DOI:
10.1039/D2RA04297E
(Paper)
RSC Adv., 2022,
12, 25280-25283
Palladium-catalyzed phosphorylation of arylsulfonium salts with P(O)H compounds via C–S bond cleavage†
Received
12th July 2022
, Accepted 31st August 2022
First published on 7th September 2022
Abstract
Herein we report a novel palladium-catalyzed phosphorylation of arylsulfonium salts with P(O)H compounds via C–S bond cleavage under mild conditions. The protocol provides a pragmatic strategy applicable to the synthesis of diverse arylphosphonates.
Arylphosphonates are ubiquitous constituents of numerous biologically active compounds, agrochemicals and functional materials,1 and also play an important role in organometallic catalysis2 and organocatalysis3 in organic synthesis. Ever since the pioneering work by Hirao and co-workers in the 1980s,4 various methods catalyzed or mediated by transition-metals for the synthesis of arylphosphonates have been developed. The cross-coupling reactions of P(O)H compounds with various aryl partners that have been reported so far include aryl halides,5 aryl boron reagents,6 aryl oxygen derivatives,7 aryl nitrogen reagents,8 and aryl amide derivatives,9 etc. (Scheme 1A).10
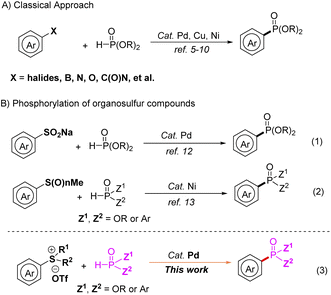 |
| Scheme 1 Transition metal-catalyzed phosphorylation reactions. | |
It is well known that organosulfur compounds have been of great importance in organic synthesis because of their accessibility and intriguing reactivity. As a valuable transformation of organosulfur compounds, transition-metal catalyzed C–S bond cleavage has attracted much attention.11 In this regard, Wang's group developed a novel palladium-catalyzed phosphonation of sodium arylsulfinates with H-phosphonate diesters in 2014 (Scheme 1B, eqn (1)).12 And then, Han's group developed the nickel-catalyzed phosphinylation of C–S bonds forming C–P bonds by using sulfides, sulfoxides and sulfones as substrates (Scheme 1B, eqn (2)).13 As a good choice for the aryl source, arylsulfonium salts are easily available, easy to handle, and reasonably reactive, they show great potential for transition metal-catalyzed transformation such as arylation, alkenylation, alkynylation, borylation, alkoxycarbonylation, and carboxylation,14 which can be viewed as an activated form of the inert C–S bond. As a continuation of our interest in the Ar–P bond construction,15 herein we wish to report palladium-catalyzed phosphorylation of arylsulfonium salts with P(O)H compounds (Scheme 1B, eqn (3)).
We commenced our investigation by studying the reaction conditions of arylsulfonium salts 1a with diethyl phosphonate 2a (Table 1). A series of Pd catalysts, including PdCl2, Pd2(dba)3, Pd(dppf)Cl2, Pd(PPh3)2Cl2, Pd(acac)2, and Pd(OAc)2 were examined in the presence of X-phos (5 mol%), K3PO4 (1.0 equiv.) in N,N-dimethylformamide (DMF) at 80 °C (Table 1, entries 1–6), which were found to be effective, thus affording the desired product 3a. Among these Pd catalysts, Pd(OAc)2 turned out to be the best catalyst for the reaction, affording the desired product in a yield of 58% (Table 1, entry 6). Subsequently, a survey of different phosphine ligands revealed that XPhos was the best choice (Table 1, entries 7–12). In order to improve the reaction yield, we opted to continue our optimization studies using different bases (Table 1, entries 13–16), but no better reactivity was achieved. Furthermore, the effect of the solvent was also probed (Table 1, entries 17–23). To our delight, iPrOH was found to be suitable for the reaction, affording the desired product 3a in 72% yield (Table 1, entry 22).
