Polymer, 2008, 49, 2911–2917, DOI:10.1016/j.polymer.2008.04.046.
- P. Katta, M. Alessandro, R. D. Ramsier and G. G. Chase, Continuous electrospinning of aligned polymer nanofibers onto a wire drum collector, Nano Lett., 2004, 4, 2215–2218, DOI:10.1021/nl0486158.
- C. Migliaresi, G. A. Ruffo, F. Zomer Volpato and D. Zeni, Advanced Electrospinning Setups and Special Fibre and Mesh Morphologies, Electrospinning Adv. Biomed. Appl. Ther., 2012, pp. 23–68 Search PubMed.
- X. F. Wang and Z. M. Huang, Melt-electrospinning of PMMA, Chin. J. Polym. Sci., 2010, 28, 45–53, DOI:10.1007/s10118-010-8208-9.
- P. D. Dalton, D. Klee and M. Möller, Electrospinning with dual collection rings, Polymer, 2005, 46, 611–614, DOI:10.1016/j.polymer.2004.11.075.
- E. Smit, U. Buttner and R. D. Sanderson, Continuous yarns from electrospun fibers, Polymer, 2005, 46, 2419–2423, DOI:10.1016/j.polymer.2005.02.002.
- Y. H. Wu, H. P. Li, X. X. Shi, J. Wan, Y. F. Liu and D. G. Yu, Effective Utilization of the Electrostatic Repulsion for Improved Alignment of Electrospun Nanofibers, J. Nanomater., 2016, 2016, 2067383, DOI:10.1155/2016/2067383.
- Z. M. Huang, Y. Z. Zhang, M. Kotaki and S. Ramakrishna, A review on polymer nanofibers by electrospinning and their applications in nanocomposites, Compos. Sci. Technol., 2003, 63, 2223–2253, DOI:10.1016/S0266-3538(03)00178-7.
- A. Abdolahi, E. Hamzah, Z. Ibrahim and S. Hashim, Synthesis of uniform polyaniline nanofibers through interfacial polymerization, Materials, 2012, 5, 1487–1494, DOI:10.3390/ma5081487.
- J. Bajakova, J. Chaloupek, D. Lukaš and M. Lacarin, Drawing-the production of individual nanofibers by experimental method, NANOCON 2011 – Conf. Proceedings, 3rd Int. Conf., 2011, pp. 322–326 Search PubMed.
- V. Beachley and X. Wen, Polymer nanofibrous structures: fabrication, biofunctionalization, and cell interactions, Prog. Polym. Sci., 2010, 35, 868–892, DOI:10.1016/j.progpolymsci.2010.03.003.
- W. C. Wang, Y. T. Cheng and B. Estroff, Electrostatic self-assembly of composite nanofiber yarn, Polymers, 2021, 23, 1–9, DOI:10.3390/polym13010012.
- X. Zhang and Y. Lu, Centrifugal spinning: an alternative approach to fabricate nanofibers at high speed and low cost, Polym. Rev., 2014, 54, 677–701, DOI:10.1080/15583724.2014.935858.
- T. Lu, Y. Li and T. Chen, Techniques for fabrication and construction of three-dimensional scaffolds for tissue engineering, Int. J. Nanomed., 2013, 8, 337–350, DOI:10.2147/IJN.S38635.
- E. Stojanovska, E. Canbay, E. S. Pampal, M. D. Calisir, O. Agma, Y. Polat, R. Simsek, N. A. S. Gundogdu, Y. Akgul and A. Kilic, A review on non-electro nanofibre spinning techniques, RSC Adv., 2016, 6, 83783–83801, 10.1039/c6ra16986d.
- J. Lin, L. Xu, Y. Huang, J. Li, W. Wang, C. Feng, Z. Liu, X. Xu, J. Zou and C. Tang, Ultrafine porous boron nitride nanofibers synthesized via a freeze-drying and pyrolysis process and their adsorption properties, RSC Adv., 2016, 6, 1253–1259, 10.1039/c5ra23426c.
- G. Che, B. B. Lakshmi, C. R. Martin, E. R. Fisher and R. S. Ruoff, Chemical Vapor Deposition Based Synthesis of Carbon Nanotubes and Nanofibers Using a Template Method, Chem. Mater., 1998, 10, 260–267, DOI:10.1021/cm970412f.
