DOI:
10.1039/D2RA04385H
(Paper)
RSC Adv., 2022,
12, 31466-31477
Development of novel isatin thiazolyl-pyrazoline hybrids as promising antimicrobials in MDR pathogens†
Received
15th July 2022
, Accepted 27th October 2022
First published on 2nd November 2022
Abstract
Microbial Multidrug Resistance (MDR) is an emerging global crisis. Derivatization of natural or synthetic scaffolds is among the most reliable strategies to search for and obtain novel antimicrobial agents for the treatment of MDR infections. Here, we successfully manipulated the synthetically flexible isatin moieties to synthesize 22 thiazolyl-pyrazolines hybrids, and assessed their potential antimicrobial activities in vitro against various MDR pathogens, using the broth microdilution calorimetric XTT reduction method. We chose 5 strains to represent the major MDR microorganisms, viz: Methicillin-resistant S. aureus (MRSA), and Vancomycin-resistant E. faecalis (VRE) as Gram-positive bacteria; Carbapenem-resistant K. pneumonia (CRKP), and Extended-spectrum beta-lactamase E. coli (ESBL-E), as Gram-negative bacteria; and Fluconazole-resistant C. albicans (FRCA), as a yeast-like unicellular fungus. The cytotoxicity of compounds 9f and 10h towards mammalian lung fibroblast (MRC-5) cells demonstrated their potential satisfactory safety margin as represented by their relatively high IC50 values. The target compounds showed promising anti-MDR activities, suggesting they are potential leads for further development and in vivo studies.
1 Introduction
Since their discovery, antimicrobial agents have been a reliable weapon in fighting life-threatening infections. However, some pathogens are naturally resistant to some antimicrobials, and several other microorganisms have become resistant through natural genetic selection and/or passing the resistance factor(s) from one organism to another, thus propagating the resistance(s) between different organisms.1 The excessive and irresponsible use of antimicrobials has played a major role in an emerging global crisis of drug resistance. Additionally, the noticeable lack of investment in new drug discovery efforts by pharma – due to low profits and harsh regulations – has exacerbated the problem.2
Multidrug resistance (MDR) is the acquired insensitivity of a microorganism toward several structurally distinct classes of antimicrobials that have different molecular targets.3 Although MDR usually evolves in nosocomial infections, several have become a widespread cause of public acquired infections.4 Opportunistic pathogenic yeast Candida albicans; Gram-negative bacteria Escherichia coli, Klebsiella pneumoniae, and Pseudomonas aeruginosa; as well as Gram-positive Vancomycin-resistant enterococcus (VRE) and Methicillin-resistant Staphylococcus aureus (MRSA) are amongst the well-known microorganisms capable of developing MDR.4 By 2050, mortality rates from infections are expected to rise significantly, and it is estimated that 10 million people will be at risk of premature death if this problem has not been addressed.5 Therefore, it's crucial to develop new antimicrobial agents with potent activity against MDR pathogens.
Strategies, to obtain new antimicrobial agents for the treatment of MDR infections, range from the production of analogs or derivatives of existing drug molecules to the discovery of novel natural or synthetic scaffolds.6 Isatin (I, Fig. 1) is a well-known heterocyclic natural compound that can be found in the human body as a metabolite of adrenaline, as well as in different plants such as Isatis and Couroupita genera.7 The synthetic flexibility of the isatin building block has prompted its broad implementation in targeted derivatization to generate novel pharmacologically active molecules.7 For example, isatin-derived Sunitinib is a multi-targeted receptor tyrosine kinase inhibitor that has been approved by the FDA for the treatment of various tumours.8 In addition, many isatin derivatives have shown antiprotozoal,9 antiviral,10 and anti-cancer activities.9,11–13
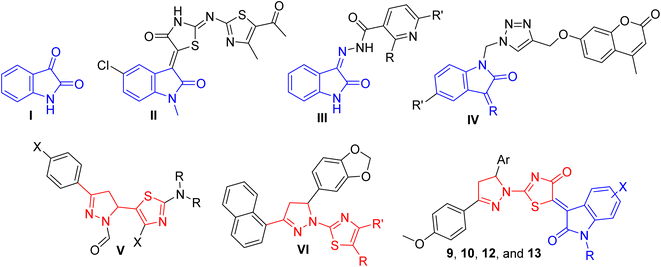 |
| Fig. 1 Chemical structure of isatin(I), antimicrobial isatin derivatives (II–IV), antimicrobial thiazolyl-pyrazoline derivatives, as well as the compounds reported in this manuscript 9a–h, 10a–h, 12a–c, and 13a–c. | |
In a previous work, we reported that the hybrid structures from combining thiazolylamino–thiazolidinone with isatin motifs are a successful approach to developing a promising scaffold with good antimicrobial and anti-mycobacterial activities. In particular, compound II (Fig. 1) demonstrated promising activity toward both VRE and MRSA (MIC = 7.81 and 3.90 mg ml−1, respectively).14 In another recent study, we reported the potent activity of isatin-based nicotinohydrazide derivatives III (Fig. 1) against bronchitis causing K. pneumonia as well as against isoniazid/streptomycin-resistant Mycobacterium tuberculosis.15 Similarly, isatin–triazole–coumarin hybrids IV (Fig. 1) showed promising activity against M. tuberculosis.16
Other heterocyclic compounds with electron-rich nitrogen and/or sulfur atoms are also recognized for their pharmacological activities. They are widely distributed in nature as alkaloids, vitamins, and glycosides.17 Pyrazolines, for instance, possess antimicrobial, anti-tubercular, anticancer, and anti-inflammatory activities.18,19 Notably, the replacement of the β-lactam ring with a pyrazolidinone ring in penicillins has retained the antibiotic activity.20 Similarly, thiazoles, have exhibited antimicrobial,21,22 anti-tuberculosis,23 and antiviral activities.24,25 Remarkably, compounds that contain thiazolyl-pyrazolines (V, Fig. 1) moieties showed effective inhibition against methicillin-susceptible/-resistant S. aureus, and vancomycin-intermediate S. aureus.26 In addition, a variety of derivatives demonstrated diverse biological activities such as antimicrobial (VI, Fig. 1),17,27 antiprotozoal28,29 anti-proliferative,27,30,31 and anti-Alzheimer32 activities.
In this study, we describe the design, synthesis, and biological evaluation of a novel set of isatin thiazolyl-pyrazolines hybrid small molecules (9a–h, 10a–h, 12a–c, and 13a–c, Fig. 1) as potential antimicrobial agents against various MDR pathogens. The antimicrobial activities of the newly synthesized molecules are screened in vitro, using the broth microdilution calorimetric XTT reduction method, against 5 MDR strains viz; Methicillin-resistant Staphylococcus aureus (MRSA), Vancomycin-resistant Enterococcus faecalis (VRE), Carbapenem-resistant Klebsiella pneumonia (CRKP), Extended-spectrum beta-lactamase Escherichia coli (ESBL-E), and Fluconazole-resistant Candida albicans.
2. Results and discussion
2.1. Chemistry
The synthetic methodology adopted to obtain target compounds (9a–h, 10a–h, 12a–c, and 13a–c) mainly involved straightforward condensation and/or cycloaddition chemistry (Schemes 1 and 2). Firstly, chalcone derivatives 3a-b were synthesized by a simple Claisen–Schmidt condensation reaction of the precursor benzaldehyde derivatives (2a-b) each with p-methoxyacetophenone (Scheme 1).
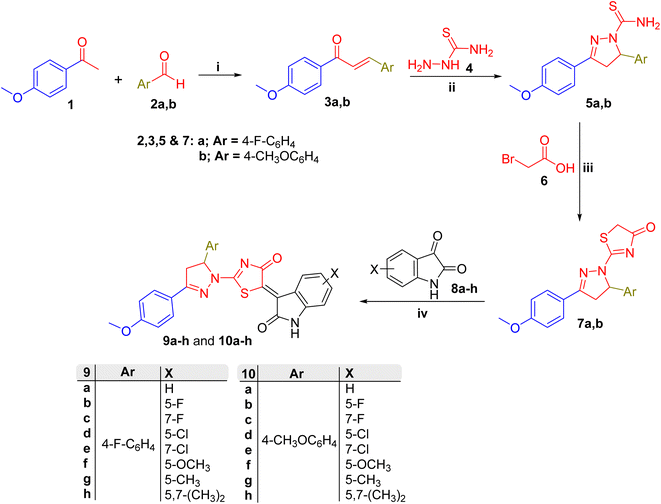 |
| Scheme 1 Reagent and conditions: (i) KOH (40%), ethanol/water 1 : 1, stirring 4 h, rt; (ii) NaOH (40% aq) (4 equiv.)/ethanol, reflux 3 h; (iii) NaOAc (2 equiv.)/AcOH, reflux 5 h; (iv) NaOAc (2 equiv.)/AcOH, reflux 3 h. | |
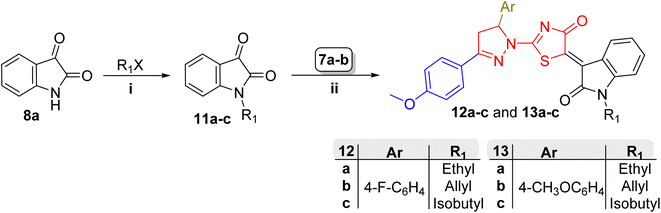 |
| Scheme 2 Reagent and conditions: (i) K2CO3 (2 equiv.), KI (0.2 equiv.)/DMF, stirring 6 h, 80 °C; (ii) NaOAc (2 equiv.)/AcOH, reflux 3 h. | |
The positive mesomeric effect exerted by the p-methoxy group in p-methoxyacetophenone may account for the relatively low acidity of the α-CH3 group and harsher enolization conditions (40% KOH) in this reaction.
Treatment of chalcones 3a-b with thiosemicarbazide (4) afforded pyrazoline derivatives 5a-b. The reaction proceeds regioselectively through a semicarbazone intermediate, formed by nucleophilic attack of the hard thiosemicarbazide N1 nucleophilic site to the hard carbonyl electrophilic site (see the Hard and Soft Acid and Base (HSAB) theory33). This rapidly cyclizes to afford the desired pyrazolines 5a-b by intramolecular addition reaction of the softer nucleophilic end (thiosemicarbazone N2) to the softer electrophilic end (β-carbon of the α,β-unsaturated C
N). Overall, only one regioisomer of the pyrazoline nucleus is formed (5a-b). The reaction requires base catalysis (40% aqueous NaOH) likely for activation of thiosemicarbazone N2 via deprotonation prior to intramolecular addition. It is noteworthy that the sp3 carbon atom (pyrazoline C5) formed during this reaction is asymmetric. Whilst the reaction is not enantioselective and the formation of 5a-b leads to a racemic mixture, the adjacent methylene group hydrogens (at pyrazoline C4) are now diastereotopic. This feature was clearly found in the splitting pattern of pyrazoline C4 protons in compounds 5, 7, 9, 10, 12 and 13 in the NMR spectra. Mutual second-order geminal, as well as vicinal (trans or cis) coupling, were observed (see 1H NMR charts of 9, 10, 12 and 13 in the ESI†) between C4 protons and with C4–C5 protons J values as high as 18 (geminal at C4) and 11 or 4 Hz (vicinal trans or cis), respectively.
