DOI:
10.1039/D2RA05558A
(Paper)
RSC Adv., 2022,
12, 30001-30007
Synthesis and biological evaluation of novel sinomenine derivatives as anti-inflammatory and analgesic agent†
Received
4th September 2022
, Accepted 5th October 2022
First published on 20th October 2022
Abstract
Sinomenine (SIN) has long been known as an anti-inflammatory drug, while poor efficiency and large-dose treatment had limited its further application. A series of novel SIN derivatives 1–26 were designed and synthesized to improve its anti-inflammatory activity. The anti-inflammatory activity evaluation showed most of the derivatives exhibited enhanced anti-inflammatory activity in vitro compared to SIN. Compound 17 significantly inhibited LPS-induced secretion of pro-inflammatory factors NO (IC50 = 30.28 ± 1.70 μM), and suppressed the expression of iNOS, IL-6 and TNF-α in RAW264.7 cells. Moreover, compound 17 showed excellent anti-inflammatory in mouse paw edema. Immunohistochemistry results revealed that compound 17 exerted anti-inflammatory activity by inhibiting the pro-inflammatory cytokine TNF-α. Furthermore, compound 17 exhibited an analgesic effect in vivo. The results attained in this study indicated that compound 17 had the potential to be developed into an anti-inflammation and analgesic agent.
Introduction
Inflammation is a common pathological process, which occurs as a result of exposure of tissues and organs to harmful stimuli such as microbial pathogens, allergens or toxic cellular components.1 The primary physical manifestations of inflammation are redness, swelling, heat, pain, and loss of function to the affected area.2 Although inflammation is a beneficial automatic defence mechanism, uncontrolled inflammation is the main cause of many serious diseases such as rheumatoid arthritis, systemic lupus erythematosus, atherosclerosis, etc.3–5
The commonly used anti-inflammatory drugs in clinics are mainly divided into glucocorticoids and nonsteroidal anti-inflammatory drug (NSAIDS).6 Glucocorticoids such as prednisolone, dexamethasone and hydrocortisone are one of the common clinical drugs, and exhibit good anti-inflammatory effects on inflammation caused by various causes. However, long-term use of these drugs can cause edema, hypokalemia, abnormal increase of blood sugar, digestive tract ulcers and other side effects.
NSAIDS have a great efficacy on inflammatory and pain by inhibiting cyclooxygenase (COX) activity. Currently, NSAIDS were among the most frequently used over-the-counter drugs in the world, accounting for 5% of all prescription drugs.7 Although the use of NSAIDS was clearly unavoidable, but the disadvantages of NSAIDS that may lead to an increased risk of cardiovascular disease and organ toxicity such as liver and kidney cannot be ignored.7,8 Yet, the development of more effective and less toxic drugs from natural products to treat acute inflammation as well as chronic inflammatory diseases remains a challenge in medicinal chemistry.9–14
Sinomenine (SIN), an alkaloid extracted from the anti-rheumatism Chinese medicine Sinomenium acutum (Thunb.) Rehd. et Wils., has long been known as an anti-inflammatory drug.15–19 SIN has been reported to inhibit the production of proinflammatory mediators, such as TNF-α, nitric oxide (NO) and IL-6, both in vitro and in vivo.20–22 Therefore, SIN was regarded as lead compounds in the drug research, especially for anti-inflammatory medicines, while low activity had limited its clinical application.23–27 To improve activity, structural modification of SIN has attracted enormous interest in recent years.28,29 Studies have shown that the phenolic hydroxyl group of C-4 was easy to oxidize and decompose and may be a factor leading to allergic reactions in vivo. Structure–activity relationships (SARs) showed that the modification at C-1 and C-4 could enhance the activity of SIN. Several SIN derivatives have been reported and exhibit strong anti-inflammatory activity (Fig. 1).30–32 Introducing amino acid group into the drug molecule could enhance its anti-tumor and anti-inflammatory activity and increase its solubility.33,34 Meanwhile, nitrogen-based heterocycles in the drug design are almost 60% of unique small-molecule drugs. A large number of nitrogen-based heterocyclic derivatives including pyrazine, pyrimidine and pyridazine have been discovered and described for their anti-inflammatory properties.35
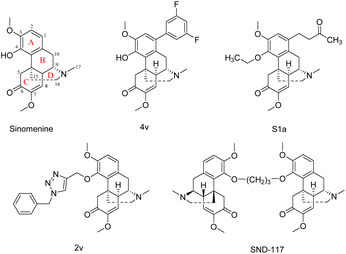 |
| Fig. 1 Structures of sinomenine and previously synthesized compounds. | |
In this study, to improve the anti-inflammatory activity of SIN, a variety of amino acid and nitrogen-based heterocyclic biologically active small fragments were introduced to SIN to synthesize compounds 1–26. The anti-inflammatory activity and cytotoxicity of all the novel derivatives were evaluated by LPS-induced RAW264.7 inflammatory cell model. Then the preliminary anti-inflammatory mechanism of compound 17 was evaluated by qPCR experiment. Furtherly, the λ-carrageenan-induced paw oedema and acetic acid-induced writhing mouse model was used to determine the anti-inflammatory and analgesic effects of compound 17. This study lays the foundation for the subsequent development of new anti-inflammatory drugs of SIN and has practical significance for the expansion of clinical applications of SIN.
