DOI:
10.1039/D2RA04759D
(Paper)
RSC Adv., 2022,
12, 33459-33468
Investigation of Fe3O4@boehmite NPs as efficient and magnetically recoverable nanocatalyst in the homoselective synthesis of tetrazoles†
Received
29th July 2022
, Accepted 12th November 2022
First published on 22nd November 2022
Abstract
Magnetic boehmite nanoparticles (Fe3O4@boehmite NPs) were synthesized from a hybrid of boehmite and Fe3O4 nanoparticles. At first, boehmite nanoparticles (aluminum oxide hydroxide) were prepared via a simple procedure in water using commercially available materials such as sodium hydroxide and aluminum nitrate. Then, these nanoparticles were magnetized using Fe3O4 NPs in a basic solution of FeCl2·4H2O and FeCl3·6H2O. Fe3O4@boehmite NPs have advantages of both boehmite nanoparticles and Fe3O4 magnetic materials. Magnetic boehmite nanoparticles have been characterized by various techniques such as TEM, SEM, EDS, WDX, ICP, FT-IR, Raman, XRD and VSM. SEM and TEM images confirmed that particles size are less than 50 nm in diameter with a cubic orthorhombic structure. Then, Fe3O4@boehmite NPs were applied as a homoselective, highly efficient, cheap, biocompatibility, heterogeneous and magnetically recoverable nanocatalyst in the synthesis of 5-substituted 1H-tetrazole derivatives. Fe3O4@boehmite NPs can be recycled for several runs in the synthesis of tetrazoles. Also, all tetrazoles were isolated in high yields, which reveals high activity of Fe3O4@boehmite NPs in the synthesis of tetrazole derivatives. Fe3O4@boehmite NPs shows a good homoselectivity in synthesis of 5-substituted 1H-tetrazole derivatives.
1 Introduction
In homogeneous catalyst systems, good catalytic activity is usually observed, due to the solubility of the catalyst in the reaction mixture and thus the easy access of the reactants to the catalyst sites. Therefore homogeneous catalysts perform better practicality than heterogeneous catalysts.1–3 However, in the homogeneous catalyst systems, purification of the products, separation and recovery of the catalyst is often difficult, costly and time consuming. Therefore, this drawbacks have limited the use of the homogeneous catalysts despite high activity and selectivity.4 Also, clean technology and green chemistry require the use of heterogeneous and recyclable catalysts.3–6 While we need to catalytic systems which have high activity and recyclability. This goal is achieved through the use of nanocatalysts which are the bridge between homogeneous and heterogeneous catalysts.7,8 However, nanoparticles (NPs) are not fully recycled by conventional and inexpensive methods such as centrifugation or filtration due to their very small size. All of these problems can be overcome using magnetic nanocatalysts or stabilizing catalyst species on magnetic substrates.8–10 In the absence of an external magnetic field, the magnetic nanoparticles (MNPs) are dispersed in the reaction mixture and make available high surface to the reacting molecules. More important, at the end of the reaction, they are quickly, easily and completely recovered from the reaction mixture using an external magnet.11–16 But these MNPs are not very stable for long times.17 Therefore, many organic or inorganic covers have been used to increase the MNPs stability.18–21 One of the most valuable and cheapest mineral compounds which is rarely used as MNPs cover is boehmite nanoparticles (BNPs) which are synthesized using inexpensive and available materials and very simple method in the aqueous environment.22 Boehmite is actually one of the polymorphs phases of aluminum oxide which called aluminum oxyhydroxide (AlOOH).23–27 BNPs with high surface area have high hydroxyl groups on their surface that causes it to be used as a coating.28,29 Therefore, magnetic boehmite nanoparticles (Fe3O4@boehmite NPs) have advantages of homogeneous catalyst (such as large surface area and activity), high stability (such as boehmite NPs) and MNPs (such as easily and magnetically separation using an external magnet). For this purpose, in this project, Fe3O4@boehmite NPs were synthesized and used as a highly efficient, environmentally friendly and magnetically reusable nanocatalyst in the synthesis of 5-substituted 1H-tetrazoles. The procedure synthesis of Fe3O4@boehmite NPs is cheap, simple and environmentally friendly. 5-Substituted tetrazoles were used in drugs and they are used as herbicides, anti-HIV drug candidates, analgesics, antimicrobial, anti-proliferative, anti-inflammatory, and anticancer agents.26,30–38 For example, Candesartan, Valsartan, Irbesartan, Losartan, Cilostazol, TAK-456, Pemiroplast and Pranlukast (Fig. 1) are several pharmacologically important of tetrazoles.39–41
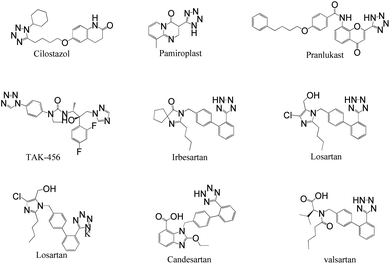 |
| Fig. 1 Several pharmacologically compounds of tetrazoles. | |
2 Experimental
2.1 Preparation of Fe3O4@boehmite NPs catalyst
The BNPs were synthesized according to previously reported procedure.44 Then, the obtained BNPs (2 g) dispersed in water at 80 °C, and then FeCl2·4H2O (7.5 mmol, 1.49 g) and FeCl3·6H2O (11.5 mmol, 3.1 g) added to the mixture. The reaction mixture stirred under N2 atmosphere. Under continuous stirring, NaOH (50 mL, 10%) added into the reaction mixture. The obtained mixture stirred for 2 h at 90 °C which black Fe3O4@boehmite NPs prepared. The obtained Fe3O4@boehmite NPs washed with distilled water and separated by external magnet each time.