- N. Keller, C. Pham-Huu, T. Shiga, C. Estournès, J. M. Grenèche and M. J. Ledoux, Mild synthesis of CoFe2O4 nanowires using carbon nanotube template: a high-coercivity material at room temperature, J. Magn. Magn. Mater., 2004, 272–276, 1642–1644, DOI:10.1016/j.jmmm.2003.12.228.
- L. Liu, H. Li, S. Fan, J. Gu, Y. Li and H. Sun, Fabrication and magnetic properties of Ni-Zn nanowire arrays, J. Magn. Magn. Mater., 2009, 321, 3511–3514, DOI:10.1016/j.jmmm.2009.06.062.
- X. Zheng, T. Bian, Y. Zhang, Y. Zhang and Z. Li, Construction of ion-imprinted nanofiber chitosan films using low-temperature thermal phase separation for selective and efficiency adsorption of Gd(III), Cellulose, 2020, 27, 455–467, DOI:10.1007/s10570-019-02804-3.
- K. Whang, C. H. Thomas, K. E. Healy and G. Nuber, A novel method to fabricate bioabsorbable scaffolds, Polymer, 1995, 36, 837–842, DOI:10.1016/0032-3861(95)93115-3.
- Q. Tarrés, A. Deltell, F. X. Espinach, M. À. Pèlach, M. Delgado-Aguilar and P. Mutjé, Magnetic bionanocomposites from cellulose nanofibers: fast, simple and effective production method, Int. J. Biol. Macromol., 2017, 99, 29–36, DOI:10.1016/j.ijbiomac.2017.02.072.
- A. S. Nain, J. A. Phillippi, M. Sitti, J. MacKrell, P. G. Campbell and C. Amon, Control of cell behavior by aligned micro/nanofibrous biomaterial scaffolds fabricated by spinneret-based tunable engineered parameters (STEP) technique, Small, 2008, 4, 1153–1159, DOI:10.1002/smll.200800101.
- P. Lu and B. Ding, Applications of Electrospun Fibers, Recent Pat. Nanotechnol., 2008, 2, 169–182, DOI:10.2174/187221008786369688.
- V. Leung and F. Ko, Biomedical applications of nanofibers, Polym. Adv. Technol., 2011, 22, 350–365, DOI:10.1002/pat.1813.
- J. Fang, H. T. Niu, T. Lin and X. G. Wang, Applications of electrospun nanofibers, Chin. Sci. Bull., 2008, 53, 2265–2286, DOI:10.1007/s11434-008-0319-0.
- Y. J. Son, W. J. Kim and H. S. Yoo, Therapeutic applications of electrospun nanofibers for drug delivery systems, Arch. Pharmacal Res., 2014, 37, 69–78, DOI:10.1007/S12272-013-0284-2.
- S. Nemati, S. jeong Kim, Y. M. Shin and H. Shin, Current progress in application of polymeric nanofibers to tissue engineering, Nano Convergence, 2019, 6(1), 1–6, DOI:10.1186/s40580-019-0209-y.
- R. L. Dahlin, F. K. Kasper and A. G. Mikos, Polymeric nanofibers in tissue engineering, Tissue Eng., Part B, 2011, 17, 349–364, DOI:10.1089/TEN.TEB.2011.0238.
- H. Li, M. Wang, G. R. Williams, J. Wu, X. Sun, Y. Lv and L. M. Zhu, Electrospun gelatin nanofibers loaded with vitamins A and E as antibacterial wound dressing materials, RSC Adv., 2016, 6, 50267–50277, 10.1039/C6RA05092A.
- F. Croisier, G. Atanasova, Y. Poumay and C. Jérôme, Polysaccharide-Coated PCL Nanofibers for Wound Dressing Applications, Adv. Healthcare Mater., 2014, 3, 2032–2039, DOI:10.1002/ADHM.201400380.
- M. H. A. Zanin, N. N. P. Cerize and A. M. de Oliveira, Production of Nanofibers by Electrospinning Technology: Overview and Application in Cosmetics, Nanocosmetics and Nanomedicines, 2011, pp. 311–332, DOI:10.1007/978-3-642-19792-5_16.