Similarly, the free ambident nucleophilic 1-carbothioamide moiety of 5a-b was utilized in a second [3 + 2] cyclization reaction; here with bromoacetic acid (6) as the ambident electrophile (Scheme 1). Again, by applying the HSAB theory, the ‘softer’ nucleophilic site (sulfur of 5) reacts regioselectively with the ‘softer’ electrophilic site (α-bromo-substituted carbon of 6). This afforded the desired pyrazolin-1-ylthiazol-4(5H)-ones (7a-b). Due to the low electrophilicity of the COOH group (resulting from the poor leaving group ability of OH), the reaction required a longer reflux period in dehydrating conditions (glacial acetic acid). A similar cyclization reaction was previously reported to afford oxazol-4(5H)-one nucleus (instead of thiazol-4(5H)-one) from the precursor hippuric acid derivative (carbamide derivative instead of carbothioamide illustrated herein) showing similar regioselectivity, while requiring harsher conditions (acetic anhydride instead of acetic acid).34 Both compound series 5a-b and 7a-b were previously reported (see the Experimental section for further details).
Both N1-unsubstituted and N1-substituted isatin derivatives were utilized for the synthesis of final compounds, (9/10a–h) and (12/13a–c) series respectively. The N1-substituted isatin derivatives were prepared by N1-alkylation of isatin (8a) via SN2 reaction with the corresponding alkyl halide (R1X, Scheme 2). For this reaction, primary alkyl halides (including the reactive allyl bromide), anhydrous potassium carbonate (as a base), and a polar aprotic solvent (DMF) were utilized to enhance the SN2 reaction conditions. It is noteworthy that the reaction rate could be enhanced as a result of adding a catalytic amount of potassium iodide as a nucleophilic catalyst (Finkelstein reaction) as has been demonstrated recently by our group.35 This reaction afforded N1-alkyl isatin derivatives 11a–c which were previously reported (see the Experimental section for further details).
Finally, target compound series (9/10a–h) and (12/13a–c) were synthesized by Knoevenagel condensation of the active methylene group within the pyrazolin-1-ylthiazol-4(5H)-one derivatives (7a-b) with the ketonic C
O group of the isatin derivatives 8a–h and 11a–c, respectively. The reaction was carried out in dehydrating conditions (refluxing glacial acetic acid) and reached completion within a relatively short period (3 h) by using a mild solvent-compatible base (sodium acetate). Analysis of the NMR spectra of the products confirms that the active methylene group at C4 of the pyrazoline ring, rather than that at C5 of thiazol-4(5H)-one (within the 7 nucleus, Scheme 1), is involved in the condensation reaction.
The 1H NMR charts of compound series (9/10a–h and 12/13a–c) (ESI†) clearly show that the pyrazoline ring was intact (showing geminal coupling of J = 18 Hz for the diastereotopic C4 protons at δ = 3.4–4.2 ppm, as discussed earlier) while the C5 methylene of thiazol-4(5H)-one is absent which conclusively shows the latter to be involved in the Knoevenagel condensation. Analogous activation of the C5 methylene group of oxazol-4(5H)-one derivative (closely related to thiazol-4(5H)-one) was carried out at similarly mild conditions (sodium acetate base at low temperature) to allow its coupling with diazonium salt (reactive electrophile).34
The regioselective Knoevenagel condensation at the thiazol-4(5H)-one (rather than pyrazoline) ring of 7 series is explained in terms of relatively easier enolization of thiazol-4(5H)-one nucleus (by deprotonation of C5 methylene group) affording stable 7-anion [I] unlike the alternative deprotonation of pyrazoline C4 which affords the less stable 7-anion [II], Scheme 3. The relatively enhanced 7-anion [I] stability difference is likely attributed to its aromatic nature, the strong negative mesomeric effect of the exocyclic carbonyl at C4 alongside sulfur atom of the thiazol-4(5H)-one ring being able to stabilize an adjacent negative charge via accepting electrons in its higher vacant orbitals,36 as shown in the canonical resonating structures outlined in Scheme 3.
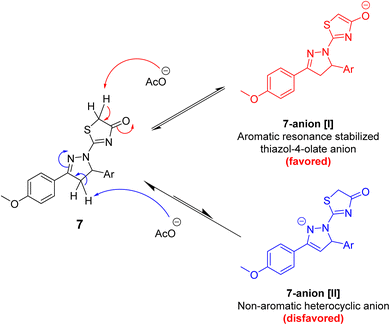 |
| Scheme 3 Structure and stability of 7-anions reflecting the relative ease of their formation upon treating 7 with a mild base and providing evidence of the regioselective Knoevenagel condensation at the thiazol-4(5H)-one ring to afford target compounds. | |
Compounds (9/10a–h and 12/13a–c) were fully characterised, their structures were confirmed by 1H and 13C NMR (See the NMR reports in the Experimental section and the spectral charts in the ESI†), and their purity was assessed by HRMS and/or CHN elemental analysis.
2.2. Biological evaluation
2.2.1. Antibacterial activities. The antibacterial activity of the synthesized isatin derivatives was screened in vitro, using the broth microdilution calorimetric XTT reduction method, against 4 MDR strains viz; Methicillin-resistant Staphylococcus aureus (MRSA, ATCC 700788) and Vancomycin-resistant Enterococcus faecalis (VRE, BAA-2365) strains as examples of Gram-positive bacteria; Carbapenem-resistant Klebsiella pneumonia (CRKP, ATCC BAA-2342) and Extended-spectrum beta-lactamase Escherichia coli (ESBL-E, BAA-199) strains to represent Gram-negative bacteria.Table 1 demonstrates the values of growth inhibition (% at 1.95 μg ml−1) and the minimum inhibitory concentration (MIC, μg ml−1) of the synthesized derivatives (9/10a–h and 12/13a–c) against MDR Gram-positive bacterial strains (MRSA and VRE). Generally, MRSA was more sensitive to the tested derivatives than VRE. Compounds 9f and 10h demonstrated 100% inhibition against MRSA at the applied concentration, with MIC of 0.98 μg ml−1. Similarly, compound 10f showed 100% inhibition, but with almost double the MIC (1.95 μg ml−1). Against VRE, the compounds 9f, 10h, and 10f retained outstanding activities, with percent inhibition ranging between 60–73%. Compound 9f was the most active with a MIC of 7.80 μg ml−1, which is approximately half that of compounds 10h and 10f (MIC of 15.63 μg ml−1). Compounds 9b, 9d, 9g, 9h, 11g, 12b, and 13b exhibited the same MIC (3.90 μg ml−1) against MRSA with percent inhibition that ranged between 80–95%, while against VRE, they showed percent inhibition of 50–60% with relatively higher MIC that ranged between 15.63 to 31.25 μg ml−1. The remaining compounds showed moderate, weak, or no activities against either strain.
Table 1 Antibacterial activity of the synthesized isatin derivatives (9/10a–h and 12/13a–c) against MDR Gram-positive strains; expressed as the mean of inhibitory percentages (inhibitory % at 1.95 μg ml−1), and minimum inhibitory concentrations (MIC) in μg ml−1
Compound |
% Inhibition with 1.95 μg ml−1 |
MIC (μg ml−1) |
MRSA |
VRE |
MRSA |
VRE |
NA: no activity. |
9a |
21.96 ± 1.1 |
NA |
250 |
NAa |
9b |
89.35 ± 0.74 |
52.19 ± 2.2 |
3.9 |
31.25 |
9c |
41.23 ± 2.2 |
21.79 ± 2.2 |
62.5 |
250 |
9d |
95.17 ± 1.3 |
61.23 ± 1.4 |
3.9 |
31.25 |
9e |
69.17 ± 1.1 |
32.17 ± 1.3 |
7.81 |
125 |
9f |
100.00 ± 0.00 |
73.25 ± 1.2 |
0.98 |
7.81 |
9g |
92.17 ± 0.96 |
56.31 ± 1.9 |
3.9 |
15.63 |
9h |
92.15 ± 0.00 |
52.17 ± 1.3 |
3.9 |
31.25 |
10a |
59.31 ± 1.4 |
NA |
15.63 |
NA |
10b |
63.25 ± 1.3 |
NA |
15.63 |
NA |
10c |
71.95 ± 1.8 |
39.27 ± 0.99 |
7.81 |
62.5 |
10d |
73.24 ± 0.97 |
50.64 ± 1.7 |
7.81 |
31.25 |
10e |
19.36 ± 0.92 |
NA |
250 |
NA |
10f |
100.00 ± 0.00 |
63.28 ± 0.84 |
1.95 |
15.63 |
10g |
82.19 ± 1.3 |
50.16 ± 0.58 |
3.9 |
31.25 |
10h |
100.00 ± 0.00 |
60.19 ± 1.9 |
0.98 |
15.63 |
12a |
71.29 ± 1.1 |
39.25 ± 1.6 |
7.81 |
125 |
12b |
91.35 ± 1.6 |
53.96 ± 0.85 |
3.9 |
62.5 |
12c |
53.21 ± 1.7 |
23.17 ± 2.2 |
62.5 |
500 |
13a |
11.96 ± 1.1 |
NA |
>1000 |
NA |
13b |
79.85 ± 1.3 |
41.96 ± 0.96 |
3.9 |
62.5 |
13c |
42.19 ± 0.85 |
NA |
125 |
NA |
Vancomycin |
98.84 ± 1.69 |
|
1.95 |
|
Linezolid |
|
91.96 ± 2.83 |
|
7.81 |
It can be inferred from the results that variation in type or position of the substituents has a profound impact on the activity of the derivatives. In the p-fluoroaryl series (9a–h), the 5-substituent on the isatin ring is critical to the activity. The derivative with a 5-methoxy substitution, compound 9f, was able to show the most potent activity against both MRSA and VRE. However, derivatives with 5-F (9b), 5-Cl (9d), 5-methyl (9g), or 5,7 dimethyl (9h) have reduced the efficacy by about 4 folds. Lack of a substituent on the isatin (9a), or shifting the substitution to position 7 (9c and 9e), dramatically diminished the activity. In contrast, in the p-methoxyaryl series (10a–h), compound 10h with a 5,7-dimethyl substituent on the isatin motif was the most potent, showing similar activity as compound 9f. Interestingly though, compound 10f with the 5-methoxy isatin moiety was still potent although showing a 2-fold increase in the MIC over 9f. Compound (10g) with 5-methyl isatin showed similar potency to 9g. 5-Halo substituents were significantly less potent mirroring the observation in the p-fluoroaryl series 9. N-substitution on the isatin ring (12/13a–c) in general reduced the efficacy, suggesting the importance of the free (NH) for the activity. Anyway, N-substituting with an allyl group (12b and 13b) retained some activity, whereas ethyl (12a and 13a) or isobutyl (12c and 13c) substituents almost abolished the activity.
Table 2 demonstrates the activities against MDR Gram-negative strains (ESBL-E and CRKP). Generally speaking, the compounds are more potent against Gram-negative strains. In the p-fluoroaryl series, compound 9f again demonstrated 100% inhibition against both ESBL-E and CRKP, with MIC of 0.24 μg ml−1 and 0.98 μg ml−1 respectively. In the p-methoxyaryl series 10, compound 10h was again potent showing 100% inhibition against ESBL-E and 80% against CRKP, with an impressive MIC of 0.48 μg ml−1 against ESBL-E, and 1.95 μg ml−1 against CRKP. Compounds 9d, 9g, 9h, 10f, & 12b demonstrated 100% inhibition toward ESBL-E, with MIC of 0.98 μg ml−1 for compounds 9g & 10f, while that for the rest of the compounds being 1.95 μg ml−1. Toward CRKP, the compounds showed 70–80% inhibition with compounds 9d, 10f, & 12b having MIC of 3.9 μg ml−1, while the other two compounds' MIC was 7.81 μg ml−1. Compounds 9b, 9e, 10c, 10d, 10g, and 13b exhibited the same MIC (3.9 μg ml−1) against ESBL-E with percent inhibition that varied between 76–100%. Against CRKP, the latter group of compounds showed percent inhibition of 70–80% with MIC that ranged from 3.9 to 7.81, except for compound 9e which declined to 61% inhibition and 31.25 μg ml−1 MIC. The remaining compounds showed moderate or weak, to almost non-sensible activities against both strains.
