DOI:
10.1039/D2RA04669E
(Paper)
RSC Adv., 2022,
12, 30404-30415
Microwave-assisted multicomponent synthesis of antiproliferative 2,4-dimethoxy-tetrahydropyrimido[4,5-b]quinolin-6(7H)-ones†
Received
26th July 2022
, Accepted 17th October 2022
First published on 25th October 2022
Abstract
In this study, we demonstrate a simple, highly efficient, rapid and convenient series of 2,4-dimethoxy-tetrahydropyrimido[4,5-b]quinolin-6(7H)-ones 4a–v. Microwave irradiation facilitates the one-pot multicomponent reaction of different aromatic aldehydes, 6-amino-2,4-dimethoxypyrimidine and dimedone using glacial acetic acid. Metal-free multicomponent synthesis, shorter reaction time, higher product yield, easy product purification without column chromatography and outstanding green credential parameters are the key features of this protocol. We analysed 4a–v against six human tumour cell lines for antiproliferative activity. 4h, 4o, 4q and 4v show good antiproliferative activity with a good in silico ADMET profile. Furthermore, 4h, 4o, 4q and 4v also show drug-likeness properties by obeying drug-like filters.
1. Introduction
The worldwide burden of tumours and cancer has been steadily increasing and is expected to continue to rise in the next decades because of population ageing and urban lifestyles.1 So, the need to develop antitumor agents has also increased in recent times. Previously we reported antiproliferative activity of pyrimidine and quinoline based heterocycles against six human tumour cell lines. In this work, we developed novel pyrimidine and quinoline based heterocycles to develop a new potent antiproliferative agent.2,3 Multicomponent reactions (MCRs) facilitated by microwave irradiation are an effective strategy from the perspective of green chemistry.4 Microwave irradiation is a powerful tool in heterocyclic chemistry as well as drug discovery.4,5 When MW radiation comes into contact with solvent, bubbles develop, grow and then burst, generating hot regions of high temperature and pressure. These hot regions generate enough energy to progress the reaction due to the elevation in number of species. These species produce sufficient kinetic energy to exceed the activation energy barrier.6 Microwave irradiation has numerous benefits, such as being homogeneous and quick heating, having shorter reaction times, higher reaction rates, lower energy consumption and low waste production.7,8 As a result, synthesis utilizing MCRs under MW irradiation proceeds effortlessly and in a benign way.
In 2010, pyrimido[4,5-b]quinolines (PQs) were reported as a new class of antitumor agents and found PQ I as a potent antitumor agent.9 Other research groups then worked on PQs and explored more antitumor PQs (II–III) (Fig. 1a).10,11 Thus, PQs are the best candidate to explore new antitumor agents. Some mechanisms for the anticancer effects of pyrimido[4,5-b]quinolines were reported including the inhibition of vascular epithelial growth factor receptor tyrosine kinase,12 topoisomerases I and Epidermal growth factor receptor.13 In other case, pyrimido[4,5-b]quinolines showed the anticancer effects through caspase-3 activation. They also inhibited tubulin polymerization, arrested cell cycle at G2/M phase and induced apoptosis.14 Methoxy group containing heterocycles have more potential of antitumor or anticancer activity. For instance, II and III of Fig. 1a contain methoxy group in their molecules. Many anticancer drugs like bosutinib,15 brigatinib,16 cabazitaxel,17 erdafitinib,18 lurbinectedin19 and trabectedin20 contain methoxy group in their chemical structure. So, here we developed methoxy group containing PQs i.e., 2,4-dimethoxy-tetrahydropyrimido[4,5-b]quinolin-6(7H)-ones (2,4-dimethoxy-THPQs).
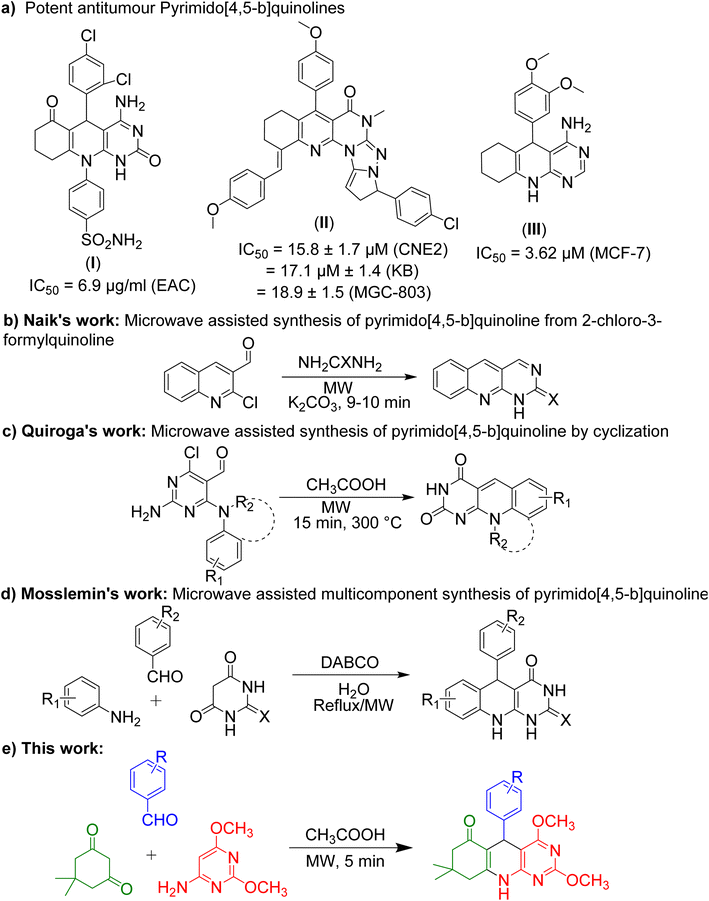 |
| Fig. 1 (a) Representative PQs with antiproliferative activity. (b–d) Reported synthetic strategies to obtain PQs. (e) Synopsis of the synthetic methodology reported in this work. | |
In our previous work, 2,4-dimethoxy-THPQs were synthesised by conventional heating in acetic acid medium.21 To improve the protocol, irradiation of microwave was used for acceleration of multicomponent synthesis. Irradiation of microwave was used in the synthesis of heterocycle because it offers unique advantages. It reduces the time of the reaction and enhances the yield.22 Fig. 1 show previous work on microwave-assisted synthesis of PQs. The microwave-assisted reaction of 2-chloro-3-formylquinoline with urea/thiourea produced PQs in 9–10 minutes (Fig. 1b).23 Microwave-assisted cyclisation reaction of 2,4-diamino-6-chloro-pyrimidine-5-carbaldehydes in the presence of acetic acid produced PQs (Fig. 1c).24 The microwave-assisted multicomponent reaction of aldehyde, amine and (thio)barbituric acid also produced PQs (Fig. 1d).25 In this work, 2,4-dimethoxy-THPQs were synthesised by microwave-assisted multicomponent reaction of aldehydes, dimedone and 6-amino-2,4-dimethoxypyrimidine in continuation of our work on exploring novel bioactive heterocycles (Fig. 1e).26–32 2,4-dimethoxy-THPQs were then evaluated for in vitro antiproliferative activity against six human tumour cell lines. Furthermore, the most potent 2,4-dimethoxy-THPQs are evaluated by in silico ADMET and drug-likeness properties.
2. Results and discussions
2.1. Chemistry
In the above concept, we selected p-chlorobenzaldehyde 1a, 6-amino-2,4-dimethoxypyrimidine 2 and dimedone 3 as model substrates to explore the microwave irradiated multicomponent synthesis of 4a (Scheme 1). In order to check the effect of the selected solvent and catalyst, we optimised the rection by different reaction conditions (Table 1). Complete conversation of 1a into 4a did not observe in catalyst-free condition with ethanol and water (Table 1, entry 1–3). As discussed earlier, p-TSA is the best catalyst for similar reactions. So, we utilised it for optimisation (Table 1, entry 4–7). Best results were observed when glacial acetic acid was used as a solvent. At room temperature, p-TSA/AcOH converted 1a to 4a in 25 minutes. In comparison, p-TSA/AcOH converted 1a to 4a in 10 minutes under reflux. Similar results are also observed in the case of glacial acetic acid only (Table 1, entry 8 and 9). So, we decided to proceed with the best-optimised condition (Table 1, entry 9) in which desired product 4a was obtained in only 10 minutes by glacial acetic acid under reflux.