- F. Yilmaz, G. Celep and G. Tetik, Nanofibers in cosmetics, Nanofiber Res.: Reaching New Heights, 2016, 127–145, DOI:10.5772/61952.
- J. H. Cha, D. H. Kim, S. J. Choi, W. T. Koo and I. D. Kim, Sub-Parts-per-Million Hydrogen Sulfide Colorimetric Sensor: Lead Acetate Anchored Nanofibers toward Halitosis Diagnosis, Anal. Chem., 2018, 90, 8769–8775, DOI:10.1021/ACS.ANALCHEM.8B01273.
- W. Rongthong, N. Niamnont, C. Srisuwannaket, N. Paradee and W. Mingvanish, Electrospun Gelatin Fiber Mats Mixed With C. carandas Extract and its Enhanced Stability and Bioactivity, J. Pharm. Sci., 2021, 110, 2405–2415, DOI:10.1016/J.XPHS.2020.12.031.
- C. C. Lin, D. H. Jiang, C. C. Kuo, C. J. Cho, Y. H. Tsai, T. Satoh and C. Su, Water-Resistant Efficient Stretchable Perovskite-Embedded Fiber Membranes for Light-Emitting Diodes, ACS Appl. Mater. Interfaces, 2018, 10, 2210–2215, DOI:10.1021/ACSAMI.7B15989.
- X. Shi, W. Zhou, D. Ma, Q. Ma, D. Bridges, Y. Ma and A. Hu, Electrospinning of Nanofibers and Their Applications for Energy Devices, J. Nanomater., 2015, 16(1), 122, DOI:10.1155/2015/140716.
- M. Venkatesan, W. C. Chen, C. J. Cho, L. Veeramuthu, L. G. Chen, K. Y. Li, M. L. Tsai, Y. C. Lai, W. Y. Lee, W. C. Chen and C. C. Kuo, Enhanced piezoelectric and photocatalytic performance of flexible energy harvester based on CsZn0.75Pb0.25I3/CNC–PVDF composite nanofibers, Chem. Eng. J., 2022, 433, 133620, DOI:10.1016/J.CEJ.2021.133620.
- Y. Bagbi, A. Pandey and P. R. Solanki, Electrospun Nanofibrous Filtration Membranes for Heavy Metals and Dye Removal, Elsevier Inc., 2018, DOI:10.1016/B978-0-12-813926-4.00015-X.
- S. Zhang, A. Tanioka and H. Matsumoto, De novo ion-exchange membranes based on nanofibers, Membranes, 2021, 11, 1–28, DOI:10.3390/membranes11090652.
- W. C. Chen, J. H. Shiao, T. L. Tsai, D. H. Jiang, L. C. Chen, C. H. Chang, B. H. Lin, J. H. Lin and C. C. Kuo, Multiple Scattering from Electrospun Nanofibers with Embedded Silver Nanoparticles of Tunable Shape for Random Lasers and White-Light-Emitting Diodes, ACS Appl. Mater. Interfaces, 2020, 12, 2783–2792, DOI:10.1021/ACSAMI.9B16059.
- J. Wei, S. Geng, O. Pitkänen, T. Järvinen, K. Kordas and K. Oksman, Green Carbon Nanofiber Networks for Advanced Energy Storage, ACS Appl. Energy Mater., 2020, 3, 3530–3540, DOI:10.1021/acsaem.0c00065.
- V. Aravindan, J. Sundaramurthy, P. Suresh Kumar, Y. S. Lee, S. Ramakrishna and S. Madhavi, Electrospun nanofibers: a prospective electro-active material for constructing high performance Li-ion batteries, Chem. Commun., 2015, 51, 2225–2234, 10.1039/C4CC07824A.
- M. Gorji, R. Bagherzadeh and H. Fashandi, Electrospun nanofibers in protective clothing, Elsevier Ltd., 2017, DOI:10.1016/B978-0-08-100907-9.00021-0.
- P. Ramesh Kumar, N. Khan, S. Vivekanandhan, N. Satyanarayana, A. K. Mohanty and M. Misra, Nanofibers: effective generation by electrospinning and their applications, J. Nanosci. Nanotechnol., 2012, 12, 1–25, DOI:10.1166/JNN.2012.5111.
