DOI:
10.1039/D2RA03774B
(Paper)
RSC Adv., 2022,
12, 23963-23972
Green synthesis of coumarin derivatives using Brønsted acidic pyridinium based ionic liquid [MBSPy][HSO4] to control an opportunistic human and a devastating plant pathogenic fungus Macrophomina phaseolina†
Received
19th June 2022
, Accepted 5th August 2022
First published on 24th August 2022
Abstract
An eco-friendly simple protocol has been devised for the preparation of coumarin derivatives using doubly Brønsted acidic task specific ionic liquid (TSIL) as a catalyst. Solvent-free conditions were employed for the reaction of different substituted phenols with β-ketoester in TSIL to produce corresponding substituted coumarin derivatives in good to excellent yields at ambient conditions; at room temperature and with reduced reaction times. The ionic liquid catalyst can be recycled and reused up to five times. All the synthesized coumarins were evaluated for their antifungal activities against Macrophomina phaseolina, a plant as well as an opportunistic human pathogenic fungus affecting more than 500 plant species worldwide and with no registered commercial fungicide available against it, to date. Amongst all the coumarins tested, compounds 3f and 3i showed excellent antifungal activity comparable to reference fungicide mancozeb. The current methodology provides an easy and expedient way to access the coumarin core in search of potential fungicides for sustainable agriculture.
1. Introduction
Coumarins (2H-chromone-2-one) are considered a significant class of heterocyclic compounds due to their versatile biological and medicinal properties such as antihelmintic, antioxidant,1 anticonvulsant,2 antitumor and anti-inflammatory activities.3 Broad range antimicrobial properties are also attributed to this core due to its distinct structural properties. Coumarins have important applications in cosmetics, fragrances, pharmaceuticals and food additives.4 It is recognized to introduce resistance in plant tissues against microbial attack, which is evident by the presence of coumarin derivatives in commercially available pesticides.5 The structures of some of the commercial coumarin based pesticides are depicted in Fig. 1, and include brodifacoum, dicoumarol, warfarin and difenacoum.6
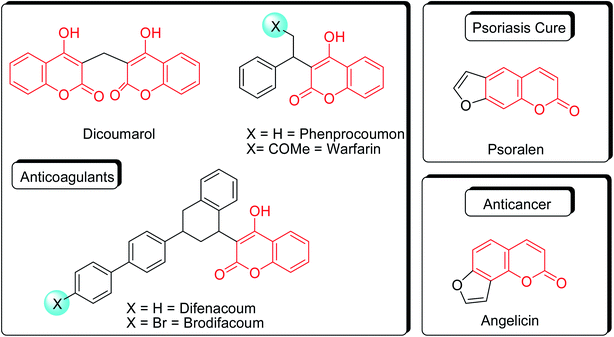 |
| Fig. 1 Some commercially available coumarin drugs. | |
One of the unique structural features of coumarin is the ease with which it could be assembled and modified using cheap and commercially available starting materials. During the past decades several methods used for the synthesis of coumarins include Knoevenagel,9 Perkin,8 Pechmann,7 Wittig,12 Claisen11 and Reformatsky reactions.10 Pechmann condensation is a widely used method in which phenols are reacted with β-ketoester to give 4-substituted coumarins in the presence of acid catalyst. Numerous Brønsted13–15 and Lewis acidic16–19 catalysts have been used like H2SO4, P2O5, H3PO4, Bi(NO3)3, Cu(ClO4)2 and others. The notability of the classical methods is significantly reduced due to the stoichiometric use of Brønsted acid and subsequent corrosion issues, water sensitivity of Lewis acids, high temperature requirements, poor selectivity and low yields.
Recently, ionic liquid (IL) became fascinating solvents and catalysis in organic synthesis owing to their unique properties like low volatility, non-inflammability, insignificant vapor pressure, high thermal stability and reusability.20 Pechmann condensation was primarily reported using chloroaluminates of imidazolium and pyridinium based ILs, drawbacks of which are their moisture and air sensitivity.21 Boroujeni et al. reported ionic liquid based catalyst [P4VPy-BuSO3H]Cl-X(AlCl3) possessing both Lewis and Brønsted acidic groups.22 1-Ethyl-3-(3-sulfopropyl)-benzimidazolium trifluoromethanesulfonate ([PSebim][OTf]) was also reported for the synthesis of biscoumarins.23 In comparison, Brønsted acidic ionic liquids (BAILs) are superior due to their comparable acidity to inorganic acid, recyclability, thermal and moisture stability.24 Amongst BAILs, the sulfonic acid functionalized cations and hydrogen sulfate bearing anions have recently been found very efficient catalysts in acid catalyzed organic transformations. 2-Pyrrolidonium hydrogen sulfate ([Hnmp][HSO4]), N-methyl-2-pyrrolidonium hydrogen sulfate ([NMP][HSO4]), triphenyl(propyl-3-sulfonyl)phosphonium toluene sulfonate, (4-sulfobutyl)tris(4-sulfophenyl)phosphonium hydrogen sulfate and N-methyl-2-pyrrolidonium dihydrogen phosphate ([NMP][H2PO4]) were reported to catalyze the fabrication of derivatives of coumarin under solvent-free environment.25 Similarly, Tiwari et al. reported triethylammonium hydrogen sulfate ([TEAN][HSO4])26 and Rezayati et al. reported 1,1′-butylenebispyridinium hydrogen sulfate (Bbpy)(HSO4)2 (ref. 27) for the synthesis of novel fungicidal coumarin derivatives. Research group of Mahato used 1-butane sulfonic acid-3-methylimidazolium tosylate ([BSMIM][OTs]) under solvent-free conditions for the regioselective production of pyrano[3,2-c] coumarins.28 Same BAIL catalyst was also reported for the synthesis of pyrano[3,2-c]coumarins via tandem reaction between α,β-unsaturated carbonyl compounds and 4-hydroxycoumarin.29 The previous reports of Pechmann condensation catalyzed by BAIL have acidic proton either on the cation or anion, therefore, requiring high catalyst loadings, elevated temperature and longer reaction times.25–29 To the best of our knowledge, Pechmann condensation has not been tried so far with BAILs having acidic proton both on anion and cation of IL. Therefore a green synthetic protocol has been devised using task specific BAIL for the quick assembly of coumarin core and their subsequent antifungal potential was evaluated against Macrophomina phaseolina, an opportunistic human and a highly problematic plant pathogenic fungus affecting more than 500 plant species worldwide and with no registered commercial fungicide available against it to date.
