DOI:
10.1039/D2RA05849A
(Paper)
RSC Adv., 2022,
12, 30712-30721
Synthesis of 5-aryl-3,3′-bis-indolyl and bis-7-aza-indolyl methanone derivatives from 5-bromo-7-azaindoles via sequential methylenation using microwave irradiation, CAN oxidation, and Suzuki coupling reactions†
Received
16th September 2022
, Accepted 18th October 2022
First published on 27th October 2022
Abstract
A catalyst-free and green chemical method has been developed for the methylenation of indole and N-methyl-7-aza indoles with aqueous formaldehyde afforded respective N,N′-dimethyl-3,3′-bis-7-azaindolylmethanes under microwave irradiation in excellent yield. Subsequent oxidation of the products thus obtained, using one electron chemical oxidant CAN afforded N,N′-dimethyl-3,3′-bis-7-azaindolylmethanone derivatives in excellent yield. This resulted in methanone derivatives with halogen substitution at the aryl ring which when subjected to Suzuki coupling with aryl boronic acids furnished highly functionalized fluorescent biaryl derivatives. Plausible mechanisms, characterization including XRD, and evaluation of photophysical properties of the Suzuki coupled products are described.
Introduction
7-Azaindole and its derivatives exhibit significant biological activities and the framework has contributed to the development of new therapeutic agents.1 For example, natural products, meriolin 1 (1) and meriolin 3 (2) showed improved potency, and several FDA-approved drugs such as vemurafenib (3) are used for the treatment of metastatic melanoma. Pexidartinib (4) is approved for the treatment of giant cell tumours associated with severe morbidity. Moreover, 7-azadindole derivative NVP-QAV680 (5) is known for the treatment of allergies and Zn-azaindole complex (6) has a bright blue emitter property. To demonstrate materials applications, and new functionality to enhance the performance of 7-azaindole derivatives, some of the triaryl boron functionalized compounds such as (7) and (8) have been reported as ‘‘bifunctional materials’’ in OLEDs (Fig. 1).2
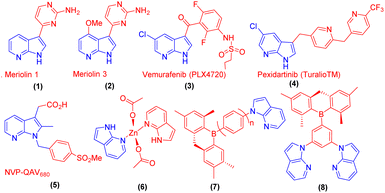 |
| Fig. 1 Drugs and materials based on 7-aza indole cores. | |
Methylenation is a well-known structural modification reaction in organic synthesis.3 Lewis acid-mediated synthesis of 3,3′-bis-7-azaindolylmethane derivatives using zinc- or acid-mediated cross-coupling reaction of 7-azaindoles with a number of diverse aldehydes provides the corresponding C3-linked methylenation products is reported.4 However, the development of the synthesis of 3-methylenation products of indoles and 7-azaindoles by green chemistry protocol using a catalyst-free, aqueous, and microwave irradiation method would be an alternate and efficient method warranted.
Oxidation of benzylic methylene with various oxidizing agents offers functional derivatives such as alcohols, aldehydes and ketones depending upon the substrates and reagents used.5 Development of synthetic methods based on utilizing green chemistry protocols such as green chemistry, clay, microwave irradiation techniques enzymatic method etc., are of current interest in the synthetic organic chemistry.6 Cerium(IV) ammonium nitrate (CAN) has emerged as a versatile reagent for a variety of synthetic transformations which have been well documented.7 Conversion of 3-methylenation products of indoles and 7-azaindoles to corresponding ketones are interesting synthetic modifications and no reports are available using mild and efficient reagents. Thus, we were interested to develop the oxidation of 3-methylenation products of indoles and 7-azaindoles using CAN as a one-electron oxidant into the corresponding ketones. Thus, herein we wish to report the details of the work carried out by initial methylenation, oxidation using CAN, and further synthetic utility of ketone products by using the Suzuki coupling reaction.
To synthesize the title compounds, a retrosynthetic analysis is shown in Scheme 1. Accordingly, the functionalized and fluorescent 3,3′-bis-7-azaindolylmethanone B derivatives were synthesized via steps involving microwave irradiated methylenation of 7-aza indoles D with aqueous formaldehyde would provide the N,N′-dimethyl-3,3′-bis-7-azaindolylmethane C followed by oxidation of compound C using CAN be followed by Suzuki coupling8 of C with aryl boronic acids.
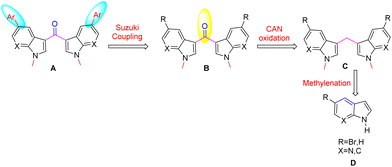 |
| Scheme 1 Retrosynthetic route for the title compounds. | |
As per the retrosynthesis shown in Scheme 1, initially, we followed the reported procedure9 for the synthesis of methylenated compound 2a′ from 7-azaindole 1 under microwave irradiation did not produce compound 2a′ but N-hydroxy methyl product in 3a′ was isolated in 44% yield and the reaction produced multiple spots and are inseparable by column chromatography. However, repeating the above reaction under sealed tube heating conditions produced 95% of a product i.e. methylated and N-hydroxy methyl product exclusively in 3a′ 95% yield (Scheme 2). The reaction suggests that when free N–H is available, under the reaction condition, it produces only N-hydroxy methyl compounds such as 3a′ and exclusive methylenated product is not observed.
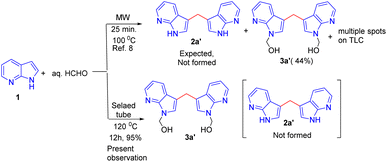 |
| Scheme 2 Initial studies. | |
Repeating the reaction of 1.0 equiv. of N-methyl-7-azaindole 2a was treated with 3.0 equiv. of 37% aq. HCHO taken in a sealed tube was heated at a slightly lower temperature of 100 °C for 12 h and gave only a trace amount of bis(1-methyl-1H-pyrrolo[2,3-b]pyridin-3-yl)methane 3a, as evidenced by spectroscopic data (Table 1, entry 1). To improve the yield of compound 3a, reactions were carried out by varying parameters such as temperature, time, and catalyst load (Table 1). Increasing the temperature to 115 °C slightly improved the yield of 3a to 35% and at 120 °C further improved the yield by 55% (Table 1, entries 2 and 3). Extending the reaction time to 15 h further improved the yield to 74% and the addition of 1 mmol amount of water to the reaction did not alter the yield (Table 1, entries 4 and 5). Repeating the reaction with paraformaldehyde at 120 °C for 12 h and 15 h afforded the expected product in 72 and 80% yields, respectively (Table 1, entries 6 and 7). Notably, to reduce the reaction time, and to improve the yield, when the mixture of 2a and aqueous formaldehyde was irradiated in a microwave oven for 15 minutes and furnished product 3a with a 45% yield. However, the best yield (85%) was obtained in a rapid reaction time of 5 min. microwave irradiation (100 °C, 150 psi, power 150) using 50% w/w montmorillonite K 10 clay as a solid acid catalyst, and this was found to be an optimum condition (Table 1, entry 9).