Table 2 Antibacterial activity of the synthesized isatin derivatives (9/10a–h and 12/13a–c) against MDR Gram-negative strains; expressed as the mean of inhibitory percentages (inhibitory % at 1.95 μg ml−1), and minimum inhibitory concentrations (MIC) in μg ml−1
Compound |
Inhibitorya % |
MIC (μg ml−1) |
ESBL-E. coli |
CRKP |
ESBL-E. coli |
CRKP |
NA: no activity. |
9a |
18.37 ± 1.2 |
6.21 ± 1.8 |
500 |
>1000 |
9b |
100.00 ± 0.00 |
80.19 ± 1.3 |
3.9 |
3.9 |
9c |
36.27 ± 1.9 |
57.31 ± 1.7 |
62.5 |
15.63 |
9d |
100.00 ± 0.00 |
79.24 ± 1.8 |
1.95 |
3.9 |
9e |
76.25 ± 1.7 |
61.08 ± 0.58 |
3.9 |
31.25 |
9f |
100 ± 0.00 |
100 ± 0.00 |
0.24 |
0.98 |
9g |
100 ± 0.00 |
71.24 ± 0.87 |
0.98 |
7.81 |
9h |
100 ± 0.00 |
71.68 ± 1.1 |
1.95 |
7.81 |
10a |
38.19 ± 1.4 |
17.92 ± 1.6 |
125 |
500 |
10b |
52.17 ± 0.58 |
26.47 ± 1.3 |
31.25 |
250 |
10c |
81.74 ± 0.58 |
69.25 ± 1.6 |
3.9 |
7.81 |
10d |
86.25 ± 2.1 |
71.25 ± 1.8 |
3.9 |
7.81 |
10e |
19.68 ± 1.5 |
12.32 ± 0.89 |
500 |
500 |
10f |
100.00 ± 0.00 |
81.74 ± 1.6 |
0.98 |
3.9 |
10g |
91.26 ± 0.82 |
76.32 ± 0.88 |
3.9 |
3.9 |
10h |
100.00 ± 0.00 |
79.35 ± 0.74 |
0.48 |
1.95 |
12a |
69.245 ± 2.1 |
41.08 ± 1.7 |
7.81 |
125 |
12b |
100.00 ± 0.00 |
83.29 ± 2.1 |
1.95 |
3.9 |
12c |
56.39 ± 2.1 |
32.19 ± 0.74 |
31.25 |
250 |
13a |
32.96 ± 0.69 |
NA |
250 |
NA |
13b |
81.39 ± 1.6 |
68.96 ± 1.3 |
3.9 |
7.81 |
13c |
43.19 ± 1.8 |
14.97 ± 0.97 |
125 |
500 |
Colistin |
100.00 ± 0.00 |
97.44 ± 2.18 |
0.98 |
1.95 |
The results indicate that, in the p-fluoroaryl series (9a–h), compound 9f showed the highest potency against both ESBL-E and CRKP. Replacing its 5-methoxy with 5-methyl (9g), 5,7 dimethyl (9h), or with a relative halogen (5-Cl, 9d), retained similar activities, especially against ESBL-E. Exchanging the 5-Cl with a smaller F-group (9b) or shifting to position 7 (9e) noticeably decreased the activities. The lack of any substituent on the isatin ring (9a) or the presence of a small fluoro substitution on position 7 (9c) almost abrogated the activity, proving the importance of 5-isatin substitution for the activity. In the p-methoxyaryl series (10a–h), compound 10h with 5,7-dimethyl isatin moiety showed similar activity as compound 9f, while shifting back to the 5-methoxy isatin (10f) maintained significant activity with MIC only doubled. Removing or swapping the isatin substituent with an alkyl or halo group considerably decreased or diminished the activity. Again, substitution on the NH of the isatin ring (12/13a–c) generally reduced the efficiency, except for compounds (12b & 13b) which retained considerable activity, emphasising the role of the allyl-substitution in regaining the lost activity due to the isatin-NH substitution.
2.2.2. Antifungal activity. The antifungal activity of the synthesized isatin derivatives was screened in vitro, using the broth microdilution calorimetric XTT reduction method, against an MDR strain viz; Fluconazole-resistant Candida albicans (FRCA, ATCC-MYA-1003A) strain as a yeast-like unicellular fungus.Table 3 presents the values of % inhibition and MIC of the compounds against Fluconazole-resistant Candida albicans (FRCA). Compound 9f again was the most potent and showed 82% inhibition, with MIC of 3.9 μg ml−1. Compounds 10d, 10f, 10h, & 12b demonstrated 60–70% inhibition with MIC of 7.81 μg ml−1. Compounds 9b, 9d, 9g, & 9h exhibited the same MIC (15.63 μg ml−1) with percent inhibition ranging around 60%. The remaining compounds showed moderate, weak, or negligible antifungal activity.
Table 3 Antifungal activity of the synthesized isatin derivatives (9/10a–h and 12/13a–c) against Fluconazole-resistant Candida albicans (ATCC-MYA-1003); expressed as the mean of inhibitory percentages (inhibitory % at 1.95 μg ml−1), and minimum inhibitory concentrations (MIC) ina μg ml−1
Compound |
Inhibitory % |
MIC (μg ml−1) |
NA: no activity. |
9a |
NA |
NA |
9b |
61.87 ± 1.1 |
15.63 |
9c |
24.16 ± 1.1 |
250 |
9d |
63.75 ± 2.1 |
15.63 |
9e |
56.31 ± 1.8 |
31.25 |
9f |
81.69 ± 1.8 |
3.9 |
9g |
58.91 ± 0.85 |
15.63 |
9h |
60.82 ± 0.85 |
15.63 |
10a |
9.12 ± 0.63 |
>1000 |
10b |
12.32 ± 1.9 |
500 |
10c |
51.74 ± 0.82 |
62.5 |
10d |
60.74 ± 0.73 |
7.81 |
10e |
NA |
NA |
10f |
69.25 ± 0.58 |
7.81 |
10g |
36.26 ± 1.4 |
125 |
10h |
65.73 ± 0.67 |
7.81 |
12a |
32.19 ± 1.3 |
125 |
12b |
67.98 ± 1.6 |
7.81 |
12c |
19.87 ± 1.9 |
500 |
13a |
NA |
NA |
13b |
46.32 ± 0.58 |
31.25 |
13c |
NA |
NA |
Ketoconazole |
97.24 ± 2.05 |
3.9 |
Among the p-fluoroaryl derivatives 9, the 5-methoxy substituted 9f once more has shown the highest potency as antifungal, roughly the same as that observed for anti-Gram-positive bacteria. Replacing the 5-methoxy with any 5-substituent slightly reduced the activity with about a 4× increase in the MIC. Removing or shifting the substitution to position 7, nearly eliminated the activity. In the p-methoxyaryl series 10, compounds with 5-Cl, 5-methoxy, or 5,7-dimethyl (10d, 10f or 10h) showed good potency with MIC only doubled compared to 9f. Removing, replacing the isatin substituent with an alkyl or small F group, or shifting to position 7 considerably abolished the activity. Once again, substitution on the NH, except for allyl compounds (12b & 13b), mostly diminished the activity.
Finally, it is noteworthy that the results confirm the superiority of compound 9f over the other derivatives as an antifungal as well as broad-spectrum antibacterial activities against Gram-positive and Gram-negative bacteria, which strongly advocates further investigation of this molecule as a promising antimicrobial compound against MDR pathogens.
2.2.3. Lack of cytotoxic activity towards mammalian cells. With the aim of exploring the safety profile of our lead compounds 9f and 10h, we analyzed their cell growth inhibitory activity in non-tumorigenic lung fibroblast MRC-5 cell line utilizing the SRB assay.37,38 As indicated in Table 4, both the examined molecules 9f and 10h displayed only very weak cytotoxicity with IC50 values of 45.02 ± 2.5 and 58.10 ± 3.2 μM, respectively.
Table 4 Cytotoxicity of compounds 9f and 10h towards non-tumorigenic lung fibroblast (MRC-5) cells
Comp |
IC50 (μM)a |
IC50 values are the mean of three separate experiments. |
9f |
45.02 ± 2.5 |
10h |
58.10 ± 3.2 |
Doxorubicin |
37.41 ± 1.7 |
3. Conclusions
Microbial resistance emerges through natural selection and/or passing the resistance factor(s) from one organism to another, leading to the global health crisis of Multidrug Resistance (MDR). In this study, we followed the approach of hybridizing the natural scaffold isatin with the synthetic thiazolyl-pyrazolines to produce 22 novel compounds, and screen them as potential antimicrobials. The in vitro screening revealed that MRSA was more sensitive to all tested compounds than VRE. The p-fluoroaryl compounds showed relatively high potency against both, as well as against ESBL-E, CRKP, and FRCA. The SAR studies showed that the variation in the type or position of the substituents has a profound impact on the activity of the tested derivatives. In the p-fluoroaryl series, the 5-substituent on the isatin ring is critical to the activity. Generally speaking, replacing the 5-methoxy with any 5-substituent maintained the activity but with an about 4-fold increase in the MIC. Removing or shifting the substitution to position 7, nearly abolished the activity. Isatin N-substitution, in general, has a negative impact on the efficacy, showing the importance of the free (NH) for the activity. Remarkably compounds 9f and 10h have shown superior potency over all other derivatives, being the most active derivatives as antifungal alongside as well as broad-spectrum antibacterial activity against Gram-positive and Gram-negative bacteria. The cytotoxicity of compounds 9f and 10h towards mammalian lung fibroblast (MRC-5) cells demonstrated their potential satisfactory safety margin as represented by their relatively high IC50 values (∼50 μM) in this cell line. This strongly supports the potential of these molecules as leads for MDR antimicrobial and further development and in vivo studies.
4. Experimental
4.1. Chemistry
4.1.1. General. Melting points were measured with a Stuart melting point apparatus and were uncorrected. The NMR spectra were recorded by Bruker 400 MHz spectrometer operating at 400 MHz (1H) and 101 MHz (13C) using deuterated trifluoroacetic acid or deuterated dimethylsulfoxide (DMSO-d6) as solvent. Elemental analyses were carried out using Thermo Scientific FLASH 2000 CHNS/O analyzer. Unless otherwise mentioned, all reagents and solvents are commercially available and were used without further purification.
4.1.2. General method for preparation of chalcones 3a-b. Chalcone derivatives 3a-b were synthesized by stirring p-methoxyacetophenone (1, 3 g, 20 mmol) with the corresponding aldehydes (2a-b, 20 mmol) at room temperature for 4 h in aqueous–ethanolic (1
:
1) potassium hydroxide (40% solution). The precipitate was filtered by suction, washed with water, and finally air-dried for several hours. Chalcones 3a-b were identified by comparing their measured melting points (105–107 °C and 100–102 °C) with the reported values (107–108 °C (ref. 39) and 102–104 °C (ref. 40)) for 3a and 3b, respectively. Yields = 88 and 85% for 3a and 3b, respectively.
4.1.3. General method for synthesis of 3,5-diaryl-4,5-dihydro-1H-pyrazole-1-carbothioamide derivatives (5a-b). To a stirred ethanolic solution (20 ml) of chalcones 3a-b (10 mmol) and thiosemicarbazide (4, 1.1 g, 12 mmol), sodium hydroxide (0.8 g, 20 mmol) was added. Then, the reaction mixture was heated at reflux for 3 h. After that, the precipitated solid was filtered while hot washed with 70% aqueous ethanol (3 × 5 ml), and finally dried at 90 °C. This afforded pyrazoline-1-carbothioamide derivatives (5a-b).41,42 Compounds 5a-b were used in the next step with no further purification.
