DOI:
10.1039/D2RA06231C
(Paper)
RSC Adv., 2022,
12, 35215-35220
DTBP-mediated cross-dehydrogenative coupling of 3-aryl benzofuran-2(3H)-ones with toluenes/phenols for all-carbon quaternary centers†
Received
4th October 2022
, Accepted 2nd December 2022
First published on 9th December 2022
Abstract
We have developed a transition-metal free protocol for efficient cross-dehydrogenative coupling of 3-aryl benzofuran-2(3H)-ones and toluenes/phenols using DTBP as an oxidant. A diverse range of 3-aryl benzofuran-2(3H)-ones, toluenes, and phenols undergo C–H bond cleavage to generate all-carbon quaternary centers in good yields, making this protocol useful for the synthesis of complex molecules. A gram scale experiment was performed in good yield.
Introduction
Cross-dehydrogenative coupling (CDC) is a straightforward strategy for carbon–carbon bond formation.1 Many pioneering efforts have been made to develop CDC methodologies to access useful motifs by using a variety of transition metal catalysts.2 As for the transition-metal free methods for CDC reactions, there are only a few pioneering works that have been developed,3 including oxidative promotion, photocatalysis and electrochemical catalysis. Although a transition-metal free CDC reaction has been developed, its application in all-carbon quaternary center construction has never been disclosed.4
All-carbon quaternary centers are broadly present in various bioactive natural products, artificially synthesized pharmaceutical and medicinal molecules,5 such as hopeahainol A,5b oxyphenisatin, TOP216 analogue,5c yuccaol,5d estrogen receptor modulator,5e cyclophilin inhibitor,5f hamigeran B (Fig. 1).5g These molecules show unique bioactivities due to the rigid three-dimensional structure. Therefore, the efficient and selective construction of all-carbon quaternary stereocenters has received intensive attention in recent decades.6 However, the construction of quaternary carbon centers via CDC reaction is rare.7 Previously, we have developed a Cu-catalyzed CDC reaction of 3-aryl benzofuranones with heteroatom arenes, which furnishes highly functionalized 3,3-diaryl benzofuranones bearing a three aryls quaternary carbon center at C3 position in good yields.7l These complex compounds can be decarbonylation to be triaryl methane easily.8 However, this protocol can be only applied to the coupling between C(sp3)–H bond and C(sp2)–H bond.
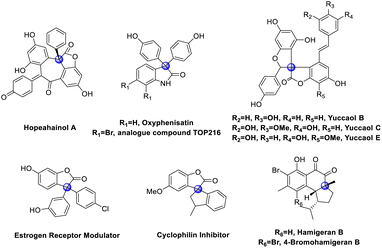 |
| Fig. 1 Bioactive all-carbon quaternary centers. | |
Herein, we disclosed the first example of the construction of all-carbon quaternary centers via a transition-metal free CDC reaction of 3-aryl benzofuran-2(3H)-ones with toluenes/phenols by using DTBP (di-t-butyl peroxide) as an oxidant, which the two different C(sp3)–H bonds can be cross-coupling site-selectively in good yields. This protocol shows a broad substrate scope and gram-scalable ability. While toluenes were changed into phenols with methyl group in this protocol, the ortho-C(sp2)–H bond of phenol was activated site selectively.
Results and discussion
Initially, we explored the reactions using 4-methylbenzofuranone (1a) and toluene (2a) as substrates under different reaction conditions (Table 1). CuI, NiCl2, and CoCl2 were then examined in the presence of DTBP as an oxidant under N2 atmosphere, the yields of 3a were 83%, 88%, 88%, respectively (entries 1–3) and changed the amount of catalyst resulting in a decrease of yield (entries 4–5). However, without a metal catalyst, the yield of 3a was also up to 88% (entry 6), indicating only DTBP is enough for this protocol. While the reaction atmosphere was changed into the air, the yield of 3a was decreased to 77% (entry 7). When DTBP was replaced by THBP, CHP and mCPBA resulted in the decrease of the yield of 3a to 10%, 27% and no product (entry 8–10). When mixed solvent was added, the target product could not be obtained (entry 11–12). Both increasing and decreasing the amount of oxidant would have a negative impact on the reaction (entry 13–14). The adoption of other temperatures also resulted in lower yields (entries 15–16). The molecular structure of 3a were being isolated as racemic mixtures and has been unambiguously confirmed by single crystal X-ray diffraction study (figure in Scheme 1, CCDC 1949691†).