Table 1 Optimization of synthesis of compound 3a
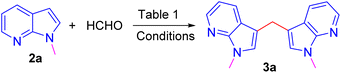
|
Entry |
Substrate |
Reagent (3.0 equiv.) |
Temp. (°C) |
Time (h) |
Yield of 3aa (%) |
Isolated yield. Irradiated at 100 W using CEM Discover-300 microwave synthesizer. |
1 |
2a |
Aq. HCHO |
100 |
12 |
Trace |
2 |
2a |
Aq. HCHO |
115 |
12 |
35 |
3 |
2a |
Aq. HCHO |
120 |
12 |
55 |
4 |
2a |
Aq. HCHO |
120 |
15 |
74 |
5 |
2a |
Aq. HCHO, H2O |
120 |
12 |
74 |
6 |
2a |
(HCHO)n |
120 |
12 |
72 |
7 |
2a |
(HCHO)n |
120 |
15 |
80 |
8 |
2a |
Aq. HCHO |
MWb |
15 min |
45 |
9 |
2a |
Aq. HCHO, 50% w/w Mont. K-10 |
MWb |
5 min |
85 |
Encouraged by the preliminary results, the scope and diversity of the reaction were explored by selecting a number of N-alkyl-7-azaindole, indole, and carbazole 2b–k (Table 2). Under optimized microwave irradiation conditions, all the reactions underwent smoothly to produce the corresponding methylenation products 3b–k in very good to excellent yields (Table 2, entries 1–7). Further, diversify the methylenation reaction compound 2c is also used as a substrate to produce a novel methylenation at C-5 position to produce compound 3c. The structure of all the products was established by spectroscopic methods such as 1H, 13C NMR, DEPT-135, and HRMS.
Table 2 Scope of the reaction for methylenationa
Entry |
Substrate 2 |
Product 3 |
MW timeb,c (min) |
Yield (%) |
Optimized condition: aq. HCHO, 50% w/w Mont. K-10, MW, 100 W. CEM Discover-300 microwave synthesizer was used. Prolonged irradiation results in decomposition or charring. |
1 |
 |
 |
5 |
74 |
2 |
 |
 |
8 |
80 |
3 |
 |
 |
8 |
85 |
4 |
 |
 |
5 |
84 |
5 |
 |
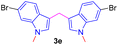 |
5 |
82 |
6 |
 |
 |
6 |
80 |
7 |
 |
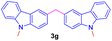 |
12 |
79 |
8 |
 |
 |
9 |
75 |
9 |
 |
 |
7 |
78 |
10 |
 |
 |
10 |
80 |
11 |
 |
 |
10 |
75 |
The structure of new compounds was assigned from spectroscopic data and a representative compound 3b structure and relative stereochemistry were assigned based on single-crystal X-ray analysis (Fig. 2).10
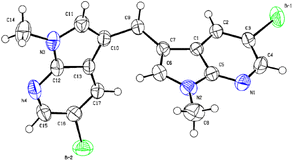 |
| Fig. 2 ORTEP diagram of compound 3b (CCDC-2191474†). | |
A plausible mechanism for the formation of bis(1-methyl-1H-pyrrolo[2,3-b]pyridin-3-yl)methane 3a from N-methyl-7-aza indole 2a with formaldehyde is shown in Scheme 3.11 Under the influence of Mont. K-10 clay, the electrophilicity of the formaldehyde is increased and undergoes a facile conjugate addition of 7-azaindole to provide hydroxy methylated intermediate E. Intermediate E upon elimination of water molecule via intermediate F to provide another intermediate G. Second addition of 7-azaindole to the intermediate G provides the product 3a.
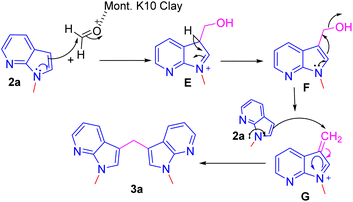 |
| Scheme 3 A plausible mechanism for the formation of compounds 3a. | |
To demonstrate scalability of the reaction a gram scale experiment has been carried out using substrate 2b to afford the expected product in 74% yield (Scheme 4).
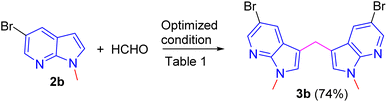 |
| Scheme 4 Gram scale synthesis of compound 3b. | |
Direct oxidation of bis(1-methyl-1H-pyrrolo[2,3-b] pyridin-3-yl) methane 3a into corresponding ketones 4a are unknown in the literature. However, these ketones were reported by a two-step synthetic protocol starting from respective methylenes.8,12 Hence, we were interested to develop a one-pot, mild and efficient procedure for this direct oxidation of 3a into its ketone 4a. To achieve the oxidation, initially, the benzylic oxidation of methylene carbon in compound 3a was treated with peroxides such as 30% H2O2, and TBHP failed to provide ketone 4a (Table 3, entries 1 and 2). To our dismay, another well-known oxidizing agent SeO2 also failed to provide the ketone (Table 3, entry 3). In addition, Cs2CO3 also failed to provide the expected product (Table 3, entry 4).13 However, a single electron chemical oxidant such as CAN was explored (Table 3, entry 5). Hence ketone 4a was contained in 70% yield by reacting the compound 3a with 3 equivalents of CAN in ACN–MeOH (1
:
3) solvent system for 30 minutes and was found as a suitable and optimized condition for the oxidation reaction. To diversify the methodology, Further under optimized condition compound 4c was synthesized from the respective 3-bromo-N-methyl-5-methyledine-bis-7-azaindole.
Table 3 Synthesis and optimization of oxidation of compound 3a using various oxidizing agents
Having followed the optimized CAN oxidizing condition, ketones 4a–e were prepared in excellent yield from respective methylated compounds 3a–e (Fig. 3).
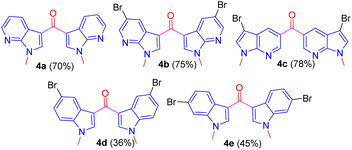 |
| Fig. 3 Synthesized ketones 4a–e. | |
The structure of all the new compounds 4a–e was assigned from spectroscopic data and a representative compound 4a structure and relative stereochemistry were assigned based on single-crystal X-ray analysis (Fig. 4).14
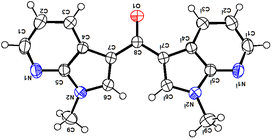 |
| Fig. 4 ORTEP diagram of compound 4a (CCDC-2102023†). | |
A plausible mechanism for the formation of compound 4 from the bis(1-methyl-1H-pyrrolo[2,3-b] pyridin-3-yl) methane 3a by CAN is shown in Scheme 5.12e Initially, the CAN oxidizes the benzylic methylene group to benzylic radical cation intermediate I, which further undergoes second oxidation by CAN and by liberating H+ ion to form the cation intermediate J. Intermediate J upon reaction with nitrate oxygen of CAN forms C–O bond intermediate K, and subsequent hydride ion elimination results the ketone 4 (Scheme 5).
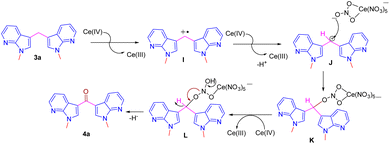 |
| Scheme 5 A plausible mechanism for the formation of compounds 4a. | |
The presence of bromine in product 4b prompted us to derivatize its biphenyl derivatives by exploiting Suzuki coupling. Biphenyls and their derivatives are important structural motifs due to applications both in academic interest and in synthetic use of various carbo- and heterocyclic frameworks.15 The asymmetric structural framework of biaryls has been effectively utilized as asymmetric catalysts, natural product synthesis, supramolecular assemblies, and organic materials.16 Thus, the halogen on the aromatic substitution was successfully utilized for Suzuki coupling with several aryl boronic acids 5a–e to provide aryl-substituted fluorescent 7-aza indole derivatives 6a–e in excellent yield (Scheme 6).17
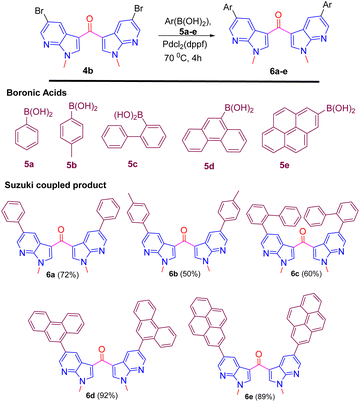 |
| Scheme 6 Suzuki coupling of compound 4b with several aryl boronic acids 5a–e. | |
The nature of biaryl-based derivatives 6a–e prompted us to evaluate the basic photophysical properties. The absorption measured in MeOH showed in the range of λmax.abs. 230–248, emission in the range of λmax.emi. 398–497, and large Stoke's shift (Table 4) has been evaluated. This basic data suggest that they are strong blue emitters (Table 4).