Table 1 Optimization of the reaction conditionsa,b
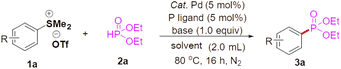
|
Entry |
Cat. |
P ligand |
Base |
Solvent |
Yieldb (%) |
1a (0.36 mmol), 2a (0.30 mmol), cat. Pd (5 mo%), ligand (5 mo%), base (0.3 mmol), solvent (2.0 mL), 80 °C, 16 h, under N2. Isolated yield. |
1 |
PdCl2 |
XPhos |
K3PO4 |
DMF |
40 |
2 |
Pd2(dba)3 |
XPhos |
K3PO4 |
DMF |
10 |
3 |
Pd(dppf)Cl2 |
XPhos |
K3PO4 |
DMF |
35 |
4 |
Pd(PPh3)2Cl2 |
XPhos |
K3PO4 |
DMF |
10 |
5 |
Pd(acac)2 |
XPhos |
K3PO4 |
DMF |
19 |
6 |
Pd(OAc)2 |
XPhos |
K3PO4 |
DMF |
58 |
7 |
Pd(OAc)2 |
dppe |
K3PO4 |
DMF |
5 |
8 |
Pd(OAc)2 |
PPh3 |
K3PO4 |
DMF |
6 |
9 |
Pd(OAc)2 |
dppp |
K3PO4 |
DMF |
15 |
10 |
Pd(OAc)2 |
JohnPhos |
K3PO4 |
DMF |
20 |
11 |
Pd(OAc)2 |
SPhos |
K3PO4 |
DMF |
32 |
12 |
Pd(OAc)2 |
Ruphos |
K3PO4 |
DMF |
30 |
13 |
Pd(OAc)2 |
XPhos |
K2HPO4 |
DMF |
50 |
14 |
Pd(OAc)2 |
XPhos |
Cs2CO3 |
DMF |
23 |
15 |
Pd(OAc)2 |
XPhos |
NaHCO3 |
DMF |
25 |
16 |
Pd(OAc)2 |
XPhos |
Et3N |
DMF |
21 |
17 |
Pd(OAc)2 |
XPhos |
K3PO4 |
DMSO |
43 |
18 |
Pd(OAc)2 |
XPhos |
K3PO4 |
DMA |
31 |
19 |
Pd(OAc)2 |
XPhos |
K3PO4 |
Dioxane |
Trace |
20 |
Pd(OAc)2 |
XPhos |
K3PO4 |
MeCN |
58 |
21 |
Pd(OAc)2 |
XPhos |
K3PO4 |
EtOH |
46 |
22 |
Pd(OAc)2 |
XPhos |
K3PO4 |
iPrOH |
72 |
23 |
Pd(OAc)2 |
XPhos |
K3PO4 |
tAmOH |
63 |
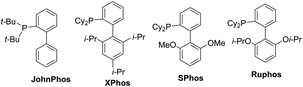 |
Having the optimized conditions established in hand, we then proceeded to explore the scope of the phosphorylation of various arylsulfonium salts with diethyl phosphonate 2a. The results were summarized in Table 2, in general moderate to good yields were obtained under the optimized reaction conditions. The phosphorylation reaction had shown excellent tolerance to both electron-withdrawing and electron-donating groups as aromatic substituents, including alkyl (1b–d), alkoxyl (1f–h), fluoro (1j), trifluoromethyl (1k), acetyl (1l), ester (1m–o), and acetamido groups (1p). For the substrate of arylsulfonium salts with the naphthalene group, the reaction gave the desired product in 70% yield. Surprisingly, the phosphorylation reaction could be conducted in the presence of unprotected active hydroxyl group (1i), affording 67% yield. The diminished yield in the case of 1d may be attributed to steric hindrance. The desired products were also obtained in good yields regardless of the alkyl substituents on the sulfonium moiety (1r–s). In addition, different substituents (1r–w) at the sulfur atom to evaluate the reactivity of leaving group on the Pd-catalyzed phosphorylation, several dialkyl-(phenyl) or alkyl(diphenyl)sulfonium triflates were tested under the optimized conditions. To our delight, the desired products were obtained in good to excellent yields (1t–v), in which ethyl- and propyl(diphenyl)-sulfonium triflates were more suitable, afforded 3a in 83%, and 89% yields, respectively.