2.2 General procedure for synthesis of tetrazoles catalyzed by Fe3O4@boehmite NPs
A mixture of sodium azide (1.5 mmol) and benzonitrile derivative (1 mmol) stirred in the presence of Fe3O4@boehmite NPs catalyst (0.015 g) in PEG at 120 °C. Reaction times controlled by TLC. After completion of the reaction, the Fe3O4@boehmite NPs catalyst isolated using an external magnet and the tetrazole products extracted by ethyl acetate and aqueous solution of HCl (4 N). The organic solvent dried over anhydrous Na2SO4, and evaporated to give the tetrazole products.
2.3 Selected spectral data
2.3.1 2-(1H-Tetrazol-5-yl)benzonitrile. 1H NMR (400 MHz, CDCl3): δH = 8.11–8.06 (t, J = 8 Hz, 2H), 7.96–7.90 (t, J = 12 Hz, 1H), 7.81–7.75 (t, J = 20 Hz, 1H) ppm.
2.3.2 5-(3-Nitrophenyl)-1H-tetrazole. 1H NMR (400 MHz, CDCl3): δH = 14.96 (br, 1H), 8.84–8.83 (t, J = 4 Hz, 1H), 8.49–8.39 (m, 2H), 7.93–7.88 (t, J = 8 Hz, 1H) ppm.
2.3.3 5-(4-Bromophenyl)-1H-tetrazole. 1H NMR (400 MHz, CDCl3): δH = 7.98–7.96 (d, J = 8 Hz, 2H), 7.84–7.82 (d, J = 8 Hz, 2H) ppm.
2.3.4 5-(4-Nitrophenyl)-1H-tetrazole. 1H NMR (400 MHz, CDCl3): δH = 14.76 (br, 1H), 8.45–8.41 (d, J = 12 Hz, 2H), 8.30–8.27 (d, J = 12 Hz, 2H) ppm.
3 Results and discussion
In this project, to combine the advantages of both MNPs and boehmite nanoparticles, magnetic boehmite nanoparticles (Fe3O4@boehmite NPs) synthesized using Fe3O4 nanoparticles and available materials through environmentally friendly and very simple procedure in aqueous media. In the next step, Fe3O4@boehmite NPs characterized by transmission electron microscopy (TEM), scanning electron microscopy (SEM), wavelength dispersive X-ray spectroscopy (WDX), energy-dispersive X-ray spectroscopy (EDS), Inductively coupled plasma (ICP), Fourier transform infrared spectroscopy (FT-IR), Raman spectroscopy, X-ray diffraction (XRD) and vibrating-sample magnetometer (VSM) techniques. The scanning electron microscope was used to obtain high-resolution SEM images of Fe3O4@boehmite NPs. The SEM images were used to studying the morphology and diameter size of Fe3O4@boehmite NPs. SEM images of Fe3O4@boehmite NPs are show in Fig. 2 which indicate particles size of Fe3O4@boehmite NPs are less than 60 nm.
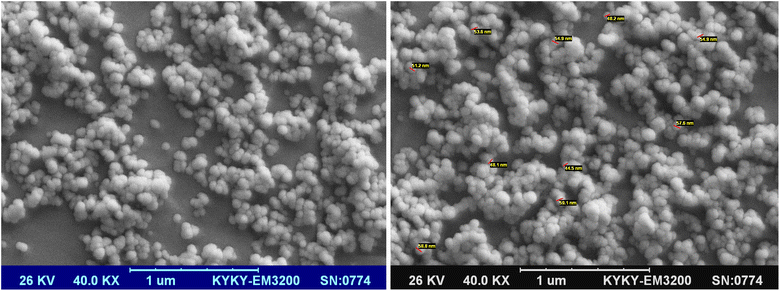 |
| Fig. 2 SEM images of Fe3O4@boehmite NPs. | |
Also, the TEM images were used to studying the morphology and size of Fe3O4@boehmite NPs. As shown in TEM images (Fig. 3), Fe3O4@boehmite NPs were formed in uniform shapes with quite homogeneous diameter.