Table 1 Survey on condition for 2a formationa
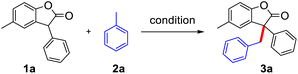
|
Entry |
Cat. (mol%) |
Oxidant |
Solvent |
Temp. (°C) |
Yielda (%) |
Reaction conditions: 1a (0.2 mmol), cat. (mol%), oxidant (0.1 mmol) and 2a (1.0 mL), 12 h, under N2, sealed tube, isolated yield. Under air. DTBP 0.2 equiv. DTBP 1.0 equiv. TBHP = t-butylhydroperoxide, CHP = cumyl hydroperoxide, mCPBA = meta-cholorperoxybenzoic acid, DMF = N,N-dimethyl-formicaci, DMSO = dimethyl sulfoxide. |
1 |
CuI (10) |
DTBP |
Toluene |
140 |
83 |
2 |
NiCl2 (10) |
DTBP |
Toluene |
140 |
88 |
3 |
CoCl2 (10) |
DTBP |
Toluene |
140 |
88 |
4 |
NiCl2 (5) |
DTBP |
Toluene |
140 |
86 |
5 |
NiCl2 (20) |
DTBP |
Toluene |
140 |
45 |
6 |
— |
DTBP |
Toluene |
140 |
88 |
7b |
— |
DTBP |
Toluene |
140 |
77 |
8 |
— |
TBHP |
Toluene |
140 |
10 |
9 |
— |
CHP |
Toluene |
140 |
27 |
10 |
— |
mCPBA |
Toluene |
140 |
0 |
11 |
— |
DTBP |
Toluene/DMF |
140 |
0 |
12 |
— |
DTBP |
Toluene/DMSO |
140 |
0 |
13c |
— |
DTBP |
Toluene |
140 |
12 |
14d |
— |
DTBP |
Toluene |
140 |
50 |
15 |
— |
DTBP |
Toluene |
160 |
87 |
16 |
— |
DTBP |
Toluene |
120 |
32 |
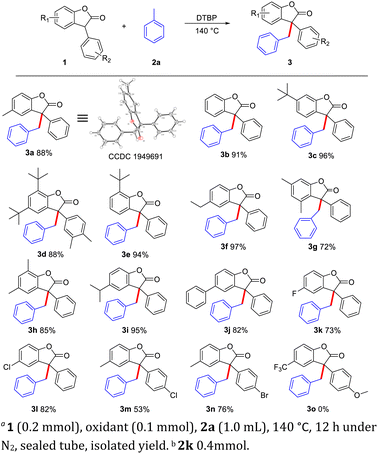 |
| Scheme 1 Substrates scope of benzofuranone.a | |
With the optimized reaction conditions in hand, we next investigated the substrate scope (Scheme 1). A variety of substituted benzofuranones (1) could proceed well in this CDC reaction to deliver corresponding products in moderate to good yields, and valuable groups such as 4-H (3b, 91%), 3-tert-butyl (3c, 96%), 2,4-di-tert-butyl (3d, 88%), 2-tert-butyl (3e, 94%), 4-ethyl (3f, 97%), 3,5-dimethyl (3g, 72%), 2,4-dimethyl (3h, 85%), 4-isopropyl (3i, 95%), 4-phenyl (3j, 82%), 4-fluorine (3k, 73%), 4-chloro (3l, 82%. 3m, 53%), and 4-bromo (3n, 76%) were tolerated. Overall, the adaptability of the reaction system to functional groups is good, only when electron-withdrawing groups are attached to the benzofuranones, a slight inhibitory effect on the reaction was observed. And strong electron withdrawing group would completely inhibit the reaction (3o). Furthermore, compared with the reactivity of HP-136 (3d), three benzofuranones (3c, 3e, 3f) have higher carbon radical trapping ability, which may have some potential to be used as a benzyl radical anti-oxidant.