2. Experimental
All the chemicals were purchased from Sigma Aldrich and Alfa-Aeser and used without any further treatment. Reported yields refer to pure crystallized products. [MBSPy][HSO4] was synthesized following the previously reported procedure.30 Pure coumarins were characterized by Fourier Transform Infrared (FTIR) analysis and proton nuclear magnetic resonance (1H NMR) spectroscopy. 1H NMR (300–400 MHz) spectra were run in D2O and DMSO-d6 relative to TMS (0.00 ppm). FTIR spectra were recorded on Agilent Cary 630 FTIR having scanning range of 400–4000 cm−1. Melting points of pure crystallized products were taken on a Gallenkamp melting apparatus and were uncorrected.
2.1. Synthesis of 1-butylsulfonic-3-methylpyridinium hydrogen sulfate [MBSPy][HSO4]
A round bottom flask was charged with 3-methylpyridine (10 mmol) and 1,4-butanesultone (10 mmol) were added and refluxed at 80 °C with constant stirring until a white solid is formed. After that, sulfuric acid (10 mmol) is added to this white solid and refluxed at 80 °C. White solid began to react with sulfuric acid as apparent by formation of pale yellowish viscous liquid. Appropriate time was given to the reaction mixture until the completion of reaction.
Pale yellow viscous liquid, Yield (85%), 1H NMR (300 MHz, D2O) δ (ppm): 8.595 (s, 1H, HAr), 8.536 (d, 1H, J = 6.3 Hz, HAr), 8.236 (d, 1H, J = 7.8 Hz, HAr), 7.8 (t, 1H, J = 6.5 Hz, HAr), 4.466 (t, 2H, J = 7.5 Hz, CH2), 2.814 (t, 2H, J = 7.5 Hz, CH2), 2.425 (s, 3H, CH3), 1.994 (m, 2H, CH2), 1.621 (m, 2H, J = 7.6 Hz, CH2).
2.2. General procedure for the synthesis of coumarins (3a–i)
A round bottom flask was charged with phenol (1a–g) (1 mmol), β-ketoester (2) (1.2 mmol) and [MBSPy][HSO4] (0.01 mmol) and stirred at room temperature. Reaction progress was monitored via thin layer chromatography using 1
:
1 ethyl acetate and n-hexane. After the appearance of single spotted product on TLC, the reaction mixture was allowed to cool, and work-up was done by addition of distilled water and stirring for 5 to 10 min. The produced crystalline product was filtered, and recrystallized using ethanol (Scheme 1).
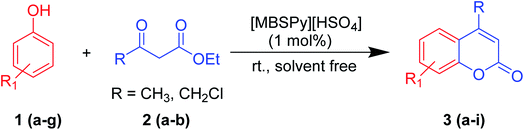 |
| Scheme 1 Synthesis of coumarin derivatives from different substituted phenols using [MBSPy][HSO4] as a catalyst. | |
2.2.1 7-Hydroxy-4-methyl-2H-chromen-2-one (3a). Colorless solid, yield (92%), MP (182–184 °C), FTIR (cm−1): 1395, 1595, 1677, 3129, 1H NMR (300 MHz, DMSO-d6) δ (ppm): 10.52 (s, 1H, OH), 7.55 (d, 1H, J = 8.7 Hz, HAr), 6.772–6.809 (dd, 1H, J = 2.4, 8.7 Hz, HAr), 6.69 (d, 1H, J = 2.4 Hz, HAr), 6.11 (s, 1H, CH), 2.34 (s, 3H, CH3).
2.2.2 7-Hydroxy-4,5-dimethyl-2H-chromen-2-one (3b). Colorless solid, yield (80%), MP (189–190 °C), FTIR (cm−1): 1362, 1513, 1619, 3325, 1H NMR (300 MHz, DMSO-d6) δ (ppm): 10.55 (s, 1H, OH), 6.56 (d, 2H, J = 10.5 Hz, HAr), 6.02 (s, 1H, CH), 3.44 (s, 3H, CH3), 2.26 (s, 3H, CH3).
2.2.3 5,7-Dihydroxy-4-methyl-2H-chromen-2-one (3c). White solid, yield (83%), MP (280–282 °C), FTIR (cm−1): 1365, 1558, 1670, 3129, 3412, 1H NMR (300 MHz, DMSO-d6) δ (ppm): 10.53 (s, 1H, OH), 10.30 (s, 1H, OH), 6.25 (d, 1H, J = 2.4 Hz, HAr), 6.16 (d, 1H, J = 2.4 Hz, HAr), 5.84 (s, 1H, CH), 2.48 (s, 3H, CH3).
2.2.4 7,8-Dihydroxy-4-methyl-2H-chromen-2-one (3d). White solid, yield (84%), MP (243–244 °C), FTIR (cm−1): 1313, 1588, 1655, 3218, 3404, 1H NMR (300 MHz, DMSO-d6) δ (ppm): 10.0 (s, 1H, OH), 9.34 (s, 1H, OH), 7.05 (d, 1H, J = 8.7 Hz, HAr), 6.79 (d, 1H, J = 8.7 Hz, HAr), 6.10 (s, 1H, CH), 2.33 (s, 3H, CH3).