Table 4 Absorption and emission properties of compounds 3a, 4b, and 6a–e
Entry |
Product |
Absorptiona λmax.abs. |
Emissiona λmax.emi. |
Stoke's shift Δῡb (cm−1) |
All the spectra were recorded in MeOH at 2 × 10−5 M at 298 K concentration. Stoke's shift = λmax.abs. − λmax.emi. [cm−1]. |
1 |
3b |
232, 299 |
412 |
18 831, 9173 |
2 |
4b |
282, 316 |
398 |
10 335, 6520 |
3 |
6a |
244, 326 |
406 |
16 353, 6044 |
4 |
6b |
249, 328 |
463 |
18 562, 8889 |
5 |
6c |
230, 324 |
408 |
18 969, 6355 |
6 |
6d |
252, 325 |
497 |
19 562, 10 649 |
7 |
6e |
274, 343 |
450 |
14 274, 6932 |
In conclusion, we have demonstrated a catalyst-free and green chemical method has been developed for the methylenation of indole and N-methyl-7-aza indoles with aqueous formaldehyde afforded respective N,N′-dimethyl-3,3′-bis-7-azaindolylmethane under MW in excellent yield. Subsequent oxidation of product using one electron chemical oxidant CAN afford N,N′-dimethyl-3,3′-bis-7-azaindolylmethanone derivatives in excellent yield. This resulted in methanone derivatives with halogen substitution at the aryl ring subjected to Suzuki coupling with aryl boronic acids furnished with highly functionalized biaryl derivatives. Plausible mechanisms, characterization including XRD, and evaluation of photophysical properties of the Suzuki coupled products are reported.
Experimental section
General remarks
All the reactions were carried out in oven-dried glassware. CEM Discover-300 microwave synthesizer was used for all the microwave irradiation reactions. The progress of the reactions was monitored by thin layer chromatography (TLC) using Merck pre-coated TLC plates (Merck 60 F254) Purification of the products was accomplished by column chromatography packed with silica gel 100–200 mesh. NMR spectra were recorded on a Bruker-400.3 MHz NMR spectrometer (400.3 MHz for 1H NMR and 100.6 MHz for 13C NMR) with CDCl3/TMS as the solvent and TMS as the internal reference. Integrals are in accordance with assignments and coupling constants are reported in Hertz (Hz). All 13C spectra are proton-decoupled. Multiplicity is indicated as follows: s (singlet), d (doublet), t (triplet), q (quartet), m (multiplet), dd (doublet of doublets), dt (doublet triplet), td (triplet of doublets), and br s (broad singlet). FTIR spectra were recorded on a PerkinElmer RX-IFT-IR spectrometer and absorbance values are reported in cm−1. ESI HRMS was performed on a Waters(R) Micromass(R) Q-TOF MicroTM mass spectrometer. Yields refer to quantities obtained after chromatography.
Experimental procedure
a. General procedure for the synthesis of methylated compounds 2a–c. In a 100 mL RB flask, under N2 atm., compound 1a–c (100 mg, 0.848 mmol) in DMF was treated with 1.1 equiv. of sodium hydride (NaH, 60% dispersed in paraffin oil) at 0 °C, and the mixture was allowed to attain RT (ca. 1 h), then 1.2 equiv. of methyl iodide was added. The reaction continued to stir at RT and the progress of the reaction was monitored by TLC. After completion of the reaction, the crude mixture was extracted with ethyl acetate and washed with distilled water and saturated brine solution. The organic phase was dried over anhydrous MgSO4 and the solvent was removed under vacuum. The crude product was purified over silica gel (100–200 mesh) column chromatography using gradient elution with EtOAc–hexane to obtain the pure products 2a–c.
b. General procedure for the synthesis of compounds 2d–f18. In a 100 mL RB flask, 1.2 equiv. powdered KOH was taken and DMF (2 mL) of solvent was added and allowed to stir for 5 min. at RT. Followed by compounds 1d–f (100 mg, 0.510 mmol) was added portion-wise and allowed to stir under N2 atm., for 5 min. The entire setup was cooled to 0 °C and CH3I (1.5 equiv.) was added dropwise via syringe over a period of 5 minutes. Warm the reaction mixture to RT. After completion of the reaction, the crude reaction mixture was quenched with saturated cold brine solution and extracted with EtOAc. The organic phase was dried over anhydrous MgSO4 and the solvent was removed under vacuum. The crude product was purified over a column chromatography of silica gel (100–200 mesh) using gradient elution with EtOAc–hexane to obtain the pure products 2d–f.
c. General procedure for the synthesis of compounds 2g19. In a 100 mL RB flask, carbazole 1g (100 mg, 0.598 mmol) in THF and potassium tert-butoxide (1.5 equiv.) were added and allowed to stir for 5 min., followed by iodomethane (1.5 equiv.) was added to the reaction mixture. Heat the reaction mixture at 50 °C for 2 h. Evaporated the solvent under reduced pressure and added water to the reaction mixture. A solid thus formed was collected by filtration and washed with cold methanol to obtain compound 2g (white crystalline solid, 95 mg).
d. General procedure for the synthesis of compounds 3a–k. In a microwave tube, a mixture of N-methyl-7-aza indoles 2a–k (100 mg, 0.7566 mmol), aq. HCHO (37%) (3 equiv.) and Mont K-10 (50 w/w) clay were microwave irradiated (100 °C, 150 W, 150psi for 5 min). After completion of the reaction (monitored by TLC), the reaction mixture was diluted with EtOAc and washed with water. The combined organic layer was dried over anhydrous MgSO4 and the solvent was evaporated under reduced pressure. The crude was purified by silica gel column chromatography by using gradient elution with EtOAc–hexane to obtain pure compounds 3a–k.
e. General procedure for the synthesis of compound 4a–e. To a solution of 3,3′-bis-7-azaindolylmethane, 3a–e (100 mg, 0.362 mmol) in MeOH
:
CH3CN (2
:
1) was added cerium(IV) ammonium nitrate (3 equiv.) in a portion-wise at 0 °C to RT for 30 min. After the completion of the reaction (monitored by TLC), the reaction mixture was diluted with EtOAc and washed with distilled water. The combined organic layer was dried over anhydrous MgSO4 and the solvent was evaporated under reduced pressure. The crude mixture was purified on a silica gel column chromatography (eluent
:
hexane/EtOAc
:
1/1) to afford the corresponding compounds 4a–e in good yields.
f. Typical procedure for Suzuki coupling reaction. In a Schlenk tube, a mixture of bis(5-bromo-1-methyl-1H-pyrrolo[2,3-b] pyridin-3-1yl) methanone, 4b (100 mg, 0.0223 mmol), aryl boronic acid (3 equiv.), PdCl2(dppf) (20 mol%) and K2CO3 (3 equiv.) in dioxane
:
H2O mixture (3
:
1, 2 mL) under N2 atm., was heated at 70 °C for 22 h. After completion of the reaction (monitored by TLC), the mixture was allowed to attain room temperature. The mixture was diluted with EtOAc, filtered through a pad of Celite and concentrated under vacuum. The crude mixture was purified by silica gel column chromatography to afford products 6a–e.