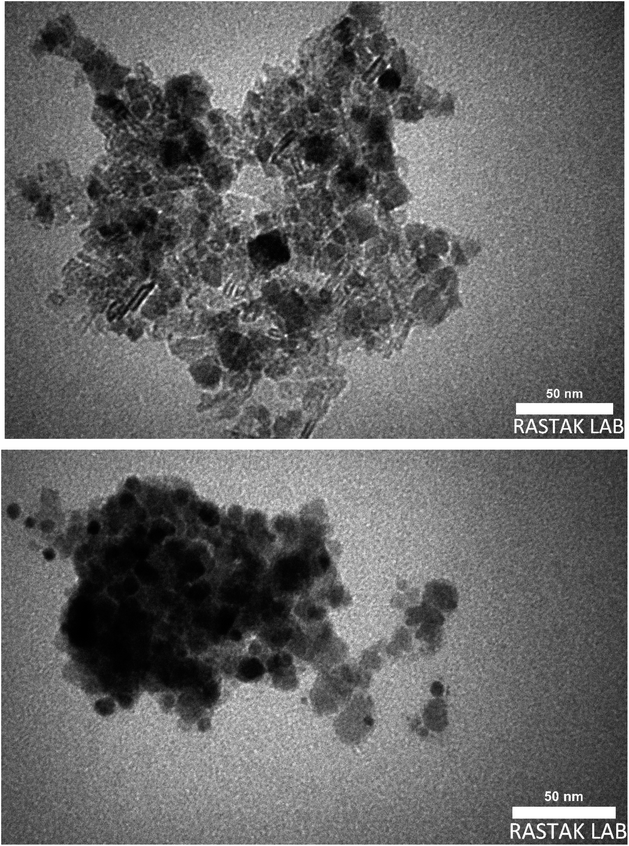 |
| Fig. 3 TEM images of Fe3O4@boehmite NPs. | |
The elements content of Fe3O4@boehmite NPs was studied by EDS analysis. The EDS analysis of Fe3O4@boehmite NPs is shown in Fig. 4 which indicate the presence of oxygen, iron and aluminum species. Also, the elements distribution of Fe3O4@boehmite NPs was studied by WDX analysis. The WDX analysis of Fe3O4@boehmite NPs is shown in Fig. 5 which indicate the uniform distribution of the elements in Fe3O4@boehmite NPs. As shown in the EDS diagram, no elements except oxygen, iron and aluminum elements were seen.
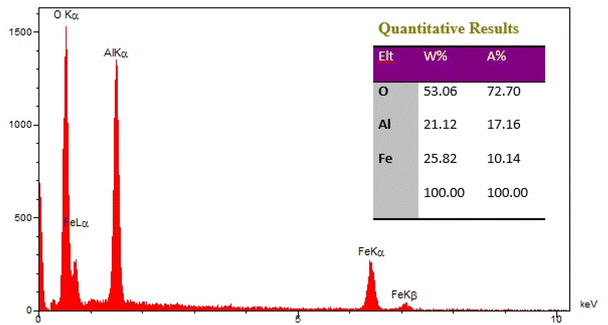 |
| Fig. 4 EDX spectrum of Fe3O4@boehmite NPs. | |
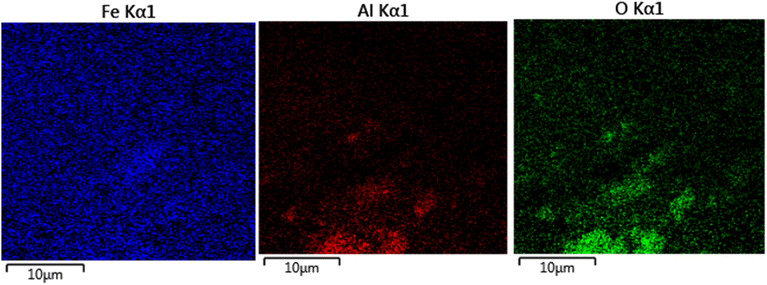 |
| Fig. 5 Elemental mapping of iron, aluminum and oxygen for Fe3O4@boehmite NPs. | |
The exact amount of iron and aluminum elements in Fe3O4@boehmite NPs was obtained by ICP analysis, which found to be 5.7 × 10−3 mol g−1 and 3.5 × 10−3 mol g−1 respectively.
The normal XRD pattern of Fe3O4@boehmite NPs is shown in Fig. 6. The XRD pattern of Fe3O4@boehmite NPs shows the several peaks of 2θ values at 30.5° (220), 35.7° (311), 43.3° (400), 53.6° (422), 57.8° (511), and 62.9° (440), which are related to the crystal phase of Fe3O4 nanoparticles.11 These results confirmed that Fe3O4 MNPs were successfully synthesized and did not any changes during synthesis of Fe3O4@boehmite NPs. These results are in agreement with the standard XRD pattern of Fe3O4 MNPs. Also, the boehmite phase in Fe3O4@boehmite NPs was characterized by the peak positions at 14.9 (020), 28.5 (120), 38.7 (031), 45.7 (131), 49.4 (051), 51.9 (200), 55.8 (151), 60.1 (080), 65.2 (231), 66.6 (002), 68.6 (171), and 72.1 (251) in the XRD pattern.27 These results confirmed that BNPS did not any changes after modification of Fe3O4 MNPs.