Table 2 Scope of the catalytic phosphorylation of arylsulfonium saltsa,b
1 (0.36 mmol), 2a (0.30 mmol), Pd(OAc)2 (5 mo%), XPhos (5 mo%), K3PO4 (0.3 mmol), iPrOH (2.0 mL), 80 °C, 16 h, under N2. Isolated yield. |
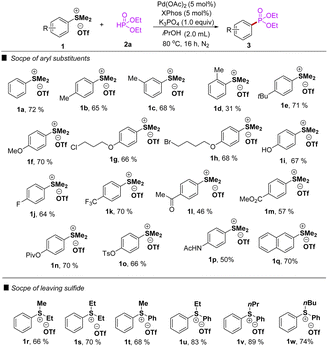 |
For further evaluation of the substrate scope, different P(O)H compounds were used to test the reaction. As shown in Scheme 3, the H-phosphonate diesters with different alkyl groups can all react smoothly with arylsulfonium salt 1v to afford the corresponding phosphorylation product (4a–c) in moderate to good yields. Ethyl phenylphosphinate (4d) had good compatibility under the optimized conditions to give the corresponding products in 82% yield. Next, some di-p-tolylphosphine oxides were subjected to the reaction at 110 °C, diphenyl- and di-p-tolylphosphine oxides show reactivity to afford the corresponding products 4e and 4f in 23% and 64% yield, respectively. Unfortunately, bis(4-fluorophenyl)-phosphine oxide was incompatible to the current catalytic system (4g) (Table 3).
Table 3 Scope of the catalytic phosphorylation of P(O)H compoundsa,b
1v (0.36 mmol), 2 (0.30 mmol), Pd(OAc)2 (5 mo%), XPhos (5 mo%), K3PO4 (0.3 mmol), iPrOH (2.0 mL), 80 °C, 16 h, under N2. Isolated yield. T = 110 °C. |
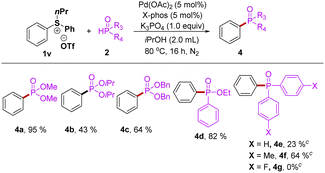 |
Interestingly, the (4-bromophenyl)dimethylsulfonium 2w was used to test the reaction, unexpected diphosphorylation product 5 and phosphorylation product bearing 4-methylthio group 6 were obtained, respectively in 22% and 35% yield (Scheme 2).
 |
| Scheme 2 Phosphorylation of the (4-bromophenyl)dimethyl sulfonium. | |
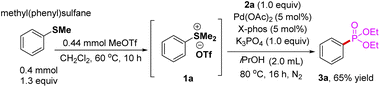 |
| Scheme 3 One-pot phosphorylation of aryl sulfide. | |
To prove the synthetic utility of the strategy, one-pot phosphorylation method was investigation. Treatment of methyl(phenyl)sulfane with MeOTf in 1,2-dichloroethane (DCE) afforded the corresponding aryl sulfonium salts 1a (confirmed with 1H NMR). After removal of all volatiles under a reduced pressure, one-pot phosphorylation was performed in the presence of Pd(OAc)2, XPhos, diethyl phosphonate 2a, K3PO4, and iPrOH. We are pleased to find that the reaction proceeded smoothly to provide the desired product 3a in 61% yield.
A plausible mechanism for the phosphorylation of arylsulfonium salts with P(O)H compounds was proposed as shown in Scheme 4. First, palladium(0) species A was generated from the reduction of palladium(II) with phosphine ligand by the aid of K3PO4.16 Then, arylsulfonium 1a would undergo oxidative addition with A to form cationic arylpalladium(II) B,17 followed by ligand exchange of diethyl phosphonate 2a with species B led to the generation of intermediate C in base condition.13 Subsequently, reductive elimination from C afforded arylphosphonate 3a and palladium (0) species A and the catalytic cycle was completed.
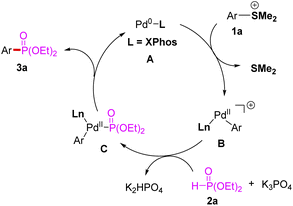 |
| Scheme 4 Plausible reaction mechanism. | |
In summary, we have developed a palladium-catalyzed phosphorylation of arylsulfonium salts with P(O)H compounds. The transformation proceeded under mild reaction conditions and had advantages include good functional group tolerance, a wide scope of substrates, and easily available arylsulfonium salts. Mechanistically, this approach involves oxidative addition and reductive elimination processes as the two key steps to afford the desired product. The protocol provides pragmatic strategy applicable to the synthesis of diverse arylphosphonates.
Conflicts of interest
There are no conflicts to declare.
Acknowledgements
This project was supported by the National Natural Science Foundation of China (No. 21762002).