Results and discussion
Chemical synthesis
Amino acids are the basic substances necessary for human life activities and have many important physiological functions.36–38 Compounds 1–14 were prepared following the procedure Scheme 1. SIN was reacted with Boc-L-amino acid in dry dichloromethane (DCM) in the presence of 1-ethyl-3-(3-dimethylaminopropyl)carbodiimide hydrochloride (EDCI) and 4-dimethylaminopyridine (DMAP) to yield compounds 1–7. Subsequently, deprotection was performed with trifluoroacetic acid (TFA) in dry DCM at room temperature to afford compounds 8–14.
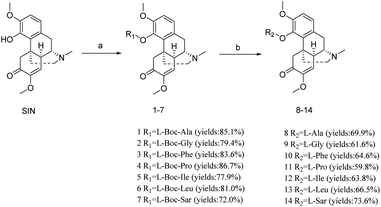 |
| Scheme 1 Synthesis of compounds 1–14. Reagents and conditions: (a) Boc-L-amino acid, EDCI, DMAP, rt, 12 h; (b) TFA, rt, 30 min. | |
The 3,5,6-trimethylpyrazine-2-chloride (TMP-Cl) was synthesized according to our previous study.39 In Scheme 2, SIN was reacted with N-heterocycles in dry DCM in the presence of EDCI and DMAP to yield compounds 16–19. As the H-1 of SIN was easily halogenated by N-bromosuccinimide (NBS), the SIN-1-Br was prepared. Then it was reacted with nitrogen-based heterocyclic to yield compounds 20–24. In the synthesis of compound 25–26, the SIN was firstly reacted with paraformaldehyde to afford the intermediate SIN-1-CH2OH, then it was reacted with N-heterocycles to yield compounds 25–26.
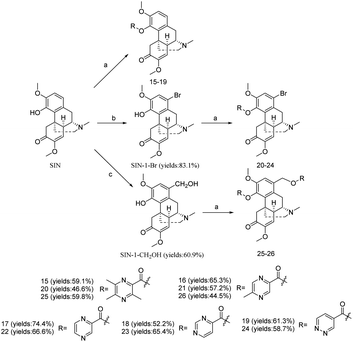 |
| Scheme 2 Synthesis of the compounds 15–26. Reagents and conditions: (a) EDCI, DMAP, rt, 12 h; (b) NBS, rt, 10 h; (c) (CH2O)n, HCl, 60 °C, 2 h. | |
Bioassay for anti-inflammatory activity in vitro
In this section, the LPS-induced RAW264.7 inflammatory cell model was used to evaluate the anti-inflammatory effects of compounds in vitro.40 NO is an important endogenous free radical involved in a variety of physiological functions and pathophysiological states. Griess assay was used to evaluate the inhibitory effect of compounds 1–26 on the overproduction of NO. If the amount of NO decreases after treatment with compounds, the inflammatory response is considered to be suppressed and the corresponding compound is an effective anti-inflammatory agent.41
Before the anti-inflammatory activity assay, we screened the cytotoxicity of the tested compound to avoid false-positive results.42 The cytotoxicity of compounds 1–26 on RAW264.7 cells was evaluated by MTT assay. The IC50 values of these compounds were summarized in Table 1. As shown, the cytotoxicity IC50 of SIN was >100 μM. Among them, the toxicity of compounds 1 (IC50 = 19.11 ± 2.43 μM), 3 (IC50 = 29.62 ± 7.48 μM), 15 (IC50 = 17.88 ± 2.24 μM), 20 (IC50 = 21.88 ± 3.51 μM), 22 (IC50 = 52.69 ± 3.19 μM) and 25 (IC50 = 54.87 ± 7.77 μM) was significantly increased compared to SIN. SARs shown that introduction of tetramethylpyrazine at C-4 could significantly improve the toxicity of SIN, but the modification of C-1 reduced the increase of toxicity. In addition, the cytotoxicity IC50 of most of the compounds did not increase significantly and all exceeded 100 μM.