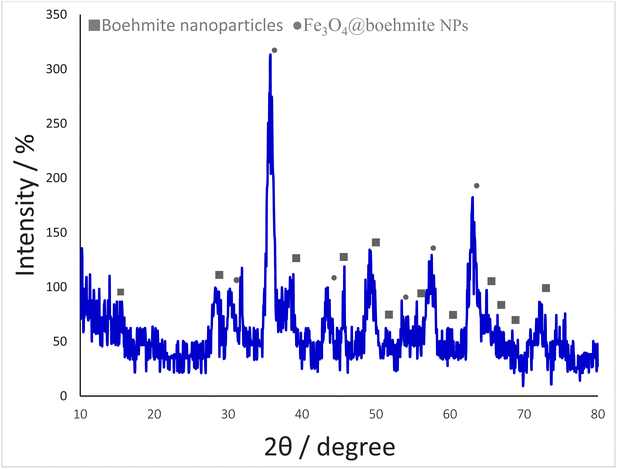 |
| Fig. 6 Normal XRD pattern of Fe3O4@boehmite NPs. | |
The FT-IR spectrum of Fe3O4 NPs, boehmite NPs and Fe3O4@boehmite NPs are shown in Fig. 7. Two bands at region 443 and 587 cm−1 in the FT-IR spectrum of the Fe3O4 NPs (Fig. 7(a)) are correspond to the vibrations of the Fe–O bonds,18,42 which these bands are present in the FT-IR spectrum of Fe3O4@boehmite NPs (Fig. 7(c)). The peaks at (490, 621 and 744 cm−1) in the FT-IR spectrum of the boehmite NPs (Fig. 7(b)) related to the Al–O bonds vibrations,27,44 which these bands are also present in the FT-IR spectrum of Fe3O4@boehmite NPs (Fig. 7(c)). Hydrogen bands of OH⋯OH and nitrate impurity vibration were indicated by the several bands at (1158 and 1077 cm−1) and (1637 cm−1) respectively in FT-IR spectrum of the boehmite NPs (Fig. 7(b)).42,43,45
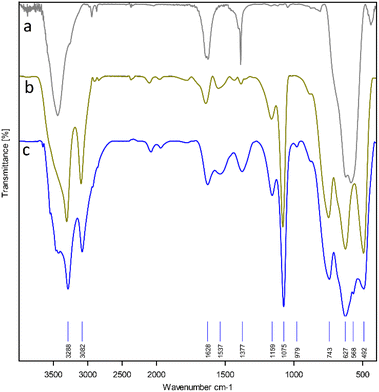 |
| Fig. 7 FT-IR spectra of Fe3O4 NPs (a), boehmite NPs (b) and Fe3O4@boehmite NPs (c). | |
The surface of Fe3O4@boehmite NPs has a large number of hydroxyl groups which the stretching vibration of them appeared above 3000 cm−1 in the FT-IR spectrum.17 Also, the vibrations of their hydrogen bands of OH⋯OH are presented at 1159 and 1075 cm−1.44,45 The several bands at 492, 627 and 743 cm−1 are corresponds to the vibration of the Al–O bonds.27,46 The characteristic the nitrate impurity were emerged at 1628 cm−1.44,47 The peaks which shown in region 452 and 568 cm−1 are related to the vibrations of the Fe–O bond of Fe3O4 NPs18 that overlap with the vibrations of the Al–O bonds.
The Raman spectrum of Fe3O4@boehmite NPs is shown in Fig. 8. The several bands at 312, 348, 513, 545, 663, 798, 1027, 1297, 1362, 1478, 1520, 1578, 1624, 1762, 2820, 2942 and 3150 cm−1 were observed in the Raman spectrum of Fe3O4@boehmite NPs. According to authentic literature, Fe3O4 NPs characterized by several peaks on 311, 540 and 665 cm−1 in Raman spectroscopy.48 Therefore, the Raman spectrum shows that Fe3O4@boehmite NPs contains Fe3O4 NPs, not Fe2O3 NPs. Also, based on another previous literature,49,50 Fe3O4 nanoparticles characterized by several peaks on 305, 513, 534 and 660 cm−1 in Raman spectroscopy. These peaks are observed in Raman spectrum of Fe3O4@boehmite NPs which are indexed to Fe3O4 NPs in Fe3O4@boehmite NPs. Meanwhile, based on same literature,49,50 γ-Fe2O3 nanoparticles characterized by several peaks on 350, 500 and 700 cm−1 in Raman spectroscopy and α-Fe2O3 nanoparticles characterized by several peaks on 221, 244, 292, 406, 497 and 611 cm−1 in Raman spectroscopy. These peaks were not observed in the Raman spectrum of Fe3O4@boehmite NPs. Therefore, Fe3O4@boehmite NPs only includes Fe3O4 NPs.
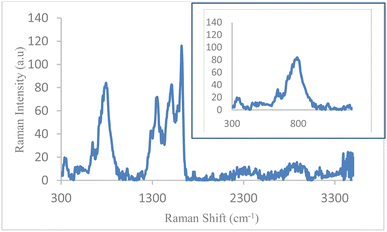 |
| Fig. 8 Raman spectrum Fe3O4@boehmite NPs. | |
The magnetic property of Fe3O4@boehmite NPs was studied by VSM technique using LBKFB device from “Magnetic Kavir Kashan”. The VSM curve of Fe3O4@boehmite NPs is shown in Fig. 9. Magnetic properties of Fe3O4@boehmite NPs was found to be 27.5 emu g−1. As expected, Fe3O4@boehmite NPs showed the lower magnetic value in comparison to Fe3O4 NPs (which is about 74.09 emu g−112) due to the coating of Fe3O4 nanoparticles by boehmite layers.