2.2.5 4-(Chloromethyl)-2H-benzo[h]chromen-2-one (3e). Light yellow solid, yield (65%), MP (220–222 °C), FTIR (cm−1): 1398, 1542, 1604, 3438, 1H NMR (300 MHz, DMSO-d6) δ (ppm): 8.517 (m, 1H, HAr), 7.845 (m, 1H, HAr), 7.70 (m, 1H, HAr), 7.60 (m, 3H, HAr), 6.62 (s, 1H, CH), 4.72 (s, 2H, CH2).
2.2.6 4-(Chloromethyl)-7-hydroxy-5-methyl-2H-chromen-2-one (3f). Brown solid, yield (74%), MP (153–154 °C), FTIR (cm−1): 1372, 1581, 1685, 3054, 1H NMR (400 MHz, DMSO-d6) δ (ppm): 9.0 (s, 1H, OH), 6.64 (d, 2H, J = 14, HAr), 6.59 (s, 1H, HAr), 6.40 (s, 1H, CH), 5.07 (s, 2H, CH2), 2.28 (s, 3H, CH3).
2.2.7 4-(Chloromethyl)-5,7-dihydroxy-2H-chromen-2-one (3g). Brown solid, yield (75%), MP (160–165 °C), FTIR (cm−1): 1275, 1514, 1633, 3240, 1H NMR (400 MHz, DMSO-d6) δ (ppm): 10.87 (s, 1H, OH), 10.41 (s, 1H, OH), 6.27 (d, 1H, J = 2.4, HAr), 6.21 (d, 1H, J = 2.4, HAr), 5.02 (s, 2H, CH2), 3.36 (s, 1H, CH).
2.2.8 4-(Chloromethyl)-7-hydroxy-2H-chromen-2-one (3h). Brown solid, yield (92%), MP (171–174 °C), FTIR (cm−1): 1310, 1513, 1635, 3233, 1H NMR (400 MHz, DMSO-d6) δ (ppm): 10.63 (s, 1H, OH), 7.65 (d, 1H, J = 8.8 Hz, HAr), 6.81–6.84 (dd, 1H, J = 2.4 Hz, HAr), 6.74 (d, 1H, J = 2 Hz, HAr), 6.41 (s, 1H, CH), 4.94 (s, 2H, CH2).
2.2.9 4-(Chloromethyl)-7,8-dihydroxy-2H-chromen-2-one (3i). Brown solid, yield (87%), MP (177–179 °C), FTIR (cm−1): 1278, 1520, 1645, 3265, 1H NMR (400 MHz, DMSO-d6) δ (ppm): 10.16 (s, 1H, OH), 9.36 (s, 1H, OH), 7.15 (d, 1H, J = 7.2 Hz, HAr), 6.82 (d, 1H, J = 8.4 Hz, HAr), 6.39 (s, 1H, CH), 4.92 (s, 2H, CH2).
2.3. Antifungal bioassays
All the nine derivatives of coumarin (3a–i) were evaluated for their antifungal activity against M. phaseolina according to a reported procedure31 with little modifications. Six milligrams of a reference fungicide mancozeb (active ingredients) and each of the nine coumarin derivatives were dissolved individually in 30 μL dimethyl sulfoxide (DMSO). Medium (3 mL) was then prepared by addition of autoclaved malt extract broth. This medium of 2 mg mL−1 concentration was divided into two 1.5 mL portions. One set was used in antifungal bioassays while the other was used for further serial double dilutions ranging from 1–0.0156 mg mL−1. Bioassays were carried out with 0.5 mL growth medium taken in each 5 mL test tube in triplicate. Likewise, a set of control treatment was prepared to maintain the same amount of DMSO for comparison. M. phaseolina inoculum suspension (10 μL) was added to each test tube and incubated at 27 °C for 120 h. The fungal growth was observed with intervals of 24 h. After 120 h, fungal biomass from each test tube was collected on filter papers, dried at 60 °C and weighed.
2.4. Statistical analysis
Data regarding fungal biomass in different treatments were subjected to two-way ANOVA. The treatment means were separated by applying LSD Test at 5% level of significance using Statistix 8.1 software.
3. Results and discussion
3.1. Characterization of [MBSPy][HSO4]
3.1.1 Thermogravimetric analysis (TGA). Thermal studies were carried out for [MBSPy][HSO4] via thermogravimetric analysis (TGA) performed simultaneously with DSC. Percent weight loss by rise in temperature is recorded with high accuracy as provided in Fig. 2. The DSC was used to obtain the thermal critical points like melting point, glass transition temperature or enthalpy specific heat of substance.
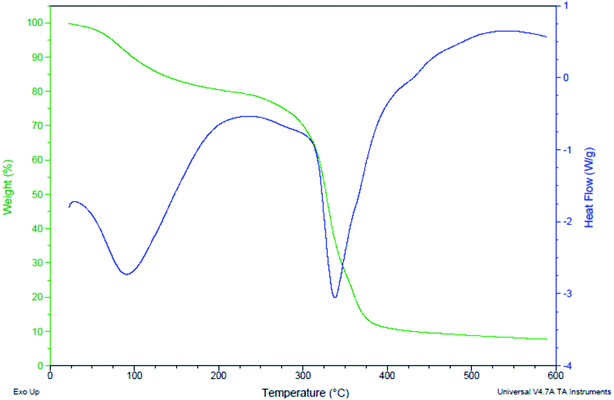 |
| Fig. 2 TGA and DSC analysis of the catalyst. | |
The TGA and DSC curves depicted the catalyst potency towards weight gain or loss as a function of temperature with time. The TGA curve dictated that the mass loss is not so significant up to 300 °C, approving the thermal stability of the catalyst. A sharp mass loss is observed after the 300 °C. The water content was calculated 8%.