Notes and references
-
(a) J. C. Tebby, Appl. Organomet. Chem., 2000, 14, 514 CrossRef CAS;
(b) D. S. Surry and S. L. Buchwald, Angew. Chem., Int. Ed., 2008, 47, 6338 CrossRef CAS PubMed;
(c) C. Queffélec, M. Petit, P. Janvier, D. A. Knight and B. Bujoli, Chem. Rev., 2012, 112, 3777 CrossRef PubMed.
- For selected reviews, see:
(a) M. McCarthy and P. J. Guiry, Tetrahedron, 2001, 57, 3809 CrossRef CAS;
(b) W. J. Tang and X. M. Zhang, Chem. Rev., 2003, 103, 3029 CrossRef CAS PubMed;
(c) R. Martin and S. L. Buchwald, Acc. Chem. Res., 2008, 41, 1461 CrossRef CAS PubMed.
- For selected reviews, see:
(a) X. Lu, C. Zhang and Z. Xu, Acc. Chem. Res., 2001, 34, 535 CrossRef CAS PubMed;
(b) J. L. Methot and W. R. Roush, Adv. Synth. Catal., 2004, 346, 1035 CrossRef CAS;
(c) Y. Wei and M. Shi, Acc. Chem. Res., 2010, 43, 1005 CrossRef CAS.
- T. Hirao, T. Masunaga, Y. Ohshiro and T. Agawa, Tetrahedron Lett., 1980, 21, 3595 CrossRef CAS.
-
(a) G. Keglevich, R. Henyecz, Z. Mucsi and N. Z. Kiss, Adv. Synth. Catal., 2017, 359, 4322 CrossRef CAS PubMed;
(b) H. Zeng, Q. Dou and C.-J. Li, Org. Lett., 2019, 21, 1301 CrossRef CAS;
(c) G. Zakirova, D. Y. Mladentsev and N. E. Borisova, Synthesis, 2019, 51, 2379 CrossRef CAS;
(d) M. S. Ghasemzadeh and B. Akhlaghinia, New J. Chem., 2019, 43, 5341 RSC;
(e) Y. Bai, N. Liu, S. Wang, S. Wang, S. Ning, L. Shi, L. Cui, Z. Zhang and J. Xiang, Org. Lett., 2019, 21, 6835 CrossRef CAS PubMed;
(f) M. N. Mungalpara, J. Wang, M. P. Coles, P. G. Plieger and G. J. Rowlands, Tetrahedron, 2018, 74, 5519 CrossRef CAS;
(g) S. Sengmany, A. Ollivier, E. L. Gall and E. Léonel, Org. Biomol. Chem., 2018, 16, 4495 RSC;
(h) A. I. Kuramshin, A. V. Plotnikova, M. V. Adel'shina and V. I. Galkin, Russ. J. Org. Chem., 2018, 54, 1746 CrossRef CAS;
(i) J. Xuan, T.-T. Zeng, J.-R. Chen, L.-Q. Lu and W.-J. Xiao, Chem.–Eur. J., 2015, 21, 4962 CrossRef CAS PubMed;
(j) D. Gelman, L. Jiang and S. L. Buchwald, Org. Lett., 2003, 5, 2315 CrossRef CAS;
(k) M. Kalek, M. Jezowska and J. Stawinski, Adv. Synth. Catal., 2014, 351, 3207 CrossRef.
-
(a) R. Zhuang, J. Xu, Z. Cai, G. Tang, M. Fang and Y. Zhao, Org. Lett., 2011, 13, 2110 CrossRef CAS PubMed;
(b) G. Hu, W. Chen, T. Fu, Z. Peng, H. Qiao, Y. Gao and Y. Zhao, Org. Lett., 2013, 15, 5362 CrossRef CAS PubMed;
(c) M. Andaloussi, J. Lindh, J. Sävmarker, P. J. R. Sjöberg and M. Larhed, Chem.–Eur. J., 2009, 15, 13069 CrossRef CAS PubMed;
(d) T. Fu, H. Qiao, Z. Peng, G. Hu, X. Wu, Y. Gao and Y. Zhao, Org. Biomol. Chem., 2014, 12, 2895 RSC;
(e) H. Wan, Y. Zhao, Q. Wang, Y. Zhang and Y. Li, Russ. J. Gen. Chem., 2016, 86, 150 CrossRef CAS;
(f) T.-H. Chen, D. M. Reddy and C.-F. Lee, RSC Adv., 2017, 7, 30214 RSC.
