DOI:
10.1039/D2RA05778F
(Paper)
RSC Adv., 2022,
12, 35199-35205
Facile preparation of urchin-like NiCo2O4 microspheres for efficient hydrogen peroxide detection†
Received
13th September 2022
, Accepted 23rd November 2022
First published on 8th December 2022
Abstract
In this work, urchin-like NiCo2O4 microspheres were prepared via a facile ionic liquid-assisted hydrothermal synthesis and used as non-enzymatic H2O2 sensors for the first time. The porous structure and high surface area of the NiCo2O4 microspheres provide plentiful active sites for electrocatalytic H2O2 oxidation. When adapted into an electrochemical sensor for H2O2, the microsensors showed fast response of 4 s, a high sensitivity of 392.5 μA·mM−1 cm−2, and a wide linear range towards H2O2 (0–14 mM). The detection limit was as low as 0.05 μM, significantly lower than other published high performance NiCo2O4-based H2O2 sensors. Furthermore, this non-enzymatic sensor exhibits good selectivity for H2O2. These results suggest that NiCo2O4 microspheres could be a promising material for trace H2O2 detection.
Introduction
H2O2 as a strong oxidant, has been widely used in the chemical industry, medical diagnosis, food safety and environmental analysis fields.1–3 H2O2 also plays an important role in living organisms as a reactive oxygen species by driving oxidative biochemical processes and maintaining physiological redox homeostasis.4 Excessive accumulation of H2O2 can cause many diseases, such as Alzheimer's disease, cardiovascular disorders, cancer, and Parkinson's.5–8 Therefore, the development of H2O2 detection technologies is critical for disease-related diagnostics.
At present, H2O2 is typically detected using techniques such as fluorescence assays, chemiluminescence, and electrochemical method.9–11 Among them, electrochemical method is considered one of the most promising method due to its low cost, fast response time, and high sensitivity.12 Electrochemical sensors generally utilize enzymatic or non-enzymatic electrochemical materials for H2O2 detection.13,14 Despite their high sensitivity and selectivity, enzymatic sensors can be expensive, sensitive to the surrounding environment (pH or temperature), and may have short lifespans, thus limiting their widespread practical applications.14 Consequently, non-enzymatic sensors have received more attention as potential alternative methods.
In recent years, transition metal oxides have been widely used as electrochemical sensor nanomaterials for H2O2 detection. NiCo2O4 is a typical binary metal oxide, in which the coupling of Ni and Co ions provides the NiCo2O4 with greater electronic conductivity than either single-component Co3O4 and NiO materials, thereby enhancing the overall electrochemical performance of the nanomaterials.15–17 Moreover, NiCo2O4 has high natural abundance, low cost and relatively low toxicity. Recently, research into NiCo2O4-based materials has mainly focused on the synthesis of various morphologies, such as nanoneedles, nanowalls, nanowires, nanorods, nano-sheets, hollow structures and multilevel structures.18–23 Among them, urchin-like structures have attracted extensive attention due to their high surface area, open structure and abundant active sites, all of which enhance the electrochemical performances of the nanomaterial.23 However, reports on the preparation of urchin-like NiCo2O4 microspheres by an ionic liquid-assisted hydrothermal synthesis pathway has not been published.
Ionic liquids are often composed of hydrophobic organic cations and hydrophilic inorganic anions and can be an environmentally friendly green solvent, they are widely used as solvents and templates in the synthesis of nanomaterials.24,25 In this work, urchin-like NiCo2O4 microspheres prepared via a facile ionic liquid-assisted hydrothermal synthesis are used as non-enzymatic H2O2 sensors for the first time. These NiCo2O4 microspheres exhibited excellent electrochemical properties and sensitivity towards H2O2 detection, demonstrating that the as-prepared hierarchical urchin-like NiCo2O4 microspheres could have potential applications as a non-enzymatic H2O2 sensor.
Experimental
Materials and reagents
NiCl2·6H2O, CoCl2·6H2O, urea (CO(NH2)2) and NaOH were provided by Tianjin Kemiou Chemical Reagent Co. Ltd. 1-Ethyl-3-methylimidazolium nitrate ([EMIm]NO3) was provided by Lanzhou Institute of Chemical Physics, CAS. H2O2 (30%, w/w) was supplied by Chongqing Wansheng Chuandong Chemical Co. Ltd. Nafion (5 wt% in ethanol) was provided by Aldrich. KCl, uric acid (UA), ascorbic acid (AA) and glucose were supplied by Aladdin Co. Ltd. All reagents were of analytical grade.