Spectroscopic data for synthesized compounds
Bis(1-methyl-1H-pyrrolo[2,3-b]pyridin-3-yl)methane (3a)20. Nature: yellow solid; yield: 144 mg (74%); Rf (40% EtOAc–hexane): 0.21; FTIR (KBr) νmax: 3052, 2940, 2829, 1533, 1478, 1403, 1260, 889, 845, 764, 591 cm−1. 1H NMR (400.3 MHz, CDCl3/TMS): δ 8.24 (dd, J = 4.7, 1.4 Hz, 2H), 7.74 (dd, J = 7.8, 1.5 Hz, 2H), 6.92 (dd, J = 7.8, 4.7 Hz, 2H), 6.85 (s, 2H), 4.10 (s, 2H), 3.75 (s, 6H). 13C NMR (101.6 MHz, CDCl3/TMS): δ 148.2, 142.9, 127.2, 120.3, 115.0, 112.3, 31.1, 21.4. DEPT-135 (101.6 MHz, CDCl3/TMS): δ 142.8, 127.3, 126.9, 114.9, 31.1, 21.3(↓). HRMS (ESI): calcd for C17H16N4 [M + H]+ m/z: 277.1453; found 277.1450.
Bis(5-bromo-1-methyl-1H-pyrrolo[2,3-b]pyridin-3-yl)methane3b. Nature: white solid; yield: 165 mg (80%); Rf (40% EtOAc–hexane): 0.26; FTIR (KBr) νmax: 3052, 2940, 2829, 1596, 1533, 1478, 1403, 1260, 1074, 889, 844, 768, 591 cm−1. 1H NMR (400.3 MHz, CDCl3/TMS): δ 8.27 (d, J = 2.1 Hz, 2H), 7.84 (d, J = 2.1 Hz, 2H), 6.86 (s, 2H), 4.01 (s, 2H), 3.74 (s, 6H). 13C NMR (101.6 MHz, CDCl3/TMS): δ 146.4, 143.4, 129.3, 128.4, 121.5, 111.4, 111.0, 31.2, 21.1. DEPT-135 (101.6 MHz, CDCl3/TMS): δ 146.5, 143.4, 129.3, 128.4, 121.5, 111.4, 111.0, 31.3, 21.1(↓). HRMS (ESI): calcd. for C17H14Br2N4 [M + H]+ m/z: 432.9663; found 432.9636.
Bis(3-bromo-1-methyl-1H-pyrrolo[2,3-b]pyridin-5-yl)methane (3c). Nature: yellow solid; yield: 175 mg (85%); Rf (40% EtOAc–hexane): 0.23; FTIR (KBr) νmax: 2918, 2860, 1471, 1400, 1257, 1140, 1075, 887, 841, 770, 602 cm−1. 1H NMR (400.3 MHz, CDCl3/TMS): δ 8.26 (s, 2H), 7.84 (s, 2H), 6.86 (s, 2H), 4.01 (s, 2H), 3.74 (s, 6H). 13C NMR (101.6 MHz, CDCl3/TMS): δ 146.6, 143.5, 129.4, 128.5, 121.6, 111.5, 111.1, 31.4, 21.3. DEPT-135 (101.6 MHz, CDCl3/TMS): δ 143.4, 129.3, 128.4, 31.3, 21.1(↓). HRMS (ESI): calcd. for C17H14Br2N4 [M + H]+ m/z: 432.9663; found 432.9682.
Bis(5-bromo-1-methyl-1H-indol-3-yl)methane (3d)21. Nature: pale pink powder; yield: 173 mg (84%); Rf (30% EtOAc–hexane): 0.42; 1H NMR (400.3 MHz, CDCl3/TMS): δ 7.71–7.65 (m, 2H), 7.32–7.23 (m, 2H), 7.17–7.10 (m, 2H), 6.76 (s, 2H), 4.08 (s, 2H), 3.68 (s, 6H). 13C NMR (101.6 MHz, CDCl3/TMS): δ 136.0, 129.6, 128.2, 124.5, 121.9, 113.7, 112.3, 110.8, 32.9, 20.8. DEPT-135 (101.6 MHz, CDCl3/TMS): δ 128.1, 124.4, 121.7, 110.7, 32.8, 20.8(↓).
Bis(6-bromo-1-methyl-1H-indol-3-yl)methane (3e)22. Nature: pale brown powder; yield: 169 mg (82%); Rf (30% EtOAc–hexane): 0.40; 1H NMR (400.3 MHz, CDCl3/TMS): δ 7.71–7.65 (m, 2H), 7.32–7.23 (m, 2H), 7.17–7.10 (m, 2H), 6.76 (s, 2H), 4.08 (s, 2H), 3.68 (s, 6H). 13C NMR (101.6 MHz, CDCl3/TMS): δ 136.0, 129.6, 128.2, 124.5, 121.9, 113.7, 112.3, 110.8, 32.9, 20.9. DEPT-135 (101.6 MHz, CDCl3/TMS): δ 128.1, 124.4, 121.7, 110.7, 32.8, 20.8(↓).
Bis(1,2-dimethyl-1H-indol-3-yl)methane (3f)23. Nature: white solid; yield: 167 mg (80%); Rf (8% EtOAc–hexane): 0.81; 1H NMR (400.3 MHz, CDCl3/TMS): δ 7.34 (d, J = 7.9 Hz, 2H), 7.13 (d, J = 8.1 Hz, 2H), 7.01 (t, J = 7.6 Hz, 2H), 6.88 (t, J = 7.4 Hz, 2H), 4.07 (s, 2H), 3.56 (s, 6H), 2.29 (s, 6H). 13C NMR (101.6 MHz, CDCl3/TMS): δ 136.7, 132.8, 128.2, 120.4, 118.6, 110.5, 108.5, 29.6, 20.1, 10.6. DEPT-135 (101.6 MHz, CDCl3/TMS): δ 120.2, 118.5, 108.3, 29.5, 19.9(↓), 10.5.
Bis(9-methyl-9H-carbazol-3-yl)methane (3g)24. Nature: white solid; yield: 164 mg (79%); Rf (8% EtOAc–hexane): 0.84; 1H NMR (400.3 MHz, CDCl3/TMS): δ 7.99–7.95 (m, 2H), 7.92–7.88 (m, 2H), 7.40–7.35 (m, 2H), 7.28 (dt, J = 20.0, 5.1 Hz, 6H), 7.11 (ddd, J = 8.0, 7.1, 1.0 Hz, 2H), 4.30 (s, 2H), 3.76 (s, 6H). 13C NMR (101.6 MHz, CDCl3/TMS): δ 141.4, 139.8, 132.9, 127.2, 125.7, 123.1, 122.9, 120.5, 118.8, 108.5, 42.1, 29.3. DEPT-135 (101.6 MHz, CDCl3/TMS): δ 127.0, 125.5, 120.4, 118.6, 108.4, 41.9(↓), 29.1. HRMS (ESI): calcd for C27H22N2 [M + H]+ m/z: 375.1861; found 375.1843.
Bis(1-benzyl-1H-pyrrolo[2,3-b]pyridin-3-yl)methane (3h). Nature: pale yellow solid; yield: 155 mg (75%); Rf (50% EtOAc–hexane): 0.25; FTIR (KBr)νmax: 3028, 2911, 1594, 1445, 1335, 1277, 111
722, 1024, 764, 705, 602 cm−1. 1H NMR (400.3 MHz, CDCl3/TMS): δ 8.23 (dd, J = 4.7, 1.5 Hz, 2H), 7.68 (dd, J = 7.8, 1.6 Hz, 2H), 7.28–7.12 (m, 6H), 7.07 (dd, J = 7.7, 1.8 Hz, 4H), 6.98–6.78 (m, 4H), 5.36 (s, 4H), 4.07 (s, 2H). 13C NMR (101.6 MHz, CDCl3/TMS): δ 148.1, 143.0, 138.0, 128.6, 127.1, 125.7, 120.1, 115.2, 112.8, 47.5, 21.7. DEPT-135 (101.6 MHz, CDCl3/TMS): δ 148.1, 143.0, 138.0, 128.6, 127.1, 125.7, 120.1, 115.2, 112.8, 47.5, 21.7. HRMS (ESI): calcd for C29H24N4 [M + H]+ m/z: 429.2079; found 429.2072.