DSC measured the catalyst energy change during the transformation as a function of temperature or time. We obtained very important quantities like the transition temperature of the material (melting point Tm, crystallization temperature Tc and glass transition temperature Tg). The water content was lost between the points 98.25 to 87.87. After that transition temperature was observed between the points 86.42 to 80.95 and the catalyst was decomposed at 300 °C (70.21 point). These data predicts the potential of synthesized catalyst with the temperature variations.
3.2. Optimization of synthesis of coumarin derivatives
Reaction of resorcinol (1a) and ethyl acetoacetate (2a) was carried out as a model reaction and the reaction conditions such as temperature, time and catalyst mol% were optimized for high yield of coumarin 3a (Table 1). At first, reaction was carried out using 1 eq. of 1a and 1.2 eq of 2a under solvent-free conditions. 1 mol% [MBSPy][HSO4] was used as catalyst at room temperature and 93% yield was obtained within one hour. Control reaction was also done without [MBSPy][HSO4] under same conditions, but no yield was obtained even after four hours (Table 1; entry 1). When temperature was elevated from room temperature to 80 °C, time of the reaction was shortened but the yield was a little lower when compared to reaction done at room temperature; 82% yield was obtained in half an hour. Thus, temperature was observed to exhibit a little effect on reaction kinetics. So, all the reactions were performed next at room temperature in solvent-free environment. An increase in percentage of catalyst was not significant as it caused subsequent decrease in percentage yield of product. Increasing the catalysts loading from 1 to 5 mol% decreased the yield to 68%. Low catalytic activity of BAIL at higher concentrations is probably due to its high acidic strength and possibility to protonate the phenolic –OH, thus lowering its nucleophilicity. Therefore, the optimum dose of catalyst for this transformation was only 1 mol% of [MBSPy][HSO4]. It demonstrated high catalytic proficiency of [MBSPy][HSO4] due to its dual acidic sites.
Table 1 Optimization of reaction conditions
Entry |
[MBSPy][HSO4] (mol%) |
Temperature (°C) |
Time (h) |
Yield of 3a (%) |
1 |
No catalyst |
Room temp. |
4 |
No reaction |
2 |
1 |
Room temp. |
1 |
93 |
3 |
1 |
80 |
0.5 |
82 |
4 |
2 |
80 |
0.5 |
81 |
5 |
2.5 |
80 |
0.5 |
75 |
6 |
5 |
80 |
0.5 |
68 |
After optimization of conditions of 3a, eight other coumarin derivatives were prepared using different substituted phenols (1a–g) and β-ketoesters (2a, 2b) under solvent-free, optimized conditions (Table 2). Yields of products and progress of reaction was affected by phenolic substitutions. Highest product selectivity was observed for reaction of resorcinol (1a) with ethylacetoacetate (2a) and ethyl chloroacetoacetate (2b). While high reaction kinetics was for ethyl chloroacetoacetate (2b) from both β-ketoesters due to its inductive effect and high reactivity, in turn. Electron donating groups such as methyl and hydroxyl ones, substituted on ortho- or para- to both hydroxyls of resorcinol, also indicated best reaction rates and yields (>80%). Reaction of 1-naphthol (1e) took relatively longer time due to the adjacent benzene ring present in it. Consequently, the catalyst is efficient for the synthesis of coumarin derivatives starting from different phenols substituted with electron-donating groups. A plausible mechanism of the reaction is provided in Fig. 3. Pechmann condensation between substituted phenol 1 and ethyl acetoacetate 2 is proposed to happen by BAIL catalysis. Condensation followed by intramolecular cyclization of resultant intermediate A, elimination of ethanol from cyclized product and subsequent dehydration led to the final targeted product 3.
Table 2 Synthesis of coumarin derivatives (3a–i) from phenols (1a–g) and ethyl acetoacetate (2a, 2b) using [MBSPy][HSO4] under solvent free conditions
Sr. no. |
Phenol |
β-Keto ester |
Product |
Time (min) |
Yield (%) |
1 |
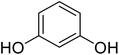 |
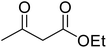 |