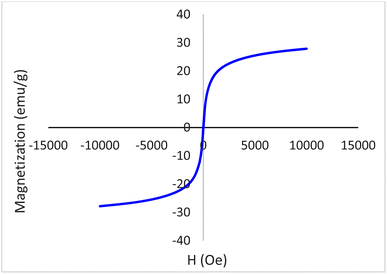 |
| Fig. 9 Magnetization curves for Fe3O4@boehmite NPs. | |
After characterization of Fe3O4@boehmite NPs, its catalytic application was studied in the synthesis of 5-substituted 1H-tetrazole derivatives (Scheme 1).
 |
| Scheme 1 Synthesis of 5-substituted 1H-tetrazoles in the presence of Fe3O4@boehmite NPs. | |
Firstly, the [3+2] cycloaddition of NaN3 with benzonitrile in the presence of Fe3O4@boehmite NPs was selected for optimizing reaction conditions (Table 1). The effect of solvent, amount of NaN3 and temperature was studied in the presence of various catalytic amount of Fe3O4@boehmite NPs. As shown, the [3+2] cycloaddition of NaN3 with benzonitrile was tested in the presence of (30, 20, 15 and 10) mg of Fe3O4@boehmite NPs catalyst (Table 1, entries 2–5), which 15 mg of Fe3O4@boehmite NPs is optimal amount of this catalyst for completing of the reaction in acceptable time (Table 1, entry 4). Reducing the amount of catalyst from 15 mg to 10 mg significantly increases in the reaction time and reduces in the product yield (Table 1, entry 5). While increasing the amount of catalyst has insignificant effect on reaction time or yield (Table 1, entry 3). Then, the optimization conditions were continued in various solvents (Table 1, entries 6–9) in the presence of 15 mg (optimal amount) of Fe3O4@boehmite NPs catalyst. Among various solvents, the best results were obtained when PEG was used as solvent (Table 1, entry 4). Also in continuation of our studying the effect of temperature and sodium azide content was examined, which the best result was observed at 120 °C using 1.5 mmol sodium azide (Table 1, entry 4).
Table 1 Optimization reaction conditions for [3+2] cycloaddition of NaN3 with benzonitrile in the presence of Fe3O4@boehmite NPs catalyst
Entry |
Catalyst (mg) |
Solvent |
NaN3 (mmol) |
Temperature (°C) |
Time (min) |
Yield (%)a |
Isolated yield. |
1 |
— |
PEG |
1.5 |
120 |
600 |
— |
2 |
30 |
PEG |
1.5 |
120 |
210 |
95 |
3 |
20 |
PEG |
1.5 |
120 |
220 |
93 |
4 |
15 |
PEG |
1.5 |
120 |
240 |
97 |
5 |
10 |
PEG |
1.5 |
120 |
600 |
88 |
6 |
15 |
PEG |
1.7 |
120 |
230 |
90 |
7 |
15 |
H2O |
1.5 |
120 |
240 |
25 |
8 |
15 |
DMSO |
1.5 |
120 |
240 |
68 |
9 |
15 |
DMF |
1.5 |
120 |
240 |
73 |
10 |
15 |
PEG |
1.5 |
100 |
240 |
60 |
After optimization of reaction conditions, the catalytic application of Fe3O4@boehmite NPs was extended to aromatic (Table 2, entries 1–12) and aliphatic (Table 2, entries 13–15) nitrile derivatives. In this regard, aromatic nitriles; including, an electron-donating or electron-withdrawing groups on aromatic ring were investigated for the synthesis of corresponding tetrazole deraivatives. As shown in Table 2, all products were obtained in acceptable times and excellent yields which indicated the excellent efficiency of Fe3O4@boehmite NPs. Also, ortho-, meta- and para-substituted benzonitriles were successfully investigated. More additions, aliphatic nitriles were investigated and corresponding tetrazoles were obtained in excellent yields.
Table 2 Synthesis of tetrazoles in the presence of Fe3O4@boehmite NPs
Entry |
Nitrile |
Product |
Time (min) |
Yielda (%) |
Isolated yield. |
1 |
 |
 |
240 |
97 |
2 |
 |
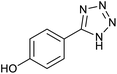 |
335 |
95 |
3 |
 |
 |
300 |
96 |
4 |
 |
 |
360 |
93 |
5 |
 |
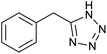 |
260 |
91 |
6 |
 |
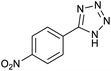 |
28 h |
90 |
7 |
 |
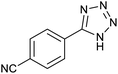 |
24 h |
92 |
8 |
 |
 |
375 |
94 |
9 |
 |
 |
610 |
92 |
10 |
 |
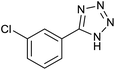 |
12.5 h |
90 |
11 |
 |
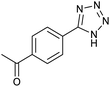 |
37 h |
85 |
12 |
 |
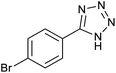 |
25 |
95 |
13 |
 |
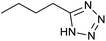 |
12 h |
87 |
14 |
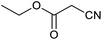 |
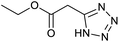 |
105 |
93 |
15 |
 |
 |
65 |
85 |
16 |
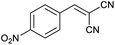 |
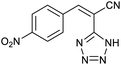 |
135 |
95 |
17 |
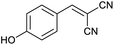 |
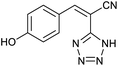 |
200 |
93 |
Homoselectivity is an interesting property which indicate in the quite the same functional groups that only one of them take part in the reaction.51,52 For example, malononitrile which has two identical cyano groups, was investigated for the synthesis of corresponding tetrazoles in the presence of Fe3O4@boehmite NPs catalyst (Table 2, entry 15). Interestingly, this catalyst shown a good selectivity in the synthesis of tetrazoles and only one of identical cyano groups in malononitrile was reacted with sodium azide and another cyano groups was remained without any change (Scheme 2).