Bis(1-benzyl-5-bromo-1H-pyrrolo[2,3-b]pyridin-3-yl)methane (3i). Nature: white solid; yield: 159 mg (78%); Rf (50% EtOAc–hexane): 0.21; FTIR (KBr) νmax: 3028, 2911, 1723, 1594, 1445, 1335, 1277, 111
722, 1024, 764, 705, 602 cm−1. 1H NMR (400.3 MHz, CDCl3/TMS): δ 8.25 (d, J = 2.1 Hz, 2H), 7.77 (d, J = 2.2 Hz, 2H), 7.35–7.12 (m, 6H), 7.13–6.99 (m, 4H), 6.89 (s, 2H), 5.33 (s, 4H), 3.99 (s, 2H). 13C NMR (101.6 MHz, CDCl3/TMS): δ 146.4, 143.6, 137.4, 129.4, 128.8, 127.7, 127.2, 121.4, 111.8, 111.2, 47.8, 21.6. DEPT-135 (101.6 MHz, CDCl3/TMS): δ 143.6, 129.4, 128.8, 127.7, 127.2, 47.8, 21.6. HRMS (ESI): calcd for C29H22Br2N4 [M + H]+ m/z: 584.0289; found 585.0286.
Bis(5-bromo-1-(prop-2-yn-1-yl)-1H-pyrrolo[2,3-b]pyridin-3-yl)methane (3j). Nature: white solid; yield: 164 mg (80%); Rf (50% EtOAc–hexane): 0.22; FTIR (KBr) νmax: 3287, 3105, 2954, 1536, 1467, 1423, 1341,1246, 1187, 1975, 927, 885, 832, 767, 690, 637. 1H NMR (400.3 MHz, CDCl3/TMS): δ 8.37 (d, J = 1.7 Hz, 2H), 7.93 (d, J = 1.9 Hz, 2H), 7.25 (d, J = 27.2 Hz, 2H), 5.05 (s, 4H), 4.14 (s, 2H), 2.41 (s, 2H). 13C NMR (101.6 MHz, CDCl3/TMS): δ 145.9, 143.8, 129.8, 126.5, 121.9, 112.4, 111.7, 73.5, 33.8, 21.6. DEPT-135 (101.6 MHz, CDCl3/TMS): δ 143.7, 129.6, 126.4, 33.6, 21.5. HRMS (ESI): calcd for C21H14Br2N4 [M + H]+ m/z: 479.9585; found 480.9702.
Bis(1-allyl-5-bromo-1H-pyrrolo[2,3-b]pyridin-3-yl)methane (3k). Nature: white solid; yield: 154 mg (75%) Rf (50% EtOAc–hexane): 0.24; 1H NMR (400.3 MHz, CDCl3/TMS): δ 8.25 (s, 2H), 7.81 (s, 2H), 6.92 (s, 2H), 5.93 (ddd, J = 22.2, 10.5, 5.4 Hz, 2H), 5.12 (d, J = 10.2 Hz, 2H), 4.98 (d, J = 17.1 Hz, 2H), 4.76 (d, J = 5.1 Hz, 4H), 4.02 (s, 2H). 13C NMR (101.6 MHz, CDCl3/TMS): δ 146.2, 143.6, 133.6, 129.5, 127.2, 121.6, 117.5, 111.5, 46.6, 29.8, 21.6. DEPT-135 (101.6 MHz, CDCl3/TMS): δ 142.5, 132.4, 128.3, 126.0, 115.4, 20.4. HRMS (ESI): calcd for C21H18Br2N4 [M + H]+ m/z: 483.9898; found 484.9649.
Bis(1-methyl-1H-pyrrolo[2,3-b]pyridin-3-yl)methanone (4a). Nature: white solid; yield: 73 mg (70%); Rf (50% EtOAc–hexane): 0.12; FTIR (KBr) νmax: 3469, 3084, 2920, 1727, 1597, 1519, 1440, 1297, 1232, 1121, 1082, 847, 795, 736, 580 cm−1. 1H NMR (400.3 MHz, CDCl3/TMS): δ 8.58 (dd, J = 7.9, 1.4 Hz, 2H), 8.38 (dd, J = 4.7, 1.4 Hz, 2H), 7.77 (s, 2H), 7.21 (dd, J = 8.6, 5.5 Hz, 2H), 3.95 (s, 6H). 13C NMR (101.6 MHz, CDCl3/TMS): δ 183.9, 148.2, 144.5, 134.2, 131.3, 119.9, 118.3, 115.6, 32.2. DEPT-135 (101.6 MHz, CDCl3/TMS): δ 144.3, 134.1, 131.2, 118.2, 32.1. HRMS (ESI): calcd for C17H14N4O [M + H]+ m/z: 291.1246; found 291.1277.
Bis(5-bromo-1-methyl-1H-pyrrolo[2,3-b]pyridin-3-yl)methanone (4b). Nature: brown powder; yield: 77 mg (75%); Rf (50% EtOAc–hexane): 0.19; FTIR (KBr) νmax: 2919, 2849, 1718, 1584, 1520, 1455, 1213, 1098, 817, 727, 586 cm−1. 1H NMR (400.3 MHz, CDCl3/TMS): δ 8.70 (d, J = 2.2 Hz, 2H), 8.39 (d, J = 2.2 Hz, 2H), 7.74 (s, 2H), 3.90 (s, 6H). 13C NMR (101.6 MHz, CDCl3/TMS): δ 182.9, 146.7, 145.5, 134.6, 132.9, 120.9, 114.6, 32.1. DEPT-135 (101.6 MHz, CDCl3/TMS): δ 145.5, 134.6, 132.9, 32.1. HRMS (ESI): calcd for C17H12Br2N4O [M + H]+ m/z: 446.9456; found 446.9450.
Bis(3-bromo-1-methyl-1H-pyrrolo[2,3-b]pyridin-5-yl)methanone (4c). Nature: brown powder; yield: 80 mg (78%); Rf (50% EtOAc–hexane): 0.19; FTIR (KBr) νmax: 3093, 2915, 2114, 1588, 1523, 1458, 1283, 1095, 887, 731
595 cm−1. 1H NMR (400.3 MHz, CDCl3/TMS): δ 8.68 (s, 2H), 8.37 (s, 2H), 7.73 (s, 2H), 3.90 (s, 6H). 13C NMR (101.6 MHz, CDCl3/TMS): δ 183.0, 146.7, 145.5, 134.8, 133.1, 121.0, 114.7, 32.3. DEPT-135 (101.6 MHz, CDCl3/TMS): δ 145.5, 134.8, 133.1, 32.3. HRMS (ESI): calcd for C17H12Br2N4O [M + H]+ m/z: 446.9456; found 446.9456.
Bis(5-bromo-1-methyl-1H-indol-3-yl)methanone (4d). Nature: brown powder; yield: 37 mg (36%); Rf (30% EtOAc–hexane): 0.10; FTIR (KBr) νmax: 3509, 3431,3106, 2094, 1608, 1517, 1458, 1361, 1211, 1128, 1062, 836, 802, 595, 478 cm−1. 1H NMR (400.3 MHz, CDCl3/TMS): δ 8.14 (d, J = 8.5 Hz, 2H), 7.47 (s, 2H), 7.42 (d, J = 1.5 Hz, 2H), 7.32 (dd, J = 8.5, 1.7 Hz, 2H), 3.74 (s, 6H). 13C NMR (101.6 MHz, CDCl3/TMS): δ 184.1, 138.3, 134.7, 126.1, 125.3, 123.8, 117.2, 112.8, 33.6, 29.8. DEPT-135 (101.6 MHz, CDCl3/TMS): δ 134.6, 125.1, 123.6, 112.6, 33.4, 26.1. HRMS (ESI): calcd for C19H14Br2N2O [M + H]+ m/z: 443.9473; found 444.9549.