Preparation of NiCo2O4
The NiCo2O4 microspheres were prepared using an ionic liquid-assisted hydrothermal method. First, 0.1 mol L−1 CoCl2·6H2O and 0.05 mol L−1 NiCl2·6H2O were dissolved in 20 mL deionized water. Second, 0.05 mol L−1 1-ethyl-3-methimidazole nitrate and 1.25 mol L−1 urea was added and stirred for 0.5 h. Next, the mixture was transferred into a stainless steel autoclave and reacted at 100 °C for 10 h. Finally, the precipitates were collected by centrifugation, dried under overnight, and calcined in air at 400 °C for 2 h.
Characterization
The morphology of products was observed using a Hitachi S-4800 Scanning electron microscopy (SEM) and ZEISS SUPRA 55 transmission electron microscopy (TEM). The annealing temperature was obtained in air by a thermogravimetric analyzer (PerkinElmer 6300). The phase structure of product was obtained using a Panalytical Empyrean X-ray diffraction spectrometer (XRD). X-ray photoelectron spectroscopy (XPS) was performed using a KRATOS AXIS ULTRA. The porosity and surface area were studied by N2 adsorption (ASAP2460).
Electrochemical measurements
Electrochemical experiments using a three-electrode system on CHI760E electrochemical workstation (Shanghai Chenhua Instrument Corporation, China) in 0.1 mol L−1 NaOH solution. Pt mesh and Hg/HgO (1 mol L−1 KCl) electrode were used as the counter electrode and reference electrode, for the fabrication of working electrode, a rotating-disk electrode (RDE, 5 mm) was polished with 0.03 μm Al2O3 slurry, followed by washing in sequence with aqueous nitric acid solution (1
:
3), water and ethanol. 2.0 mg of NiCo2O4 microspheres mixed with 50 μL Nafion was dispersed in 450 μL isopropanol aqueous solution (1
:
3 vol/vol isopropanol/water) and sonicated for 1 h. Finally, the well-dispersed ink (10 μL) was drop-casted onto the well-polished RDE surface, and then naturally dried to obtain working electrode (NiCo2O4/RDE). RDE rotation speed was 1600 rpm.
Results and discussion
Characterization of materials
To investigate the initial growth and formation mechanisms of the NiCo2O4 urchin-like microspheres, a detailed time course study was carried out. The synthetic reaction was monitored for up to 10 h, and scans of the related products at various time intervals (Fig. S1a–e†). After reacting for 2 h, a small number of nanowires clustered within the crystal nucleus into a broom shape (Fig. S1a†). Loose sea urchin-like structures gradually to form between 4–8 h (Fig. S1b–d†). When the reaction time was increased to 10 h, the urchin-like microspheres could eventually be obtained (Fig. S1e†). From this sequence, a possible formation mechanism is proposed as follows: first, the ionic liquids can exhibit easily aggregate and form micelles in solution.25 After further adding urea into the Ni2+ and Co2+ containing water solution, the OH− anion slowly released during urea hydrolysis, then the metal cations (Co2+ and Ni2+) reacted with the OH− anion to form the NiCo2(OH)6 nucleus.26 The large number of NiCo2(OH)6 nuclei were easily adsorbed on micellar surfaces of ionic liquid due to the low surface energy of ionic liquids. These nascent crystal nuclei further grow and self-assemble into nanorods, and eventually form the precursor of urchin-like NiCo2O4 microspheres. After calcination in air away the ionic liquid, the NiCo2(OH)6 are eventually decomposed into NiCo2O4 oxides, causing development of urchin-like NiCo2O4 hollow microspheres (Fig. 2e). Notably, in the absence of the ionic liquid [EMIm]NO3, only NiCo2O4 solid microspheres were obtained by hydrothermal synthesis (Fig. S1f and g†). Therefore, the ionic liquid [EMIm]NO3 is essential for the formation of the urchin-like microspheres. The relevant reactions are as follows:26,27 |
6CO(NH2)2 → C3N6H6 + 6NH3 + 3CO2
| (1) |
|
NH3 + H2O → NH4+ + OH−
| (2) |
|
Ni2+ + 2Co2+ + 6OH− → NiCo2(OH)6
| (3) |
|
2NiCo2(OH)6 + O2 → 2NiCo2O4 + 6H2O
| (4) |
The optimal calcination temperature of the precursor was determined by thermogravimetric analysis (TG). According to the TG analysis (Fig. 1a), the 3.5 wt% loss below 200 °C due to dehydration. There was a significant reduction in wt% between 200 °C to 400 °C (22.2 wt%), indicating the decomposition of the precursor. Beyond 400 °C, the weight remains constant, indicating that the formation of a stable product. Therefore, the calcination temperature chosen for this study was 400 °C for 2 h.