On the other hand, the scope of toluene analogues was also tested (Scheme 2). A series of aryl-substituted toluenes were subjected to this CDC reaction to react with benzofuranone 1a, and various products were obtained in moderate to good yields. The substituted groups with electron-donating and electron-withdrawing groups at the para or ortho position of the aryl ring could proceed smoothly to afford the desired products (4a–4i). In addition, the radical trapping reagent 2, 6-di-tert-butyl-4-methylphenol (BHT) was also applicable in this CDC reaction to furnish 4h in 61% yield, showing that this reaction may involve a carbon radical center. These results showed that the method is suitable for multi-functionalized steric substrates. Nevertheless, for the large steric biphenyl methane, it could also get the target product 4j in 35% yield. The reaction system could also be applied to heterocyclic systems. 6-Methylquinoline (2k) could also undergo CDC cross-coupling to get the product (4k). But 2-methyindole (2l) could only get the product substituted at the 3-position as a triaryl methane molecule (4l). Other indoles also gave the same 3-substituted indole products (4m, 3n) in 76% and 55%, respectively. And the furan has two products (4o, 4o′) which have been isolated respectively.8 It should be noted that, for the synthesis of 4p and 4q in Pd or Cu systems,7c,l there are no products obtained. While this protocol can afford 4p and 4q in 34 and 55% yield, respectively (Scheme 2b). When we chose n-propyl benzene as substrate which bearing benzylic C–H bond, methylene C–H bond and methyl C–H, we can get the benzylic product 4r in 75% yield, while only trace (<1%) amount of side-products 4r′ and 4r′′ were detected (Scheme 2c). This result shows a good site-selectivity of our method.
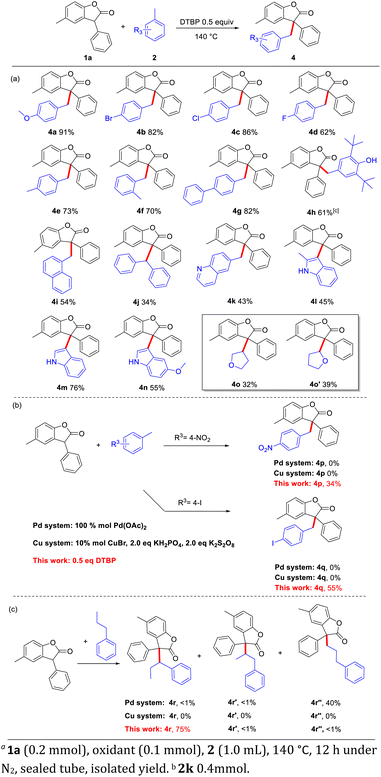 |
| Scheme 2 Substrates scope of toluene.a | |
When the steric o-tBu substituted groups were removed from BHT, the ortho-C–H functionalization of phenols could engage in this process to deliver the triaryl all-carbon quaternary centers (Scheme 3). A series of phenols, such as electron-donating groups and halogen groups, were tolerated in this CDC reaction to react with benzofuranone 1a to give 5a–5g in good yields. Side-products of C–O construction were detected especially in the target product (5′) with low yield (the ratio of 5
:
5′ is shown in the parenthesis). When there were electron donating groups in the para position of phenols, it was beneficial to the generation of radicals, so the yield would be better. When there were other groups in the ortho position of phenols, radicals were not easy to transfer, so the yield of 5 would be reduced. Halogenated phenol, especially iodophenol, were more active, and would get side reactions, leading to low yield. However, when 1a reacted with the thiophenol, only the C–S cross coupling product was obtained (6a, 30%). This result was consistent with the literature,9 which the ortho position of phenol is indeed easy to activate as C–C bond formation rather than C–O bond formation, while that of thiophenol is more difficult to be activated, and the direct construction of C–S bond was observed.