Bis(6-bromo-1-methyl-1H-indol-3-yl)methanone (4e). Nature: brown powder; yield: 46 mg (45%); Rf (75% EtOAc–hexane): 0.15; FTIR (KBr) νmax: 2918, 2853, 1724, 1588, 1523, 1452, 1367, 1211, 1095, 816, 731, 666, 595 cm−1. 1H NMR (400.3 MHz, CDCl3/TMS): δ 8.14 (d, J = 8.5 Hz, 2H), 7.46 (s, 2H), 7.41 (d, J = 1.4 Hz, 2H), 7.32 (dd, J = 8.5, 1.6 Hz, 2H), 3.73 (s, 6H). 13C NMR (101.6 MHz, CDCl3/TMS): δ 184.1, 138.3, 134.7, 126.1, 125.3 123.8, 117.5, 117.0, 112.8, 33.6. DEPT-135 (101.6 MHz, CDCl3/TMS): δ 134.6, 125.2, 123.6, 112.6, 77.2, 33.5. HRMS (ESI): calcd for C19H14Br2N2O [M + H]+ m/z: 443.9473; found 444.9549.
Bis(1-methyl-5-phenyl-1H-pyrrolo[2,3-b]pyridin-3-yl)methanone (6a). Nature: brown powder; yield: 71 mg (72%); Rf (60% EtOAc–hexane): 0.20; FTIR (KBr) νmax: 2928, 2857, 1996, 1724, 1594, 1530, 1452, 1368, 1258, 1129, 1083, 892, 770, 697 cm−1. 1H NMR (400.3 MHz, CDCl3/TMS): δ 8.82 (d, J = 2.1 Hz, 2H), 8.63 (d, J = 2.1 Hz, 2H), 7.83 (s, 2H), 7.68–7.55 (m, 4H), 7.42 (t, J = 7.6 Hz, 4H), 7.32 (t, J = 7.4 Hz, 2H), 3.99 (s, 6H). 13C NMR (101.6 MHz, DMSO) δ 138.6, 136.9, 129.5, 127.2, 119.4, 51.6, 31.7. DEPT-135 (101.6 MHz, CDCl3) δ 144.0, 134.5, 129.2, 128.9, 127.5, 127.3, 32.0. HRMS (ESI): calcd for C29H22N4O [M + H]+ m/z: 443.1872; found 443.1837.
Bis(1-methyl-5-(p-tolyl)-1H-pyrrolo[2,3-b]pyridin-3-yl)methanone (6b). Nature: yellow solid; yield: 52 mg (50%); Rf (75% EtOAc–hexane): 0.16; FTIR (KBr) νmax: 3106, 3015, 2911, 2107, 1601, 1517, 1445, 1355, 1211, 1089, 887, 822, 738, 576, 524 cm−1. 1H NMR (400.3 MHz, CDCl3/TMS): δ 8.79 (s, 2H), 8.61 (s, 2H), 7.80 (s, 2H), 7.52 (d, J = 7.6 Hz, 4H), 7.35–7.04 (m, 4H), 3.97 (s, 6H), 2.35 (s, 6H). 13C NMR (101.6 MHz, DMSO) δ 182.6, 147.4, 146.4, 144.3, 143.1, 137.5, 137.0, 136.9, 135.6, 131.9, 130.8, 129.9, 127.6, 127.0, 120.9, 119.3, 113.5, 113.3, 112.9, 31.8, 20.8. DEPT-135 (101.6 MHz, CDCl3) δ 143.9, 134.4, 129.6, 128.9, 127.4, 32.0, 21.1. HRMS (ESI): calcd for C31H26N4O [M + H]+ m/z: 471.2185; found 471.2191.
Bis(5-([1,1′-biphenyl]-2-yl)-1-methyl-1H-pyrrolo[2,3-b]pyridin-3-yl)methanone (6c). Nature: white powder; yield: 79 mg (60%); Rf (60% EtOAc–hexane): 0.40; FTIR (KBr) νmax: 3052, 2916, 1603, 1526, 1462, 1090, 897, 763, 737, 705, 577 cm−1.1H NMR (400.3 MHz, CDCl3/TMS): δ 8.65 (d, J = 2.1 Hz, 2H), 8.06 (d, J = 2.1 Hz, 2H), 7.82 (s, 2H), 7.55–7.44 (m, 8H), 7.25–7.16 (m, 10H), 3.96 (s, 6H). 13C NMR (101.6 MHz, CDCl3/TMS): δ 183.9, 146.5, 141.4, 141.1, 137.9, 134.3, 132.4, 131.6, 131.5, 130.8, 130.2, 128.3, 127.9, 127.8, 126.8, 119.4, 115.7, 32.2. DEPT-135 (101.6 MHz, CDCl3/TMS): δ 146.4, 134.2, 131.5, 131.3, 130.7, 130.0, 128.1, 127.8, 127.7, 126.6, 32.0. HRMS (ESI): calcd. for C41H30N4O [M + H]+ m/z: 595.2498; found 595.2487.
Bis(1-methyl-5-(phenanthren-9-yl)-1H-pyrrolo[2,3-b]pyridin-3-yl)methanone (6d). Nature: white powder; yield: 132 mg (92%); Rf (75% EtOAc–hexane): 0.42; FTIR (KBr) νmax: 3035, 2918, 2100, 1983, 1594, 1523, 1445, 1400, 1355, 1218, 1128, 1075, 978, 887, 738, 595 cm−1. 1H NMR (400.3 MHz, CDCl3/TMS): δ 8.75 (d, J = 2.1 Hz, 2H), 8.70 (d, J = 8.2 Hz, 2H), 8.64 (d, J = 8.0 Hz, 2H), 8.54 (d, J = 2.1 Hz, 2H), 7.90 (s, 2H), 7.82 (td, J = 8.6, 1.2 Hz, 4H), 7.68 (s, 2H), 7.62–7.50 (m, 6H), 7.44 (ddd, J = 8.2, 7.0, 1.2 Hz, 2H), 4.01 (s, 6H). 13C NMR (101.6 MHz, CDCl3/TMS): δ 183.9, 147.9, 146.2, 135.9, 134.7, 132.1, 131.4, 130.8, 130.2, 128.9, 126.7, 123.1, 122.7, 119.5, 115.8, 32.2. DEPT-135 (101.6 MHz, CDCl3/TMS): δ 146.1, 134.6, 131.9, 128.8, 126.5, 123.0, 122.6, 32.1. HRMS (ESI): calcd for C45H30N4O [M + H]+ m/z: 643.2498; found 643.2506.
Bis(1-methyl-5-(pyren-2-yl)-1H-pyrrolo[2,3-b]pyridin-3-yl)methanone (6e). Nature: brown powder; yield: 137 mg (89%); Rf (75% EtOAc–hexane): 0.42; FTIR (KBr) νmax: 2916, 2846, 1718, 1603, 1526, 1448, 1090, 833, 731 cm−1. 1H NMR (400.3 MHz, CDCl3/TMS) δ 8.84 (d, J = 2.1 Hz, 2H), 8.63 (d, J = 2.1 Hz, 2H), 8.18–8.00 (m, 12H), 7.94 (dt, J = 9.6, 7.7 Hz, 8H), 4.04 (s, 6H). 13C NMR (101.6 MHz, CDCl3/TMS) δ 183.9, 147.8, 146.6, 134.7, 132.4, 131.8, 131.6, 131.0, 129.1, 128.4, 127.9, 127.7, 127.5, 126.2, 125.3, 125.0, 124.8, 119.5, 115.8, 32.2. Dept-135 (101.6 MHz, CDCl3/TMS) δ 146.5, 134.6, 132.3, 128.3, 127.5, 126.0, 125.2, 124.7, 32.1. HRMS (ESI): calcd for C49H30N4O [M + H]+ m/z: 691.2498; found 691.2485.