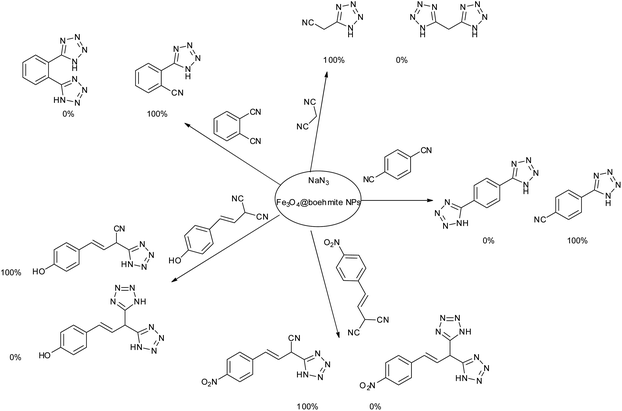 |
| Scheme 2 Homoselectivity of Fe3O4@boehmite NPs catalyst in the [3+2] cycloaddition of NaN3 with dicyano substituted derivatives. | |
Also, benzylidenemalononitrile derivatives bearing electron-withdrawing (Table 2, entry 16) and electron–donor (Table 2, entry 17) functional groups were investigated for the synthesis of tetrazoles in the presence of Fe3O4@boehmite NPs catalyst. These substrate have two cyano-functional groups with same position in their structure which afforded the mono [3+2] cycloaddition with sodium azide in the presence of Fe3O4@boehmite NPs catalyst. Phthalonitrile and terphthalonitrile (Table 2, entries 7 and 8) were also investigated and the products were obtained in high yield and excellent homoselectivity (Scheme 2). Therefore, these results were indicated the high efficiency of Fe3O4@boehmite NPs catalyst in the synthesis of the wide range of tetrazoles.
Reusability of catalysts is one of the principles of green chemistry in the design of chemical processes, which is the most important advantage of heterogeneous catalysts over homogeneous catalysts. Therefore, the reusability of Fe3O4@boehmite NPs catalyst was studied in the [3+2] cycloaddition of NaN3 with benzonitrile (Fig. 10). In this studying, in the end of each reaction, Fe3O4@boehmite NPs catalyst was recovered using an external magnet and washed with water and ethyl acetate. Subsequently the recovered catalyst was charged by same reactants for next run. As shown in Fig. 10, Fe3O4@boehmite NPs catalyst reused up to 6 times.
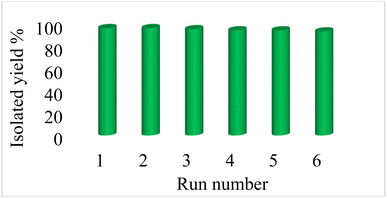 |
| Fig. 10 Recyclability of Fe3O4@boehmite NPs catalyst in the [3+2] cycloaddition of NaN3 with benzonitrile. | |
In order to studying the iron leaching from Fe3O4@boehmite NPs catalyst in the reaction mixture, the [3+2] cycloaddition of NaN3 with benzonitrile was repeated under optimized conditions. In the end of the reaction, the catalyst was isolated by external magnet and the amount of probably leached iron in the reaction solution was calculated by AAS analysis, which a significant amount of iron was not detected in the reaction solution. Therefore, Fe3O4@boehmite NPs are formed by strong bonds between boehmite and Fe3O4 NPs.
The efficiency and practically of Fe3O4@boehmite NPs catalyst was compared to previously catalysts in the synthesis of 5-phenyl-1H-tetrazole from the [3+2] cycloaddition of NaN3 with benzonitrile (Table 3). As shown the higher yield of the product was obtained in the presence of Fe3O4@boehmite NPs catalyst than other catalysts. Also, the reaction time in the presence of Fe3O4@boehmite NPs catalyst is shorter than other catalysts. Fe3O4@boehmite NPs catalyst has the advantages of both MNPs and BNPs and it can be reused for several times. While purification of the products and reusing of the homogeneous catalysts are often difficult, costly and time consuming. The synthesis of tetrazole derivatives were out come in PEG as green solvents in the presence of Fe3O4@boehmite NPs catalyst, while some previously procedures used organic solvents.
Table 3 Comparison of Fe3O4@boehmite NPs catalyst in the [3+2] cycloaddition of NaN3 with benzonitrile
Entry |
Catalyst |
Time (h) |
Yield (%) |
Ref. |
1 |
Cu-TBA@biochar |
7 |
98 |
26 |
2 |
CoY zeolite |
14 |
90 |
53 |
3 |
Cu–Zn alloy nanopowder |
10 |
95 |
54 |
4 |
B(C6F5)3 |
8 |
94 |
55 |
5 |
Fe3O4@SiO2/Salen Cu(II) |
7 |
90 |
56 |
6 |
Fe3O4/ZnS HNSs |
24 |
81.1 |
57 |
7 |
Mesoporous ZnS |
36 |
86 |
58 |
8 |
Cu(OAc)2 |
12 |
98 |
59 |
9 |
CuFe2O4 |
12 |
82 |
60 |
10 |
Nano ZnO/Co3O4 |
12 |
90 |
61 |
11 |
Cu(II)-adenine-MCM-41 |
5 |
92 |
62 |
12 |
Pd-isatin-boehmite |
8 |
94 |
63 |
13 |
Fe3O4@boehmite NPs |
4 |
97 |
This work |
4 Conclusions
In conclusion, magnetic boehmite nanoparticles was synthesized as an efficient and reusable heterogeneous catalyst for the homoselective synthesis of 5-substituted 1H-tetrazoles. This catalyst is composed of boehmite nanoparticles and Fe3O4 nanoparticles, therefore it has advantages of both boehmite nanoparticles (such as high surface area and stability) and Fe3O4 (such as easy separation by an external magnet) systems. Magnetic boehmite nanoparticles can be recovered and reused up to 6 times without any significant loss of its activity. Magnetic boehmite nanoparticles was characterized by TEM, SEM, EDS, WDX, ICP, FT-IR, Raman, XRD and VSM techniques.