Table 1 The cytotoxicity of SIN and compounds in RAW264.7 cells
Compound |
Cytotoxicity IC50 (μM) |
Compound |
Cytotoxicity IC50 (μM) |
SIN |
>100 |
13 |
>100 |
Indo |
>100 |
14 |
>100 |
1 |
19.11 ± 2.43 |
15 |
17.88 ± 2.24 |
2 |
>100 |
16 |
>100 |
3 |
29.62 ± 7.48 |
17 |
>100 |
4 |
>100 |
18 |
>100 |
5 |
>100 |
19 |
>100 |
6 |
>100 |
20 |
21.88 ± 3.51 |
7 |
>100 |
21 |
>100 |
8 |
>100 |
22 |
52.69 ± 3.19 |
9 |
>100 |
23 |
>100 |
10 |
>100 |
24 |
>100 |
11 |
>100 |
25 |
54.87 ± 7.77 |
12 |
>100 |
26 |
>100 |
Then, LPS-induced RAW 264.7 cells were used to evaluate the inhibitory activity of all compounds 1–26 on NO production, and calculate the IC50. As depicted in Table 2, the IC50 of SIN was 70.86 ± 1.00 μM, indicating that the anti-inflammatory activity of SIN was relatively weak. The anti-inflammatory activity of most of the compounds increased after modification of amino acids and nitrogen-based heterocyclic ring compared to SIN. The elementary SARs of NO inhibitory activity indicated that introduction of protected amino acid (Boc group) at C-4 could enhance anti-inflammatory activities, and deprotect the Boc group could reduce anti-inflammatory efficacy. Among the nitrogen-containing heterocyclic compounds, the compound with pyrazine group showed stronger activity compared to these compounds with pyridazine and pyrimidine. While the effect of substituted halogen atoms at the C-1 position on the anti-inflammatory activity of the compounds was less. Among all the derivatives, compound 17 showed strong anti-inflammatory activity (IC50 = 30.28 ± 1.70 μM), which was twice as strong as SIN, and it was worth to further investigate its mechanism of action and anti-inflammatory activity in vivo.
Table 2 The inhibitory effects on NO production of SIN and compound 1–26 in LPS-induced RAW264.7 cellsa
Compound |
Activity IC50 (μM) |
Compound |
Activity IC50 (μM) |
NS: data not shown because activity IC50 is higher than cytotoxicity IC50. |
SIN |
70.86 ± 1.00 |
13 |
69.19 ± 9.02 |
Indo |
44.67 ± 2.29 |
14 |
41.69 ± 3.66 |
1 |
NS |
15 |
NS |
2 |
42.27 ± 2.26 |
16 |
50.40 ± 4.62 |
3 |
NS |
17 |
30.28 ± 1.70 |
4 |
51.38 ± 3.89 |
18 |
63.99 ± 1.32 |
5 |
44.11 ± 2.24 |
19 |
77.81 ± 6.04 |
6 |
59.50 ± 3.56 |
20 |
NS |
7 |
59.28 ± 4.64 |
21 |
48.69 ± 2.06 |
8 |
68.87 ± 3.70 |
22 |
NS |
9 |
53.36 ± 2.51 |
23 |
58.84 ± 5.59 |
10 |
72.27 ± 1.64 |
24 |
53.36 ± 4.64 |
11 |
52.77 ± 4.67 |
25 |
NS |
12 |
60.40 ± 3.32 |
26 |
69.10 ± 3.76 |
Effect of compound 17 on iNOS, IL-6 and TNF-α mRNA expression in LPS-induced RAW264.7 cells
The inflammatory response is generally regulated by a complex network of cytokines and chemokines; clinical interventions have clearly demonstrated that IL-6, TNF-α are essential in this process. IL-6 and TNF-α contributed to the exacerbation of the inflammatory response by activating endothelial cells and attracting immune cells to accumulate at the site of inflammation. In addition, the excessive production of NO could affect the NF-κB signaling pathway, which in turn affects the expression of cytokines and chemokines including IL-6 and TNF-α.43 In this section, qPCR was performed to qualification of genes including iNOS, IL-6, and TNF-α.