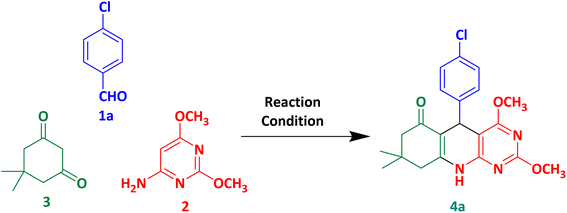 |
| Scheme 1 Three-component synthesis of 4a from p-chlorobenzaldehyde, 6-amino-2,4-dimethoxypyrimidine and dimedone. | |
Table 1 Optimization of solvent and catalyst for the preparation of compound 4a under Microwave irradiationa
Entry |
Catalyst |
Solventb |
Temperature |
Time (min) |
Conversion relative to aldehydec |
Reaction condition: 1 mmol p-chlorobenzaldehyde 1a, 1 mmol 6-amino-2,4-dimethoxypyrimidine 2 and 1 mmol dimedone 3, 200 W MW. 3 mL solvent. Observed from TLC analysis. Obtained by conventional heating. |
1 |
— |
Water |
RT |
— |
— |
2 |
— |
Ethanol |
RT |
— |
— |
3 |
— |
Ethanol |
Reflux |
35 |
Incomplete |
4 |
p-TSA (20 mol%) |
Ethanol |
Reflux |
45 |
100% |
5 |
p-TSA (20 mol%) |
Acetonitrile |
Reflux |
30 |
100% |
6 |
p-TSA (20 mol%) |
Acetic acid |
RT |
25 |
100% |
7 |
p-TSA (20 mol%) |
Acetic acid |
Reflux |
10 |
100% |
8 |
— |
Acetic acid |
RT |
25 |
100% |
9 |
— |
Acetic acid |
Reflux |
10 |
100% |
10 |
— |
Acetic acid |
Reflux |
90 |
100%d |
In order to check the effect of microwave irradiation power, we optimised the rection by applying different microwave irradiation power (Table 2). The best results were obtained by applying 75 W MW with the highest 84% isolated yield in only 5 minutes.
Table 2 Optimization of the power of microwave irradiation for synthesis of 4aa
Entry |
Power (W) |
Time (Min) |
Yieldb (%) |
Reaction condition: 1 mmol p-chlorobenzaldehyde 1a, 1 mmol 6-amino-2,4-dimethoxypyrimidine 2 and 1 mmol dimedone 3, 3 mL acetic acid, reflux. Isolated yield. |
1 |
200 |
10 |
78 |
2 |
150 |
10 |
82 |
3 |
150 |
5 |
83 |
4 |
100 |
10 |
82 |
5 |
75 |
10 |
80 |
6 |
75 |
5 |
84 |
7 |
50 |
5 |
83 |
We also carried out a reaction with a similar protocol with conventional heating (Table 1, entry 10). It produced 4a in 90 minutes by glacial acetic acid under reflux with 73% isolated yield. To evaluate the greenness of the conventional vs. microwave synthesis, the green metrics (see ESI† for the definition of green metrics and detailed calculation process) were conducted and the results are shown in Tables 3 and 4. The AE for both conventional vs. microwave synthesis is 91.74% due to the same reactants. AEf, CE, RME, OE and MP of microwave is higher than the conventional method. Whereas PMI, E-factor, SI and WI of microwave is lower than the conventional method. So, from both ways, the microwave process is greener than the conventional method for the reaction of model substrates 1a, 2 and 3.
Table 3 Green metrics (AE, AEf, CE, RME, OE and MP) analysis for conventional and microwave synthesis of 4a
Method |
% Yielda |
AE |
AEf |
CE |
RME |
OE |
MP |
Isolated yield. Abbreviation: AE: atom economy, AEf: atom efficiency, CE: carbon efficiency, RME: reaction mass efficiency, OE: optimum efficiency, MP: mass productivity. |
Conventional |
73 |
91.74 |
66.97 |
72.52 |
66.67 |
72.67 |
0.95 |
Microwave |
84 |
91.74 |
77.06 |
83.28 |
76.55 |
83.44 |
1.09 |
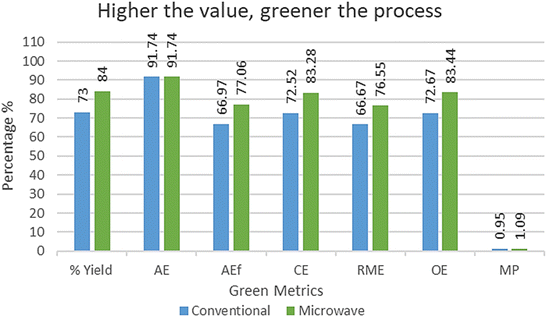 |
Table 4 Green metrics (PMI, E-factor, SI and WI) analysis for conventional and microwave synthesis of 4aa
Method |
Time (min) |
PMI |
E-factor |
SI |
WI |
PMI: process mass intensity, E-factor: environmental factor, SI: solvent intensity and WI: water intensity. |
Conventional |
90 |
105.17 |
104.17 |
34.71 |
68.97 |
Microwave |
5 |
91.59 |
90.59 |
30.23 |
60.06 |
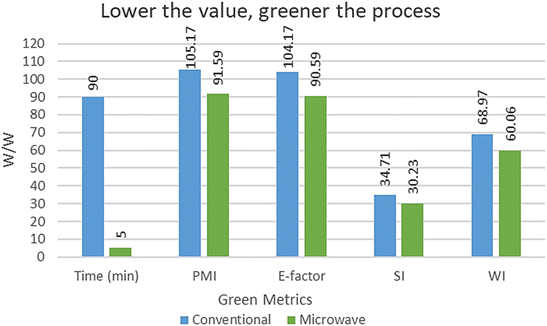 |
With the best optimal reaction condition in hand, we then explore the substrate scope of this 2,4-dimethoxy-THPQ derivative using different substituted aromatic/heteroaromatic aldehyde 1a–v with dimedone 2 and 6-amino-2,4-dimethoxypyrimidine 3. Under this optimal reaction conditions, reaction performed smoothly with both electron-releasing and electron-withdrawing substituted aldehydes and give excellent yield up to 87%.
Using this green protocol, we synthesize twenty-two 2,4-dimethoxy-THPQs 4a–v from variety of substrate (Table 5). In primary investigation, formation of desired product was confirmed by TLC analyses using mixture of 30% ethyl acetate and 70% n-hexane. The desired product was obtained with minor impurities, which were easily removed by washing of ice-cold diethyl ether. The derivatives 4p–t were purified by washing with ice-cold aqueous methanol. The derivative 4u was soluble in water when reaction mixture poured into water, after 20 minutes pure solid product was formed which was isolated by filtration.
Table 5 Substrate scope of the synthesis of 2,4-dimethoxy-THPQs 4a–va
Reaction condition: 1 mmol aldehyde 1, 1 mmol 6-amino-2,4-dimethoxypyrimidine 2 and 1 mmol dimedone 3, 3 mL AcOH, 75 W MW, Reflux. Isolated yields are mentioned with the product. |
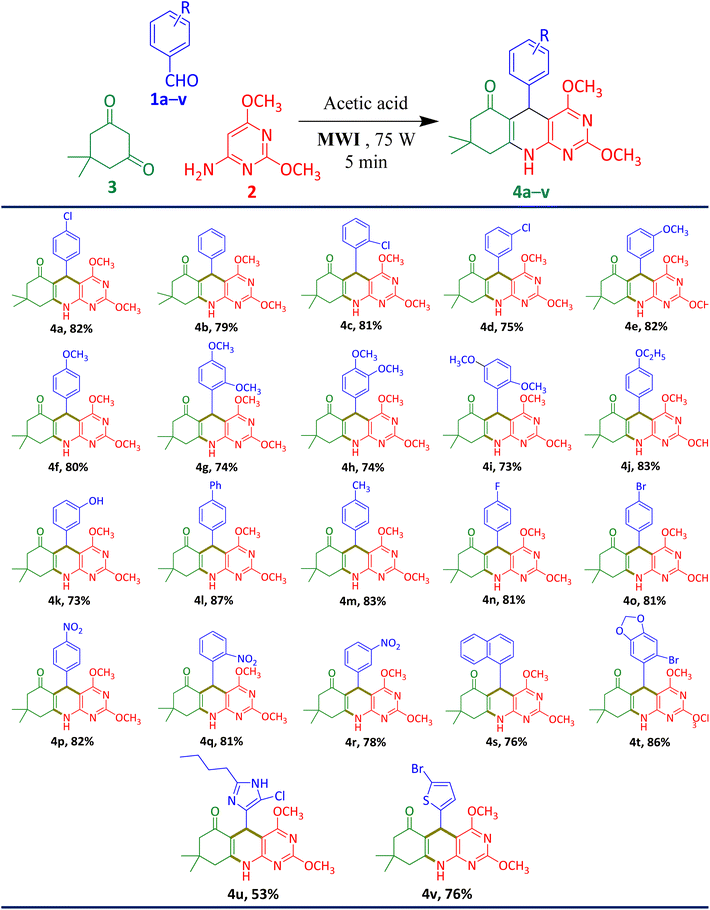 |
The structure of all the synthesized compound were characterised via. 1H NMR, 13C NMR and either HRMS or LCMS analysis. Furthermore, we developed a single crystal of product 4f and 4o and it was studied using single-crystal X-ray diffraction (SCXRD). The structure of 4f and 4o were fully confirmed by results of the analysis. The single-crystal XRD data of 4f shows that it was crystalises in monoclinic crystal system, space group C2/c with unit cell dimension a = 17.3963(6) Å, b = 22.485(1) Å, c = 14.0877(9) Å, α = 90°, β = 127.7461(13)°, γ = 90°, V = 4357.3(4) Å3, Z = 8, density = 1.206 g cm−3 and linear absorption coefficient 0.084 mm−1. The product 4o crystalises in triclinic crystal system, space group P-1 with unit cell dimension a = 6.0680(2) Å, b = 13.0347(5) Å, c = 13.5866(5) Å, α = 103.302(3)°, β = 95.6327(18)°, γ = 103.2902(19)°, V = 1004.76(7) Å3, Z = 2, density = 1.469 g cm−3 and linear absorption coefficient 2.072 mm−1. XRD data were deposited online to Cambridge Crystallographic Data Centre (CCDC). CCDC deposition number 2157101 and 2149724 contain the ESI crystallographic data for this paper.