Conflicts of interest
There are no conflicts to declare.
Acknowledgements
Author thank the research facilities of Ilam University, Ilam, Iran, for financial support of this research project.
References
- Y. Zhang, L. Duan and H. Esmaeili, Biomass Bioenergy, 2022, 158, 106356 CrossRef CAS.
- S. Yadav and P. Malhotra, in Metal-Organic Frameworks (MOFs) as Catalysts, 2022, pp. 355–367 Search PubMed.
- V. S. Shende, V. B. Saptal and B. M. Bhanage, Chem. Rec., 2019, 19, 2022 CrossRef CAS PubMed.
- S. Hübner, J. G. Vries and V. Farina, Adv. Synth. Catal., 2016, 358, 3 CrossRef.
- H. R. Sonawane, J. V. Deore and P. N. Chavan, ChemistrySelect, 2022, 7, e202103900 CrossRef CAS.
- M. Miceli, P. Frontera, A. Macario and A. Malara, Catalysts, 2021, 11, 591 CrossRef CAS.
- P. Rai and D. Gupta, Synth. Commun., 2021, 51, 3059 CrossRef CAS.
- J. Min, Z. Xia, T. Zhang, H. Su, Y. Zhi and S. Shan, Chem. Pap., 2021, 75, 2965 CrossRef CAS.
- P. Kumar, V. Tomar, D. Kumar, R. KumarJoshi and M. Nemiwal, Tetrahedron, 2022, 106–107, 132641 CrossRef CAS.
- P. Kumar, V. Tomar, R. Kumar Joshi and M. Nemiwal, Synth. Commun., 2022, 52, 795 CrossRef CAS.
- M. Nikoorazm, P. Moradi, N. Noori and G. Azadi, J. Iran. Chem. Soc., 2021, 18, 467 CrossRef CAS.
- L. Shiri and B. Tahmasbi, Phosphorus, Sulfur, Silicon Relat. Elem., 2017, 192, 53 CrossRef CAS.
- R. Tandon, N. Tandon and S. M. Patil, RSC Adv., 2021, 11, 29333 RSC.
- T. Cheng, D. Zhang, H. Li and G. Liu, Green Chem., 2014, 16, 3401 RSC.
- M. Ghobadi, M. Kargar Razi, R. Javahershenas and M. Kazemi, Synth. Commun., 2021, 51, 647 CrossRef CAS.
- S. Sayyahi, M. Fallah-Mehrjardi and S. J. Saghanezhad, Mini-Rev. Org. Chem., 2021, 18, 11 CrossRef CAS.
- B. Tahmasbi and A. Ghorbani-Choghamarani, New J. Chem., 2019, 43, 14485 RSC.
- A. Ghorbani-Choghamarani, B. Tahmasbi, R. H. E. Hudson and A. Heidari, Microporous Mesoporous Mater., 2019, 284, 366 CrossRef CAS.
- P. Moradi and M. Hajjami, RSC Adv., 2022, 12, 13523 RSC.
- P. Moradi and M. Hajjami, New J. Chem., 2021, 45, 2981 RSC.
- P. Moradi and M. Hajjami, RSC Adv., 2021, 11, 25867 RSC.
- A. Mohammadinezhad and B. Akhlaghinia, Green Chem., 2017, 19, 5625 RSC.
- J. De Bellis, C. Ochoa-Hernández, C. Farès, H. Petersen, J. Ternieden, C. Weidenthaler, A. P. Amrute and F. Schüth, J. Am. Chem. Soc., 2022, 144, 9421 CrossRef CAS.
- M. Barik, J. Mishra, S. Dabas, E. Chinnaraja, S. Subramanian and P. S. Subramanian, New J. Chem., 2022, 46, 695 RSC.
- A. Kausar, J. Plast. Film Sheeting, 2022, 38, 278 CrossRef CAS.
- P. Moradi, M. Hajjami and B. Tahmasbi, Polyhedron, 2020, 175, 114169 CrossRef.
- A. Jabbari, P. Moradi, M. Hajjami and B. Tahmasbi, Sci. Rep., 2022, 12, 11660 CrossRef CAS PubMed.
- J. Karger-Kocsis and L. Lendvai, J. Appl. Polym. Sci., 2018, 135, 45573 CrossRef.
- B. Tahmasbi and A. Ghorbani-Choghamarani, Appl. Organomet. Chem., 2017, 31, e3644 CrossRef.