The effect of compound 17 with different concentrations on iNOS, IL-6 and TNF-α expression are shown in Fig. 2. Compared with the control group, the expression of iNOS mRNA was significantly increased in the model group after LPS induction, indicating that the inflammation model was successfully established. Compared with the model group, the compound 17 with high dose group (60 μM) could significantly inhibit the expression of iNOS mRNA. Compared with the model group, compound 17 with low dose group (15 μM), medium dose group (30 μM) and high dose group (60 μM) could significantly inhibit the expression of IL-6 mRNA. The treatment group inhibited TNF-α mRNA expression in a dose-dependent manner. Compared with the model group, compound 17 with the low dose group (15 μM) had no significant effect on the expression of TNF-α mRNA; the medium dose group (30 μM) could significantly reduce the expression of TNF-α mRNA; while the high dose group (60 μM) could inhibit the expression of TNF-α mRNA extremely significantly.
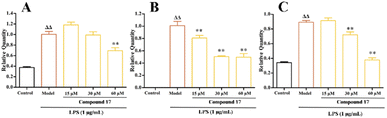 |
| Fig. 2 Effect of compound 17 on LPS-induced iNOS, IL-6 and TNF-α mRNA expression in RAW264.7 cells ( ± s, n = 3). (A) qPCR results of iNOS mRNA levels. (B) qPCR results of IL-6 mRNA levels. (C) qPCR results of TNF-α mRNA levels. Compared with the control group, ΔΔP < 0.01; compared with the model group, **P < 0.01. | |
In general, the RNA reduction of iNOS, IL-6 and TNF-α genes interprets the effects of compounds 17 on anti-inflammatory activity in RAW264.7 cells.
Bioassay for anti-inflammatory activity in vivo
Mouse paw edema method is widely used for the screening and evaluation of anti-inflammatory drugs due to its reliability and reproducibility.44,45 In this section, compound 17 with relative potent anti-inflammatory activity in vitro was selected and further evaluated with a λ-carrageenan-induced mouse paw edema test using the indomethacin as the positive drug. As shown in Fig. 3, after the injection of λ-carrageenan for 2 h in the model group mice, the paws showed obvious swelling, and the edema rate was around 60%.
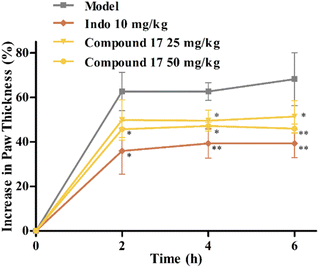 |
| Fig. 3 In vivo anti-inflammatory activities of compound 17 ( ± SEM, n = 8). Compared with the model group, *P < 0.05, **P < 0.01. | |
The indomethacin group (10 mg kg−1) significantly inhibited the development of edema at 2–6 h. Administration (i. g.) of compound 17 at both 25 and 50 mg kg−1 doses significantly attenuated edema formation at 2–6 h. This suggested that compound 17 might be a potential anti-inflammatory agent with some in vivo anti-inflammatory activity.
The histological examination results were shown in Fig. 4. In the blank group, the articular cartilage was smooth and the subcutaneous tissue was dense without significant edema (Fig. 4A). After injection of λ-carrageenan, paw biopsies of control animals showed marked vasodilation and tissue edema. The subcutaneous tissue gap was enlarged, but articular cartilage was smooth and no lesions were observed (Fig. 4B). Paw biopsies of animals treated with indomethacin and compound 17 showed a reduction in the λ-carrageenan-induced inflammatory response. Among them, the compound 17 with high dose group (50 mg kg−1) showed a reduction of edemas, and the subcutaneous tissue was dense (Fig. 4E).
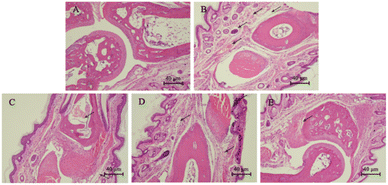 |
| Fig. 4 Effects of compound 17 on morphological changes in paw edema tissue by hematoxylin–eosin staining. (A) Blank group. (B) Model group. (C) Indo group. (D) Compound low dose group (25 mg kg−1). (E) Compound 17 high dose group (50 mg kg−1). | |
The effects of compound 17 on the levels of TNF-α were shown in Fig. 5. No significant positive brownish-yellow staining was observed in the blank group, indicating less TNF-α expression (Fig. 5A). Compared with the blank group, the model group showed a large amount of brownish-yellow colouring at the articular cartilage, around the blood vessels and in the bone, indicating a large amount of TNF-α expression (Fig. 5B). The high dose group significantly inhibited TNF-α secretion around osteocytes compared with the model group, and only a small amount of positive coloration was seen at the articular cartilage (Fig. 5E), indicating that compound 17 significantly inhibited the expression of TNF-α inflammatory cytokines at the toe joint in mice. These results were also confirmed by the qPCR analysis (Fig. 2).