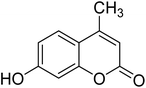 |
60 |
92 |
(1a) |
(2a) |
(3a) |
2 |
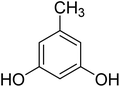 |
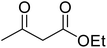 |
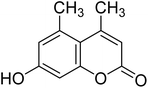 |
60 |
80 |
(1b) |
(2a) |
(3b) |
3 |
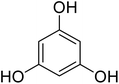 |
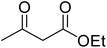 |
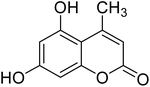 |
40 |
83 |
(1c) |
(2a) |
(3c) |
4 |
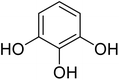 |
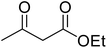 |
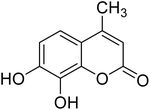 |
40 |
84 |
(1d) |
(2a) |
(3d) |
5 |
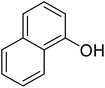 |
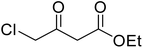 |
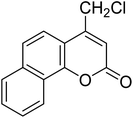 |
80 |
65 |
(1e) |
(2b) |
(3e) |
6 |
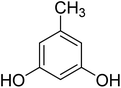 |
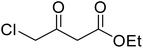 |
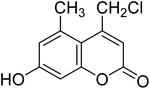 |
80 |
74 |
(1f) |
(2b) |
(3f) |
7 |
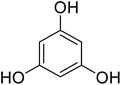 |
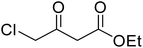 |
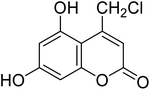 |
90 |
75 |
(1g) |
(2b) |
(3g) |
8 |
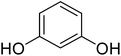 |
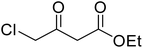 |
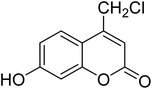 |
50 |
92 |
(1a) |
(2b) |
(3h) |
9 |
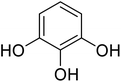 |
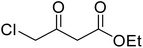 |
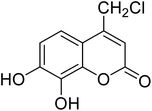 |
55 |
87 |
(1d) |
(2b) |
(3i) |
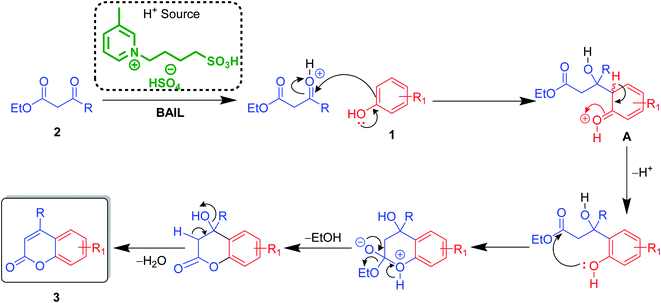 |
| Fig. 3 Proposed Mechanism of the reaction. | |
3.3. Catalyst recycling
After completion of reaction and collection of coumarin derivatives via filtration, BAIL catalyst was regenerated by water evaporation from the filtrate. It is washed with distilled ethyl acetate to eliminate the possibility of any impurities in it. The recovered catalyst was monitored for its activity up to five reaction cycles (Fig. 4). Recycling reactions show 100% selectivity of recovered catalyst for coumarins synthesis. For the first run carried out with freshly prepared ionic liquid catalyst, conversion rate is 92%, and it is reduced just to 85% for the fifth run (Table 3) thus exhibiting high recycling ability of the catalyst.
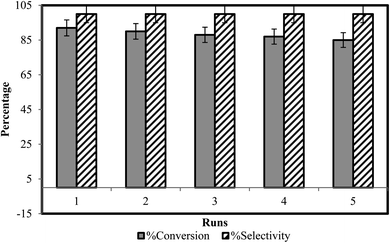 |
| Fig. 4 Recycling of [MBSPy][HSO4] catalyst. | |
Table 3 Analysis of variance (ANOVA) for the effect of different concentrations of coumarin derivatives on biomass of Macrophomina phaseolina
Sources of variation |
df |
SS |
MS |
F values |
Significant at P ≤ 0.001. |
Compounds (P) |
10 |
4979 |
497.9 |
3098a |
Concentration (C) |
6 |
406 |
67.7 |
420a |
P × C |
60 |
173 |
2.9 |
18a |
Error |
154 |
25 |
0.16 |
|
Total |
230 |
5584 |
|
|
3.4. Antifungal activity against Macrophomina phaseolina
ANOVA presented in Table 3 clearly indicated the significant effect of compounds (P), concentration (C) as well as P × C for fungal biomass production at P ≤ 0.001. Results regarding antifungal activity of different derivatives of coumarin are shown in Table 4. Different concentrations of DMSO in control also had significant effect on fungal growth. With an increase in DMSO concentration, a gradual decrease in biomass of the fungal pathogen was recorded. The reference fungicide mancozeb was found most effective in controlling the fungal growth. It's all concentrations completely inhibited the fungal growth throughout the experiment with MIC 0.0312 mg mL−1 after 120 h. None of the synthesized derivatives of coumarins completely controlled the fungal growth at the end of experiment (120 h). However, different derivatives delayed the fungal growth for variable time periods. Derivatives 6 and 9 proved more antifungal than rest of the synthesized compounds. All the concentrations of compound 3i controlled fungal growth up to 96 h and the fungal growth was recorded after 120 h with 85–91% decrease in fungal biomass over different corresponding control treatments. Likewise, compound 3f decreased fungal biomass by 90–92% over control. Other compounds were less effective than 3f and 3i. Compounds 3(a–e), 3g and 3h reduced fungal biomass by 66–81%, 64–74%, 64–92%, 60–86%, 69–96%, 55–82% and 56–86%, respectively.