Herein, we proposed plausible reaction root based on the literature reports,33,34 (Scheme 2). Firstly, Knoevenagel condensation was carried out in between aromatic aldehyde and dimedone in presence of acetic acid under microwave irradiation and resulting in the formation of possible Knoevenagel adduct (K) by elimination of first water molecule. In next step, 6-amino-2,4-dimethoxy aniline readily undergoes nucleophilic attack on this Knoevenagel adduct (K) and produce an imine derivative. In the final step, this imine derivative undergoes intramolecular ring-closing step via C–N bond formation by elimination of second water molecule in presence of acetic acid and furnished the final product.
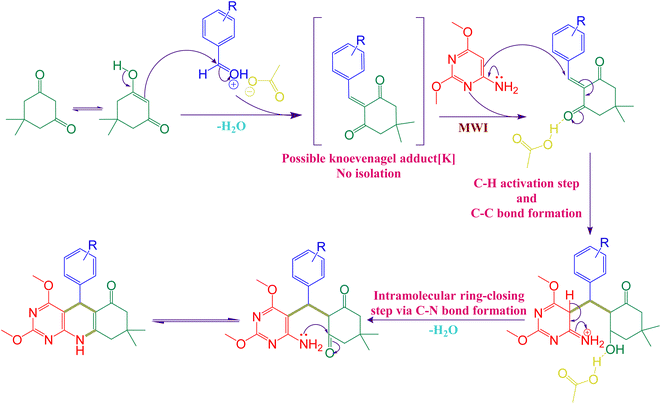 |
| Scheme 2 A plausible reaction root for the formation of 2,4-dimethoxy-THPQs. | |
2.2. Antiproliferative activity
The antiproliferative activity of 2,4-dimethoxy-THPQs was 4a–v studied in a panel of six human solid tumour cell lines. From the initial set of compounds 4a–v derivatives, 4c–e, 4l and 4s were not tested due to their poor solubility under the experimental conditions. The results reported as 50% growth inhibition (GI50) are shown in Table 6. The results are best compared in the GI50 range plot (Fig. 2). The data from the GI50 range plot reveals that most of the compounds have similar activity across the cell line panel. The most active compounds of the series (4h, 4o, 4q and 4v) displayed GI50 values at comparable range that the one displayed by CDDP. From the results, it is not possible to obtain a clear structure–activity relationship (SAR). For instance, compound 4h holds an electron-donating group (OMe) at para position and 4o has an electron-withdrawing group (Br), whilst 4q bears a nitro group in ortho position.
Table 6 Antiproliferative activity (GI50, μM) against human solid tumour cell lines
Compound |
A549 (lung) |
HBL-100 (breast) |
HeLa (cervix) |
SW1573 (lung) |
T-47D (breast) |
WiDr (colon) |
4a |
13 ± 5.1 |
24 ± 7.9 |
17 ± 5.8 |
27 ± 0.71 |
26 ± 6.5 |
17 ± 1.6 |
4b |
29 ± 4.2 |
38 ± 8.1 |
28 ± 4.8 |
37 ± 7.5 |
33 ± 5.7 |
31 ± 9.1 |
4f |
30 ± 4.5 |
41 ± 8.6 |
33 ± 0.87 |
38 ± 0.95 |
32 ± 1.7 |
39 ± 6.5 |
4g |
38 ± 1.6 |
41 ± 12 |
34 ± 3.4 |
46 ± 1.4 |
33 ± 10 |
49 ± 12 |
4h |
37 ± 4.8 |
1.6 ± 0.47 |
27 ± 1.4 |
43 ± 6.5 |
23 ± 3.1 |
42 ± 8.4 |
4i |
45 ± 9.7 |
64 ± 31 |
40 ± 14 |
15 ± 6.0 |
37 ± 4.7 |
44 ± 7.5 |
4j |
28 ± 5.5 |
35 ± 7.8 |
34 ± 11 |
21 ± 7.0 |
39 ± 16 |
44 ± 21 |
4k |
51 ± 7.7 |
81 ± 17 |
>100 |
60 ± 2.6 |
83 ± 29 |
83 ± 18 |
4m |
24 ± 1.3 |
29 ± 13 |
13 ± 6.1 |
30 ± 0.87 |
21 ± 7.1 |
30 ± 3.6 |
4n |
19 ± 3.5 |
24 ± 3.7 |
27 ± 4.6 |
32 ± 5.4 |
17 ± 1.6 |
26 ± 1.1 |
4o |
7.7 ± 2.4 |
19 ± 7.9 |
8.3 ± 1.1 |
20 ± 1.3 |
16 ± 3.2 |
12 ± 5.8 |
4p |
23 ± 6.1 |
33 ± 4.2 |
15 ± 0.4 |
21 ± 8.6 |
27 ± 1.9 |
31 ± 4.6 |
4q |
12 ± 4.7 |
10 ± 3.2 |
4.0 ± 0.98 |
8.0 ± 0.45 |
10 ± 2.8 |
19 ± 0.88 |
4r |
25 ± 3.4 |
35 ± 5.1 |
30 ± 8.9 |
47 ± 15 |
32 ± 0.14 |
36 ± 1.9 |
4t |
30 ± 1.9 |
37 ± 15 |
25 ± 11 |
17 ± 0.77 |
48 ± 12 |
35 ± 0.4 |
4u |
47 ± 15 |
88 ± 21 |
71 ± 26 |
35 ± 1.7 |
92 ± 13 |
80 ± 29 |
4v |
9.5 ± 0.15 |
17 ± 7.9 |
11 ± 3.4 |
6.0 ± 0.10 |
16 ± 6.3 |
14 ± 4.1 |
CDDP |
4.9 ± 0.2 |
1.9 ± 0.2 |
1.8 ± 0.5 |
2.7 ± 0.4 |
17 ± 3.3 |
23 ± 4.3 |
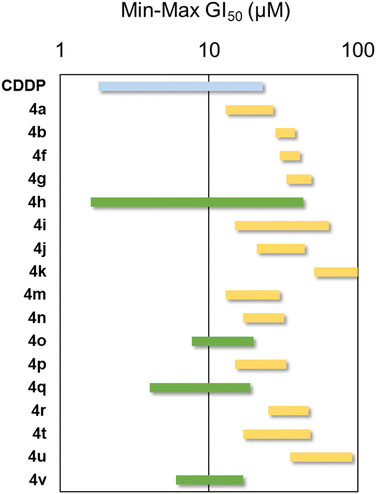 |
| Fig. 2 GI50 range plot against human solid tumour cell lines. Cisplatin (CDDP) was used as reference anticancer drug (blue bar). Green bars indicate the most active compounds. | |
2.3. ADMET prediction
Potent antiproliferative 2,4-dimethoxy-THPQs 4h, 4o, 4q and 4v were evaluated for in silico ADMET prediction which is important drug profiling for novel drug discovery.35 Various filters such as Lipinski filter, Ghose filter, Veber filter, Egan filter and Muegge filter are help to predict drug likeness based on their physicochemical properties. 4h, 4o, 4q and 4v were evaluated for their ADMET properties with the help of the online web server SwissADME (http://www.swissadme.ch/). Calculated physicochemical properties of are summarised in Table 7.