4.1.4. General method for synthesis of 2-(4,5-dihydro-1H-pyrazol-1-yl)thiazol-4(5H)-one derivatives (7a-b). The corresponding pyrazoline-1-carbothioamide derivative (5, 7 mmol),bromoacetic acid (6, 1.07 g, 7.7 mmol, 1.1 equiv.) and anhydrous sodium acetate (1.1 g, 14 mmol, 2 equiv.) were refluxed in glacial acetic acid (8 ml) for 5 h. At the end of this period, the precipitated solid was filtered while hot, washed with minimal volume of glacial acetic acid, then with plenty of water and finally with petroleum ether. Drying at 90 °C afforded compounds (7a-b), which were used in the next step without further purification.2-(5-(4-Fluorophenyl)-3-(4-methoxyphenyl)-4,5-dihydro-1H-pyrazol-1-yl)thiazol-4(5H)-one 7a; white crystals, yield = 75%, m.p. = 209–210 °C (reported m.p. = 210–212 °C).41
2-(3,5-Bis(4-methoxyphenyl)-4,5-dihydro-1H-pyrazol-1-yl)thiazol-4(5H)-one 7b; white crystals, yield = 75%, m.p. = 216–218 °C (reported m.p. = 220–222 °C).41
4.1.5. General preparation of N-alkyl isatin derivatives (11a–c). To a stirred DMF solution (6 ml) of isatin (8a, 1 g, 6.8 mmol) was portionwise added a DMF solution (2 ml) of the corresponding alkyl halide (7.5 mmol, 1.1 equiv.). Then anhydrous K2CO3 (1.9 g, 13.6 mmol, 2 equiv.) and KI (cat.) were added in one portion. The resulting slurry was stirred at 80 °C for 6 h then cooled to room temperature. The reaction mixture was poured portionwise into a beaker containing vigorously stirred cold water/ice mixture (25 ml). The precipitate was allowed to settle down, filtered by suction, air-dried, and then recrystallized from 95% ethanol to afford the desired N-alkyl isatin derivatives (11a–c).431-Ethylindoline-2,3-dione 11a; yellow powder, yield = 84%, m.p. = 85–87 °C.
1-Allylindoline-2,3-dione 11b; yellow powder, yield = 80%, m.p. = 81–83 °C.
1-Isobutylindoline-2,3-dione 11c; orange powder, yield = 78%, m.p. = 71–73 °C.
4.1.6. General method for synthesis of 2-(4,5-dihydro-1H-pyrazol-1-yl)-5-(isatin-3-ylidene)thiazol-4(5H)-one derivatives (9a–h, 10a–h, 12a–c and 13a–c). The corresponding 2-(4,5-dihydro-1H-pyrazol-1-yl)thiazol-4(5H)-one derivative (7a-b, 0.5 mmol) as well as the appropriate isatin (8a–h) or N-alkylisatin (11a-c) derivative (0.55 mmol, 1.1 equiv.) and anhydrous sodium acetate (0.12 g, 1.5 mmol, 3 equiv.) were allowed to reflux for 3 h in glacial acetic acid (4 ml). The precipitated solid was subjected to hot filtration, washing with glacial acetic acid, then with plenty of water, and finally with petroleum ether. Recrystallization from ethanol/DMF (4
:
1) followed by drying at 90 °C afforded the desired compounds (9a–h, 10a–h, 12a–c and 13a–c) which were sufficiently pure for analysis as illustrated below.
4.1.6.1. 2-(5-(4-Fluorophenyl)-3-(4-methoxyphenyl)-4,5-dihydro-1H-pyrazol-1-yl)-5-(2-oxoindolin-3-ylidene)thiazol-4(5H)-one (9a). Yellow powder (yield 85%), m.p. >300 °C, 1H NMR (400 MHz, DMSO-d6), δ 11.14 (s, 1H, –NH– ‘isatin’), 8.90 (d, J = 7.9 Hz, 1H, Ar-H), 7.90–7.82 (m, 2H, Ar-H), 7.35 (ddd, J = 13.9, 8.2, 4.4 Hz, 3H, Ar-H), 7.22 (td, J = 9.4, 8.7, 2.9 Hz, 2H, Ar-H), 7.12–6.99 (m, 3H, Ar-H), 6.91 (d, J = 7.7 Hz, 1H, Ar-H), 5.95 (dd, JXA = 11.0, JXB = 4.0 Hz, 1H, pyrazoline-HX (17-CH–)), 4.13 (dd, JAX = 18.2, JAB = 11.0 Hz, 1H, pyrazoline-HA (16-CHA–)), 3.84 (s, 3H, –OCH3), 3.51 (dd, JBA = 18.2, JBX = 4.0 Hz, 1H, pyrazoline-HB (16-CHB–)). 13C NMR (101 MHz, DMSO-d6) δ 179.37 (–C
O), 169.48 (–C
O), 162.68 (–N–C
N), 162.41 (ArC-F), 160.94 (ArC-OCH3), 136.81, 132.15, 130.02, 128.61, 128.48, 126.38, 122.37, 122.29, 122.25, 120.85, 120.21, 120.15, 116.36, 116.14, 116.05, 115.04, 114.99, 110.59, 106.06, 63.49 (–CH–), 55.99 (Ar-OCH3), 43.84 (–CH2–). Elemental analysis for C27H19FN4O3S: calcd C, 65.05; H, 3.84; N, 11.24, found C, 64.88; H, 3.86; N, 11.31.
4.1.6.2. 5-(5-Fluoro-2-oxoindolin-3-ylidene)-2-(5-(4-fluorophenyl)-3-(4-methoxyphenyl)-4,5-dihydro-1H-pyrazol-1-yl)thiazol-4(5H)-one (9b). Yellow powder (yield 83%), m.p. >300 °C, 1H NMR (400 MHz, TFA-d), δ 8.66 (d, J = 6.6 Hz, 1H, Ar-H), 8.19–8.10 (m, 2H, Ar-H), 7.62–7.52 (m, 2H, Ar-H), 7.45–7.35 (m, 2H, Ar-H), 7.39–7.29 (m, 2H, Ar-H), 7.33–7.26 (m, 2H, Ar-H), 6.32 (dd, JXA = 10.0, JXB = 3.6 Hz, 1H, pyrazoline-HX (17-CH–)), 4.57 (dd, JAX = 18.4, JAB = 10.1 Hz, 1H, pyrazoline-HA (16-CHA–), 4.17 (s, 3H, –OCH3), 3.87 (dd, JBA = 18.3, JBX 3.6 Hz, 1H, pyrazoline-HB (16-CHB–)). 13C NMR (101 MHz, TFA-d), δ 170.21 (–C
O), 170.02 (–C
O), 166.11 (–N–C
N), 164.29 (ArC-F), 158.68 (ArC-OCH3), 139.24 (ArC-F), 130.90, 129.97, 127.47, 121.85, 120.39, 118.61, 117.26, 116.39, 115.79, 114.88, 112.97, 112.72, 110.15, 66.57 (–CH–), 54.96 (Ar-OCH3), 45.19 (–CH2–). Elemental analysis for C27H18F2N4O3S: calcd C, 62.78; H, 3.51; N, 10.85, found C, 62.96; H, 3.49; N, 10.91.
4.1.6.3. 5-(6-Fluoro-2-oxoindolin-3-ylidene)-2-(5-(4-fluorophenyl)-3-(4-methoxyphenyl)-4,5-dihydro-1H-pyrazol-1-yl)thiazol-4(5H)-one (9c). Yellow powder (yield 88%), m.p. >300 °C, 1H NMR (400 MHz, TFA-d), δ 8.80 (s, 1H, Ar-H), 8.20 (m, 2H, Ar-H), 7.60 (m, 2H, Ar-H), 7.50 (t, 8.9 Hz, 1H, Ar-H), 7.47–7.29 (m, 4H, Ar-H), 6.35 (dd, J = 10.1, 3.6 Hz, 1H, Ar-H), 6.35 (dd, JXA = 10.1, JXB = 3.6 Hz, 1H, pyrazoline-HX (17-CH–)), 4.61 (dd, JAX = 18.4, JAB = 10.1 Hz, 1H, pyrazoline-HA (16-CHA–)), 4.20 (s, 3H, –OCH3), 3.91 (dd, JBA = 18.3, JBX 3.6 Hz, 1H, pyrazoline-HB (16-CHB–)). 13C NMR (101 MHz, TFA-d), δ 170.19 (–C
O), 169.50 (–C
O), 165.97 (–N–C
N), 165.43 (ArC-F), 164.32 (ArC-OCH3), 148.92 (ArC-F), 130.93, 129.99, 127.42, 125.55, 122.17, 121.57, 120.42, 118.66, 117.29, 115.84, 114.91, 113.03, 110.22, 66.59 (–CH–), 54.99 (Ar-OCH3), 45.23 (–CH2–). Elemental analysis for C27H18F2N4O3S: calcd C, 62.78; H, 3.51; N, 10.85; found C, 62.93; H, 3.50; N, 10.89.
4.1.6.4. 5-(6-Chloro-2-oxoindolin-3-ylidene)-2-(5-(4-fluorophenyl)-3-(4-methoxyphenyl)-4,5-dihydro-1H-pyrazol-1-yl)thiazol-4(5H)-one (9d). Yellow powder (yield 85%), m.p. >300 °C, 1H NMR (400 MHz, TFA-d), δ 8.89 (s, 1H, Ar-H), 8.14 (d, J = 8.6 Hz, 2H, Ar-H), 7.64 (dd, J = 8.4, 1.7 Hz, 1H, Ar-H), 7.57 (dd, J = 8.6, 4.6 Hz, 2H, Ar-H), 7.32 (dt, J = 24.3, 8.4 Hz, 5H, Ar-H), 6.33 (dd, JXA = 10.1, JXB = 3.5 Hz, 1H, pyrazoline-HX (17-CH–)), 4.57 (dd, JAX = 18.3, JAB = 9.9 Hz, 1H, pyrazoline-HA (16-CHA–)), 4.17 (s, 3H, –OCH3), 3.87 (dd, JBA = 18.3, JBX 3.3 Hz, 1H, pyrazoline-HB (16-CHB–)). 13C NMR (101 MHz, TFA-d) δ 170.22 (–C
O), 169.82 (–C
O), 166.03 (–N–C
N), 165.39 (ArC-F), 164.31 (ArC-OCH3), 141.34 (ArC-Cl), 134.98, 130.90, 130.62, 130.00, 129.22, 127.37, 127.00, 120.37, 120.25, 117.27, 115.87, 114.88, 113.05, 110.29, 66.57 (–CH–), 54.96 (Ar-OCH3), 45.19 (–CH2–). Elemental analysis for C27H18ClFN4O3S: calcd C, 60.85; H, 3.40; N, 10.51, found C, 61.04; H, 3.43; N, 10.46.