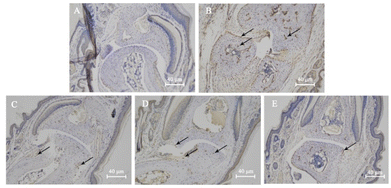 |
| Fig. 5 Representative immunohistochemical staining for the levels of TNF-α. (A) Blank group. (B) Model group. (C) Indo group. (D) Compound low dose group (25 mg kg−1). (E) Compound 17 high dose group (50 mg kg−1). | |
Bioassay for analgesia activity in vivo
The acetic acid-induced writhing test has been widely used for testing the analgesic effect.46,47 It is a non-selective model for analgesic studies, given that an intraperitoneal injection of acetic acid triggers the release of cytokines and chemokines. The effect of compound 17 on the writhing response in mice were shown in Fig. 6. The present result showed the positive control drug Voltaren (19.5 mg kg−1) could reduce the number of writhes considerably. And the SIN at a dose of 200 mg kg−1 and the compound 17 at a dose of 50 mg kg−1 exhibited almost no analgesic effects. However, compound 17 at a dose of 100 and 200 mg kg−1 could significantly decrease the number of writhes compared to that of the control group. Meanwhile, the analgesic activity of the compound 17 at a dose of 200 mg kg−1 was stronger than that of Voltaren group. The results showed that compound 17 significantly reduced the number of writhes induced by acetic acid, suggesting that compound 17 has peripheral analgesic effects.
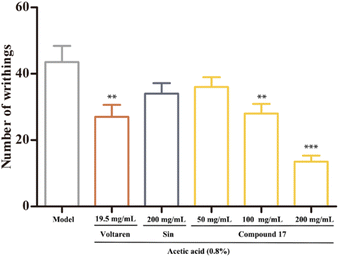 |
| Fig. 6 Anti-nociceptive effects of compound 17 in mice. Compared with the model group, **P < 0.01. | |
Molecular docking
NO is an important signal molecule in the body, which is produced by iNOS catalyzing L-arginine. iNOS is a carrier of gas-to-cell intercellular information transmission, which is activated in the inflammatory response, which increases iNOS expression and catalyzes NO production. NF-κB is a classic inflammatory signaling pathway. Activation of NF-κB stimulates the release of IL-6, TNF-α and NO when stimulated, which may worsen the inflammatory response.
To better understand the interaction of compound 17 with NF-κB and iNOS, CDOCKER was running by fitting compound 17 into the active site of NF-κB and iNOS. As shown in Fig. 7A and B, compound 17 skeleton was placed in the active cavity of NF-κb P65 formed by ARG 354, TYR 357, GLU 360, LYS 541, GLN 606, LYS 572, PRO 543 etc. van der Waals, hydrogen bonds, pi-cation and pi-alkyl interactions between compound 17 and NF-κB protein further stabilized the interaction. As shown in Fig. 7C and D, compound 17 skeleton was placed in the active cavity of iNOS formed by PRO 350, GLY 202, TRP 463, ILE 201, CYS 200, ARG 199 etc. Hydrogen bonds, pi-sulfur and alkyl interactions between compound 17 and iNOS protein further stabilized the interaction.
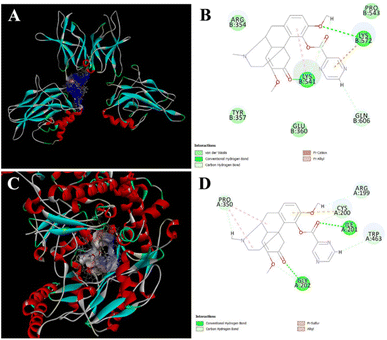 |
| Fig. 7 Molecular docking. Predicted docking pose of compound 17 in complex with NF-κB (PDB 1VKX) and iNOS (PDB 4NOS). (A) Overall structure of NF-κB/compound 17 complex. The hydrophilic surface is coloured in blue. (B) Local view of residues interacting with NF-κB and compound 17. (C) Overall structure of iNOS/compound 17 complex. The hydrophilic surface is coloured in blue. (D) Local view of residues interacting with iNOS and compound 17. | |
Conclusions
Above all, a series of novel SIN derivatives were designed and synthesized, all of them were characterized by 1H NMR, 13C NMR and HR-MS. In vitro anti-inflammatory activity and cytotoxicity evaluation showed that most of the derivatives exhibited stronger anti-inflammatory activity compared to SIN, especially the compound 17 showed the most potent activity. Study of anti-inflammatory mechanism in vitro showed that compound 17 could inhibit the expression of iNOS mRNA, reduce the overproduction of NO and inhibit the expression of IL-6 and TNF-α mRNA. Experiments in vivo showed that compound 17 exhibited an ameliorating effect on λ-carrageenan induced paw oedema in mice. Pathological analysis and immunohistochemical results also indicated that it showed an ameliorating effect on subcutaneous edema of the mouse plantar and inhibited the expression of TNF-α inflammatory cytokines. The acetic acid-induced writhing test also demonstrated that compound 17 had good analgesic activity. Altogether, the results of the series of SIN derivatives supported the potential value of anti-inflammatory and had practical significance for the expansion of clinical applications of SIN.