Table 7 Physicochemical properties of 4h, 4o, 4q and 4v with various filters for drug-likenessa
Comp. |
MW |
RB |
HBA |
HBD |
MR |
TPSA |
X log P |
W log P |
M log P |
NR |
NC |
NH |
Atom |
Abbreviation: MW: molecular weight; RB: rotational bond; HBA: H-bond acceptor; HBD: H-Bond Donor; MR: Molecular Refractivity; TPSA: Topological Polar Surface Area; NR: No. of ring; NC: No. of Carbon; NH: No. of Heteroatoms. |
4h |
425.48 |
5 |
7 |
1 |
118.58 |
91.8 |
3.62 |
3.14 |
1.97 |
4 |
23 |
8 |
58 |
4o |
444.32 |
3 |
5 |
1 |
113.3 |
73.34 |
4.36 |
3.89 |
3.18 |
4 |
21 |
7 |
50 |
4q |
410.42 |
4 |
7 |
1 |
114.42 |
119.16 |
3.5 |
3.03 |
1.7 |
4 |
21 |
9 |
52 |
4v |
450.35 |
3 |
5 |
1 |
111.17 |
101.58 |
4.41 |
3.95 |
2.78 |
4 |
19 |
8 |
47 |
Lipinski filter |
Ghose filter |
Veber filter |
Egan filter |
Muegge filter |
MW ≤ 500 |
160 ≤ MW ≤ 480 |
RB ≤ 10 |
WlogP ≤ 5.88 |
200 ≤ MW ≤ 600 |
M log P ≤ 4.15 |
−0.4 ≤ W log P ≤ 5.6 |
TPSA ≤ 140 |
TPSA ≤ 131.6 |
−2 X log P ≤ 5; TPSA ≤ 150 |
HBA ≤ 10 |
40 ≤ MR ≤ 130 |
|
|
NR ≤ 7; NC > 4; NH > 1; RB ≤ 15; HBA ≤ 10; HBD ≤ 5 |
HBD ≤ 5 |
20 ≤ atoms ≤ 70 |
|
|
|
Bioavailability radar plot of 2,4-dimethoxy-THPQs 4h, 4o, 4q and 4v show in Fig. 3 display that all four 2,4-dimethoxy-THPQs have drug like radar plot. Calculated bioavailability score found as 0.55 for all 2,4-dimethoxy-THPQs. To predict the gastrointestinal absorption and blood–brain barrier permeability of 2,4-dimethoxy-THPQs 4h, 4o, 4q and 4v, BOILED-Egg delineation was used. BOILED-Egg delineation of these 2,4-dimethoxy-THPQs is illustrated in Fig. 4. “The white region is the physicochemical space of molecules with highest probability of being absorbed by the gastrointestinal tract, and the yellow region (yolk) is the physicochemical space of molecules with highest probability to permeate to the brain”.36 4h, 4q and 4v show high gastrointestinal absorbance, and they have no blood–brain permeability. While 4o show blood–brain permeability. Red dots for 4o and 4v denoted that they are not P-gp substrates while blue dots for 4h and 4q denoted that they are P-gp substrates.
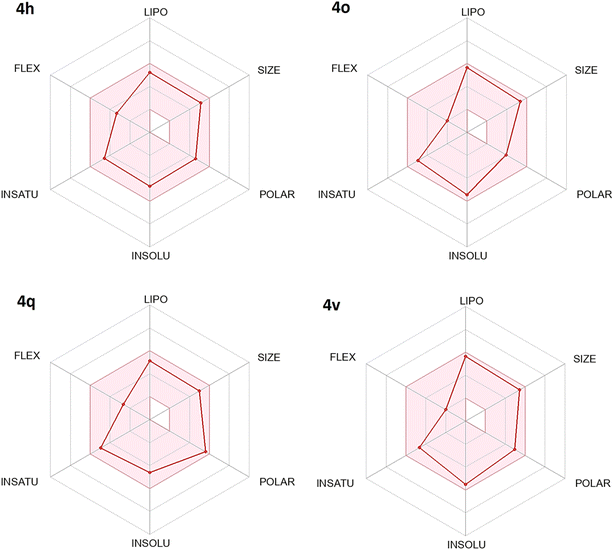 |
| Fig. 3 Bioavailability radar of 2,4-dimethoxy-THPQs 4h, 4o, 4q and 4v. | |
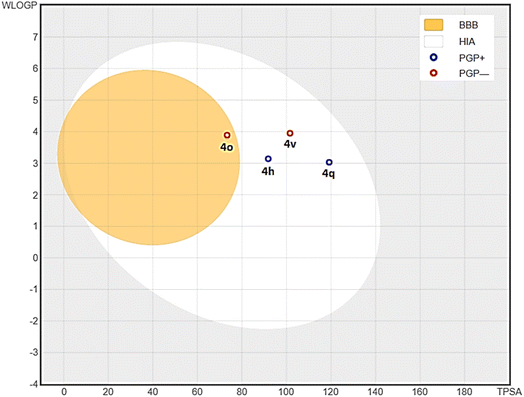 |
| Fig. 4 BOILED-Egg model of 2,4-dimethoxy-THPQs 4h, 4o, 4q and 4v. | |
3. Experimental section
3.1. General methods
For synthesis, all chemical reagents were purchased from the TCI, Sigma-Aldrich and Sisco Research Laboratories Pvt. Ltd and used without further purification. The microwave-assisted reactions were performed in a “SINEO UWave-1000 Microwave, UV, US Synthesis Extraction Reactor”. The progress of all chemical reactions was monitored by thin-layer chromatography (TLC, on aluminium plates pre-coated with F254 silica gel 60). Melting points of all solid compounds were determined by the open capillary tube method and are uncorrected. High-resolution mass spectra (HRMS) were acquired using Agilent technologies model G6564 QTOF. The samples were ionized in positive ion mode using a MALDI or ESI ionization sources. The LCMS analysis was collected on an MS-Agilent 6120 quadrupole. Nuclear magnetic resonance spectra (1H NMR & 13C NMR) were recorded on a Bruker 500 MHz WB FT-NMR spectrometer having proton noise decoupling mode with a standard 5 mm probe using CDCl3 and DMSO-d6 solution. Abbreviations are used for the 1H NMR signal are as follows: s = singlet, d = doublet, t = triplet, q = quartet dd = double doublet, td = triple doublet, m = multiplet. The chemical shifts are reported in parts per million and coupling constants (J) are provided in Hertz.
3.2. General procedure for synthesis of 2,4-dimethoxy-THPQs 4a–v
A mixture of aromatic aldehydes (1, 1 mmol), 6-amino-2,4-dimethoxypyrimidine (2, 1 mmol), dimedone (3, 1 mmol) and acetic acid (3 mL) were charged into a microwave vessel. The reaction mixture was heated at 110 °C (75 W) by MW irradiation for 5 min. The reaction progress was monitored on TLC using n-hexane
:
ethyl acetate (70
:
30, v/v) as mobile phase. After complete consumption of starting material, the reaction mixture was cooled to room temperature and poured into 20 mL cold water. The obtained crude product was filtered and dried in an oven. Further purification, the crude product was stirred in 5 mL ice-cold diethyl ether or methanol as mentioned in results and discussion to obtain pure product. The products 4f and 4o were crystallised by a slow evaporation method using a mixture of 20 mL dichloromethane and 3 mL methanol.
3.2.1. 5-(4-Chlorophenyl)-2,4-dimethoxy-8,8-dimethyl-5,8,9,10-tetrahydropyrimido[4,5-b]quinolin-6(7H)-one (4a). White solid, mp. 208–210 °C; 1H-NMR (500 MHz, CDCl3) (δ, ppm): 8.46 (s, 1H, NH), 7.26 (d, J = 8.5 Hz, 2H, ArH), 7.17 (d, J = 8.5 Hz, 2H, ArH), 5.17 (s, 1H, CH), 3.92 (s, 3H, OCH3), 3.89 (s, 3H, OCH3), 2.46 (s, 2H, CH2), 2.26 (d, J = 16 Hz, 1H, CH2), 2.19 (d, J = 16 Hz, 1H, CH2), 1.09 (s, 3H, CH3), 1.00 (s, 3H, CH3); 13C-NMR (125 MHz, CDCl3) (δ, ppm): 195.0, 168.8, 163.4, 156.5, 150.3, 144.5, 131.8, 129.3, 128.1, 111.6, 95.9, 54.4, 54.1, 50.6, 41.2, 33.8, 32.6, 29.4, 27.3; HRMS(ESI-MS) 400.0996 [M + H]+.
3.2.2. 2,4-Dimethoxy-8,8-dimethyl-5-phenyl-5,8,9,10-tetrahydropyrimido[4,5-b]quinolin-6(7H)-one (4b). White solid, mp 244–246 °C; 1H-NMR (500 MHz, CDCl3) (δ, ppm): 8.05 (s, 1H, NH), 7.31 (d, J = 7.5 Hz, 2H, ArH), 7.20 (t, J = 7.5 Hz, 2H, ArH), 7.11 (t, J = 7.25 Hz, 1H, ArH), 5.21 (s, 1H, CH), 3.91 (s, 3H, OCH3), 3.89 (s, 3H, OCH3), 2.45 (s, 2H, CH2), 2.26 (d, J = 16.5 Hz, 1H, CH2), 2.19 (d, J = 16 Hz, 1H, CH2), 1.09 (s, 3H, CH3), 1.01 (s, 3H, CH3); 13C-NMR (125 MHz, CDCl3) (δ, ppm): 195.0, 168.8, 163.3, 156.5, 149.9, 145.9, 128.0, 127.9, 126.2, 112.1, 96.3, 54.4, 54.1, 50.7, 41.3, 34.2, 32.6, 29.4, 27.3; MS(MM-ES + APCI) 364.20 [M − H]−.