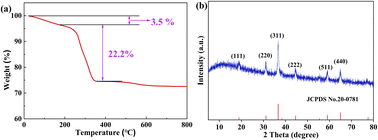 |
| Fig. 1 (a) Thermogravimetric analysis of precursor. (b) XRD diagram of NiCo2O4. | |
The phase structures of the calcined product were obtained by XRD measurements (Fig. 1b). The XRD pattern exhibited six characteristic diffraction peaks at 18.9°, 31.1°, 36.7°, 44.6°, 59.1° and 64.9°. All these peaks can be attributed to the (111), (220), (311), (222), (511) and (440) crystal planes of NiCo2O4 (JCPDS No. 20-0781), indicating successful synthesis of NiCo2O4.
The morphologies of the products were obtained by SEM (Fig. 2). The precursor displays an urchin-like microsphere with a morphology of about 5 μm and consists of smooth nanowires (Fig. 2b). After calcination, the resulting NiCo2O4 maintains the same morphology as that of the precursor (Fig. 2c and e), but the NiCo2O4 microspheres are hollow structures and the nanowires of NiCo2O4 microspheres are composed of NiCo2O4 nanoparticles 25 nm in diameter (Fig. 2d and f). Meanwhile, the lattice images of HRTEM (Fig. 2g) showed d-spacing of 0.424, 0.208, 0.144 and 0.160 nm, corresponding to (111), (400), (440) and (422) crystal planes of NiCo2O4, respectively.
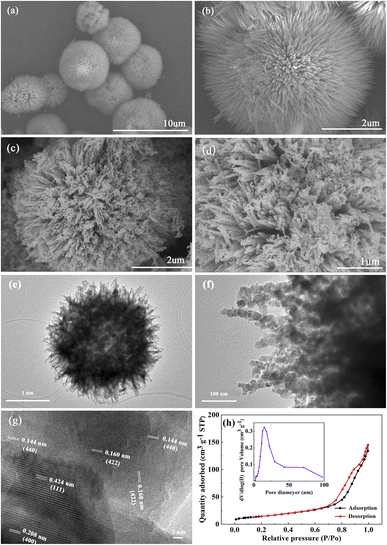 |
| Fig. 2 SEM results of precursor (a and b) and NiCo2O4 (c and d). TEM (e and f) and HRTEM (g) image of NiCo2O4. (h) N2 adsorption isotherm and the corresponding pore diameter distribution (inset) of NiCo2O4. | |
The porosity and surface area of NiCo2O4 microspheres were studied by N2 adsorption and shown in Fig. 2h. A type IV isotherm was observed, confirming the a mesoporous structure of NiCo2O4 microspheres.28 From this isotherm, the BET specific surface area was calculated as 52.15 m2 g−1, and the average pore diameter and accumulative pore volume were calculated to be 17 nm and 0.21 cm3 g−1, respectively. The high surface area and porous structure of NiCo2O4 should provide sufficient space for mass transfer, and also increase the available more active sites, both of which improve the electrocatalytic activity for H2O2 oxidation.