Table 4 Minimum inhibitory concentrations of fungicide mancozeb and coumarine derivatives against Macrophomina phaseolinaa
Treatments |
Compound conc. (mg mL−1) |
DMSO conc. (μL mL−1) |
Time (h) |
Fungal biomass (mg) |
24 |
48 |
72 |
96 |
120 |
Values with different letters show significant difference as determined by LSD test at 5% level of significance. ± indicates standard errors of means of three replicates. − no fungal growth. + fungal growth appeared. |
Control |
0 |
10 |
+ |
+ |
+ |
+ |
+ |
16.8 ± 0.20 D |
0 |
5 |
+ |
+ |
+ |
+ |
+ |
17.7 ± 0.15 CD |
0 |
2.5 |
+ |
+ |
+ |
+ |
+ |
17.7 ± 0.25 CD |
0 |
1.25 |
+ |
+ |
+ |
+ |
+ |
18.6 ± 0.26 BC |
0 |
0.625 |
+ |
+ |
+ |
+ |
+ |
19.0 ± 0.26 A-C |
0 |
0.3125 |
+ |
+ |
+ |
+ |
+ |
19.5 ± 0.18 AB |
0 |
0.1562 |
+ |
+ |
+ |
+ |
+ |
20.5 ± 0.17 A |
Mancozeb |
2 |
10 |
− |
− |
− |
− |
− |
0 ± 0.0 c |
1 |
5 |
− |
− |
− |
− |
− |
0 ± 0.0 c |
0.5 |
2.5 |
− |
− |
− |
− |
− |
0 ± 0.0 c |
0.25 |
1.25 |
− |
− |
− |
− |
− |
0 ± 0.0 c |
0.125 |
0.625 |
− |
− |
− |
− |
− |
0 ± 0.0 c |
0.0625 |
0.3125 |
− |
− |
− |
− |
− |
0 ± 0.0 c |
0.0312 |
0.1562 |
− |
− |
− |
− |
− |
0 ± 0.0 c |
Compound 3a |
2 |
10 |
− |
− |
+ |
+ |
+ |
3.2 ± 0.23 S-W |
1 |
5 |
− |
− |
+ |
+ |
+ |
4.4 ± 0.15 Q-U |
0.5 |
2.5 |
− |
− |
+ |
+ |
+ |
4.5 ± 0.15 Q-T |
0.25 |
1.25 |
− |
− |
+ |
+ |
+ |
4.8 ± 0.20 M-R |
0.125 |
0.625 |
− |
− |
+ |
+ |
+ |
5.7 ± 0.17 K-Q |
0.0625 |
0.3125 |
− |
− |
+ |
+ |
+ |
6.3 ± 0.15 I-N |
0.0312 |
0.1562 |
− |
− |
+ |
+ |
+ |
6.9 ± 0.06 H-K |
Compound 3b |
2 |
10 |
− |
− |
+ |
+ |
+ |
4.4 ± 0.19 Q-T |
1 |
5 |
− |
− |
+ |
+ |
+ |
4.4 ± 0.20 Q-T |
0.5 |
2.5 |
− |
− |
+ |
+ |
+ |
5.3 ± 0.18 L-Q |
0.25 |
1.25 |
− |
− |
+ |
+ |
+ |
6.2 ± 0.09 I-P |
0.125 |
0.625 |
− |
− |
+ |
+ |
+ |
6.3 ± 0.17 I-N |
0.0625 |
0.3125 |
− |
− |
+ |
+ |
+ |
7.2 ± 0.09 G-K |
0.0312 |
0.1562 |
− |
− |
+ |
+ |
+ |
7.4 ± 0.12 F-J |
Compound 3c |
2 |
10 |
− |
− |
− |
− |
+ |
1.4 ± 0.12 YZa-c |
1 |
5 |
− |
− |
− |
− |
+ |
1.4 ± 0.18 YZa-c |
0.5 |
2.5 |
− |
− |
− |
− |
+ |
1.3 ± 0.06 Za-c |
0.25 |
1.25 |
− |
− |
+ |
+ |
+ |
3.6 ± 0.23 R-V |
0.125 |
0.625 |
− |
− |
+ |
+ |
+ |
4.3 ± 0.18 Q-U |
0.0625 |
0.3125 |
− |
− |
+ |
+ |
+ |
6.3 ± 0.09 I-O |
0.0312 |
0.1562 |
− |
− |
+ |
+ |
+ |
7.4 ± 0.12 G-J |
Compound 3d |
2 |
10 |
− |
− |
− |
− |
+ |
2.3 ± 0.15 V-ZAB |
1 |
5 |
− |
− |
− |
+ |
+ |
4.6 ± 0.13 P-S |
0.5 |
2.5 |
− |
− |
+ |
+ |
+ |
6.4 ± 0.12 I-M |
0.25 |
1.25 |
− |
− |
+ |
+ |
+ |
7.0 ± 0.12 H-K |
0.125 |
0.625 |
− |
− |
+ |
+ |
+ |
7.6 ± 0.44 E-I |
0.0625 |
0.3125 |
− |
− |
+ |
+ |
+ |
8.1 ± 0.20 E-H |
0.0312 |
0.1562 |
− |
− |
+ |
+ |
+ |
8.2 ± 0.19 E-H |
Compound 3e |
2 |
10 |
− |
− |
− |
− |
+ |
0.7 ± 0.12 bc |
1 |
5 |
− |
− |
− |
− |
+ |
0.8 ± 0.27 a-c |
0.5 |
2.5 |
− |
− |
− |
− |
+ |
1.5 ± 0.20 X-Za-c |
0.25 |
1.25 |
− |
− |
− |
− |
+ |
2.9 ± 0.20 T-Y |
0.125 |
0.625 |
− |
− |
+ |
+ |
+ |
4.6 ± 0.18 O-S |
0.0625 |
0.3125 |
− |
− |
+ |
+ |
+ |
5.0 ± 0.15 M-R |
0.0312 |
0.1562 |
− |
− |
+ |
+ |
+ |
6.4 ± 0.29 I-N |
Compound 3f |
2 |
10 |
− |
− |
− |
− |
+ |
1.4 ± 0.20 X-Za-c |
1 |
5 |
− |
− |
− |
− |
+ |
1.4 ± 0.12 X-Za-c |
0.5 |
2.5 |
− |
− |
− |
− |
+ |
1.4 ± 0.18 X-Za-c |
0.25 |
1.25 |
− |
− |
− |
− |
+ |
1.4 ± 0.09 X-Za-c |
0.125 |
0.625 |
− |
− |
− |
− |
+ |
1.7 ± 0.12 W-Zab |
0.0625 |
0.3125 |
− |
− |
+ |
+ |
+ |
1.8 ± 0.37 W-Zab |
0.0312 |
0.1562 |
− |
− |
+ |
+ |
+ |
2.5 ± 0.11 V-Z |
Compound 3g |
2 |
10 |
− |
− |
− |
− |
+ |
3.0 ± 0.34 T-X |
1 |
5 |
− |
− |
+ |
+ |
+ |
5.0 ± 0.37 M-R |
0.5 |
2.5 |
− |
− |
+ |
+ |
+ |
5.2 ± 0.18 L-Q |
0.25 |
1.25 |
− |
− |
+ |
+ |
+ |
5.8 ± 0.17 J-Q |
0.125 |
0.625 |
− |
− |
+ |
+ |
+ |
6.7 ± 0.27 H-L |
0.0625 |
0.3125 |
− |
− |
+ |
+ |
+ |
8.8 ± 0.09 E-G |
0.0312 |
0.1562 |
− |
− |
+ |
+ |
+ |
9.2 ± 0.12 E |
Compound 3h |
2 |
10 |
− |
− |
− |
− |
+ |
2.4 ± 0.23 V-Z |
1 |
5 |
− |
− |
− |
− |
+ |
2.5 ± 0.09 V-Z |
0.5 |
2.5 |
− |
− |
− |
− |
+ |
2.5 ± 0.18 V-Z |
0.25 |
1.25 |
− |
− |
− |
+ |
+ |
4.2 ± 0.40 Q-U |
0.125 |
0.625 |
− |
− |
− |
+ |
+ |
4.8 ± 0.22 N-S |
0.0625 |
0.3125 |
− |
− |
+ |
+ |
+ |
6.8 ± 0.12 H-L |
0.0312 |
0.1562 |
− |
− |
+ |
+ |
+ |
9.0 ± 0.12 EF |
Compound 3i |
2 |
10 |
− |
− |