- C. G. Neochoritis, T. Zhao and A. Dömling, Chem. Rev., 2019, 119, 1970 CrossRef CAS.
- S. A. Hamrahian, S. Salehzadeh, J. Rakhtshah, F. Haji babaei and N. Karami, Appl. Organomet. Chem., 2019, 33, e4723 CrossRef.
- P. K. Samanta, R. Biswas, T. Das, M. Nandi, B. Adhikary, R. M. Richards and P. Biswas, J. Porous Mater., 2019, 26, 145 CrossRef CAS.
- F. Rezaei, M. Ali Amrollahi and R. Khalifeh, Inorg. Chim. Acta., 2019, 489, 8 CrossRef CAS.
- P. Akbarzadeh, N. Koukabi and E. Kolvari, Res. Chem. Intermed., 2019, 45, 1009 CrossRef CAS.
- A. Maleki, M. Niksefat, J. Rahimi and S. Azadegan, Polyhedron, 2019, 167, 103 CrossRef CAS.
- A. Sarvary and A. Maleki, Mol. Divers., 2015, 19, 189 CrossRef CAS.
- A. Maleki and A. Sarvary, RSC Adv., 2015, 5, 60938 RSC.
- M. Nikoorazm, B. Tahmasbi, S. Gholami and P. Moradi, Appl. Organomet. Chem., 2020, 34, e5919 CAS.
- R. Kant, V. Singh and A. Agarwal, C. R. Chimie, 2016, 19, 306 CrossRef.
- P. Kumar Samanta, R. Biswas, T. Das, M. Nandi, B. Adhikary, R. M. Richards and P. Biswas, J. Porous Mater., 2019, 26, 145 CrossRef.
- G. M. Ojeda-Carralero, J. Coro and A. Valdés-Palacios, Chem. Heterocycl. Compd., 2020, 56, 408 CrossRef CAS.
- A. Ghorbani-Choghamarani, B. Tahmasbi and Z. Moradi, Appl. Organomet. Chem., 2017, 31, e3665 CrossRef.
- A. Ghorbani-Choghamarani, Z. Seydyosefi and B. Tahmasbi, C. R. Chimie, 2018, 21, 1011 CrossRef CAS.
- A. Ghorbani-Choghamarani, B. Tahmasbi and P. Moradi, RSC Adv., 2016, 6, 43205 RSC.
- L. Rajabi and A. A. Derakhshan, Sci. Adv. Mater., 2010, 2, 163 CrossRef CAS.
- M. Mirzaee, B. Bahramian and A. Amoli, Appl. Organomet. Chem., 2015, 29, 593 CrossRef CAS.
- V. Vatanpour, S. S. Madaenia, L. Rajabi, S. Zinadini and A. A. Derakhshan, J. Membr. Sci., 2012, 401–402, 132 CrossRef CAS.
- J. M. Li, Phys. Rev. B, 2000, 61, 6876 CrossRef CAS.
- X. Zhang, Y. Niu, X. Meng, Y. Li and J. Zhao, CrystEngComm, 2013, 15, 8166 RSC.
- P. Kumar, H. No-Lee and R. Kumar, J. Mater. Sci.: Mater. Electron., 2014, 25, 4553 CrossRef CAS.
- G. Chehardoli and M. A. Zolfgol, Phosphorus, Sulfur Silicon Relat. Elem., 2010, 185, 193 CrossRef CAS.
- A. Hasaninejad, G. Chehardoli, M. A. Zolfgol and A. Abdoli, Phosphorus, Sulfur Silicon Relat. Elem., 2011, 186, 271 CrossRef CAS.
- V. Rama, K. Kanagaraj and K. Pitchumani, J. Org. Chem., 2011, 76, 9090 CrossRef CAS PubMed.
- G. Aridoss and K. K. Laali, Eur. J. Org. Chem., 2011, 2011, 6343 CrossRef CAS.
- S. Kumar Prajapti, A. Nagarsenkar and B. Nagendra Babu, Tetrahedron Lett., 2014, 55, 3507 CrossRef.
- F. Dehghani, A. R. Sardarian and M. Esmaeilpour, J. Organomet. Chem., 2013, 743, 87 CrossRef CAS.
- G. Qi, W. Liu and Z. Bei, Chin. J. Chem., 2011, 29, 131 CrossRef CAS.
- L. Lang, H. Zhou, M. Xue, X. Wang and Z. Xu, Mater. Lett., 2013, 106, 443 CrossRef CAS.
- P. Mani, A. K. Singh and S. K. Awasthi, Tetrahedron Lett., 2014, 55, 1879 CrossRef CAS.
- B. Sreedhar, A. Suresh Kumar and D. Yada, Tetrahedron Lett., 2011, 52, 3565 CrossRef CAS.
- S. M. Agawane and J. M. Nagarkar, Catal. Sci. Technol., 2012, 2, 1324 RSC.
- M. Nikoorazm, A. Ghorbani-Choghamaranai, M. Khanmoradi and P. Moradi, J. Porous Mater., 2018, 25, 1831 CrossRef CAS.
- A. Jabbari, B. Tahmasbi, M. Nikoorazm and A. Ghorbani-Choghamarani, Appl. Organomet. Chem., 2018, 32, e4295 CrossRef.
|
This journal is © The Royal Society of Chemistry 2022 |
Click here to see how this site uses Cookies. View our privacy policy here.