In order to confirm the surface chemical compositions of NiCo2O4 microspheres, we performed the XPS analysis (Fig. 3). The XPS full spectrum analysis (Fig. 3a) indicated only Ni, Co, O, and C in the NiCo2O4 microspheres. The Ni 2p spectra (Fig. 3b) shows two major peaks and two shakeup satellites (designated as “Sat.”). The binding energies at 871.8 eV and 853.4 eV correspond to the Ni 2p1/2 and Ni 2p3/2, respectively. The Ni 2p1/2 and Ni 2p3/2 levels were best fitted with double peaks (Ni2+ and Ni3+) at high resolution. Similarly, the Co 2p spectra (Fig. 3c) shows the binding energy of Co 2p3/2 and Co 2p1/2 states at 779.1 eV and 794.2 eV, where the Co3+ and Co2+ valences are also clearly shown in the Co 2p3/2 and Co 2p1/2 levels. The presence of redox couples of Ni3+/Ni2+ and Co3+/Co2+ can significantly improve the electrocatalytic activity.29 In addition, the O1s fine spectrum consists of three components (Fig. 3d). The binding energies of 529.1 eV, 530.6 eV and 532.3 eV were attributed to the lattice oxygen (designated as O1), oxygen withincrystalline defects (designated as O2) and physicochemically adsorbed oxygen (designated as O3), respectively.30–32
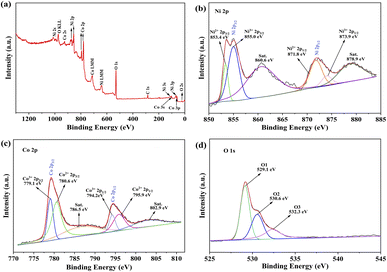 |
| Fig. 3 XPS full spectrum (a), Ni 2p (b), Co 2p (c), and O 1s (d) for NiCo2O4. | |
Electrochemical properties of NiCo2O4/RDE
Oxidation of H2O2 at the NiCo2O4/RDE. The electrochemical properties of NiCo2O4 towards H2O2 oxidation were investigated by cyclic voltammetry (CV) and shown in Fig. 4a. The CV plot of NiCo2O4/RDE shows two pairs of redox peaks in the absence of H2O2, which correspond to the redox processes of Co3+/Co2+ and Ni3+/Ni2+ redox couples in the alkaline electrolyte.33 Interestingly, upon addition of H2O2 into the NaOH electrolyte, the peak current of NiCo2O4/RDE increased significantly. This increased current correlated with increases in the H2O2 concentration (Fig. 4b). As a control, the CV obtained from a bare RDE is also shown in Fig. 4a.
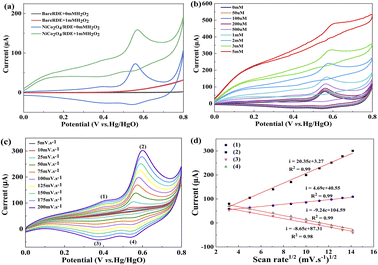 |
| Fig. 4 (a) CVs of NiCo2O4/RDE with the absence and presence of 1 mM H2O2 in 0.1 M NaOH solution (scan rate 50 mV s−1). (b) CVs of NiCo2O4/RDE with the addition of different amounts of H2O2 (scan rate 50 mV s−1). (c) CVs of NiCo2O4/RDE at different scan rates with the presence of 1 mM H2O2. (d) The plot of cathodic and anodic peak currents vs. scan rates. | |
The bare RDE only exhibited a weaker double-layer charging current, and does not show any redox peaks. These results suggested that the as-prepared NiCo2O4 microspheres are redox active and show electrocatalytic activity towards H2O2 oxidation. Furthermore, the CVs of NiCo2O4/RDE were studied at different scan rates (Fig. 4c). The anodic and cathodic peak currents increase with an increasing scanning rates, and the peak currents were directly proportional to the square root of scan rates (Fig. 4d). The above results suggested that the electrocatalytic/RDE was controlled by diffusion.33 A possible catalytic mechanism involving NiCo2O4 is proposed as follows:33
|
NiCo2O4 + H2O + OH− → 2CoOOH + NiOOH + e−
| (5) |
|
2CoOOH + 3H2O2 → 2Co(OH)2 + 2H2O + 2O2
| (6) |
|
2NiOOH + 3H2O2 → 2Ni(OH)2 + 2H2O + 2O2
| (7) |
Amperometric response of the NiCo2O4/RDE towards H2O2. We investigated the effect of the applied potential and catalyst loading on the amperometric response of NiCo2O4/RDE towards H2O2 oxidation as shown in Fig. S2.† A potential of 0.58 V was selected as the optimal applied potential by comparing the noise current and sensitivity (Fig. S2a and b†). The sensitivity and linear range reached a maximum value when 200 μg cm−2 NiCo2O4 was loaded onto the RDE (Fig. S2c and Table S1†). Therefore, this amount was used in the following studies.The steady state amperometric response of NiCo2O4/RDE to successive additions of H2O2 at 0.58 V are shown in Fig. 5a. The NiCo2O4/RDE responded immediately following H2O2 addition, and the current stabilized within 4 s after adding H2O2 into the NaOH solution, suggesting that the NiCo2O4/RDE has a rapid and sensitive response behavior to H2O2. The response current was measured as a function of H2O2 concentration, which exhibited a linear response between 0 μM to 14 mM (Fig. 5b). The calculated sensitivity and detection limit of H2O2 from these measurements were 392.50 μA mM−1 cm−2 and 0.05 μM (S/N = 3), respectively. This marks a significant decreased in detection limit in comparison to previously reported NiCo2O4-based H2O2 nonenzymatic biosensors (Table 1). The rapid and sensitive response of the NiCo2O4 urchin-like microspheres was attributed to their unique hierarchical architecture, as shown in Fig. 5c. The NiCo2O4 urchin-like microsphere consists of nanowires and abundant pores, which not only are beneficial to the transport of H2O2 and electrolyte, but also allow the oxygen to rapidly escape from the surface of the catalyst, and the H2O2 and electrolyte to effectively infiltrate into the catalyst for continuing catalysis processes.