3.2.3. 5-(2-Chlorophenyl)-2,4-dimethoxy-8,8-dimethyl-5,8,9,10-tetrahydropyrimido[4,5-b]quinolin-6(7H)-one (4c). White solid, mp 306–308 °C; 1H-NMR (500 MHz, CDCl3) (δ, ppm): 7.43 (s, 1H, NH), 7.36 (dd, J = 8 Hz, 1H, ArH), 7.25 (dd, J = 8 Hz, 1H, ArH), 7.11 (td, J = 7 Hz, 1H, ArH), 7.04 (td, J = 8 Hz, 1H, ArH), 5.51 (s, 1H, CH), 3.90 (s, 3H, OCH3), 3.83 (s, 3H, OCH3), 2.45–2.35 (m, 2H, CH2), 2.23 (d, J = 16.5 Hz, 1H, CH2), 2.15 (d, J = 16.5 Hz, 1H, CH2), 1.09 (s, 3H, CH3), 1.02 (s, 3H, CH3); 13C-NMR (125 MHz, CDCl3) (δ, ppm): 194.8, 169.0, 163.5, 156.1, 149.8, 142.8, 133.3, 131.7, 129.7, 127.4, 126.3, 54.5, 53.9, 50.5, 41.5, 33.7, 32.5, 29.3, 27.4; HRMS(+ESI) 400.1422 [M + H]+.
3.2.4. 5-(3-Chlorophenyl)-2,4-dimethoxy-8,8-dimethyl-5,8,9,10-tetrahydropyrimido[4,5-b]quinolin-6(7H)-one (4d). White solid, mp 240–242 °C; 1H-NMR (500 MHz, CDCl3) (δ, ppm): 7.56 (s, 1H, NH), 7.23–7.21 (m, 2H, ArH), 7.15–7.09 (m, 2H, ArH), 5.16 (s, 1H, CH), 3.92 (s, 3H, OCH3), 3.90 (s, 3H, OCH3), 2.44 (s, 2H, CH2), 2.26 (d, J = 16 Hz, 1H, CH2), 2.20 (d, J = 16.5 Hz, 1H, CH2), 1.10 (s, 3H, CH3), 1.02 (s, 3H, CH3); 13C-NMR (125 MHz, CDCl3) (δ, ppm): 194.8, 168.9, 163.5, 156.0, 149.7, 147.7, 133.9, 129.2, 127.9, 126.5, 126.3, 111.6, 95.6, 54.7, 54.2, 50.6, 41.4, 34.1, 32.7, 29.2, 27.4; MS(MM-ES + APCI) 398.20 [M − H]+.
3.2.5. 2,4-Dimethoxy-5-(3-methoxyphenyl)-8,8-dimethyl-5,8,9,10-tetrahydropyrimido[4,5-b]quinolin-6(7H)-one (4e). White solid, mp 224–226 °C; 1H-NMR (500 MHz, CDCl3) (δ, ppm): 7.18 (s, 1H, NH), 7.13–6.65 (m, 4H, ArH), 5.18 (s, 1H, CH), 3.90 (s, 3H, OCH3), 3.89 (s, 3H, OCH3), 3.75 (s, 3H, OCH3), 2.44 (d, J = 16.5 Hz, 1H, CH2), 2.39 (d, J = 17 Hz, 1H, CH2), 2.25 (d, J = 16.5 Hz, 1H, CH2), 2.21 (d, J = 16.5 Hz, 1H, CH2), 1.09 (s, 3H, CH3), 1.02 (s, 3H, CH3); 13C-NMR (125 MHz, CDCl3) (δ, ppm): 194.9, 168.8, 163.3, 159.4, 156.1, 149.4, 147.3, 128.9, 120.3, 113.9, 112.1, 111.4, 96.0, 55.1, 54.5, 54.1, 50.6, 41.5, 34.1, 32.7, 29.3, 27.4; MS(MM-ES + APCI) 394.20 [M − H]+.
3.2.6. 2,4-Dimethoxy-5-(4-methoxyphenyl)-8,8-dimethyl-5,8,9,10-tetrahydropyrimido[4,5-b]quinolin-6(7H)-one (4f). White solid, mp 198–200 °C; 1H-NMR (500 MHz, CDCl3) (δ, ppm): 8.60 (s, 1H, NH), 7.23 (d, J = 8.5 Hz, 2H, ArH), 6.74 (d, J = 8.5 Hz, 2H, ArH), 5.16 (s, 1H, CH), 3.91 (s, 3H, OCH3), 3.90 (s, 3H, OCH3), 3.73 (s, 3H, OCH3), 2.46–2.45 (m, 2H, CH2), 2.27–2.18 (m, 2H, CH2), 1.08 (s, 3H, CH3), 1.01 (s, 3H, CH3); 13C-NMR (125 MHz, CDCl3) (δ, ppm): 195.2, 168.7, 163.1, 157.9, 156.5, 150.2, 138.5, 129.3, 128.8, 113.5, 113.4, 112.0, 96.5, 55.1, 54.3, 54.0, 50.7, 41.1, 33.2, 32.5, 29.4, 27.3; MS(MM-ES + APCI) 394.20 [M − H]+.
3.2.7. 5-(2,4-dimethoxyphenyl)-2,4-dimethoxy-8,8-dimethyl-5,8,9,10-tetrahydropyrimido[4,5-b]quinolin-6(7H)-one (4g). White solid, mp 138–140 °C; 1H-NMR (500 MHz, CDCl3) (δ, ppm): 8.33 (s, 1H, NH), 7.30 (d, J = 8.5 Hz, 1H, ArH), 6.39 (dd, J = 8.5 Hz, 1H, ArH), 6.35 (d, J = 2 Hz, 1H, ArH), 5.24 (s, 1H, CH), 3.89 (s, 3H, OCH3), 3.85 (s, 3H, OCH3), 3.75 (s, 3H, OCH3), 3.71 (s, 3H, OCH3), 2.42 (d, J = 17 Hz, 1H, CH2), 2.35 (d, J = 16.5 Hz, 1H, CH2), 2.21 (d, J = 16.5 Hz, 1H, CH2), 2.13 (d, J = 16.5 Hz, 1H, CH2), 1.07 (s, 3H, CH3), 0.97 (s, 3H, CH3); 13C-NMR (125 MHz, CDCl3) (δ, ppm): 195.1, 168.6, 162.9, 159.2, 158.5, 156.9, 150.3, 131.6, 126.0, 110.5, 103.7, 98.6, 95.7, 55.2, 55.2, 54.3, 53.9, 50.7, 41.3, 32.5, 31.5, 29.6, 26.7; HRMS(+ESI) 426.2021 [M + H]+.
3.2.8. 5-(3,4-dimethoxyphenyl)-2,4-dimethoxy-8,8-dimethyl-5,8,9,10-tetrahydropyrimido[4,5-b]quinolin-6(7H)-one (4h). White solid, mp 208–210 °C; 1H-NMR (500 MHz, CDCl3) (δ, ppm): 8.46 (s, 1H, NH), 6.97–6.70 (m, 3H, ArH), 5.17 (s, 1H, CH), 3.921 (s, 3H, OCH3), 3.916 (s, 3H, OCH3), 3.84 (s, 3H, OCH3), 3.80 (s, 3H, OCH3), 2.47 (s, 2H, CH2), 2.27 (d, J = 16.5 Hz, 1H, CH2), 2.22 (d, J = 16.5 Hz, 1H, CH2), 1.09 (s, 3H, CH3), 1.03 (s, 3H, CH3); 13C-NMR (125 MHz, CDCl3) (δ, ppm): 195.2, 168.8, 163.1, 156.5, 150.1, 148.4, 147.4, 138.9, 119.5, 112.0, 111.7, 110.8, 96.4, 55.8, 55.7, 54.4, 54.1, 50.6, 41.2, 33.6, 32.5, 29.6, 27.2; HRMS(+ESI) 426.2026 [M + H]+.
3.2.9. 5-(2,5-dimethoxyphenyl)-2,4-dimethoxy-8,8-dimethyl-5,8,9,10-tetrahydropyrimido[4,5-b]quinolin-6(7H)-one (4i). White solid, mp 206–208 °C; 1H-NMR (500 MHz, CDCl3) (δ, ppm): 7.26, 7.21 (s, 2H, NH + CDCl3), 6.95 (d, J = 3 Hz 1H, ArH), 6.71 (d, J = 9 Hz, 1H, ArH), 6.65–6.63 (m, H, ArH), 5.28 (s, 1H, CH), 3.89 (s, 3H, OCH3), 3.83 (s, 3H, OCH3), 3.75 (s, 3H, OCH3), 3.71 (s, 3H, OCH3), 2.42 (d, J = 17 Hz, 1H, CH2), 2.30 (d, J = 17 Hz, 1H, CH2), 2.21 (d, J = 16.5 Hz, 1H, CH2), 2.14 (d, J = 16.5 Hz, 1H, CH2), 1.08 (s, 3H, CH3), 0.99 (s, 3H, CH3); 13C-NMR (125 MHz, CDCl3) (δ, ppm): 194.8, 168.7, 163.1, 153.1, 152.1, 134.4, 117.2, 112.0, 111.9, 110.7, 95.4, 56.2, 55.6, 54.4, 54.0, 50.6, 41.59, 32.55, 32.0, 29.4, 27.0; HRMS(+ESI) 426.2020 [M + H]+.