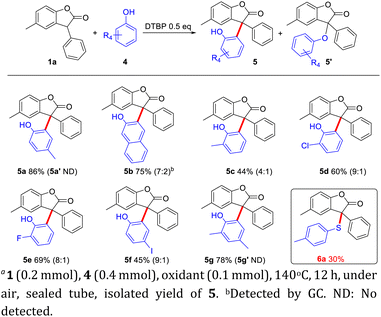 |
| Scheme 3 Substrates scope of phenols.a | |
Gram scale experiments were performed for the synthesis of 3a and 5a, and the yields are up to 65% and 71%, respectively (Scheme 4a). Further amplify the amount of reaction may have the risk of explosion, and a special reactor would be needed. Compound 5a contains lactone and phenol groups, making its transformation to other useful motifs possible. For example, it gave the target decarbonyl product 7 with two phenols in the yield of 85%. And it could be trifluoroacetylated by trifluoroacetic anhydride to 8 in 60% yield. Also compound 8 could undergo dehydroxylation to form product 9 with 83% yield (Scheme 4b).8
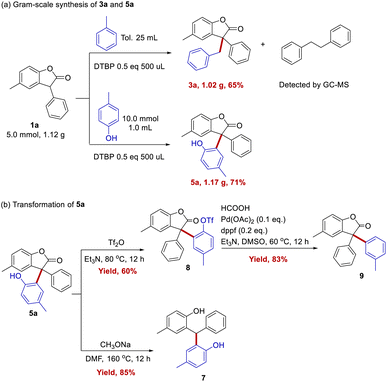 |
| Scheme 4 Gram-scale synthesis and transformation. | |
To probe the possible reaction mechanism, some control experiments were conducted. An intermolecular competition experiment between toluene and the deuterated toluene was carried out to investigate the kinetic isotope effect (KIE), which was found to be significant (kH/kD = 5.6) (Scheme 5a). In the hydrocarbon activation reaction, when kH/kD > 2, the cleavage of the C–H bond may be considered to be the rate-determining step of the reaction.10 The cleavage of the C–H bond may be the rate-determining step in this system. It was observed that the reaction was suppressed by the addition of a radical scavenger 2,2,6,6-tetramethyl-1-piperidinyloxy (Tempo) and 1,4-benzoquinone (BQ), implying the involvement of a radical species (Scheme 5b). And butylated hydroxytoluene (BHT) has trapped the radical of 1a instead of toluene radicals. In addition, the toluene homo-coupling product was detected by GC-MS. Based on the observation, the reaction appears to go through a plausible radicals pathway. When 1a was subjected to the reaction system without toluene, only self-coupling product 10 was obtained under the action of DTBP. And 3a could be obtained in 66% yield from 10 after reacting with toluene (Scheme 5c). At the same time, the reaction of 1a with naphthoquinone (11) did not produce naphthol, which may indicate that the reaction with phenol does not involve intermediates of quinone (Scheme 5d).
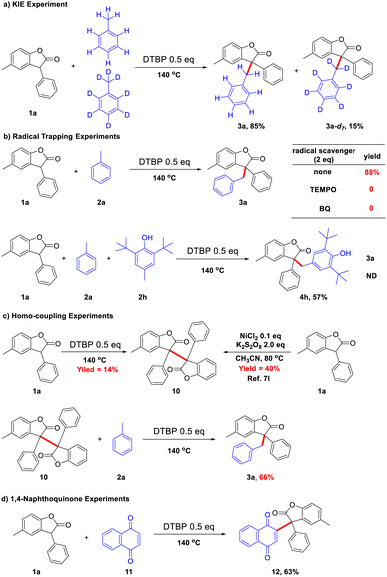 |
| Scheme 5 Control experiments. | |
Based on the above results and those reported in the literature,9,11 a possible pathway for the cross-dehydrogenative coupling reaction was proposed (Scheme 6). 3-Aryl benzofuran-2(3H)-ones and toluene/phenols respectively generated free carbon radicals with DTBP, and then two radicals were cross-coupling to obtain 3a and 4a, respectively. Probably, the 3-aryl benzofuran-2(3H)-ones could also generate relative radical by reacting with benzyl radical species, which can be easily trapped by another benzyl radical to target products.
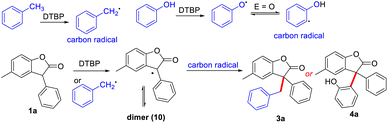 |
| Scheme 6 Plausible mechanism. | |
Conclusions
In summary, we have disclosed the first transition-metal free synthesis of all-carbon quaternary centers via a robust, efficient, and practical cross-dehydrogenative coupling of benzofuranones and toluenes/phenols. A diverse array of functional groups are tolerated in this transformation. A reaction mechanism with radical participation is proposed. This simple method would be useful for the synthesis of complex molecules.
Conflicts of interest
There are no conflicts to declare.
Acknowledgements
The authors thank the Natural Science Foundation of China (21676076, 21878081, 21971060 and 22278120), Hu-Xiang High Talent in Hunan Province (2018RS3042) and Recruitment Program for Foreign Experts of China (WQ20164300353 and G2022160001) for financial support.