 |
| Fig. 5 (a) Amperometric response of NiCo2O4/RDE at 0.58 V and its corresponding calibration plot (b). (c) Schematic illustration of the oxidation process of H2O2 on the NiCo2O4. (d) Amperometric response of NiCo2O4/RDE in 0.1 M NaOH buffer following sequential addition of 1 mM H2O2, 0.1 mM KCL, 1 mM H2O2, 0.1 mM urea, 1 mM H2O2, 0.1 mM UA, 1 mM H2O2, 0.1 mM AA, 1 mM H2O2, 0.1 mM glucose. | |
Table 1 The performance comparison of our proposed with other published for H2O2 sensors
Material |
Linear ranges (mM) |
Detection limit (μM) |
Ref. |
NiCo2O4 |
0–14 |
0.05 |
This work |
NHGH/NiCo2O4 |
0.001–0.51 |
0.136 |
33 |
ZnO/Co3O4/NiCo2O4/Ni foam |
0.0002–2.4 |
0.163 |
16 |
Co3O4/NCNTs |
0.005–11 |
1 |
34 |
Co3O4/NiCo2O4 |
0.05–41.7 |
0.2578 |
35 |
NiO/Ti3C2Tx |
0.01–4.54 |
0.348 |
36 |
PbS/Co3O4 |
0.005–0.25 |
1.2 |
37 |
NixCoy-DO |
0–0.4 |
0.28 |
38 |
NiCo2O4/CoNiO2@pRGO600 |
0.005–3, 3–12 |
0.41 |
39 |
NiO-MNS |
0.01–0.8 |
0.62 |
40 |
Ni/NiO@C |
0.05–6.7, 8.7–80.7 |
0.9 |
41 |
Assessing the selectivity and stability of the NiCo2O4/RDE sensor for H2O2 detection. To investigate the selectivity of the NiCo2O4/RDE sensor for H2O2 detection, the amperometric responses of NiCo2O4/RDE to H2O2 were studied in the presence of common biological interfering compounds, including KCl, urea, AA, UA and glucose (Fig. 5d). These results demonstrate that there is almost a negligible amperometric response towards all reagents except for H2O2, which shows a significant amperometric response. Therefore, the NiCo2O4 microspheres appear to be excellent selective for H2O2 detection. In order to assess the reproducibility of NiCo2O4/RDE as athe H2O2 sensor, five different NiCo2O4/RDE were independently fabricated by the same method. The amperometric responses of these electrodes to addition of 1 mM H2O2 were recorded. As a result, the relative standard deviation (RSD) of the response current was 5.4% (n = 5). The NiCo2O4/RDE sensor maintains 98.1% of its initial amperometric response upon addition of 1 mM H2O2 after storage in air for 15 days. For each individual sensor, the RSD in measured current response from fifteen successive measurments 0.43% (n = 15). Moreover, the stability of urchin-like morphology after the fifteen successive test was further checked by TEM and HRTEM analysis. The TEM image clearly show the urchin-like morphology, even after the fifteen successive test (Fig. S1h†). The lattice images of HRTEM (inset of Fig. S1h†) showed d-spacing of 0.246, 0.234 and 0.208 nm, corresponding to (311), (222) and (400) crystal planes of NiCo2O4, respectively. All these results suggest that the sensor had good reproducibility, repeatability and stability for the H2O2 detection.
Conclusion
In conclusion, urchin-like NiCo2O4 microspheres were successfully fabricated via a relatively simple ionic liquid-assisted hydrothermal method and were used as an effective electrochemical sensor for H2O2. The results demonstrate that the urchin-like NiCo2O4 microspheres had structural properties that were ideal for its use as a non-enzymatic H2O2 sensor. The H2O2 sensor based on NiCo2O4 microspheres exhibited a fast detection response, a wide linear range, and high sensitivity towards H2O2. The high H2O2 detection performance achieved by NiCo2O4 in this study suggests these microspheres could have promising potential applications as H2O2 sensors in the medical diagnosics and biotechnology sectors.
Author contributions
Jiaojiao Jiang: data curation, writing – original draft. Zhixuan Zhang: validation. Chong Yang: conceptualization, data curation. Rui Wang: methodology, data curation. Zhouling Wu: project administration, writing – review & editing.