-
(a) G. Yang, C. Shen, L. Zhang and W. Zhang, Tetrahedron Lett., 2011, 52, 5032 CrossRef CAS;
(b) J. Yang, T. Chen and L.-B. Han, J. Am. Chem. Soc., 2015, 137, 1782 CrossRef CAS PubMed;
(c) J. Yang, J. Xiao, T. Chen and L.-B. Han, J. Org. Chem., 2016, 81, 3911 CrossRef CAS PubMed;
(d) L.-L. Liao, Y.-Y. Gui, X.-B. Zhang, G. Shen, H.-D. Liu, W.-J. Zhou, J. Li and D.-G. Yu, Org. Lett., 2017, 19, 3735 CrossRef CAS.
-
(a) W. Xu, G. Hu, P. Xu, Y. Gao, Y. Yin and Y. Zhao, Adv. Synth. Catal., 2014, 356, 2948 CrossRef CAS;
(b) R. Berrino, S. Cacchi, G. Fabrizi, A. Goggiamani and P. Stabile, Org. Biomol. Chem., 2010, 8, 4518 RSC;
(c) Y. He, H. Wu and F. D. Toste, Chem. Sci., 2015, 6, 1194 RSC;
(d) S. Wang, D. Qiu, F. Mo, Y. Zhang and J. Wang, J. Org. Chem., 2016, 81, 11603 CrossRef CAS PubMed.
-
(a) C. Liu and M. Szostak, Angew. Chem., Int. Ed., 2017, 56, 12718 CrossRef CAS;
(b) J. Dong, L. Liu, X. Ji, Q. Shang, L. Liu, L. Su, B. Chen, R. Kan, Y. Zhou, S.-F. Yin and L.-B. Han, Org. Lett., 2019, 21, 3198 CrossRef CAS.
- For selected examples, see:
(a) G. Yang, C. Shen, M. Quan and W. Zhang, Tetrahedron, 2016, 72, 333 CrossRef CAS;
(b) R. A. Dhokale and S. B. Mhaske, Org. Lett., 2013, 15, 2218 CrossRef CAS;
(c) C. Shen, G. Yang and W. Zhang, Org. Lett., 2013, 15, 5722 CrossRef CAS PubMed;
(d) C. Shen, G. Yang and W. Zhang, Org. Biomol. Chem., 2012, 10, 3500 RSC;
(e) C. Feng, M. Ye, K. J. Xiao, S. Li and J. Q. Yu, J. Am. Chem. Soc., 2013, 135, 9322 CrossRef CAS PubMed;
(f) T. Kagayama, A. Nakano, S. Sakaguchi and Y. Ishii, Org. Lett., 2006, 8, 407 CrossRef CAS PubMed;
(g) S. Wang, R. Guo, G. Wang, S.-Y. Chen and X.-Q. Yu, Chem. Commun., 2014, 50, 12718 RSC;
(h) C. Li, T. Yano, N. Ishida and M. Murakami, Angew. Chem., Int. Ed., 2013, 52, 9801 CrossRef CAS PubMed;
(i) X. Mao, X. Ma, S. Zhang, H. Hu, C. Zhu and Y. Cheng, Eur. J. Org. Chem., 2013, 4245 CrossRef CAS;
(j) J. S. Zhang, T. Chen, J. Yang and L. B. Han, Chem. Commun., 2015, 51, 7540 RSC;
(k) S. Wang, Q. Xue, Z. Guan, Y. Ye and A. Lei, ACS Catal., 2021, 11(7), 4295 CrossRef CAS;
(l) C. Liu, C.-L. Ji, T. Zhou, X. Hong and M. Szostak, Org. Lett., 2019, 21, 9256 CrossRef CAS PubMed;
(m) J.-S. Zhang, T. Chen, J. Yang and L.-B. Han, Chem. Commun., 2015, 51, 7540 RSC;
(n) L. Chen, Y. Zhu, T. Chen, L. Liu, J.-S. Zhang and L.-B. Han, Org. Biomol. Chem., 2018, 16, 5090 RSC;
(o) K. Xu, L. Liu, Z. Li, T. Huang, K. Xiang and T. Chen, J. Org. Chem., 2020, 85, 14653 CrossRef CAS;
(p) H. McErlain, L. M. Riley and A. Sutherland, J. Org. Chem., 2021, 86, 17036 CrossRef CAS PubMed.