− |
− |
+ |
1.5 ± 0.18 X-Za-c |
1 |
5 |
− |
− |
− |
− |
+ |
1.6 ± 0.21 W-Za-c |
0.5 |
2.5 |
− |
− |
− |
− |
+ |
1.3 ± 0.12 YZa-c |
0.25 |
1.25 |
− |
− |
− |
− |
+ |
1.8 ± 0.12 W-Za-c |
0.125 |
0.625 |
− |
− |
− |
− |
+ |
1.9 ± 0.26 W-Za-c |
0.0625 |
0.3125 |
− |
− |
− |
− |
+ |
2.8 ± 0.19 U-Z |
0.0312 |
0.1562 |
− |
− |
− |
− |
+ |
3.0 ± 0.25 T-X |
Critical value for comparison (P ≤ 0.001) |
1.6 |
4. Conclusion
Doubly Brønsted acidic task specific ionic liquid (TSIL) is utilized as effective catalyst for the production of potential fungicidal coumarin derivatives for sustainable agriculture. Nine different compounds were prepared using substituted phenol and ethyl acetoacetate or its chloro-derivative under ambient, solvent-free conditions in significantly high yields. The synthesis is highly green and benign due to recoverable and recyclable TSIL catalyst, ambient conditions like room temperature and short reaction times. 93% Yield was obtained at room temperature in just one hour with only 1 mol% catalyst. From all the tested compounds, compound 3f and 3i showed excellent antifungal activity comparable to reference fungicide mancozeb.
Conflicts of interest
There are no conflicts to declare.
Acknowledgements
This work was financially supported by Higher Education Commission (HEC) Pakistan, under the NRPU Research Grant, project no: 8639/Punjab/NRPU/R&D/HEC/2017 and TDF Research Grant, project no. TDF03-294. School of Chemistry, University of the Punjab is acknowledged for its support towards this project.
References
- S. Stanchev, V. Hadjimitova, T. Traykov, T. Boyanov and I. Manolov, Investigation of the antioxidant properties of some new 4-hydroxycoumarin derivatives, Eur. J. Med. Chem., 2009, 44, 3077–3082 CrossRef CAS PubMed
. - K. V. Sashidhara, A. Kumar, M. Kumar, A. Srivastava and A. Puri, Synthesis and antihyperlipidemic activity of novel coumarin bisindole derivatives, Bioorg. Med. Chem. Lett., 2010, 20, 6504–6507 CrossRef CAS
. - R. S. Keri, K. M. Hosamani, R. V. Shingalapur and M. H. Hugar, Analgesic, anti-pyretic and DNA cleavage studies of novel pyrimidine derivatives of coumarin moiety, Eur. J. Med. Chem., 2010, 45, 2597–2605 CrossRef CAS PubMed
. - A. J. Cohen, Critical review of the toxicology of coumarin with special reference to interspecies differences in metabolism and hepatotoxic response and their significance to man, Food Cosmet. Toxicol., 1979, 17, 277–289 CrossRef CAS
. - A. Basile, S. Sorbo, V. Spadaro, M. Bruno, A. Maggio, N. Faraone and S. Rosselli, Antimicrobial and antioxidant activities of coumarins from the roots of Ferulago campestris (Apiaceae), Molecules, 2009, 14, 939–952 CrossRef CAS PubMed
. - N. O. Mahmoodi, F. G. Pirbasti and Z. Jalalifard, Recent advances in the synthesis of biscoumarin derivatives, J. Chin. Chem. Soc., 2018, 65, 383–394 CrossRef CAS
. - F. Bigi, L. C. Hesini, R. Maggi and G. Sartori, Montmorillonite KSF as an inorganic, water stable, and reusable catalyst for the knoevenagel synthesis of coumarin-3-carboxylic acids, J. Org. Chem., 1999, 64, 1033–1035 CrossRef CAS PubMed
. - B. J. Donnelly, D. M. X. Donnelly and A. M. O. Sullivan, Dalbergia Species-VI: The occurrence of melannein in the genus Dalbergia, Tetrahedron, 1968, 24, 2617–2622 CrossRef CAS
. - V. H. Pechmann, Neue Bildungsweise der Cumarine. Synthese des Daphnetins. I, Ber. Dtsch. Chem. Ges., 1884, 17, 929–936 CrossRef
. - I. Yavari, R. H. Shoar and A. Zonuzi, A new and efficient route to 4-carboxymethylcoumarins mediated by vinyltriphenylphosphonium salt, Tetrahedron Lett., 1998, 39, 2391–2392 CrossRef CAS
. - N. Cairns, L. M. Harwood and D. P. Astles, Tandem thermal Claisen-Cope rearrangements of coumarate derivatives. Total synthesis of the naturally occurring coumarins: suberosin, demethylsuberosin, ostruthin, balsamiferone and gravelliferone, J. Chem. Soc., Perkin Trans. 1, 1994, 1, 3101–3107 RSC
. - R. L. Shirner, The Reformatsky reaction, Org. React., 1942, 1, 1–37 Search PubMed
. - Z. Cao, G.-X. Zhou, C. Ma, K. Jiang and G.-J. Mei, Brønsted acid catalyzed domino 1,6-addition/intramolecular cyclization reactions: diastereoselective synthesis of dihydrocoumarin frameworks, Synthesis, 2018, 50, 1307–1314 CrossRef CAS