Ethical approval
All animal procedures were performed in accordance with the Guidelines for Care and Use of Laboratory Animals of Beijing University of Chinese Medicine and experiments were approved by the Animal Ethics Committee of Beijing University of Chinese Medicine.
Author contributions
Feng Gao: conceptualization, methodology, validation, writing – original draft. Ziqi Dai: investigation, validation. Qianwen Wu: investigation. Desheng Cai: investigation. Mingjun Lu: data curation. Zijie Zhang: investigation. Qi Zeng: investigation. Tong Zhang: writing – reviewing and editing. Bingxian Shang: writing – reviewing and editing. Bing Xu: funding acquisition, supervision. Haimin Lei: resources, supervision, project administration, funding acquisition.
Conflicts of interest
There are no conflicts to declare.
Acknowledgements
This research was funded by the National Natural Science Foundation of China (no. 82104365, 81173519 and 81603256), the 111 project (no. B21028), the Fundamental Research Funds for the Central Universities (no. 2020-JYB-XJSJJ-007), Beijing “high-grade, precision and advanced” project, Beijing Key Laboratory for Basic and Development Research on Chinese Medicine (Beijing, 100102).
Notes and references
- R. Medzhitov, Origin and physiological roles of inflammation, Nature, 2008, 454(7203), 428–435 CrossRef CAS PubMed.
- C. N. Serhan, Treating inflammation and infection in the 21st century: new hints from decoding resolution mediators and mechanisms, FASEB J., 2017, 31(4), 1273–1288 CrossRef CAS PubMed.
- C. N. Serhan and B. D. Levy, Resolving in inflammation: emergence of the pro-resolving superfamily of mediators, J. Clin. Invest., 2018, 128(7), 2657–2669 CrossRef PubMed.
- I. B. McInnes and G. Schett, The Pathogenesis of Rheumatoid Arthritis, N. Engl. J. Med., 2011, 365, 2205–2219 CrossRef PubMed.
- J. S. Smolen, D. Aletaha and I. B. McInnes, Rheumatoid arthritis, Lancet, 2016, 388, 2023–2038 CrossRef CAS.
- J. A. Sparks, Rheumatoid Arthritis, Ann. Intern. Med., 2019, 170(1), ITC1–ITC16 CrossRef PubMed.
- S. Bindu, S. Mazumder and U. Bandyopadhyay, Non-steroidal anti-inflammatory drugs (NSAIDs) and organ damage: A current perspective, Biochem. Pharmacol., 2020, 180, 114147 CrossRef CAS PubMed.
- A. M. Schjerning, P. McGettigan and G. Gislason, Cardiovascular effects and safety of (non-aspirin) NSAIDs, Nat. Rev. Cardiol., 2020, 17(9), 574–584 CrossRef CAS PubMed.
- A. Gosslau, S. Li and C. T. Ho, et al., The importance of natural product characterization in studies of their anti-inflammatory activity, Mol. Nutr. Food Res., 2011, 55(1), 74–82 CrossRef CAS PubMed.
- A. Koeberle and O. Werz, Multi-target approach for natural products in inflammation, Drug discovery today, 2014, 19(12), 1871–1882 CrossRef CAS PubMed.
- K. Chen, W. Sun and Y. Jiang, et al., Ginkgolide B suppresses TLR4-mediated inflammatory response by inhibiting the phosphorylation of JAK2/STAT3 and p38 MAPK in high glucose-treated HUVECs, Oxid. Med. Cell. Longevity, 2017, 1–12 Search PubMed.
- Y. Dai, S. R. Chen and L. Chai, et al., Overview of pharmacological activities of Andrographis paniculata and its major compound andrographolide, Crit. Rev. Food Sci. Nutr., 2019, 59(sup1), S17–S29 CrossRef CAS PubMed.
- K. Yuan, X. Li and Q. Lu, et al., Application and mechanisms of triptolide in the treatment of inflammatory diseases—a review, Front. Pharmacol., 2019, 10, 1469 CrossRef CAS PubMed.