Conflicts of interest
There are no conflicts to declare.
Acknowledgements
This work was supported by the Youth Program of National Natural Science Foundation of China (22102221), the Guizhou Provincial Natural Science Foundation (QKH-J[2019]1339 and QKH-J[2020]1Z006), and the projects of Zunyi Medical University (F-943).
Notes and references
- M. Asif, H. T Wang, D. Shuang, A. Aziz, G. A. Zhang, F. Xiao and H. F. Liu, Metal oxide intercalated layered double hydroxide nanosphere: with enhanced electrocatalytic activity towards H2O2 for biological application, Sens. Actuators, B, 2017, 239, 243–252 CrossRef CAS.
- Y. Shu, B. Li, Q. Xu, P. Gu, X. Xiao, F. P. Liu, L. Y. Yu, H. Pang and X. Y. Hu, Cube-like CoSn(OH)6 nanostructure for sensitive electrochemical detection of H2O2 in human serum sample, Sens. Actuators, B, 2017, 241, 528–533 CrossRef CAS.
- B. Sherino, S. Mohamad, S. N. A. Halim and N. S. A. Manan, Electrochemical detection of hydrogen peroxide on a new microporous Ni-metal organic framework material-carbon paste electrode, Sens. Actuators, B, 2018, 254, 1148–1156 CrossRef CAS.
- M. M. Liu, R. Liu and W. Chen, Graphene wrapped Cu2O nanocubes: Non-enzymatic electrochemical sensors for the detection of glucose and hydrogen peroxide with enhanced stability, Biosens. Bioelectron., 2013, 25, 206–212 CrossRef PubMed.
- T. Shi, D. M. K. V. Soest, P. E. Polderman, B. M. T. Burgering and T. B. Dansen, DNA damage and oxidant stress activate p53 through differential upstream signaling pathways, Free Radical Biol. Med., 2021, 172, 298–311 CrossRef CAS PubMed.
- Y. J. Sun, M. C. Luo, Y. N. Qin, S. H. Zhu, Y. J. Li, N. Y. Xu, X. X. Meng, Q. S. Ren, L. Wang and S. J. Guo, Atomic-thick PtNi nanowires assembled on graphene for high-sensitivity extracellular hydrogen peroxide sensors, ACS App. Mater. Interfaces, 2017, 9, 34715–34721 CrossRef CAS PubMed.
- F. Y. Xu, W. Tang, S. S. Kang, J. S. Song and X. R. Duan, A highly sensitive and photo-stable fluorescent probe for endogenous intracellular H2O2 imaging in live cancer cells, Dyes Pigm., 2018, 153, 61–66 CrossRef CAS.
- P. Cecilia, H. Silvana and D. Fiorentini, Peroxiporins in Cancer, Int. J. Mol. Sci., 2019, 20, 1371 CrossRef PubMed.
- S. Duanghathaipornsuk, E. J. Farrell, A. C. Albarubio, P. Zelenay and D. S. Kim, Detection technologies for reactive oxygen species: fluorescence and electrochemical methods and their applications, Biosensors, 2021, 11, 30 CrossRef CAS PubMed.
- B. Kalyanaraman, G. Cheng, M. Hardy, O. Ouari, B. Bennett and J. Zielonka, Teaching the basics of reactive oxygen species and their relevance to cancer biology: Mitochondrial reactive oxygen species detection, redox signaling, and targeted therapies, Redox Biol., 2018, 15, 347–362 CrossRef CAS PubMed.
- H. Cheng, Z. Y. Zhou and T. Liu, Electro-spinning fabrication of nitrogen, phosphorus co-doped porous carbon nanofiber as an electro-chemiluminescent sensor for the determination of cyproheptadine, RSC Adv., 2020, 10, 23091–23096 RSC.
- E. Dumitrescu and S. Andreescu, Bioapplications of electrochemical sensors and biosensors, Methods Enzymol., 2017, 589, 301–350 CAS.
- D. Thatikayala, D. Ponnamma, K. K. Sadasivuni, J. J. Cabibihan, A. K. Al-Ali, R. A. Malik and B. Min, Progress of Advanced Nanomaterials in the Non-Enzymatic Electrochemical Sensing of Glucose and H2O2, Biosensors, 2020, 10(11), 151 CrossRef CAS PubMed.
- S. A. Hira, M. Nallal, K. Rajendran, S. Song, S. Park, J. M. Lee, S. H. Joo and K. H. Park, Ultrasensitive detection of hydrogen peroxide and dopamine using copolymer-grafted metal-organic framework based electrochemical sensor, Anal. Chim. Acta, 2020, 1118, 26–35 CrossRef CAS PubMed.