Notes and references
- Selected reviews on CDC reaction:
(a) M. K. Lakshman and P. K. Vuram, Chem. Sci., 2017, 8, 5845 RSC;
(b) L. Lv and Z. Li, Top. Curr. Chem., 2016, 374, 38 CrossRef PubMed;
(c) S. A. Girard, T. Knauber and C.-J. Li, Angew. Chem., Int. Ed., 2014, 53, 74 CrossRef CAS;
(d) C. Zhang, C. Tang and N. Jiao, Chem. Soc. Rev., 2012, 41, 3464 RSC;
(e) C. Liu, H. Zhang, W. Shi and A. Lei, Chem. Rev., 2011, 111, 1780 CrossRef CAS PubMed;
(f) C. S. Yeung and V. M. Dong, Chem. Rev., 2011, 111, 1215 CrossRef CAS;
(g) C.-L. Sun, B.-J. Li and Z.-J. Shi, Chem. Rev., 2011, 111, 1293 CrossRef CAS PubMed;
(h) S. H. Cho, J. Y. Kim, J. Kwak and S. Chang, Chem. Soc. Rev., 2011, 40, 5068 RSC;
(i) C.-J. Li, Acc. Chem. Res., 2009, 42, 335 CrossRef CAS PubMed;
(j) C.-J. Li and Z. Li, Pure Appl. Chem., 2006, 78, 935 CrossRef CAS;
(k) X.-X. Guo, D.-W. Gu, Z. Wu and W. Zhang, Chem. Rev., 2015, 115, 1622 CrossRef CAS PubMed;
(l) L. C. M. Castro and N. Chatani, Chem. Lett., 2015, 44, 410 CrossRef CAS;
(m) X. Cen, K. M. Engle, D.-H. Wang and J.-Q. Yu, Angew. Chem., Int. Ed., 2009, 48, 5094 CrossRef PubMed.
- Selected examples of transition metal catalyzed CDC reaction, palladium catalyzed:
(a) J. Zhang, Y. Zhuang, Y. Ma, X. Yang and M. Szostak, Adv. Synth. Catal., 2019, 361, 5709 CrossRef CAS;
(b) C. Dai, Z.-B. Huang, L. Liu, Y. Han, D.-Q. Shi and Y. Zhao, Eur. J. Org. Chem., 2020, 2020, 826 CrossRef CAS;
(c) E. Kianmehr, M. Torabi, M. Rezazadeh Khalkhali, N. Faghih and K. M. Khan, Eur. J. Org. Chem., 2015, 2015, 2796 CrossRef CAS;
(d) Ruthenium catalyzed: S. Dana, D. Chowdhury, A. Mandal, F. A. S. Chipem and M. Baidya, ACS Catal., 2018, 8, 10173 CrossRef CAS;
(e) C. Heitz, A. W. Jones, B. S. Oezkaya, C. L. Bub, M.-L. LouillatHabermeyer, V. Wagner and F. W. Patureau, Chem.–Eur. J., 2016, 22, 17980 CrossRef CAS PubMed;
(f) Q. Huang, S. Fu, S. Ke, H. Xiao, X. Zhang and S. Lin, Eur. J. Org. Chem., 2015, 2015, 6602 CrossRef CAS;
(g) Cobalt catalyzed: B. Lin, S. Shi, R. Lin, Y. Cui, M. Fang, G. Tang and Y. Zhao, J. Org. Chem., 2018, 83, 6754 CrossRef CAS PubMed;
(h) M. Khrizanforov, S. Strekalova, V. Khrizanforova, A. Dobrynin, K. Kholin, T. Gryaznova, V. Grinenko, A. Gubaidullin, M. K. Kadirov and Y. Budnikova, Top. Catal., 2018, 61, 1949 CrossRef CAS;
(i) T. Li, Y. Yang, B. Li and P. Yang, Chem. Commun., 2019, 55, 353 RSC;
(j) Copper catalyzed: G. S. Grandhi, S. Dana, A. Mandal and M. Baidya, Org. Lett., 2020, 22, 2606 CrossRef CAS PubMed;
(k) R. M. Gorman, T. E. Hurst, W. F. Petersen and R. J. K. Taylor, Tetrahedron, 2019, 75, 130711 CrossRef;
(l) M. Kumar, Raziullah, A. A. Khan, A. Ahmad, H. S. Dutta, R. Kant and D. Koley, J. Org. Chem., 2019, 84, 13624 CrossRef CAS PubMed;
(m) Other transition catalyzed: L. K. Jin, L. Wan, J. Feng and C. Cai, Org. Lett., 2015, 17, 4726 CrossRef CAS;
(n) K. Yang and Q. Song, Org. Lett., 2015, 17, 548 CrossRef CAS PubMed;
(o) S. S. Kotha, S. Chandrasekar, S. Sahu and G. Sekar, Eur. J. Org. Chem., 2014, 2014, 7451 CrossRef CAS.