- P. Barone, M. Barberio, V. Pingitore and A. Bonanno, Transport Properties of Alkali-Doped Multi Walled Carbon Nanotubes, J. Nanosci. Nanotechnol., 2012, 12(12), 9295–9298, DOI:10.1166/jnn.2012.6781.
- F. Fadil, N. D. N. Affandi, M. I. Misnon, N. N. Bonnia, A. M. Harun and M. K. Alam, Polymers, 2021, 13(13), 2087, DOI:10.3390/POLYM13132087.
- S. Ganguly, N. Kanovsky, P. Das, A. Gedanken and S. Margel, Photopolymerized Thin Coating of Polypyrrole/Graphene Nanofiber/Iron Oxide onto Nonpolar Plastic for Flexible Electromagnetic Radiation Shielding, Strain Sensing, and Non-Contact Heating Applications, Adv. Mater. Interfaces, 2021, 8(23), 2101255, DOI:10.1002/ADMI.202101255.
- A. Mahapatra, B. G. Mishra and G. Hota, Studies on electrospun alumina nanofibers for the removal of chromium(vi) and fluoride toxic ions from an aqueous system, Ind. Eng. Chem. Res., 2013, 52, 1554–1561, DOI:10.1021/ie301586j.
- M. Venkatesan, L. Veeramuthu, F. C. Liang, W. C. Chen, C. J. Cho, C. W. Chen, J. Y. Chen, Y. Yan, S. H. Chang and C. C. Kuo, Evolution of electrospun nanofibers fluorescent and colorimetric sensors for environmental toxicants, pH, temperature, and cancer cells – a review with insights on applications, Chem. Eng. J., 2020, 397, 125431, DOI:10.1016/J.CEJ.2020.125431.
- A. Aldalbahi, M. E. El-Naggar, M. H. El-Newehy, M. Rahaman, M. R. Hatshan and T. A. Khattab, Effects of technical textiles and synthetic nanofibers on environmental pollution, Polymers, 2021, 13, 1–26, DOI:10.3390/POLYM13010155.
- J. Cao, Z. Cheng, L. Kang, M. Lin and L. Han, Patterned nanofiber air filters with high optical transparency, robust mechanical strength, and effective PM2.5 capture capability, RSC Adv., 2020, 10, 20155–20161, 10.1039/D0RA01967D.
- X. Qin and S. Subianto, Electrospun nanofibers for filtration applications, Electrospun Nanofibers, 2017, pp. 449–466, DOI:10.1016/B978-0-08-100907-9.00017-9.
- M. Toriello, M. Afsari, H. K. Shon and L. D. Tijing, Progress on the fabrication and application of electrospun nanofiber composites, Membranes, 2020, 10, 1–35, DOI:10.3390/membranes10090204.
- L. Veeramuthu, M. Venkatesan, F. C. Liang, J. S. Benas, C. J. Cho, C. W. Chen, Y. Zhou, R. H. Lee and C. C. Kuo, Conjugated Copolymers through Electrospinning Synthetic Strategies and Their Versatile Applications in Sensing Environmental Toxicants, pH, Temperature, and Humidity, Polymers, 2020, 12, 587, DOI:10.3390/POLYM12030587.
- M. Liu, X. P. Duan, Y. M. Li, D. P. Yang and Y. Z. Long, Electrospun nanofibers for wound healing, Mater. Sci. Eng., C, 2017, 76, 1413–1423, DOI:10.1016/J.MSEC.2017.03.034.
- F. Fadil, N. D. N. Affandi, M. I. Misnon, N. N. Bonnia, A. M. Harun and M. K. Alam, Review on Electrospun Nanofiber-Applied Products, Polymers, 2021, 13, 2087, DOI:10.3390/POLYM13132087.
- A Literature Investigation about Electrospinning and Nanofibers, Ingenta Connect Search PubMed.
- G. R. Williams, N. P. Chatterton, T. Nazir, D. G. Yu, L. M. Zhu and C. J. Branford-White, Electrospun nanofibers in drug delivery: recent developments and perspectives, Ther. Delivery, 2012, 3, 515–533 CrossRef CAS PubMed.
- A. Formhals, Process and Apparatus for Preparing Artificial Threads, US Pat., 1975504, 1934 Search PubMed.
|
This journal is © The Royal Society of Chemistry 2022 |
Click here to see how this site uses Cookies. View our privacy policy here.