. - B. S. Kuarm, P. A. Crooks and B. Rajitha, Polyvinylsulfonic acid: An efficient and recyclable Brønsted acid catalyst for Pechmann condensation, Int. J. Res. Pharm. Biomed. Sci., 2012, 3, 50–53 Search PubMed
. - N. D. Kokare, J. N. Sangshetti and D. B. Shinde, Oxalic acid catalyzed solvent-free one pot synthesis of coumarins, Chin. Chem. Lett., 2007, 18, 1309–1312 CrossRef CAS
. - D. S. Bose, A. P. Rudradas and M. H. Babu, The indium(III) chloride-catalyzed von Pechmann reaction, Tetrahedron Lett., 2002, 43, 9195–9197 CrossRef
. - G. Smitha and C. S. Reddy, ZrCl4-catalyzed Pechmann reaction, Synth. Commun., 2004, 34, 3997–4003 CrossRef CAS
. - S. K. De and R. A. Gibbs, An efficient and practical procedure for the synthesis of 4-substituted coumarins, Synthesis, 2005, 8, 1231–1233 CrossRef
. - V. M. Alexander, R. P. Bhat and S. D. Samant, Bismuth(III) nitrate pentahydrate – a mild and inexpensive reagent for synthesis of coumarins under mild conditions, Tetrahedron Lett., 2005, 46, 6957–6959 CrossRef CAS
. - A. M. Asim, M. Uroos, S. Naz, M. Sultan, G. Griffin, N. Muhammad and A. S. Khan, Acidic ionic liquids: Promising and cost-effective solvents for processing of lignocellulosic biomass, J. Mol. Liq., 2019, 287, 110943 CrossRef CAS
. - M. K. Potdar, S. S. Mohile and M. M. Salunkhe, Coumarin syntheses via Pechmann condensation in Lewis acidic chloroaluminate ionic liquid, Tetrahedron Lett., 2001, 42, 9285–9287 CrossRef CAS
. - K. P. Boroujeni and P. Ghasemi, Synthesis and application of a novel strong and stable supported ionic liquid catalyst with both Lewis and Brønsted acid sites, Catal. Commun., 2013, 37, 50–54 CrossRef
. - W. Li, Y. Wang, Z. Wang, L. Dai and Y. Wang, Novel So3H functionalized ionic liquid based on benzimidazolium cation: efficient and recyclable catalysis for one-pot synthesis of biscoumarin derivatives, Catal. Lett., 2011, 141, 1651 CrossRef CAS
. - A. R. Hajipour, N. Sheikhan, M. A. Alaei and A. Zarei, Brønsted acidic ionic liquid as the efficient and reusable catalyst for synthesis of coumarins via Pechmann condensation under solvent-free conditions, Iran. J. Catal., 2015, 5, 231–236 CAS
. - H. R. Shaterian and M. Aghakhanizadeh, Ionic-liquid-catalyzed green synthesis of coumarin derivatives under solvent-free conditions, Chin. J. Catal., 2013, 34, 1690–1696 CrossRef CAS
. - S. Tiwari, J. Seijas, M. Vazquez-Tato, A. Sarkate, K. Karnik and A. Nikalje, Facile synthesis of novel coumarin derivatives, antimicrobial analysis, enzyme assay, docking study, ADMET prediction and toxicity study, Molecules, 2017, 22, 1172 CrossRef PubMed
. - S. Rezayati, F. Sheikholeslami-Farahani, F. Rostami-Charati and S. A. Sharif Abad, One-pot synthesis of coumarine derivatives using butylenebispyridinium hydrogen sulfate as novel ionic liquid catalyst, Res. Chem. Intermed., 2015, 42(5), 4097–4107 CrossRef
. - S. Mahato, S. Santra, R. Chatterjee, G. V. Zyryanov, A. Hajra and A. Majee, Brønsted acidic ionic liquid-catalyzed tandem reaction: An efficient approach towards regioselective synthesis of pyrano[3,2-c] coumarins under solvent-free conditions bearing lower E-factors, Green Chem., 2017, 19, 3282–3295 RSC
. - A. C. Cole, J. L. Jensen, I. Ntai, K. L. T. Tran, K. J. Weaver, D. C. Forbes and J. H. Davis, Novel brønsted acidic ionic liquids and their use as dual solvent-catalysts, J. Am. Chem. Soc., 2002, 124, 5962–5963 CrossRef CAS PubMed
. - S. Naz, M. Uroos and N. Muhammad, Effect of molecular structure of cation and anions of ionic liquids and co-solvents on selectivity of 5-hydroxymethylfurfural from sugars, cellulose and real biomass, J. Mol. Liq., 2021, 334, 116523 CrossRef CAS
. - S. Javed, Z. Mahmood, K. M. Khan, S. D. Sarker, A. Javaid, I. H. Khan and A. Shoaib, Lupeol acetate as a potent antifungal compound against opportunistic human and phytopathogenic mold Macrophomina phaseolina, Sci. Rep., 2021, 11, 8417 CrossRef CAS PubMed
.
|
This journal is © The Royal Society of Chemistry 2022 |
Click here to see how this site uses Cookies. View our privacy policy here.