4.1.6.5. 5-(6-Chloro-2-oxoindolin-3-ylidene)-2-(5-(4-fluorophenyl)-3-(4-methoxyphenyl)-4,5-dihydro-1H-pyrazol-1-yl)thiazol-4(5H)-one (9e). Yellow powder (yield 80%), m.p. >300 °C, 1H NMR (400 MHz, TFA-d), δ 8.85 (d, J = 6.0 Hz, 1H, Ar-H), 8.17 (d, J = 8.2 Hz, 2H, Ar-H), 7.68 (d, J = 7.9 Hz, 1H, Ar-H), 7.59 (dd, J = 8.7, 4.4 Hz, 2H, Ar-H), 7.35 (dd, J = 18.5, 8.0 Hz, 5H, Ar-H), 6.37–6.30 (m, 1H, pyrazoline-HX (17-CH–)), 4.59 (dd, JAX = 17.5, JAB = 7.7 Hz, 1H, pyrazoline-HA (16-CHA–)), 4.19 (s, 3H, –OCH3), 3.89 (d, J = 17.9 Hz, 1H, pyrazoline-HB (16-CHB–)). 13C NMR (101 MHz, TFA-d) δ 170.27 (–C
O), 170.21 (–C
O), 165.99 (–N–C
N), 164.33 (ArC-F), 162.92 (ArC-OCH3), 140.14 (ArC-Cl), 134.79, 130.93, 129.98, 128.75, 128.10, 127.40, 125.40, 120.69, 120.39, 120.39, 117.06, 115.11, 114.90, 66.60 (–CH–), 54.97 (Ar-OCH3), 45.21 (–CH2–). Elemental analysis for C27H18ClFN4O3S: calcd C, 60.85; H, 3.40; N, 10.51, found 61.03; H, 3.41; N, 10.47.
4.1.6.6. 2-(5-(4-Fluorophenyl)-3-(4-methoxyphenyl)-4,5-dihydro-1H-pyrazol-1-yl)-5-(5-methoxy-2-oxoindolin-3-ylidene)thiazol-4(5H)-one (9f). Red powder (yield 78%), m.p. >300 °C, 1H NMR (400 MHz, TFA-d), δ 8.69 (d, J = 2.5 Hz, 1H, Ar-H), 8.13 (d, J = 8.9 Hz, 2H, Ar-H), 7.61–7.52 (m, 2H, Ar-H), 7.42–7.33 (m, 3H, Ar-H), 7.33–7.26 (m, 4H, Ar-H), 6.30 (dd, JXA = 10.1, JXB = 3.7 Hz, 1H, pyrazoline-HX (17-CH–),4.56 (dd, JAX = 18.4, JAB = 10.1 Hz, 1H, pyrazoline-HA (16-CHA–), 4.16 (s, 6H, (–OCH3)2, 3.86 (dd, JBA = 18.4, JBX 3.7 Hz, 1H, pyrazoline-HB (16-CHB–)). 13C NMR (101 MHz, TFA-d) δ 170.09 (–C
O), 169.95 (–C
O), 166.17 (–N–C
N), 165.39 (ArC-OCH3), 164.24 (ArC-F), 162.89 (ArC-OCH3), 155.30, 138.21, 130.87, 127.47, 126.23, 121.75, 120.40, 118.58, 117.26, 116.33, 115.77, 114.86, 112.95, 110.14, 66.52, 56.21 (–CH–), 54.95 (Ar-OCH3), 45.16 (–CH2–). Elemental analysis for C28H21FN4O4S: calcd C, 63.63; H, 4.00; N, 10.60, found 63.81; H, 3.98; N, 10.66.
4.1.6.7. 2-(5-(4-Fluorophenyl)-3-(4-methoxyphenyl)-4,5-dihydro-1H-pyrazol-1-yl)-5-(5-methyl-2-oxoindolin-3-ylidene)thiazol-4(5H)-one (9g). Yellow powder (yield 75%), m.p. >300 °C, 1H NMR (400 MHz, TFA-d), δ 8.82 (s, 1H, Ar-H), 8.30–8.20 (m, 2H, Ar-H), 7.73–7.65 (m, 2H, Ar-H), 7.63 (d, J = 7.9 Hz, 1H, Ar-H), 7.55–7.47 (m, 2H, Ar-H), 7.46–7.35 (m, 2H, Ar-H), 7.29 (t, J = 6.1 Hz, 1H, Ar-H), 6.41 (dd, JXA = 10.2, JXB = 3.8 Hz, 1H, pyrazoline-HX (17-CH–), 4.67 (dd, JAX = 18.3, JAB = 10.1 Hz, 1H, pyrazoline-HA (16-CHA–), 4.26 (s, 3H, –OCH3), 3.97 (dd, JBA = 18.4, JBX 3.7 Hz, 1H, pyrazoline-HB (16-CHB–)) 2.64 (s, 3H, –CH3). 13C NMR (101 MHz, TFA-d) δ 170.21 (–C
O), 169.93 (–C
O), 166.54 (–N–C
N), 164.29 (ArC-F), 163.13 (ArC-OCH3), 141.00, 136.65, 135.58, 130.93, 130.22, 127.60, 124.47, 120.57, 119.49, 118.70, 115.89, 114.97, 113.08, 111.79, 110.26, 66.51 (Ar-OCH3), 55.06 (–CH–), 45.27 (–CH2–), 19.39 (–CH3). Elemental analysis for C28H21FN4O3S: calcd C, 65.61; H, 4.13; N, 10.93, found C, 65.50; H, 4.16; N, 11.00.
4.1.6.8. 5-(5,7-Dimethyl-2-oxoindolin-3-ylidene)-2-(5-(4-fluorophenyl)-3-(4-methoxyphenyl)-4,5-dihydro-1H-pyrazol-1-yl)thiazol-4(5H)-one (9h). Red powder (yield 84%), m.p. >300 °C, 1H NMR (400 MHz, TFA-d), δ 8.52 (d, J = 1.5 Hz, 1H, Ar-H), 8.13–8.05 (m, 2H, Ar-H), 7.58–7.49 (m, 2H, Ar-H), 7.39–7.26 (m, 5H, Ar-H), 7.29–7.21 (m, 2H, Ar-H), 6.25 (dd, JXA = 10.1, JXB = 3.6 Hz, 1H, pyrazoline-HX (17-CH–), 4.51 (dd, JAX = 18.3, JAB = 10.1 Hz, 1H, pyrazoline-HA (16-CHA–), 4.12 (s, 3H, –OCH3), 3.81 (dd, JBA = 18.4, JBX 3.7 Hz, 1H, pyrazoline-HB (16-CHB–)) 2.46 (s, 3H, –CH3), 2.39 (s, 3H, –CH3). 13C NMR (101 MHz, TFA-d) δ 170.28 (–C
O), 169.69 (–C
O), 166.48 (–N–C
N), 165.34 (ArC-F), 164.13 (ArC-OCH3), 163.02, 139.26, 138.20, 135.29, 130.76, 130.05, 127.83, 127.43, 124.05, 121.37, 120.44, 116.96, 115.74, 114.81, 112.93, 110.11, 66.32 (Ar-OCH3), 54.91 (–CH–), 45.11 (–CH2–), 19.19 (Ar-CH3), 14.03 (Ar-CH3). Elemental analysis for C29H23FN4O3S: calcd C, 66.15; H, 4.40; N, 10.64, found C, 65.96; H, 4.41; N, 10.58.
4.1.6.9. 2-(3,5-Bis(4-methoxyphenyl)-4,5-dihydro-1H-pyrazol-1-yl)-5-(2-oxoindolin-3-ylidene)thiazol-4(5H)-one (10a). Yellow powder (yield 82%), m.p. >300 °C, 1H NMR (400 MHz, TFA-d), δ 9.00 (d, J = 8.0 Hz, 1H, Ar-H), 8.24 (d, J = 8.0 Hz, 2H, Ar-H), 7.80 (t, J = 7.6 Hz, 1H, Ar-H), 7.70 (d, J = 8.0 Hz, 2H, Ar-H), 7.55–7.34 (m, 6H, Ar-H), 6.37 (dd, JXA = 10.2, JXB = 4.0 Hz, 1H, pyrazoline-HX (17-CH–), 4.65 (dd, JAX = 17.7, JAB = 9.5 Hz, 1H, pyrazoline-HA (16-CHA–), 4.26 (s, 3H, –OCH3), 4.24 (s, 3H, –OCH3), 3.99 (dd, JBA = 18.4, JBX 4.0 Hz, 1H, pyrazoline-HB (16-CHB–)). 13C NMR (101 MHz, TFA-d) δ 170.20 (–C
O), 170.17 (–C
O), 166.42 (–N–C
N), 164.27 (ArC-OCH3), 160.17 (ArC-OCH3), 143.16, 135.87, 130.94, 129.88, 127.63, 127.31, 124.86, 120.64, 118.71, 115.90, 114.96, 113.08, 110.27, 66.88 (–CH–), 55.23 (Ar-OCH3), 55.07 (Ar-OCH3), 45.24 (–CH2–). Elemental analysis for C28H22N4O4S: calcd C, 65.87; H, 4.34; N, 10.97, found C, 65.62; H, 4.35; N, 11.05.
4.1.6.10. 2-(3,5-Bis(4-methoxyphenyl)-4,5-dihydro-1H-pyrazol-1-yl)-5-(5-fluoro-2-oxoindolin-3-ylidene)thiazol-4(5H)-one (10b). Red powder (yield 87%), m.p. >300 °C, 1H NMR (400 MHz, TFA-d), δ 8.74 (d, J = 6.6 Hz, 1H, Ar-H), 8.26–8.19 (m, 2H, Ar-H), 7.67–7.60 (m, 2H, Ar-H), 7.50–7.36 (m, 6H, Ar-H), 6.36 (dd, JXA = 10.0, JXB = 3.8 Hz, 1H, pyrazoline-HX (17-CH–), 4.63 (dd, JAX = 18.4, JAB = 10.1 Hz, 1H, pyrazoline-HA (16-CHA–), 4.25 (s, 3H, –OCH3), 4.21 (s, 3H, –OCH3), 3.97 (dd, JBA = 18.4, JBX 3.9 Hz, 1H, pyrazoline-HB (16-CHB–)). 13C NMR (101 MHz, TFA-d) δ 170.42 (–C
O), 170.09 (–C
O), 166.06 (–N–C
N), 164.31 (ArC-F), 162.56 (ArC-OCH3), 160.14 (ArC-OCH3), 158.76, 139.26, 130.97, 127.53, 127.24, 122.10, 120.55, 118.67, 116.73, 115.92, 115.85, 114.94, 113.04, 110.22, 66.97 (–CH–), 55.19 (Ar-OCH3), 55.04 (Ar-OCH3), 45.22 (–CH2–). Elemental analysis for C28H21FN4O4S: calcd C, 63.63; H, 4.00; N, 10.60, found 63.73; H, 3.98; N, 10.65.
4.1.6.11. 2-(3,5-Bis(4-methoxyphenyl)-4,5-dihydro-1H-pyrazol-1-yl)-5-(7-fluoro-2-oxoindolin-3-ylidene)thiazol-4(5H)-one (10c). Red powder (yield 76%), m.p. >300 °C, 1H NMR (400 MHz, TFA-d), δ 8.85 (t, J = 7.2 Hz, 1H, Ar-H), 8.31–8.22 (m, 2H, Ar-H), 7.69–7.40 (m, 8H, Ar-H), 6.40 (dd, JXA = 10.1, JXB = 2.9 Hz, 1H, pyrazoline-HX (17-CH–), 4.67 63 (dd, JAX = 10.1, JAB = 18.4 Hz, 1H, pyrazoline-HA (16-CHA–), 4.28 (s, 3H, –OCH3), 4.25 (s, 3H, –OCH3), 4.0 (dd, JBA = 18.4, JBX 3.0 Hz, 1H, pyrazoline-HB (16-CHB–)). 13C NMR (101 MHz, TFA-d) δ 170.41 (–C
O), 169.57 (–C
O), 165.95 (–N–C
N), 164.34 (ArC-F), 160.18 (ArC-OCH3), 146.55, 131.01, 127.30, 125.46, 122.17, 121.63, 120.59, 118.71, 115.89, 114.97, 113.08, 110.26, 67.00 (–CH–), 55.23 (Ar-OCH3), 55.08 (Ar-OCH3), 45.26 (–CH2–). Elemental analysis for C28H21FN4O4S: calcd C, 63.63; H, 4.00; N, 10.60, found C, 63.81; H, 4.01; N, 10.63.