Conflicts of interest
There are no conflicts to declare.
Acknowledgements
PE thanks VIT, Vellore for the research fellowship. SK thanks VIT Management for providing infrastructure facilities and VIT SEED GRANT for carrying out this work.
Notes and references
- D. R. Motati, R. Amaradhi and T. Ganesh, Org. Chem. Front., 2021, 8, 466–513 RSC.
- S. B. Zhao and S. Wang, Chem. Soc. Rev., 2010, 39, 3142–3156 RSC.
-
(a) Y. Chen, Chem.–Eur. J., 2019, 25, 3405–3439 CrossRef CAS PubMed;
(b) C. Sun, X. Zou and F. Li, Chem.–Eur. J., 2013, 19, 14030–14033 CrossRef CAS PubMed;
(c) C. Qiao, X. F. Liu, H. C. Fu, H. P. Yang, Z. B. Zhang and L. N. He, Chem.–Asian J., 2018, 13, 2664–2670 CrossRef CAS PubMed;
(d) P. T. Ha, O. T. Nguyen, K. D. Huynh, T. T. Nguyen and N. T. Phan, Synlett, 2018, 19, 2031–2034 Search PubMed;
(e) M. Elagawany, L. Maram and B. Elgendy, RSC Adv., 2021, 11, 7564–7569 RSC.
-
(a) S. H. Lee, K. Kim, Y. U. Jeon, A. Kundu, P. Dey, J. Y. Hwang, N. K. Mishra, H. S. Kim and I. S. Kim, Tetrahedron Lett., 2019, 60, 150974 CrossRef;
(b) A. Z. Halimehjani and V. Barati, ChemistrySelect, 2018, 3, 3024–3028 CrossRef CAS.
-
(a) X. Han, Z. Zhou, C. Wan, Y. Xiao and Z. Qin, Synthesis, 2013, 45, 615 CrossRef CAS;
(b) Y. Hu, L. Zhou and W. Lu, Synthesis, 2017, 49, 4007 CrossRef CAS;
(c) M. R. Maurya, B. Sarkar, A. Kumar, N. Ribeiro, A. Miliute and J. C. Pessoa, New J. Chem., 2019, 43, 17620 RSC;
(d) K. N. Parida, S. Jhulki, S. Mandal and J. N. Moorthy, Tetrahedron, 2012, 68, 9763 CrossRef CAS;
(e) S. V. Lieberman and Connor, R., Org. Synth., 1938, 18, 61 CrossRef CAS;
(f) T. Nishimura, Org. Synth., 1956, 36, 58 CrossRef CAS;
(g) W. H. Hartford and M. Darrin, Chem. Rev., 1958, 58, 1–61 CrossRef CAS;
(h) I. Vlattas, I. T. Harrison, L. Tokes, J. H. Fried and A. D. Cross, J. Org. Chem., 1968, 33, 4176–4179 CrossRef CAS;
(i) C. S. Marvel, J. S. Saunders and C. G. Overberger, J. Am. Chem. Soc., 1946, 68, 1085–1088 CrossRef CAS PubMed;
(j) M. S. Carpenter, W. M. Easter and T. F. Wood, J. Org. Chem., 1951, 16, 586–617 CrossRef CAS;
(k) L. Syper, Tetrahedron Lett., 1966, 7, 4493–4498 CrossRef;
(l) W. S. Trahanovsky and L. B. Young, J. Org. Chem., 1966, 31, 2033–2035 CrossRef CAS;
(m) H. Gilman, C. G. Brannen and R. K. Ingham, J. Am. Chem. Soc., 1956, 78, 1689–1692 CrossRef CAS;
(n) E. Ganin and I. Amer, Synth. Commun., 1995, 25, 3149–3154 CrossRef CAS;
(o) R. Hosseinzadeh, M. Tajbakhsh and H. Vahedi, Synlett, 2005, 18, 2769–2770 CrossRef;
(p) M. Ghaffarzadeh, M. Bolourtchian, M. Gholamhosseni and F. Mohsenzadeh, Appl. Catal., A, 2007, 333, 131–135 CrossRef CAS.
-
(a) M. Tobiszewski, M. Marć, A. Gałuszka and J. Namieśnik, Molecules, 2015, 20, 10928–10946 CrossRef CAS PubMed;
(b) I. T. Horvath and P. T. Anastas, Chem. Rev., 2007, 107, 2169–2173 CrossRef CAS PubMed;
(c) H. E. D. M. Saleh, and M. Koller, in Green Chemistry, IntechOpen, 2018 Search PubMed;
(d) Z. Zhang, J. Wen, M. Wang, C. G. Yan and Z. Shi, Green Synthesis and Catalysis, 2021, 2, 275–285 CrossRef;
(e) Y. Kim and C. J. Li, Green Synthesis and Catalysis, 2020, 1, 1–11 CrossRef;
(f) P. Anastas and N. Eghbali, Chem. Soc. Rev., 2010, 39, 301–312 RSC.
-
(a) V. Sridharan and J. C. Menéndez, Chem. Rev., 2010, 110, 3805–3849 CrossRef CAS PubMed;
(b) V. Nair and A. Deepthi, Chem. Rev., 2007, 107, 1861–1891 CrossRef;
(c) V. Nair, L. Balagopal, R. Rajan and J. Mathew, Acc. Chem. Res., 2004, 37, 21–30 CrossRef CAS PubMed and references cited therein.
(d) P. Shanmugam, V. Vaithiyanathan and V. Baby, Tetrahedron Lett., 2006, 47, 6851–6855 CrossRef CAS;
(e) P. Shanmugam and V. Vaithiyanathan, Can. J. Chem., 2009, 87, 591 CrossRef CAS.
-
(a) S. Darses, Tetrahedron Lett., 1996, 37, 3857–3860 CrossRef CAS;
(b) L. Chen, D. R. Sanchez, B. Zhang and B. P. Carrow, J. Am. Chem. Soc., 2017, 139, 12418–12421 CrossRef CAS;
(c) L. Chen, H. Francis and B. P. Carrow, ACS Catal., 2018, 8, 2989–2994 CrossRef CAS;
(d) I. A. Sanhueza, Angew. Chem., Int. Ed., 2021, 60, 7007–7012 CrossRef CAS.
- T. Pillaiyar, M. Köse, K. Sylvester, H. Weighardt, D. Thimm, G. Borges, I. Förster, I. von Kügelgen and C. E. Müller, J. Med. Chem., 2017, 60, 3636–3655 CrossRef CAS PubMed.
- CCDC-2191474 (3b) contains the supplementary crystallographic data for this paper†..
- F. Pu, Y. Li, Y. H. Song, J. Xiao, Z. W. Liu, C. Wang, Z. T. Liu, J. G. Chen and J. Lu, Adv. Synth. Catal., 2016, 358, 539–542 CrossRef CAS.