- W. Liu, Y. Zhang and W. Zhu, et al., Sinomenine inhibits the progression of rheumatoid arthritis by regulating the secretion of inflammatory cytokines and monocyte/macrophage subsets, Front. Immunol., 2018, 9, 2228–2241 CrossRef PubMed.
- H. Feng, K. Yamaki and H. Takano, et al., Effect of sinomenine on collagen-induced arthritis in mice, Autoimmunity, 2007, 40(7), 532–539 CrossRef CAS PubMed.
- S. Liu, Y. Man and L. Zhao, Sinomenine inhibits lipopolysaccharide-induced inflammatory injury by regulation of miR-101/MKP-1/JNK pathway in keratinocyte cells, Biomed. Pharmacother., 2018, 101, 422–429 CrossRef CAS PubMed.
- L. Yi, Y. Lyn and C. Peng, et al., Sinomenine inhibits fibroblast-like synoviocyte proliferation by regulating α7nAChR expression via ERK/Egr-1 pathway, Int. Immunopharmacol., 2018, 56, 65–70 CrossRef CAS PubMed.
- M. F. Yue, X. Y. Zhang and Y. N. Dou, et al., Gut-sourced vasoactive intestinal polypeptide induced by the activation of α7 nicotinic acetylcholine receptor substantially contributes to the anti-inflammatory effect of sinomenine in collagen-induced arthritis, Front. Pharmacol., 2018, 9, 1–13 CrossRef PubMed.
- L. Yi, J. Ke and J. Liu, et al., Sinomenine increases adenosine A2A receptor and inhibits NF-κB to inhibit arthritis in adjuvant-induced-arthritis rats and fibroblast-like synoviocytes through α7nAChR, J. Leukocyte Biol., 2021, 110, 1113–1120 CrossRef CAS PubMed.
- Y. Liu, C. Zhao and Q. Ma, et al., Sinomenine retards LPS-elicited inflammation via down-regulating CCAT1 in HaCaT cells, Life Sci., 2019, 233, 1–9 Search PubMed.
- K. Liao, X. Su and K. Lei, et al., Sinomenine protects bone from destruction to ameliorate arthritis via activating p62Thr269/Ser272-Keap1-Nrf2 feedback loop, Biomed. Pharmacother., 2021, 135, 1–11 CrossRef PubMed.
- R. B. Yao, Z. M. Zhao and L. J. Zhao, et al., Sinomenine inhibits the inflammatory responses of human fibroblast-like synoviocytes via the TLR4/MyD88/NF-κB signaling pathway in rheumatoid arthritis, Pharmazie, 2017, 72(6), 355–360 CAS.
- L. Weiwei, Q. Xian and J. Wei, et al., Effects and safety of Sinomenine in treatment of rheumatoid arthritis contrast to methotrexate: a systematic review and Meta-analysis, J. Tradit. Chin. Med., 2016, 36(5), 564–577 CrossRef.
- Q. Wang and X. K. Li, Immunosuppressive and anti-inflammatory activities of sinomenine, Int. Immunopharmacol., 2011, 11(3), 373–376 CrossRef CAS PubMed.
- Z. Shu, Y. Cao and Y. Tao, et al., Polyvinylpyrrolidone microneedles for localized delivery of sinomenine hydrochloride: preparation, release behavior of in vitro & in vivo, and penetration mechanism, Drug Delivery, 2020, 27(1), 642–651 CrossRef CAS PubMed.
- X. Chen, D. Li and H. Zhang, et al., Sinomenine-phenolic acid coamorphous drug systems: Solubilization, sustained release, and improved physical stability, Int. J. Pharm., 2021, 598, 1–11 CrossRef PubMed.
- H. Song, J. Wen and H. Li, et al., Enhanced transdermal permeability and drug deposition of rheumatoid arthritis via sinomenine hydrochloride-loaded antioxidant surface transethosome, Int. J. Nanomed., 2019, 14, 3177–3188 CrossRef CAS PubMed.
- S. Li, M. Gao and X. Nian, et al., Design, Synthesis, Biological Evaluation and Silico Prediction of Novel Sinomenine Derivatives, Molecules, 2021, 26(11), 3466 CrossRef CAS PubMed.
- Z. Zhang, H. Wang and J. Yuan, et al., Design, synthesis, and pharmacological evaluation of sinomenine derivatives on rings A and C: Novel compounds screening for aplastic anemia targeting on cytotoxic T lymphocyte, Eur. J. Med. Chem., 2021, 225, 113791 CrossRef CAS PubMed.