4.1.6.12. 2-(3,5-Bis(4-methoxyphenyl)-4,5-dihydro-1H-pyrazol-1-yl)-5-(5-chloro-2-oxoindolin-3-ylidene)thiazol-4(5H)-one (10d). Orange powder (yield 85%), m.p. >300 °C, 1H NMR (400 MHz, TFA-d), δ 8.99 (s, 1H, Ar-H), 8.37–8.22 (m, 2H, Ar-H), 7.78–7.65 (m, 3H, Ar-H), 7.49–7.35 (m, 5H, Ar-H), 6.41 (dd, JXA = 10.1, JXB = 3.9 Hz, 1H, pyrazoline-HX (17-CH–), 4.67 (dd, JAX = 17.6, JAB = 9.4 Hz, 1H, pyrazoline-HA (16-CHA–) 4.28 (s, 3H, –OCH3), 4.25 (s, 3H, –OCH3), 4.01 (dd, JBA = 18.3, JBX 4.2 Hz, 1H, yrazoline-HB (16-CHB–)). 13C NMR (101 MHz, TFA-d) δ 170.48 (–C
O), 169.88 (–C
O), 165.97 (–N–C
N), 164.38 (ArC-OCH3), 160.20 (ArC-OCH3), 141.41, 134.99 (ArC-Cl), 131.01, 130.66, 129.27, 127.59, 127.29, 120.56, 118.72, 115.90, 114.99, 113.09, 110.27, 67.02 (–CH–), 55.25 (Ar-OCH3), 55.08 (Ar-OCH3), 45.25 (–CH2–). Elemental analysis for C28H21ClN4O4S: calcd C, 61.71; H, 3.88; N, 10.28, found C, 61.89; H, 4.00; N, 10.32.
4.1.6.13. 2-(3,5-Bis(4-methoxyphenyl)-4,5-dihydro-1H-pyrazol-1-yl)-5-(7-chloro-2-oxoindolin-3-ylidene)thiazol-4(5H)-one (10e). Orange powder (yield 81%), m.p. >300 °C, 1H NMR (400 MHz, TFA-d), δ 8.95 (d, J = 7.1 Hz, 1H, Ar-H), 8.30 (d, J = 8.0 Hz, 1H, Ar-H), 7.81–7.74 (m, 1H, Ar-H), 7.72–7.64 (m, 2H, Ar-H), 7.51–7.42 (m, 2H, Ar-H), 7.46–7.38 (m, 4H, Ar-H), 6.41 (dd, JXA = 10.0, JXB = 3.8 Hz, 1H, pyrazoline-HX (17-CH–), 4.67 (dd, JAX = 18.4, JAB = 10.0 Hz, 1H, pyrazoline-HA (16-CHA–), 4.29 (s, 3H, –OCH3), 4.26 (s, 3H, –OCH3), 4.01 (dd, JBA = 18.4, JBX 3.9 Hz, 1H, pyrazoline-HB (16-CHB–). 13C NMR (101 MHz, TFA-d) δ 170.43 (–C
O), 169.68 (–C
O), 165.89 (–N–C
N), 164.37 (ArC-OCH3), 160.19 (ArC-OCH3), 140.22, 134.78 (ArC-Cl), 131.02, 127.57, 127.31, 125.46, 120.59, 118.71, 117.36, 115.90, 114.98, 113.08, 110.27, 67.02 (–CH–), 55.24 (Ar-OCH3), 55.09 (Ar-OCH3), 45.27 (–CH2–). Elemental analysis for C28H21ClN4O4S: calcd C, 61.71; H, 3.88; N, 10.28, found, 61.83; H, 3.87; N, 10.24.
4.1.6.14. 2-(3,5-Bis(4-methoxyphenyl)-4,5-dihydro-1H-pyrazol-1-yl)-5-(5-methoxy-2-oxoindolin-3-ylidene)thiazol-4(5H)-one (10f). Red powder (yield 88%), m.p. >300 °C, 1H NMR (400 MHz, TFA-d), δ 8.84 (d, J = 2.6 Hz, 1H, Ar-H), 8.33–8.26 (m, 2H, Ar-H), 7.75–7.68 (m, 2H, Ar-H), 7.57–7.50 (m, 1H, Ar-H), 7.49–7.41 (m, 5H, Ar-H), 6.42 41 (dd, JXA = 10.0, JXB = 3.9 Hz, 1H, pyrazoline-HX (17-CH–), 4.70 (dd, JAX = 18.4, JAB = 10.1 Hz, 1H, pyrazoline-HA (16-CHA–), (dd, J = 18.4, 10.1 Hz, 1H), 4.32 (s, 3H, –OCH3), 4.31 (s, 3H, –OCH3), 4.28 (s, 3H, –OCH3), 4.04 (dd, JBA = 18.4, JBX 3.9 Hz, 1H, pyrazoline-HB (16-CHB–)). 13C NMR (101 MHz, TFA-d) δ 170.38 (–C
O), 170.12 (–C
O), 166.20 (–N–C
N), 164.35 (ArC-OCH3), 160.22 (ArC-OCH3), 155.45 (ArC-OCH3), 138.31, 131.01, 127.59, 127.34, 121.87, 120.65, 118.74, 116.41, 116.01, 115.93, 115.01, 113.11, 112.89, 110.30, 67.01 (–CH–), 56.36 (Ar-OCH3), 55.26 (Ar-OCH3), 55.11 (Ar-OCH3), 45.26 (–CH2–). Elemental analysis for C29H24N4O5S: calcd C, 64.43; H, 4.48; N, 10.36, found C, 64.27; H, 4.51; N, 10.39.
4.1.6.15. 2-(3,5-Bis(4-methoxyphenyl)-4,5-dihydro-1H-pyrazol-1-yl)-5-(5-methyl-2-oxoindolin-3-ylidene)thiazol-4(5H)-one (10g). Red powder (yield 80%), m.p. >300 °C, 1H NMR (400 MHz, TFA-d), δ 8.87 (s, 1H, Ar-H), 8.28 (dd, J = 4.3, 2.3 Hz, 2H, Ar-H), 7.79–7.72 (m, 2H, Ar-H), 7.72–7.64 (m, 1H, Ar-H), 7.55–7.38 (m, 5H, Ar-H), 6.43 (dd, JXA = 9.9, JXB = 3.7 Hz, 1H, pyrazoline-HX (17-CH–), 4.70 (dd, JAX = 18.4, JAB = 10.0 Hz, 1H, pyrazoline-HA (16-CHA–), 4.30 (s, 3H, –OCH3), 4.30 (s, 3H, –OCH3), 4.04 (dd, JBA = 18.4, JBX 3.7 Hz, 1H, pyrazoline-HB (16-CHB–)) 2.69 (s, 3H, –CH3). 13C NMR (101 MHz, TFA-d) δ 170.29 (–C
O), 170.12 (–C
O), 166.44 (–N–C
N), 164.30 (ArC-OCH3), 162.92 (ArC-OCH3), 160.23, 140.98, 136.62, 130.97, 130.26, 127.73, 127.36, 124.61, 120.69, 118.77, 115.95, 115.01, 113.14, 111.81, 110.33, 66.89 (–CH–), 55.29 (Ar-OCH3), 55.10 (Ar-OCH3), 45.26 (–CH2–), 19.46 (Ar-CH3). Elemental analysis for C29H24N4O4S: calcd C, 66.40; H, 4.61; N, 10.68 found C, 66.58; H, 4.59; N, 10.73.
4.1.6.16. 2-(3,5-Bis(4-methoxyphenyl)-4,5-dihydro-1H-pyrazol-1-yl)-5-(5,7-dimethyl-2-oxoindolin-3-ylidene)thiazol-4(5H)-one (10h). Red powder (yield 84%), m.p. >300 °C, 1H NMR (400 MHz, TFA-d), δ 8.93 (s, 1H, Ar-H), 8.49 (d, J = 5.3 Hz, 2H, Ar-H), Ar-H, 8.04–7.99 (m, 2H, Ar-H), 7.75 (s, 3H, Ar-H), 7.73 (d, J = 5.1 Hz, 2H, Ar-H), 7.67–7.60 (m, 2H, Ar-H), 6.68 (s, 1H, pyrazoline-H), 4.96–4.85 (m, 1H, pyrazoline-H), 4.61–4.51 (m, 6H, (–OCH3)2), 4.31–4.26 (m, 1H, pyrazoline-H), 2.90 (s, 3H, –CH3), 2.80 (s, 3H, –CH3). 13C NMR (101 MHz, TFA-d) δ 173.88 (–C
O), 173.64 (–C
O), 170.25 (–N–C
N), 164.60 (ArC-OCH3), 147.75 (ArC-OCH3), 138.53, 131.21, 128.23, 127.94, 127.63, 119.07, 116.24, 115.25, 113.44, 110.61, 67.13 (–CH–), 55.59 (Ar-OCH3), 55.41 (Ar-OCH3), 45.54 (–CH2–), 19.78 (Ar-CH3), 14.63 (Ar-CH3). Elemental analysis for C30H26N4O4S: calcd C, 66.90; H, 4.87; N, 10.40, found C, 67.11; H, 4.85; N, 10.46.
4.1.6.17. 5-(1-Ethyl-2-oxoindolin-3-ylidene)-2-(5-(4-fluorophenyl)-3-(4-methoxyphenyl)-4,5-dihydro-1H-pyrazol-1-yl)thiazol-4(5H)-one (12a). Orange powder (yield 73%), m.p. >300 °C, 1H NMR (400 MHz, TFA-d), δ 8.84 (d, J = 7.9 Hz, 1H, Ar-H), 8.11–8.05 (m, 2H, Ar-H), 7.66 (t, J = 7.6 Hz, 1H, Ar-H), 7.50 (dd, J = 8.6, 4.7 Hz, 2H, Ar-H), 7.33 (t, J = 7.8 Hz, 1H, Ar-H), 7.31–7.20 (m, 4H, Ar-H), 7.16 (d, J = 7.9 Hz, 1H, Ar-H), 6.21 (dd, JXA = 10.1, JXB = 3.8 Hz, 1H, pyrazoline-HX (17-CH–), 4.51 (dd, JAX = 18.3, JAB = 10.1 Hz, 1H, pyrazoline-HA (16-CHA–), 4.10 (s, 3H, –OCH3), 4.18–4.16 (m, 2H, –CH2), 3.79 (dd, JBA = 18.4, JBX 3.7 Hz, 1H, pyrazoline-HB (16-CHB–), 1.59 (t, J = 7.0 Hz, 3H, –CH3). 13C NMR (101 MHz, TFA-d) δ 168.77 (–C
O), 167.91 (–C
O), 165.99 (–N–C
N), 164.00 (ArC-OCH3), 162.00 (ArC-F), 144.10, 135.14, 133.21 130.17, 129.19, 127.31, 127.09, 124.11, 120.17, 119.10, 115.10, 114.16, 109.24, 66.13 (–CH–), 55.20 (Ar-OCH3), 54.27 (Ar-OCH3), 45.03 (–CH2–), 35.23 (–CH2–), 10.13 (–CH3). Elemental analysis for C29H23FN4O3S: calcd C, 66.15; H, 4.40; N, 10.64, found C, 65.93; H, 4.41; N, 10.71.