3.2.10. 5-(4-ethoxyphenyl)-2,4-dimethoxy-8,8-dimethyl-5,8,9,10-tetrahydropyrimido[4,5-b]quinolin-6(7H)-one (4j). White solid, mp 192–194 °C; 1H-NMR (500 MHz, CDCl3) (δ, ppm): 8.49 (s, 1H, NH), 7.21 (d, J = 9 Hz, 2H, ArH), 6.73 (d, J = 8.5 Hz, 2H, ArH), 5.15 (s, 1H, CH), 3.97–3.92 (m, 2H, CH2), 3.91 (s, 3H, OCH3), 3.89 (s, 3H, OCH3), 2.46–2.44 (m, 2H, CH2), 2.27–2.18 (m, 2H, CH2), 1.35 (t, J = 7 Hz, 3H, CH3), 1.08 (s, 3H, CH3), 1.00 (s, 3H, CH3); 13C-NMR (125 MHz, CDCl3) (δ, ppm): 195.2, 168.7, 163.1, 157.4, 157.3, 156.5, 150.1, 138.3, 129.3, 128.8, 113.9, 112.1, 96.5, 63.2, 54.3, 54.0, 50.7, 41.1, 33.2, 32.5, 29.4, 27.3, 14.9; HRMS(+ESI) 410.2076 [M + H]+.
3.2.11. 5-(3-hydroxyphenyl)-2,4-dimethoxy-8,8-dimethyl-5,8,9,10-tetrahydropyrimido[4,5-b]quinolin-6(7H)-one (4k). Light brown solid, mp 292–294 °C; 1H-NMR (500 MHz, CDCl3 and DMSO-d6) (δ, ppm): 9.73 (s, 1H, NH), 8.89 (s, 1H, OH), 6.95 (t, J = 8 Hz, 1H, ArH), 6.70–6.68 (m, 2H, ArH), 6.53–6.51 (m, 1H, ArH), 5.01 (s, 1H, CH), 3.88 (s, 3H, OCH3), 3.87 (s, 3H, OCH3), 2.47 (s, 2H, CH2), 2.19 (d, J = 16 Hz, 1H, CH2), 2.10 (d, J = 16 Hz, 1H, CH2), 1.07 (s, 3H, CH3), 1.00 (s, 3H, CH3); 13C-NMR (125 MHz, CDCl3 and DMSO-d6) (δ, ppm): 194.5, 168.4, 163.2, 157.1, 156.8, 151.5, 147.9, 128.8, 118.7, 114.5, 113.2, 110.7, 95.8, 54.5, 53.9, 50.8, 33.8, 32.5, 29.4, 27.4; HRMS(+ESI) 382.1793 [M + H]+.
3.2.12. 5-([1,1′-biphenyl]-4-yl)-2,4-dimethoxy-8,8-dimethyl-5,8,9,10-tetrahydropyrimido[4,5-b]quinolin-6(7H)-one (4l). White solid, mp 246–248 °C; 1H-NMR (500 MHz, CDCl3) (δ, ppm): 8.06 (s, 1H, NH), 7.53 (d, J = 7 Hz, 2H, ArH), 7.44 (d, J = 8.5 Hz, 2H, ArH), 7.40–7.37 (m, 4H, ArH), 7.30–7.26 (m, 1H, ArH), 5.25 (s, 1H, CH), 3.920 (s, 3H, OCH3), 3.915 (s, 3H, OCH3), 2.47 (s, 2H, CH2), 2.27 (d, J = 16.5 Hz, 1H, CH2), 2.22 (d, J = 16.5 Hz, 1H, CH2), 1.10 (s, 3H, CH3), 1.03 (s, 3H, CH3); 13C-NMR (125 MHz, CDCl3) (δ, ppm): 195.1, 168.9, 163.3, 156.4, 150.1, 145.0, 141.1, 139.0, 128.6, 128.4, 127.0, 126.9, 126.8, 112.0, 96.2, 54.5, 54.2, 50.7, 41.3, 33.9, 32.7, 29.4, 27.4; HRMS(+ESI) 442.2121 [M + H]+.
3.2.13. 2,4-Dimethoxy-8,8-dimethyl-5-(p-tolyl)-5,8,9,10-tetrahydropyrimido[4,5-b]quinoline-6(7H)-one (4m). White solid, mp 208–210 °C; 1H-NMR (500 MHz, CDCl3) (δ, ppm): 8.68 (s, 1H, NH), 7.21 (d, J = 8 Hz, 2H, ArH), 7.01 (d, J = 8 Hz, 2H, ArH), 5.19 (s, 1H, CH), 3.901 (s, 3H, OCH3), 3.897 (s, 3H, OCH3), 2.49–2.42 (m, 2H, CH2), 2.26 (d, J = 14.5 Hz, 1H, CH2), 2.23 (d, J = 15.5 Hz, 1H, CH2), 1.08 (s, 3H, CH3), 1.01 (s, 3H, CH3); 13C-NMR (125 MHz, CDCl3) (δ, ppm): 195.2, 168.7, 163.1, 156.6, 150.4, 143.1, 135.6, 128.8, 127.7, 112.0, 96.5, 54.3, 54.1, 50.7, 41.1, 33.7, 32.6, 29.5, 27.4, 21.2; HRMS(+ESI) 380.1969 [M + H]+.
3.2.14. 5-(4-fluorophenyl)-2,4-dimethoxy-8,8-dimethyl-5,8,9,10-tetrahydropyrimido[4,5-b]quinolin-6(7H)-one (4n). White solid, mp 200–202 °C; 1H-NMR (500 MHz, CDCl3) (δ, ppm): 8.47 (s, 1H, NH), 7.29–7.26 (m, 2H, ArH), 6.91–6.86 (m, 2H, ArH), 5.18 (s, 1H, CH), 3.92 (s, 3H, OCH3), 3.90 (s, 3H, OCH3), 2.47 (s, 2H, CH2), 2.26 (d, J = 16 Hz, 1H, CH2), 2.20 (d, J = 16 Hz, 1H, CH2), 1.09 (s, 3H, CH3), 1.00 (s, 3H, CH3); 13C-NMR (125 MHz, CDCl3) (δ, ppm): 195.1, 168.8, 162.8 (d, J = 127.9 Hz), 160.3, 156.5, 150.2, 141.8 (d, J = 2.9 Hz), 129.3 (d, J = 7.8 Hz), 114.7 (d, J = 21.2 Hz), 111.9, 96.2, 54.4, 54.1, 50.6, 41.2, 33.6, 32.6, 29.4, 27.3; HRMS(+ESI) 384.1719 [M + H]+.
3.2.15. 5-(4-bromophenyl)-2,4-dimethoxy-8,8-dimethyl-5,8,9,10-tetrahydropyrimido[4,5-b]quinolin-6(7H)-one (4o). Light brown solid, mp 214–216 °C; 1H-NMR (500 MHz, CDCl3) (δ, ppm): 8.47 (s, 1H, NH), 7.32 (d, J = 8.5 Hz, 2H, ArH), 7.20 (d, J = 7.5 Hz, 2H, ArH), 5.16 (s, 1H, CH), 3.92 (s, 3H, OCH3), 3.89 (s, 3H, OCH3), 2.46 (s, 2H, CH2), 2.26 (d, J = 16.5 Hz, 1H, CH2), 2.19 (d, J = 16 Hz, 1H, CH2), 1.09 (s, 3H, CH3), 1.00 (s, 3H, CH3); 13C-NMR (125 MHz, CDCl3) (δ, ppm): 195.0, 168.8, 163.4, 156.5, 150.3, 145.0, 131.1, 129.7, 120.0, 111.5, 95.8, 54.4, 54.1, 50.6, 41.2, 33.9, 32.6, 29.4, 27.3; MS(MM-ES + APCI) 442.20 [M − H]−.
3.2.16. 2,4-Dimethoxy-8,8-dimethyl-5-(4-nitrophenyl)-5,8,9,10-tetrahydropyrimido[4,5-b]quinolin-6(7H)-one (4p). Light yellow solid, mp 234–236 °C; 1H-NMR (500 MHz, CDCl3) (δ, ppm): 8.87 (s, 1H, NH), 8.09 (d, J = 8.5 Hz, 2H, ArH), 7.50 (d, J = 8.5 Hz, 2H, ArH), 5.29 (s, 1H, CH), 3.94 (s, 3H, OCH3), 3.90 (s, 3H, OCH3), 2.53 (s, 2H, CH2), 2.28 (d, J = 16 Hz, 1H, CH2), 2.19 (d, J = 16.5 Hz, 1H, CH2), 1.11 (s, 3H, CH3), 1.00 (s, 3H, CH3); 13C-NMR (125 MHz, CDCl3) (δ, ppm): 195.0, 168.8, 163.6, 156.6, 153.2, 151.1, 146.3, 128.9, 123.4, 110.7, 95.0, 54.6, 54.3, 50.1, 41.1, 34.8, 32.6, 29.4, 27.2; HRMS(+ESI) 411.1660 [M + H]+.