- W. F. Liu, Z. H. Zhou, L. Yin, Y. M. Zhu, J. Zhao, B. Zhu, L. B. Zheng, Q. Jin and L. Wang, A novel self-powered bioelectrochemical sensor based on CoMn2O4 nanoparticle modified cathode for sensitive and rapid
detection of hydrogen peroxide, Sens. Actuators, B, 2018, 271, 247–255 CrossRef CAS.
- B. Xue, K. Z. Li, S. Y. Gu, L. L. Zhang and J. H. Lu, Ni foam-supported ZnO nanowires and Co3O4/NiCo2O4 double-shelled nanocages for efficient hydrogen peroxide detection, Sens. Actuators, B, 2018, 262, 828–836 CrossRef CAS.
- R. Kumar, NiCo2O4 nano-microstructures as high-performance biosensors: a review, Nano-Micro Lett., 2020, 12, 2150–5551 Search PubMed.
- Q. Guo, W. Zeng and Y. Q. Li, Highly sensitive non-enzymatic glucose sensor based on porous NiCo2O4 nanowires grown on nickel foam, Mater. Lett., 2019, 256, 126603 CrossRef CAS.
- L. J. Liu, Z. H. Wang, J. H. Yang, G. L. Liu, J. J. Li, L. Guo, S. L. Chen and Q. H. Guo, NiCo2O4 nanoneedle-decorated electrospun carbon nanofiber nanohybrids for sensitive non-enzymatic glucose sensors, Sens. Actuators, B, 2018, 258, 920–928 CrossRef CAS.
- Y. Ni, J. Xu, H. Liu and S. J. Shao, Fabrication of RGO-NiCo2O4 nanorods composite from deep eutectic solvents for nonenzymatic amperometric sensing of glucose, Talanta, 2018, 185, 335–343 CrossRef CAS PubMed.
- X. M. Zhang, Y. X. Zhao, Y. T. Wu and Z. X. Mao, Two-dimensional hexagonal NiCo2O4 nanoplates@PEDOT/RGO nanocomposite: A design and construction high selective H2O2 sensing interface, J. Electrochem. Soc., 2020, 167, 067519 CrossRef CAS.
- Q. Chen, Y. H. Zhang, S. Y. Ma, Y. H. Wang, P. Y. Wang, G. H. Zhang, D. J. Gengzang, H. Y. Jiao, M. X. Wang and W. J. Chen, Multishelled NiO/NiCo2O4 hollow microspheres derived from bimetal-organic frameworks as high-performance sensing material for acetone detection, J. Hazard. Mater., 2021, 415, 125662 CrossRef CAS PubMed.
- M. Z. Khan, J. H. Zhu and X. H. Liu, Reduced graphene oxide-conjugated urchin-like NiCo2O4 nanostructures for individual detection of o-nitro and p-amino phenol, ACS Omega, 2019, 4, 11433–11439 CrossRef CAS PubMed.
- L. J. Yang, Y. D. Hu, Q. Wang, Y. Y. Dong and L. Zhang, Ionic liquid-assisted electrochemical determination of pyrimethanil using reduced graphene oxide conjugated to flower-like NiCo2O4, Anal. Chim. Acta, 2016, 935, 104–112 CrossRef CAS PubMed.
- A. Rehman and X. Zeng, Ionic liquids as green solvents and electrolytes for robust chemical sensor development, Acc. Chem. Res., 2012, 45, 1667–1677 CrossRef CAS PubMed.
- X. M. Li, L. F. Jiang, C. Zhou, J. P. Liu and H. B. Zeng, Integrating large specific surface area and high conductivity in hydrogenated NiCo2O4 double-shell hollow spheres to improve supercapacitors, NPG Asia Mater., 2015, 7, 165–172 CrossRef.
- L. F. Shen, Q. Che, H. S. Li and X. G. Zhang, Mesoporous NiCo2O4 nanowire arrays grown on carbon textiles as binder-free flexible electrodes for energy storage, Adv. Funct. Mater., 2014, 24, 2630–2637 CrossRef CAS.
- Y. F. Zhang, M. Z. Ma, J. Yang, H. Q. Su, W. Huang and X. C. Dong, Selective synthesis of hierarchical mesoporous spinel NiCo2O4 for high-performance supercapacitors, Nanoscale, 2014, 6, 4303–4308 RSC.