4.1.6.18. 5-(1-Allyl-2-oxoindolin-3-ylidene)-2-(5-(4-fluorophenyl)-3-(4-methoxyphenyl)-4,5-dihydro-1H-pyrazol-1-yl)thiazol-4(5H)-one (12b). Orange powder (yield 77%), m.p. >300 °C, 1H NMR (400 MHz, TFA-d), δ 8.87 (dd, J = 8.0, 1.1 Hz, 1H, Ar-H), 8.14–8.05 (m, 2H, Ar-H), 7.66 (td, J = 7.8, 1.1 Hz, 1H, Ar-H), 7.55–7.46 (m, 2H, Ar-H), 7.35 (td, J = 7.8, 0.9 Hz, 1H), 7.33–7.23 (m, 4H, Ar-H), 7.17 (d, J = 7.9 Hz, 1H, Ar-H), 6.22 (dd, JXA = 10.1, JXB = 3.7 Hz, 1H, pyrazoline-HX (17-CH–), 6.01 (ddt, J = 17.1, 10.4, 5.2 Hz, 1H, –CH
), 5.50–5.38 (m, 2H,
CH2), 4.64 (dt, J = 5.4, 1.6 Hz, 2H, –CH2–), 4.50 (dd, JAX = 18.3, JAB = 10.1 Hz, 1H, pyrazoline-HA (16-CHA–), 4.11 (s, 3H), 3.80 (dd, JBA = 18.4, JBX 3.7 Hz, 1H, pyrazoline-HB (16-CHB–). 13C NMR (101 MHz, TFA-d) δ 169.77 (–C
O), 167.96 (–C
O), 166.46 (–N–C
N), 164.13 (ArC-OCH3), 162.93 (ArC-F), 144.70, 135.42, 133.62, 130.81, 129.74, 128.44, 127.45, 124.82, 120.41, 118.98, 118.05, 116.94, 115.71, 114.81, 112.89, 110.62 (
CH–), 110.08 (
CH2–), 66.37 (–CH–), 54.90 (Ar-OCH3), 45.13 (–CH2–), 43.04 (–CH2–). Elemental analysis for C30H23FN4O3S: calcd C, 66.90; H, 4.30; N, 10.40, found C, 67.13; H, 4.31; N, 10.36.
4.1.6.19. 2-(5-(4-Fluorophenyl)-3-(4-methoxyphenyl)-4,5-dihydro-1H-pyrazol-1-yl)-5-(1-isobutyl-2-oxoindolin-3-ylidene)thiazol-4(5H)-one (12c). Yellow powder (yield 73%), m.p. >300 °C, 1H NMR (400 MHz, TFA-d), δ 8.87 (d, J = 7.9 Hz, 1H, Ar-H), 8.15–8.07 (m, 2H, Ar-H), 7.68 (t, J = 7.6 Hz, 1H, Ar-H), 7.52 (dd, J = 8.6, 4.7 Hz, 2H, Ar-H), 7.35 (t, J = 7.8 Hz, 1H, Ar-H), 7.33–7.22 (m, 4H, Ar-H), 7.18 (d, J = 7.9 Hz, 1H, Ar-H), 6.23 (dd, JXA = 10.1, JXB = 3.7 Hz, 1H, pyrazoline-HX (17-CH–), 4.50 (dd, JAX = 18.3, JAB = 10.1 Hz, 1H, pyrazoline-HA (16-CHA–), 4.13 (s, 3H, –OCH3), 3.84 (d, J = 7.7 Hz, 2H, –CH2–), 3.78 (dd, JBA = 18.4, JBX 3.7 Hz, 1H, pyrazoline-HB (16-CHB–), 2.42–2.30 (m, 1H, (–CH–), 1.17 (dd, J = 6.7, 1.5 Hz, 6H, (–CH3)2). 13C NMR (101 MHz, TFA-d) δ 169.73 (–C
O), 168.21 (–C
O), 166.56 (–N–C
N), 164.13 (ArC-OCH3), 163.03 (ArC-F), 145.26, 135.39, 133.77, 130.82, 129.98, 129.79, 127.45, 124.74, 120.43, 118.98, 117.17, 115.78, 114.82, 112.97, 110.41, 66.35 (–CH2–), 54.91 (Ar-OCH3), 48.51 (–CH2–), 45.13 (–CH2–), 27.00 (–CH–), 18.34 (–CH3)2). Elemental analysis for C31H27FN4O3S: calcd C, 67.13; H, 4.91; N, 10.10, found C, 67.29; H, 4.88; N, 10.16.
4.1.6.20. 2-(3,5-Bis(4-methoxyphenyl)-4,5-dihydro-1H-pyrazol-1-yl)-5-(1-ethyl-2-oxoindolin-3-ylidene)thiazol-4(5H)-one (13a). Orange powder (yield 80%), m.p. >300 °C, 1H NMR (400 MHz, TFA-d), δ 8.91 (d, J = 7.8 Hz, 1H, Ar-H), 8.15 (d, J = 8.5 Hz, 2H, Ar-H), 7.74 (t, J = 7.7 Hz, 1H, Ar-H), 7.55 (d, J = 8.3 Hz, 2H, Ar-H), 7.40 (t, J = 7.7 Hz, 1H, Ar-H), 7.33–7.26 (m, 4H, Ar-H), 7.24 (d, J = 7.9 Hz, 1H, Ar-H), 6.24 43 (dd, JXA = 10.0, JXB = 3.6 Hz, 1H, pyrazoline-HX (17-CH–), 4.53 (dd, JAX = 18.3, JAB = 9.8 Hz, 1H, pyrazoline-HA (16-CHA–), 4.18 (s, 3H, –OCH3), 4.16–4.14 (m, 2H, –CH2–), 4.12 (s, 3H, –OCH3), 3.87 (dd, JBA = 18.3, JBX 3.3 Hz, 1H, pyrazoline-HB (16-CHB–), 1.58 (t, J = 7.0 Hz, 3H, –CH3). 13C NMR (101 MHz, TFA-d) δ 169.93 (–C
O), 167.77 (–C
O), 166.50 (–N–C
N), 164.12 (ArC-OCH3), 160.00 (ArC-OCH3), 144.50, 135.45, 133.84, 130.85, 129.91, 127.51, 127.19, 124.81, 120.57, 119.20, 115.80, 114.86, 109.94, 66.73 (–CH–), 55.10 (Ar-OCH3), 54.97 (Ar-OCH3), 45.13 (–CH2–), 35.83 (–CH2–), 10.83 (–CH3). Elemental analysis for C30H26N4O4S: calcd C, 66.90; H, 4.87; N, 10.40, found C, 67.08; H, 4.88; N, 10.37.
4.1.6.21. 5-(1-Allyl-2-oxoindolin-3-ylidene)-2-(3,5-bis(4-methoxyphenyl)-4,5-dihydro-1H-pyrazol-1-yl)thiazol-4(5H)-one (13b). Yellow powder (yield 73%), m.p. >300 °C, 1H NMR (400 MHz, TFA-d), δ 8.94 (d, J = 7.5 Hz, 1H, Ar-H), 8.17 (d, J = 8.2 Hz, 2H, Ar-H), 7.74 (t, J = 7.6 Hz, 1H, Ar-H), 7.57 (d, J = 7.9 Hz, 2H, Ar-H), 7.42 (t, J = 7.2 Hz, 1H, Ar-H), 7.31 (dd, J = 8.3, 4.8 Hz, 4H, Ar-H), 7.24 (d, J = 7.8 Hz, 1H, Ar-H), 6.26 (dd, JXA = 8.3, JXB = 3.5 Hz, 1H, pyrazoline-HX (17-CH–), 6.08 (ddt, J = 13.8, 7.3 Hz, 1H, –CH
), 5.57–5.45 (m, 2H,
CH2), 4.72 (d, J = 4.7 Hz, 2H, –CH2–), 4.55 (dd, JAX = 17.9, JAB = 8.3 Hz, 1H, pyrazoline-HA (16-CHA–), 4.19 (s, 3H, –OCH3), 4.14 (s, 3H, –OCH3), 3.89 (dd, JBA = 17.9, JBX 3.5 Hz, 1H, pyrazoline-HB (16-CHB–). 13C NMR (101 MHz, TFA-d) δ 169.99 (–C
O), 168.10 (–C
O), 166.48 (–N–C
N), 164.16 (ArC-OCH3), 160.03 (ArC-OCH3), 144.73, 135.44, 133.61, 130.87, 129.79, 128.51, 127.50, 127.21, 124.89, 120.56, 119.07, 118.10 (
CH2–), 115.83, 114.87, 110.69, 66.77 (–CH–), 55.12 (Ar-OCH3), 54.98 (Ar-OCH3), 45.15 (–CH2–), 43.11 (–CH2–). Elemental analysis for C31H26N4O4S: calcd C, 67.62; H, 4.76; N, 10.18, found 67.83; H, 4.74; N, 10.11.
4.1.6.22. 2-(3,5-Bis(4-methoxyphenyl)-4,5-dihydro-1H-pyrazol-1-yl)-5-(1-isobutyl-2-oxoindolin-3-ylidene)thiazol-4(5H)-one (13c). Yellow powder (yield 72%), m.p. >300 °C, 1H NMR (400 MHz, TFA-d), δ 8.86 (d, J = 7.9 Hz, 1H, Ar-H), 8.13–8.06 (m, 2H, Ar-H), 7.67 (t, J = 7.7 Hz, 1H, Ar-H), 7.49 (d, J = 8.6 Hz, 2H, Ar-H), 7.33 (t, J = 7.8 Hz, 1H, Ar-H), 7.23 (t, J = 8.9 Hz, 4H, Ar-H), 7.16 (d, J = 8.0 Hz, 1H, Ar-H), 6.18 (dd, JXA = 10.1, JXB = 3.8 Hz, 1H, pyrazoline-HX (17-CH–), 4.46 (dd, JAX = 18.4, JAB = 10.1 Hz, 1H, pyrazoline-HA (16-CHA–), 4.09 (d, J = 22.3 Hz, 6H, (–OCH3)2), 3.83 (d, J = 7.1 Hz, 2H, –CH2–), 3.78 (dd, JBA = 18.4, JBX 3.8 Hz, 1H, pyrazoline-HB (16-CHB–), 2.35 (dt, J = 13.7, 6.9 Hz, 1H, –CH–), 1.16 (dd, J = 6.6, 2.0 Hz, 6H, (–CH3)2). 13C NMR (101 MHz, TFA-d) δ 169.84 (–C
O), 168.21 (–C
O), 166.43 (–N–C
N), 164.05 (ArC-OCH3), 159.92 (ArC-OCH3), 145.18, 135.29, 133.58, 130.79, 129.74, 127.47, 127.13, 124.85, 124.71, 120.52, 118.97, 118.54, 115.73, 114.79, 112.91, 110.38, 110.10, 66.65 (–CH2–), 55.03 (Ar-OCH3), 54.90 (Ar-OCH3), 48.49 (–CH2–), 45.06 (–CH2–), 26.99 (–CH–), 18.33 (–CH3)2). Elemental analysis for C32H30N4O4S: calcd C, 67.83; H, 5.34; N, 9.89, found C, 68.07; H, 5.31; N, 9.83.
4.2. Biological evaluation
The experimental procedures for antimicrobial XTT44,45 and SRB cytotoxicity37,38 assays are provided in the ESI.†
Conflicts of interest
No potential conflict of interest was reported by the author(s).
Acknowledgements
The authors extend their appreciation to the Princess Nourah bint Abdulrahman University Researchers Supporting Project number (PNURSP2022R25), Princess Nourah bint Abdulrahman University, Riyadh, Saudi Arabia. The authors would like to thank the Deanship of Scientific Research at Umm Al-Qura University for supporting this work by Grant Code: (22UQU4290565DSR87), maabourehab@uqu.edu.sa.