3.2.17. 2,4-Dimethoxy-8,8-dimethyl-5-(2-nitrophenyl)-5,8,9,10-tetrahydropyrimido[4,5-b]quinolin-6(7H)-one (4q). Light yellow solid, mp 270–272 °C; 1H-NMR (500 MHz, CDCl3) (δ, ppm): 7.79 (s, 1H, NH), 7.78–7.77 (m, 1H, ArH), 7.43–7.35 (m, 2H, ArH), 7.24–7.22 (m, 1H, ArH), 6.03 (s, 1H, CH), 3.91 (s, 3H, OCH3), 3.82 (s, 3H, OCH3), 2.47–2.43 (m, 2H, CH2), 2.25 (d, J = 16.5 Hz, 1H, CH2), 2.16 (d, J = 16.5 Hz, 1H, CH2), 1.10 (s, 3H, CH3), 1.01 (s, 3H, CH3); 13C-NMR (125 MHz, CDCl3) (δ, ppm): 194.7, 169.0, 163.6, 156.0, 150.3, 148.8, 140.2, 132.4, 130.8, 126.9, 124.2, 111.3, 95.3, 54.6, 54.2, 50.4, 41.4, 32.6, 30.2, 29.1, 27.6; HRMS(+ESI) 411.1660 [M + H]+.
3.2.18. 2,4-Dimethoxy-8,8-dimethyl-5-(2-nitrophenyl)-5,8,9,10-tetrahydropyrimido[4,5-b]quinolin-6(7H)-one (4r). Off white solid, mp 236–238 °C; 1H-NMR (500 MHz, CDCl3) (δ, ppm): 8.38 (s, 1H, NH), 8.13 (s, 1H, ArH), 7.99 (d, J = 8 Hz, 1H, ArH), 7.74 (d, J = 7.5 Hz, 1H, ArH), 7.40 (t, J = 8 Hz, 1H, ArH), 5.30 (s, 1H, CH), 3.94 (s, 3H, OCH3), 3.90 (s, 3H, OCH3), 2.52 (s, 2H, CH2), 2.28 (d, J = 16.5 Hz, 1H, CH2), 2.19 (d, J = 16.5 Hz, 1H, CH2), 1.11 (s, 3H, CH3), 1.02 (s, 3H, CH3); 13C-NMR (125 MHz, CDCl3) (δ, ppm): 195.0, 168.8, 163.6, 156.3, 150.8, 148.3, 147.9, 134.5, 128.8, 122.8, 121.5, 110.9, 95.2, 54.6, 54.3, 50.5, 41.2, 34.6, 32.6, 29.4, 27.3; HRMS(+ESI) 411.1660 [M + H]+.
3.2.19. 2,4-Dimethoxy-8,8-dimethyl-5-(naphthalen-1-yl)-5,8,9,10-tetrahydropyrimido[4,5-b]quinolin-6(7H)-one (4s). Off white solid, mp 180–182 °C; 1H-NMR (500 MHz, CDCl3) (δ, ppm): 8.79 (s, 1H, NH), 8.56–8.36 (m, 1H, ArH), 6.73 (d, J = 8.5 Hz, 2H, ArH), 5.15 (s, 1H, CH), 3.97–3.92 (m, 2H, CH2), 3.91 (s, 3H, OCH3), 3.89 (s, 3H, OCH3), 2.46–2.44 (m, 2H, CH2), 2.27–2.18 (m, 2H, CH2), 1.35 (t, J = 7 Hz, 3H, CH3), 1.08 (s, 3H, CH3), 1.00 (s, 3H, CH3); 13C-NMR (125 MHz, CDCl3) (δ, ppm): 195.3, 169.0, 163.0, 156.2, 133.4, 131.1, 128.1, 127.0, 126.7, 125.5, 125.3, 125.0, 113.4, 97.5, 54.4, 53.8, 50.5, 41.1, 32.5, 29.7, 29.4, 27.3; HRMS(+ESI) 415.2121 [M + NH4]+[−H2O].
3.2.20. 5-(6-bromobenzo[d][1,3]dioxol-5-yl)-2,4-dimethoxy-8,8-dimethyl-5,8,9,10-tetrahydropyrimido[4,5-b]quinolin-6(7H)-one (4t). White solid, mp 296–298 °C; 1H-NMR (500 MHz, CDCl3) (δ, ppm): 7.40 (s, 1H, NH), 6.92 (s, 1H, ArH), 6.75 (s, 1H, ArH), 7.74 (d, J = 7.5 Hz, 1H, ArH), 5.88 (dd, J = 8 Hz, 2H, OCH2O), 5.43 (s, 1H, CH), 3.91 (s, 3H, OCH3), 3.87 (s, 3H, OCH3), 2.45–2.37 (m, 2H, CH2), 2.24 (d, J = 16.5 Hz, 1H, CH2), 2.18 (d, J = 16.5 Hz, 1H, CH2), 1.10 (s, 3H, CH3), 1.05 (s, 3H, CH3); 13C-NMR (125 MHz, CDCl3) (δ, ppm): 195.0, 169.1, 163.4, 155.8, 149.6, 147.2, 146.5, 114.0, 112.5, 111.7, 110.4, 101.5, 95.7, 54.5, 53.9, 50.6, 41.5, 35.5, 32.6, 29.1, 27.7; HRMS(+ESI) 488.0807 [M + H]+.
3.2.21. 5-(2-Butyl-5-chloro-1H-imidazole-4-yl)-2,4-dimethoxy-8,8-dimethyl-5,8,9,10-tetrahydropyrimido[4,5-b]quinolin-6(7H)-one (4u). Light brown solid, mp 212–214 °C; 1H-NMR (500 MHz, CDCl3) (δ, ppm): 9.91 (s, 1H, NH), 8.00 (s, 1H, NH), 5.22 (s, 1H, CH), 3.94 (s, 3H, OCH3), 3.90 (s, 3H, OCH3), 2.58 (td, J = 7.5 Hz, 2H, CH2), 2.43 (d, J = 16.5 Hz, 1H, CH2), 2.38 (d, J = 17 Hz, 1H, CH2), 2.29 (d, J = 16.5 Hz, 1H, CH2), 2.25 (d, J = 16.5 Hz, 1H, CH2), 1.64 (quin, J = 7.5 Hz, 2H, CH2), 1.32 (sextet, J = 7.5 Hz, 2H, CH2),1.10 (s, 3H, CH3), 1.03 (s, 3H, CH3), 0.90 (t, J = 7.5 Hz, 3H, CH3); 13C-NMR (125 MHz, CDCl3) (δ, ppm): 196.8, 169.1, 163.7, 156.5, 151.6, 145.3, 126.3, 124.1, 108.7, 91.9, 54.6, 54.3, 50.5, 41.21, 32.7, 30.2, 28.9, 28.3, 27.6, 25.8, 22.3, 13.8; HRMS(+ESI) 446.1946 [M + H]+.
3.2.22. 5-(5-Bromothiophen-2-yl)-2,4-dimethoxy-8,8-dimethyl-5,8,9,10-tetrahydropyrimido[4,5-b]quinolin-6(7H)-one (4v). Off white solid, mp 206–208 °C; 1H-NMR (500 MHz, CDCl3) (δ, ppm): 8.50 (s, 1H, NH), 6.76 (d, J = 3.5 Hz, 1H, ArH), 6.57 (dd, J = 3.5 Hz, 1H, ArH), 5.49 (s, 1H, CH), 3.98 (s, 3H, OCH3), 3.94 (s, 3H, OCH3), 2.48 (d, J = 17 Hz, 1H, CH2), 2.44 (d, J = 17 Hz, 1H, CH2), 2.30 (s, 2H, CH2), 1.11 (s, 3H, CH3), 1.09 (s, 3H, CH3); 13C-NMR (125 MHz, CDCl3) (δ, ppm): 195.0, 168.8, 163.4, 156.4, 151.0, 150.8, 129.3, 123.9, 110.6, 109.8, 94.8, 54.5, 54.2, 50.5, 41.1, 32.5, 29.3, 29.3, 27.5; HRMS(+ESI) 451.0624 [M + NH4]+[−H2O].
3.3. Antiproliferative activity
The antiproliferative activity was measured using our implementation of the National Cancer Institute (NCI) screening protocol.37 We used the following human solid tumour cell lines: A549 (non-small cell lung), HBL-100 (breast), HeLa (cervix), SW1573 (non-small cell lung), T-47D (breast), and WiDr (colon). Cell seeding densities were 2500 (A549, HBL-100, HeLa, and SW1573) or 5000 (T-47D and WiDr) cells per well. Tested compounds were dissolved in DMSO at an initial concentration of 40 mM. DMSO was used as negative control (0.25% v/v). The results were expressed as GI50, i.e. the dose that causes 50% growth inhibition after 48 h of exposure.