-
(a) Y. Ma, J. You and F. Song, Chem.–Eur. J., 2013, 19, 1189–1193 CrossRef CAS PubMed;
(b) R. Shen, T. Kusakabe, K. Takahashi and K. Kato, Org. Biomol. Chem., 2014, 12, 4602–4609 RSC;
(c) X. Zheng, Z. Huang, Q. Zheng, L. Wang, C. Zhang and G. Gao, Org. Lett., 2022, 24, 4197–4201 CrossRef CAS;
(d) S. Kotha, R. Ali, V. Srinivas and N. G. Krishna, Tetrahedron, 2015, 71, 129–138 CrossRef CAS;
(e) T. Pillaiyar, M. Köse, K. Sylvester, H. Weighardt, D. Thimm, G. Borges, I. Förster, I. von Kügelgen and C. E. Müller, J. Med. Chem., 2017, 60, 3636–3655 CrossRef CAS PubMed;
(f) S. K. Guchhait, M. Kashyap and H. Kamble, J. Org. Chem., 2011, 76, 4753–4758 CrossRef CAS PubMed;
(g) P. Neupane, A. A. Salim and R. J. Capon, Tetrahedron Lett., 2020, 61, 151651 CrossRef CAS;
(h) T. Das, A. Chakraborty and A. Sarkar, Tetrahedron Lett., 2014, 55, 7198–7202 CrossRef CAS.
-
(a) A. T. Khan, T. Khan, L. H. Choudhury and S. Ghosh, Tetrahedron Lett., 2007, 48, 2271–2274 CrossRef CAS;
(b) D. Shi, Y. Ren, H. Jiang, J. Lu and X. Cheng, Dalton Trans., 2013, 42, 484–491 RSC;
(c) J. Młochowski and H. Wójtowicz-Młochowska, Molecules, 2015, 20, 10205–10243 CrossRef PubMed;
(d) R. Chebolu, A. Bahuguna, R. Sharma, V. K. Mishra and P. C. Ravikumar, Chem. Commun., 2015, 51, 15438–15441 RSC;
(e) P. Shanmugam, V. Vaithiyanathan and K. Selvakumar, Tetrahedron Lett., 2008, 49, 2119–2123 CrossRef CAS.
- CCDC-2102023 (4a) contains the supplementary crystallographic data for this paper†..
-
(a) J. A. Lopez and M. F. Greaney, Chem. Soc. Rev., 2016, 45, 6766–6798 RSC;
(b) C. Ni, D. Zha, H. Ye, Y. Hai, Y. Zhou, E. V. Anslyn and L. You, Angew. Chem., Int. Ed., 2018, 57, 1300–1305 (Angew. Chem., 2018, 130, 1314–1319) CrossRef CAS PubMed;
(c) Z. J. Jain, P. S. Gaide and R. S. Kankte, Arabian J. Chem., 2017, 10, S2051–S2066 CrossRef CAS;
(d) J. Wang, G. Cooper, D. Tulumello and A. P. Hitchcock, J. Phys. Chem. A, 2005, 109, 10886–10896 CrossRef CAS PubMed;
(e) J. Hassan, M. Sévignon, C. Gozzi, E. Schulz and M. Lemaire, Chem. Rev., 2002, 102, 1359–1470 CrossRef CAS PubMed;
(f) D. A. Horton, G. T. Bourne and M. L. Smythe, Chem. Rev., 2003, 103, 893–930 CrossRef CAS PubMed;
(g) Y. Tuanli, A. C. Marino and C. L. Richard, J. Org. Chem., 2005, 70, 3511–3517 CrossRef PubMed;
(h) H. He, C. Wang, T. Wang, N. Zhou, Z. Wen, S. Wang and L. He, Dyes Pigm., 2015, 113, 174–180 CrossRef CAS;
(i) J. Zhang, J. Su, Y. Ma and H. H. Guo, J. Phys. Chem. B, 2012, 116, 2075–2089 CrossRef CAS PubMed.
-
(a) G. Bringmann, A. J. Price Mortimer, P. A. Keller, M. J. Gresser, J. Garner and M. Breuning, Angew. Chem., Int. Ed., 2005, 44, 5384–5427 (Angew. Chem., 2005, 117, 5518–5563) CrossRef CAS PubMed;
(b) E. Kumarasamy, R. Raghunathan, M. P. Sibi and J. Sivaguru, Chem. Rev., 2015, 115, 11239–11300 CrossRef CAS PubMed;
(c) J. Wencel-Delord, A. Panossian, F. R. Leroux and F. Colobert, Chem. Soc. Rev., 2015, 44, 3418–3430 RSC;
(d) M. Liu, L. Zhang and T. Wang, Chem. Rev., 2015, 115, 7304–7397 CrossRef CAS PubMed;
(e) T. R. Cook and P. J. Stang, Chem. Rev., 2015, 115, 7001–7045 CrossRef CAS PubMed;
(f) E. Yashima, N. Ousaka, D. Taura, K. Shimomura, T. Ikai and K. Maeda, Chem. Rev., 2016, 116, 13752–13990 CrossRef CAS PubMed;
(g) S. Yuan, Y. Chen, J. Qin, W. Lu, L. Zou, Q. Zhang, X. Wang, X. Sun and H. Zhou, J. Am. Chem. Soc., 2016, 138, 8912–8919 CrossRef CAS PubMed;
(h) T. Qin, S. L. Skraba-Joiner, Z. G. Khalil, R. P. Johnson, R. J. Capon Jr and J. A. Porco, Nat. Chem., 2015, 7, 234–240 CrossRef CAS PubMed;
(i) V. Bhat, E. R. Welin, X. Guo and B. M. Stoltz, Chem. Rev., 2017, 117, 4528–4561 CrossRef CAS PubMed;
(j) G. Bringmann, T. Gulder, T. A. M. Gulder and M. Breuning, Chem. Rev., 2011, 111, 563–639 CrossRef CAS PubMed.
- P. Kannaboina, K. A. Kumar and P. Das, Org. Lett., 2016, 18, 900–903 CrossRef CAS PubMed.
- P. Dudhe, M. A. Krishnan, K. Yadav, D. Roy, K. Venkatasubbaiah, B. Pathak and V. Chelvam, Beilstein J. Org. Chem., 2021, 17, 1453–1463 CrossRef CAS PubMed.
- G. Shi, T. Yoon, S. Cha, S. Kim, M. Yousuf, N. Ahmed, D. Kim, H. W. Kang and K. S. Kim, ACS Sens., 2018, 3, 1102–1108 CrossRef CAS PubMed.
- S. H. Lee, K. Kim, Y. U. Jeon, A. Kundu, P. Dey, J. Y. Hwang, N. K. Mishra, H. S. Kim and I. S. Kim, Tetrahedron Lett., 2019, 60, 150974 CrossRef.
- K. Takaishi, H. Kosugi, R. Nishimura, Y. Yamada and T. Ema, Chem. Commun., 2021, 57, 8083–8086 RSC.
- C. Qiao, X. F. Liu, H. C. Fu, H. P. Yang, Z. B. Zhang and L. N. He, Chem.–Asian J., 2018, 13, 2664–2670 CrossRef CAS PubMed.
- P. K. Pradhan, S. Dey, V. S. Giri and P. Jaisankar, Synthesis, 2005, 2005, 1779–1782 CrossRef.
- Y. C. Zheng, M. L. Zheng, S. Chen, Z. S. Zhao and X. M. Duan, J. Mater. Chem. B, 2014, 2, 2301–2310 RSC.
Footnote |
† Electronic supplementary information (ESI) available. Copies of 1H NMR, 13C NMR, DEPT-135, HRMS spectra for all the new compounds, and single-crystal XRD data for compounds 3b and 4a are provided. CCDC 2191474 and 2102023. For ESI and crystallographic data in CIF or other electronic format see DOI: https://doi.org/10.1039/d2ra05849a |
|
This journal is © The Royal Society of Chemistry 2022 |
Click here to see how this site uses Cookies. View our privacy policy here.