References
- M. H. Richmond, Resistance Factors and Their Ecological Importance to Bacteria and to Man, in Progress in Nucleic Acid Research and Molecular Biology, ed. J. N. Davidson and W. E. Cohn, Academic Press, 1973, pp. 191–248 Search PubMed.
- C. L. Ventola, The antibiotic resistance crisis: part 1: causes and threats, Pharmacol. Ther., 2015, 40(4), 277–283 Search PubMed.
- W. H. Organization, Antimicrobial resistance: global report on surveillance, World Health Organization, 2014 Search PubMed.
- D. van Duin and D. L. Paterson, Multidrug-Resistant Bacteria in the Community: Trends and Lessons Learned, Infect. Dis. Clin., 2016, 30(2), 377–390 CrossRef PubMed.
- J. O’Neill, Antimicrobial Resistance: Tackling a Crisis for the Health and Wealth of Nations: December 2014, Review on antimicrobial resistance, 2014 Search PubMed.
- C. Crabb, Global Alliance at full steam for new TB drugs, Bull. World Health Organ., 2002, 80(6), 517 Search PubMed.
- J. F. Da Silva, S. J. Garden and A. C. Pinto, The chemistry of isatins: a review from 1975 to 1999, J. Braz. Chem. Soc., 2001, 12, 273–324 CrossRef CAS.
- G. M. Blumenthal, et al., FDA approval summary: sunitinib for the treatment of progressive well-differentiated locally advanced or metastatic pancreatic neuroendocrine tumors, Oncol., 2012, 17(8), 1108 CrossRef CAS PubMed.
- W. M. Eldehna, et al., Development of novel benzofuran-isatin conjugates as potential antiproliferative agents with apoptosis inducing mechanism in Colon cancer, J. Enzyme Inhib. Med. Chem., 2021, 36(1), 1424–1435 Search PubMed.
- P. A. T. De Moraes Gomes, L. J. Pena and A. C. L. Leite, Isatin Derivatives and Their Antiviral Properties Against Arboviruses: A Review, Mini Rev. Med. Chem., 2019, 19(1), 56–62 CrossRef PubMed.
- W. M. Eldehna, et al., Development of isatin-thiazolo[3,2-a]benzimidazole hybrids as novel CDK2 inhibitors with potent in vitro apoptotic anti-proliferative activity: Synthesis, biological and molecular dynamics investigations, Bioorg. Chem., 2021, 110, 104748 CrossRef CAS PubMed.
- H. A. Abdel-Aziz, et al., Isatin-benzoazine molecular hybrids as potential antiproliferative agents: synthesis and in vitro pharmacological profiling, Drug Des. Dev. Ther., 2017, 11, 2333 CrossRef CAS.
- R. E. Ferraz de Paiva, et al., Anticancer Compounds Based on Isatin-Derivatives: Strategies to Ameliorate Selectivity and Efficiency, Front. Mol. Biosci., 2021, 7, 627272 CrossRef PubMed.
- M. F. Abo-Ashour, et al., Novel indole-thiazolidinone conjugates: Design, synthesis and whole-cell phenotypic evaluation as a novel class of antimicrobial agents, Eur. J. Med. Chem., 2018, 160, 49–60 CrossRef CAS PubMed.
- Z. M. Elsayed, et al., Development of novel isatin–nicotinohydrazide hybrids with potent activity against susceptible/resistant Mycobacterium tuberculosis and bronchitis causing–bacteria, J. Enzyme Inhib. Med. Chem., 2021, 36(1), 384–393 CrossRef CAS PubMed.
- Y.-L. Fan, X. Ke and M. Liu, Coumarin–triazole Hybrids and Their Biological Activities, J. Heterocycl. Chem., 2018, 55(4), 791–802 CrossRef CAS.
- Z. A. Kaplancıklı, et al., Synthesis and Antimicrobial Activity of Some Thiazolyl-Pyrazoline Derivatives, Phosphorus, Sulfur, Silicon Relat. Elem., 2007, 182(4), 749–764 CrossRef.
- B. Varghese, S. N. Al-Busafi, F. O. Suliman and S. M. Al-Kindy, Unveiling a versatile heterocycle: pyrazoline–a review, RSC Adv., 2017, 7(74), 46999–47016 RSC.
- F. Turkan, et al., Synthesis, characterization, molecular docking and biological activities of novel pyrazoline derivatives, Arch. Pharmazie, 2019, 352(6), 1800359 CrossRef.
- A. Zervosen, et al., Development of New Drugs for an Old Target — The Penicillin Binding Proteins, Molecules, 2012, 17(11), 12478–12505 CrossRef CAS PubMed.
-
(a) M. Haroun, C. Tratrat, E. Tsolaki and A. Geronikaki, Thiazole-Based Thiazolidinones as Potent Antimicrobial Agents. Design, Synthesis and Biological Evaluation, Comb. Chem. High Throughput Screen., 2016, 19(1), 51–57 CrossRef CAS PubMed;
(b) R. E. Khidre and I. A. M. Radini, Design, synthesis and docking studies of novel thiazole derivatives incorporating pyridine moiety and assessment as antimicrobial agents, Sci. Rep., 2021, 11, 7846 CrossRef CAS PubMed.
- A. V. Lozynskyi, H. O. Derkach, V. V. Zasidko, Y. T. Konechnyi, N. S. Finiuk, Y. T. Len, R. V. Kutsyk, M. S. Regeda and R. B. Lesyk, Antimicrobial and cytotoxic activities of thiazolo [4, 5-b] pyridine derivatives, Biopolym. Cell, 2021, 37(2), 153 Search PubMed.
- D. Ashtekar, et al., A rapid method for the evaluation of new antituberculous agents, Chemotherapy, 1987, 33(1), 22–27 CrossRef CAS.
- G. Maass, et al., Viral resistance to the thiazolo-iso-indolinones, a new class of nonnucleoside inhibitors of human immunodeficiency virus type 1 reverse transcriptase, Antimicrob. Agents Chemother., 1993, 37(12), 2612–2617 CrossRef CAS PubMed.
- C. Deng, H. Yan, J. Wang, B. S. Liu, K. Liu and Y. M. Shi, The anti-HIV potential of imidazole, oxazole and thiazole hybrids: A mini-review, Arab. J. Chem., 2022, 104242 CrossRef CAS.
- V. Cuartas, et al., New thiazolyl-pyrazoline derivatives bearing nitrogen mustard as potential antimicrobial and antiprotozoal agents, Arch. Pharm., 2020, 353(5), e1900351 CrossRef CAS.
- E. Mansour, et al., A new series of thiazolyl pyrazoline derivatives linked to benzo[1,3]dioxole moiety: Synthesis and evaluation of antimicrobial and anti-proliferative activities, Synth. Commun., 2020, 50(3), 368–379 CrossRef CAS.
- V. Cuartas, et al., New thiazolyl-pyrazoline derivatives bearing nitrogen mustard as potential antimicrobial and antiprotozoal agents, Arch. Pharm., 2020, 353(5), e1900351 CrossRef CAS PubMed.
- A. Arwansyah, et al., Theoretical studies of Thiazolyl-Pyrazoline derivatives as promising drugs against malaria by QSAR modelling combined with molecular docking and molecular dynamics simulation, Mol. Simul., 2021, 1–14 Search PubMed.
- H.-H. Wang, et al., Synthesis, molecular docking and evaluation of thiazolyl-pyrazoline derivatives containing benzodioxole as potential anticancer agents, Bioorg. Med. Chem., 2013, 21(2), 448–455 CrossRef CAS PubMed.
- P.-C. Lv, et al., Synthesis, molecular docking and evaluation of thiazolyl-pyrazoline derivatives as EGFR TK inhibitors and potential anticancer agents, Bioorg. Med. Chem. Lett., 2011, 21(18), 5374–5377 CrossRef CAS PubMed.
- B. Sever, et al., Thiazolyl-pyrazoline derivatives: In vitro and in silico evaluation as potential acetylcholinesterase and carbonic anhydrase inhibitors, Int. J. Biol. Macromol., 2020, 163, 1970–1988 CrossRef CAS.
- T.-L. Ho, Hard soft acids bases (HSAB) principle and organic chemistry, Chem. Rev., 1975, 75(1), 1–20 CrossRef CAS.
- O. M. Aly, et al., Synthesis, cytotoxicity, docking study, and tubulin polymerization inhibitory activity of novel 1-(3, 4-dimethoxyphenyl)-5-(3, 4, 5-trimethoxyphenyl)-1h-1, 2, 4-triazole-3-carboxanilides, Arch. Pharmazie, 2014, 347(9), 658–667 CrossRef CAS PubMed.
- M. M. Al-Sanea, et al., Development of 3-methyl/3-(morpholinomethyl) benzofuran derivatives as novel antitumor agents towards non-small cell lung cancer cells, J. Enzym. Inhib. Med. Chem., 2021, 36(1), 987–999 CrossRef CAS PubMed.
- A. Jacobs, Understanding Organic Reaction Mechanisms, Cambridge University Press, Cambridge, 1997 Search PubMed.
- P. Skehan, et al., New colorimetric cytotoxicity assay for anticancer-drug screening, J. Natl. Cancer Inst., 1990, 82(13), 1107–1112 CrossRef CAS PubMed.
- W. M. Eldehna, et al., Identification of 3-(piperazinylmethyl) benzofuran derivatives as novel type II CDK2 inhibitors: design, synthesis, biological evaluation, and in silico insights, J. Enzym. Inhib. Med. Chem., 2022, 37, 1227–1240 CrossRef CAS PubMed.
- M. D. Bowman, M. M. Jacobson and H. E. Blackwell, Discovery of fluorescent cyanopyridine and deazalumazine dyes using small molecule macroarrays, Org. Lett., 2006, 8(8), 1645–1648 CrossRef CAS PubMed.
- F. Kayamba, et al., Design and synthesis of quinoline-pyrimidine inspired hybrids as potential plasmodial inhibitors, Eur. J. Med. Chem., 2021, 217, 113330 CrossRef CAS PubMed.
- K.-M. Qiu, et al., Design, synthesis and biological evaluation of pyrazolyl-thiazolinone derivatives as potential EGFR and HER-2 kinase inhibitors, Bioorg. Med. Chem., 2012, 20(6), 2010–2018 CrossRef CAS PubMed.
- S. Kanmazalp, et al., Crystal Structure and Hirshfeld Surface Analysis of 3, 5-Bis (4-Methoxyphenyl)-4, 5-Dihydro-1H-Pyrazole-1-Carbothioamide, J. Struct. Chem., 2020, 61(1), 126–132 CrossRef CAS.
- M. F. Abo-Ashour, et al., Novel hydrazido benzenesulfonamides-isatin conjugates: Synthesis, carbonic anhydrase inhibitory activity and molecular modeling studies, Eur. J. Med. Chem., 2018, 157, 28–36 CrossRef CAS PubMed.
- F. V. Loures and S. M. Levitz, XTT assay of antifungal activity, Bio-Protoc., 2015, 5(15), e1543 Search PubMed.
- H. A. Abuelizz, et al., In silico study and biological screening of benzoquinazolines as potential antimicrobial agents against methicillin-resistant Staphylococcus aureus, carbapenem-resistant Klebsiella pneumoniae, and fluconazole-resistant Candida albicans, Microb. Pathog., 2021, 160, 105157 CrossRef CAS PubMed.
|
This journal is © The Royal Society of Chemistry 2022 |
Click here to see how this site uses Cookies. View our privacy policy here.