- Y. R. Zhou, Y. Zhao, B. H. Bao and J. X. Li, SND-117, a sinomenine bivalent alleviates type II collagen-induced arthritis in mice, Int. Immunopharmacol., 2015, 26(2), 423–431 CrossRef CAS PubMed.
- Z. Zhao, J. Xiao and J. Wang, et al., Anti-inflammatory effects of novel sinomenine derivatives, Int. Immunopharmacol., 2015, 29(2), 354–360 CrossRef CAS PubMed.
- X. Chai, Z. Guan and S. Yu, et al., Design, synthesis and molecular docking studies of sinomenine derivatives, Bioorg. Med. Chem. Lett., 2012, 22(18), 5849–5852 CrossRef CAS PubMed.
- A. C. L. Leite, F. F. Barbosa and M. V. O. Cardoso, et al., Phthaloyl amino acids as anti-inflammatory and immunomodulatory prototypes, Med. Chem. Res., 2014, 23(4), 1701–1708 CrossRef CAS.
- L. Pang, C. Y. Liu and G. H. Gong, et al., Synthesis, in vitro and in vivo biological evaluation of novel lappaconitine derivatives as potential anti-inflammatory agents, Acta Pharm. Sin. B, 2020, 10(4), 628–645 CrossRef CAS PubMed.
- Y. Yang, Q. Zhu and Y. Zhong, et al., Synthesis, anti-microbial and anti-inflammatory activities of 18β-glycyrrhetinic acid derivatives, Bioorg. Chem., 2020, 101, 103985 CrossRef CAS PubMed.
- H. V. Robles, K. F. C. Ochoa and P. Nava, et al., Analyzing beneficial effects of nutritional supplements on intestinal epithelial barrier functions during experimental colitis, J. Visualized Exp., 2017, 119, 1–10 Search PubMed.
- F. He, C. Wu and P. Li, et al., Functions and signaling pathways of amino acids in intestinal inflammation, BioMed Res. Int., 2018, 2018, 1–9 Search PubMed.
- C. Wu, X. Qin and H. Du, et al., The immunological function of GABAergic system, Front. Biosci., 2017, 22, 1162–1172 CrossRef CAS PubMed.
- R. Zhao, X. Zhang and G. Wu, et al., Synthesis optimization of 2-chloromethyl-3, 5, 6-trimethylpyrazine derivative as the intermediate of ligustrazine, Northwest Pharm. J., 2018, 03, 380e384 Search PubMed.
- S. B. Park, G. H. Park and Y. Um, et al., Wood-cultivated ginseng exerts anti-inflammatory effect in LPS-stimulated RAW264. 7 cells, Int. J. Biol. Macromol., 2018, 116, 327–334 CrossRef CAS PubMed.
- W. Liu, Z. Song and H. Wang, et al., Diterpenoids as potential anti-inflammatory agents from Ajuga pantantha, Bioorg. Chem., 2020, 101, 103966 CrossRef CAS PubMed.
- J. Jin, P. Teng and H. L. Liu, et al., Microfluidics assisted synthesis and bioevaluation of sinomenine derivatives as antiinflammatory agents, Eur. J. Med. Chem., 2013, 62, 280–288 CrossRef CAS PubMed.
- S. Mitchell, J. Vargas and A. Hoffmann, Signaling via the NFκB system, Wiley Interdiscip. Rev.: Syst. Biol. Med., 2016, 8(3), 227–241 CAS.
- I. Posadas, M. Bucci and F. Roviezzo, et al., Carrageenan-induced mouse paw oedema is biphasic, age-weight dependent and displays differential nitric oxide cyclooxygenase-2 expression, Br. J. Pharmacol., 2004, 142(2), 331–338 CrossRef CAS PubMed.
- S. Abubakar, M. A. Al-Mansoub and V. Murugaiyah, et al., The phytochemical and anti-inflammatory studies of Dillenia suffruticosa leaves, Phytother. Res., 2019, 33(3), 660–675 CrossRef CAS PubMed.
- N. Liu, G. X. Zhang and Y. T. Niu, et al., Anti-inflammatory and analgesic activities of indigo through regulating the IKKβ/IκB/NF-κB pathway in mice, Food Funct., 2020, 11(10), 8537–8546 RSC.
- R. Tesfaye, A. Degu and B. Abebe, et al., Evaluation of Analgesic and Anti-inflammatory Potential of 80% Methanol Leaf Extract of Otostegia integrifolia Benth (Lamiaceae), J. Inflammation Res., 2020, 13, 1175 CrossRef CAS PubMed.
|
This journal is © The Royal Society of Chemistry 2022 |
Click here to see how this site uses Cookies. View our privacy policy here.