- Z. Y. Yu, H. J. Li, X. M. Zhang, N. K. Liu, W. L. Tan, X. Zhang and L. L. Zhang, Facile synthesis of NiCo2O4@Polyaniline core-shell nanocomposite for sensitive determination of glucose, Biosens. Bioelectron., 2016, 161–165 CrossRef CAS PubMed.
- J. Yang, M. Cho and Y. Lee, Synthesis of hierarchical NiCo2O4 hollow nanorods via sacrificial-template accelerate hydrolysis for electrochemical glucose oxidation, Biosens. Bioelectron., 2016, 75, 15–22 CrossRef CAS PubMed.
- L. Liu, H. J. Zhang, J. Yang, Y. P. Mu and Y. Wang, Self-assembled novel dandelion-like NiCo2O4 microspheres@nanomeshes with superior electrochemical performance for supercapacitors and lithium-ion batteries, J. Mater.
Chem. A, 2015, 3, 22393–22403 RSC.
- X. X. Yu, Z. J. Sun, Z. P. Yan, B. Xiang, X. Liu and P. W. Du, Direct growth of porous crystalline NiCo2O4 nanowire arrays on a conductive electrode for high-performance electrocatalytic water oxidation, J. Mater. Chem. A, 2014, 2, 20823–20831 RSC.
- Z. W. Lu, L. Wu, J. J. Zhang, W. L. Dai, G. Q. Mo and J. S. Ye, Bifunctional and highly sensitive electrochemical non-enzymatic glucose and hydrogen peroxide biosensor based on NiCo2O4 nanoflowers decorated 3D nitrogen doped holey graphene hydrogel, Mater. Sci. Eng., C, 2019, 102, 708–717 CrossRef CAS PubMed.
- Y. N. Qin, Y. J. Sun, Y. J. Li, C. J. Li, L. Wang and S. J. Guo, MOF derived Co3O4/N-doped carbon nanotubes hybrids as efficient catalysts for sensitive detection of H2O2 and glucose, Chin. Chem. Lett., 2020, 31, 5 Search PubMed.
- T. J. Liu, X. Y. Zhang, K. Fu, N. Zhou, J. P. Xiong and Z. Q. Su, Fabrication of Co3O4/NiCo2O4 nanocomposite for detection of H2O2 and dopamine, Biosensors, 2021, 11, 452 CrossRef CAS PubMed.
- R. Rajendran, C. H. Zhao, R. Muniyandi, R. Krishnamoorthy, P. L. Zhu, W. L. Xuan, Z. X. Xu and F. Wang, Porous nickel oxide microsphere and Ti3C2Tx hybrid derived from metal-organic framework for battery-type supercapacitor electrode and non-enzymatic H2O2 sensor, Electrochim. Acta, 2019, 322, 134771 CrossRef.
- P. P. Wang, L. Cao, Y. Chen, Y. Wu and J. W. Di, Photoelectrochemical biosensor based on Co3O4 nanoenzyme coupled with PbS quantum dots for hydrogen peroxide detection, ACS Appl. Nano Mater., 2019, 2, 2204–2211 CrossRef CAS.
- Q. Li, J. Niu, M. L. Dou, Z. P. Zhang and F. Wang, Porous microtubes of nickel-cobalt double oxides as non-enzymatic hydrogen peroxide sensors, Chin. Chem. Lett., 2021, 32, 1181–1185 CrossRef CAS.
- M. H. Wang, C. B. Wang, Y. K. Liu, B. HU, L. H. He, Y. S. Ma, Z. H. Zhang, B. B. Gui and M. Di, Nonenzymatic amperometric sensor for hydrogen peroxide released from living cancer cells based on hierarchical NiCo2O4-CoNiO2 hybrids embedded in partially reduced graphene oxide, Microchim. Acta, 2020, 187, 436 CrossRef CAS PubMed.
- Q. Li, W. B. Gao, X. P. Zhang, H. T. Liu, M. L. Dou, Z. P. Zhang and F. Wang, Mesoporous NiO nanosphere: a sensitive strain sensor for determination of hydrogen peroxide, RSC Adv., 2018, 8, 13401–13407 RSC.
- X. Q. Ma, K. L. Tang, M. Y. Yang, W. B. Shi and W. X. Zhao, Metal-organic framework-derived yolk-shell hollow Ni/NiO@C microspheres for bifunctional non-enzymatic glucose and hydrogen peroxide biosensors, J. Mater. Sci., 2021, 56, 1–15 Search PubMed.
|
This journal is © The Royal Society of Chemistry 2022 |
Click here to see how this site uses Cookies. View our privacy policy here.