- For selected reviews, see:
(a) S. R. Dubbaka and P. Vogel, Angew. Chem., Int. Ed., 2005, 44, 7674 CrossRef CAS;
(b) L. Wang, W. He and Z. Yu, Chem. Soc. Rev., 2013, 42, 599 RSC;
(c) S. G. Modha, V. P. Mehta and E. V. Van der Eycken, Chem. Soc. Rev., 2013, 42, 5042 RSC;
(d) F. Pan and Z.-J. Shi, ACS Catal., 2014, 4, 280 CrossRef CAS.
- T. Miao and L. Wang, Adv. Synth. Catal., 2014, 356, 967 CrossRef CAS.
- J. Yang, J. Xiao, T. Chen, S.-F. Yin and L.-B. Han, Chem. Commun., 2016, 52, 12233 RSC.
-
(a) J. Srogl, G. D. Allred and L. S. Liebeskind, J. Am. Chem. Soc., 1997, 119, 12376 CrossRef CAS;
(b) N.-N. Ma, J.-A. Ren, X. Liu, X.-Q. Chu, W. Rao and Z.-L. Shen, Org. Lett., 2022, 24, 1953 CrossRef CAS;
(c) D. Vasu, H. Yorimitsu and A. Osuka, Angew. Chem., Int. Ed., 2015, 54, 7162 CrossRef CAS PubMed;
(d) P. Cowper, Y. Jin, M. D. Turton, G. Kociok-Kohn and S. E. Lewis, Angew. Chem., Int. Ed., 2016, 55, 2564 CrossRef CAS PubMed;
(e) H. Kawashima, T. Yanagi, C.-C. Wu, K. Nogi and H. Yorimitsu, Org. Lett., 2017, 19, 4552 CrossRef CAS PubMed;
(f) J.-N. Zhao, M. Kayumov, D.-Y. Wang and A. Zhang, Org. Lett., 2019, 21, 7303 CrossRef CAS PubMed;
(g) S.-M. Wang, H.-X. Song, X.-Y. Wang, N. Liu, H.-L. Qin and C.-P. Zhang, Chem. Commun., 2016, 52, 11893 RSC;
(h) Z.-Y. Tian, S.-M. Wang, S.-J. Jia, H.-X. Song and C.-P. Zhang, Org. Lett., 2017, 19, 5454 CrossRef CAS PubMed;
(i) H. Minami, S. Otsuka, K. Nogi and H. Yorimitsu, ACS Catal., 2018, 8, 579 CrossRef CAS;
(j) H. Minami, K. Nogi and H. Yorimitsu, Org. Lett., 2019, 21, 2518 CrossRef CAS PubMed;
(k) F. Berger, M. B. Plutschack, J. Riegger, W. Yu, S. Speicher, M. Ho, N. Frank and T. Ritter, Nature, 2019, 567, 223 CrossRef CAS PubMed;
(l) T. Yanagi, R. J. Somerville, K. Nogi, R. Martin and H. Yorimitsu, ACS Catal., 2020, 10, 2117 CrossRef CAS.
-
(a) H. Luo, H. Liu, X. Chen, K. Wang, X. Luo and K. Wang, Chem. Commun., 2017, 53, 956 RSC;
(b) H. Luo, K. Sun, Q. Xie, X. Li, X. Zhang and X. Luo, Asian J. Org. Chem., 2020, 9, 2083 CrossRef CAS;
(c) K. Sun, H. Liu, Q. Xie and H. Luo, Chin. J. Org. Chem., 2020, 40, 2275 CrossRef CAS.
-
(a) R. Martin and S. L. Buchwald, Acc. Chem. Res., 2008, 41, 1461 CrossRef CAS;
(b) J. R. DeBergh, N. Niljianskul and S. L. Buchwal, J. Am. Chem. Soc., 2013, 135, 10638 CrossRef CAS PubMed.
- H. Minami, K. Nogi and H. Yorimitsu, Org. Lett., 2019, 21, 2518 CrossRef CAS PubMed.
|
This journal is © The Royal Society of Chemistry 2022 |
Click here to see how this site uses Cookies. View our privacy policy here.