3.4. ADMET prediction
In silico ADMET prediction of potent antiproliferative 2,4-dimethoxy-THPQs 4h, 4o, 4q and 4v were calculated by web tool SwissADME (http://www.swissadme.ch/).38
4. Conclusion
In conclusion, we established MWI facilitated green synthetic protocol for a series of 2,4-dimethoxy-THPQs from multicomponent reaction between aldehyde 1, dimedone 2 and 6-amino-2,4-dimethoxypyrimidine 3 using glacial acetic acid. The main features of this green synthetic protocol are the use of commercially available cheap starting materials, metal-free multicomponent synthesis, short reaction time, higher product yield and no need to column chromatography for product purification. Biological screening showed that the compounds are able to inhibit proliferation of human tumour cells at the micromolar range. The SAR study was not able to provide information for the future design of new analogues. In silico ADMET prediction of antiproliferative 2,4-dimethoxy-THPQs 4h, 4o, 4q and 4v show good bioavailability radar plot. Furthermore, BOILED-Egg delineation of 4h, 4o, 4q and 4v show that 4o have BBB permeability while other show high intestinal absorption. All these also have drug-like properties. Further biological studies will be necessary to identify the cellular targets involved in the biological effects.
Conflicts of interest
The authors declare no conflict of interest.
Acknowledgements
SGP, RMV, PJP and HMP are grateful to the Department of Chemistry, Sardar Patel University for providing lab facilities. SGP is grateful to the UGC, New Delhi for a UGC-JRF (NTA Ref. No.: 201610157514; dated: 01.04.2021). A. G.-B., A. P. and J. M. P. thank the Canary Islands Government (ProID2020010101, ACIISI/FEDER, UE) for financial support. A. P. thanks the EU Social Fund (FSE) and the Canary Islands ACIISI for a predoctoral grant TESIS2020010055. All authors are thankful to Manoj Mangukiya, Associate Analytical Scientist, Aether Industries Ltd, Surat, for the LCMS experiments.
References
- V. A. Katzke, R. Kaaks and T. Kühn, Cancer J., 2015, 21 Search PubMed.
- R. M. Vala, M. G. Sharma, D. M. Patel, A. Puerta, J. M. Padrón, V. Ramkumar, R. L. Gardas and H. M. Patel, Arch. Pharm., 2021, 354, 2000466 CrossRef CAS.
- D. M. Patel, M. G. Sharma, R. M. Vala, I. Lagunes, A. Puerta, J. M. Padrón, D. P. Rajani and H. M. Patel, Bioorg. Chem., 2019, 86, 137–150 CrossRef CAS PubMed.
- B. Jiang, F. Shi and S.-J. Tu, Curr. Org. Chem., 2010, 14, 357–378 CrossRef CAS.
- S. Gorle, S. Maddila, S. N Maddila, K. Naicker, M. Singh, P. Singh and S. B Jonnalagadda, Anticancer Agents Med Chem., 2017, 17, 464–470 CrossRef CAS.
- S. Moloi, S. Maddila and S. B. Jonnalagadda, Res. Chem. Intermed., 2017, 43, 6233–6243 CrossRef CAS.
- E. S. H. El Ashry, E. Ramadan, A. A. Kassem and M. Hagar, Adv. Heterocycl. Chem., 2005, 88, 1–110 CrossRef.
- Y. Gu, Green Chem., 2012, 14, 2091–2128 RSC.
- S. I. Alqasoumi, A. M. Al-Taweel, A. M. Alafeefy, E. Noaman and M. M. Ghorab, Eur. J. Med. Chem., 2010, 45, 738–744 CrossRef CAS.
- A. A. Abu-Hashem and A. S. Aly, Arch. Pharmacal Res., 2012, 35, 437–445 CrossRef CAS.
- M. B. El-Ashmawy, M. A. El-Sherbeny and N. S. El-Gohary, Med. Chem. Res., 2013, 22, 2724–2736 CrossRef CAS.
- M. M. Ghorab, F. A. Ragab, H. I. Heiba and W. M. Ghorab, J. Heterocycl. Chem., 2011, 48, 1269–1279 CrossRef CAS.
- R. A. Mekheimer, S. M. R. Allam, M. A. Al-Sheikh, M. S. Moustafa, S. M. Al-Mousawi, Y. A. Mostafa, B. G. M. Youssif, H. A. M. Gomaa, A. M. Hayallah, M. Abdelaziz and K. U. Sadek, Bioorg. Chem., 2022, 121, 105693 CrossRef CAS PubMed.
- M. R. Selim, M. A. Zahran, A. Belal, M. S. Abusaif, S. A. Shedid, A. B. M. Mehany, G. A. M. Elhagali and Y. A. Ammar, Anti-Cancer Agents Med. Chem., 2019, 19, 439–452 CrossRef CAS.
- E. Tieger, V. Kiss, G. Pokol, Z. Finta, J. Rohlíček, E. Skořepová and M. Dušek, CrystEngComm, 2016, 18, 9260–9274 RSC.
- S. Bedi, S. A. Khan, M. M. AbuKhader, P. Alam, N. A. Siddiqui and A. Husain, Saudi Pharm. J., 2018, 26, 755–763 CrossRef.
- J. Wan, Y. Qiao, X. Chen, J. Wu, L. Zhou, J. Zhang, S. Fang and H. Wang, Adv. Funct. Mater., 2018, 28, 1804229 CrossRef.
- K. Montazeri and J. Bellmunt, Expert Rev. Clin. Pharmacol., 2020, 13, 1–6 CrossRef CAS PubMed.
- A. Markham, Drugs, 2020, 80, 1345–1353 CrossRef CAS PubMed.
- A. K. Larsen, C. M. Galmarini and M. D'Incalci, Cancer Chemother. Pharmacol., 2016, 77, 663–671 CrossRef CAS.
- S. G. Patel, R. M. Vala, P. J. Patel, D. B. Upadhyay, V. Ramkumar, R. L. Gardas and H. M. Patel, RSC Adv., 2022, 12, 18806–18820 RSC.
- A. Sharma, P. Appukkuttan and E. Van der Eycken, Chem. Commun., 2012, 48, 1623–1637 RSC.
- H. R. Prakash Naik, H. S. Bhojya Naik, T. R. Ravikumar Naik, H. R. Naik, D. S. Lamani and T. Aravinda, J. Sulfur Chem., 2008, 29, 583–592 CrossRef.
- J. Quiroga, J. Trilleras, B. Insuasty, R. Abonía, M. Nogueras, A. Marchal and J. Cobo, Tetrahedron Lett., 2010, 51, 1107–1109 CrossRef CAS.
- M. H. Mosslemin, E. Zarenezhad, N. Shams, M. N. S. Rad, H. Anaraki-Ardakani and R. Fayazipoor, J. Chem. Res., 2014, 38, 169–171 CrossRef CAS.
- V. Tandon, R. M. Vala, A. Chen, R. L. Sah, H. M. Patel, M. C. Pirrung and S. Banerjee, Biosci. Rep., 2022, 42, BSR20212721 CrossRef CAS.
- M. G. Sharma, J. Pandya, D. M. Patel, R. M. Vala, V. Ramkumar, R. Subramanian, V. K. Gupta, R. L. Gardas, A. Dhanasekaran and H. M. Patel, Polycyclic Aromat. Compd., 2021, 41, 1495–1505 CrossRef CAS.
- H. M. Patel, D. P. Rajani, M. G. Sharma and H. G. Bhatt, Lett. Drug Des. Discovery, 2019, 16, 119–126 CrossRef CAS.
- D. M. Patel, R. M. Vala, M. G. Sharma, D. P. Rajani and H. M. Patel, ChemistrySelect, 2019, 4, 1031–1041 CrossRef CAS.
- H. M. Patel, Curr. Bioact. Compd., 2018, 14, 278–288 CrossRef CAS.
- M. G. Sharma, R. M. Vala, D. M. Patel, I. Lagunes, M. X. Fernandes, J. M. Padrón, V. Ramkumar, R. L. Gardas and H. M. Patel, ChemistrySelect, 2018, 3, 12163–12168 CrossRef CAS.
- H. M. Patel, Green Sustainable Chem., 2015, 5, 137 CrossRef CAS.
- D. M. Patel, R. M. Vala, M. G. Sharma, D. P. Rajani and H. M. Patel, ChemistrySelect, 2019, 4, 1031–1041 CrossRef CAS.
- J. Jin, J. Zhang, F. Liu, W. Shang, Y. Xin and S. Zhu, Chin. J. Chem., 2010, 28, 1217–1222 CrossRef CAS.
- J. A. Mokariya, A. G. Kalola, P. Prasad and M. P. Patel, Mol. Diversity, 2022, 26, 963–979 CrossRef CAS PubMed.
- A. Daina and V. Zoete, ChemMedChem, 2016, 11, 1117–1121 CrossRef CAS.
- I. Lagunes, E. Martín-Batista, G. Silveira-Dorta, M. X. Fernandes and J. M. Padrón, J. Mol. Clin. Med., 2018, 1, 77–84 Search PubMed.
- A. Daina, O. Michielin and V. Zoete, Sci. Rep., 2017, 7, 42717 CrossRef.
|
This journal is © The Royal Society of Chemistry 2022 |
Click here to see how this site uses Cookies. View our privacy policy here.