- Selected examples of metal-free CDC reaction, oxidative promoted:
(a) H. Jiang, X. Tang, S. Liu, L. Wang, H. Shen, J. Yang, H. Wang and Q.-W. Gui, Org. Biomol. Chem., 2019, 17, 10223 RSC;
(b) Q. Chen, G. Yu, X. Wang, Y. Ou and Y. Huo, Green Chem., 2019, 21, 798 RSC;
(c) F. Jafarpour and M. Darvishmolla, Org. Biomol. Chem., 2018, 16, 3396 RSC;
(d) Q. Yang, X. Han, J. Zhao, H.-Y. Zhang and Y. Zhang, J. Org. Chem., 2019, 84, 11417 CrossRef CAS PubMed;
(e) Photocatalysis: G. Kibriya, A. K. Bagdi and A. Hajra, J. Org. Chem., 2018, 83, 10619 CrossRef CAS PubMed;
(f) W. Wei, L. Wang, H. Yue, P. Bao, W. Liu, C. Hu, D. Yang and H. Wang, ACS Sustainable Chem. Eng., 2018, 6, 17252 CrossRef CAS;
(g) Electrochemical catalysis: M.-J. Luo, Y. Li, X.-H. Ouyang, J.-H. Li and D.-L. He, Chem. Commun., 2020, 56, 2707 RSC;
(h) Y.-Z. Yang, R.-J. Song and J.-H. Li, Org. Lett., 2019, 21, 3228 CrossRef CAS PubMed;
(i) Z. J. Wu and H.-C. Xu, Angew. Chem., Int. Ed., 2017, 56, 4734 CrossRef CAS PubMed.
- D. J. C. Constable, P. J. D. Lorenz, J. D. Hayler, G. R. Humphrey, J. L. Leazer Jr, R. J. Linderman, K. J. Manley, B. A. Pearlman, A. Wells, A. Zaks and T. Y. Zhang, Green Chem., 2007, 9, 411 RSC.
- Selected all-carbon quaternary centers as bioactive compounds:
(a) T. Ling and F. Rivas, Tetrahedron, 2016, 72, 6729 CrossRef CAS;
(b) D. J. Newman and G. M. Cragg, J. Nat. Prod., 2016, 79, 629 CrossRef CAS PubMed;
(c) K. C. Nicolaou, S. A. Snyder, X.-H. Huang, K. B. Simonsen, A. E. Koumbis and A. Bigot, J. Am. Chem. Soc., 2004, 126, 10162 CrossRef CAS PubMed;
(d) M. K. Christensen, K. D. Erichsen, C. Trojel-Hansen, J. Tjørnelund, S. J. Nielsen, K. Frydenvang, T. N. Johansen, B. Nielsen, M. Sehested, P. B. Jensen, M. Ikaunieks, A. Zaichenko, E. Loza, I. Kalvinsh and F. Björkling, J. Med. Chem., 2010, 53, 7140 CrossRef CAS;
(e) S. Piacente, P. Montoro, W. Oleszek and C. Pizza, J. Nat. Prod., 2004, 67, 882 CrossRef CAS PubMed;
(f) X. Peng, Z. Hu, J. Zhang, W. Ning, S. Zhang, C. Dong, X. Shi and H.-B. Zhou, Chem. Commun., 2019, 55, 14570 RSC;
(g) S. Daum, M. Schumann, S. Mathea, T. Aumuller, M. A. Balsley, S. L. Constant, B. F. de Lacroix, F. Kruska, M. Braun and C. Schiene-Fischer, Biochemistry, 2009, 48, 6268 CrossRef CAS;
(h) K. D. Wellington, R. C. Cambie, P. S. Rutledge and P. R. Bergquist, J. Nat. Prod., 2000, 63, 79 CrossRef CAS.
- Selected examples of the all-carbon quaternary centers formation:
(a) R. Long, J. Huang, J. Gong and Z. Yang, Nat. Prod. Rep., 2015, 32, 1584 RSC;
(b) I. Marek, Y. Minko, M. Pasco, T. Mejuch, N. Gilboa, H. Chechik and P. J. Das, J. Am. Chem. Soc., 2014, 136, 2682 CrossRef CAS PubMed;
(c) B. M. Trost and C. Jiang, Synthesis, 2006, 369 CrossRef CAS;
(d) K. W. Quasdorf and L. E. Overman, Nature, 2014, 516, 181 CrossRef CAS PubMed;
(e) C. G. Watson, A. Balanta, T. G. Elford, S. Essafi, J. N. Harvey and V. K. Aggarwal, J. Am. Chem. Soc., 2014, 136, 17370 CrossRef CAS PubMed;
(f) R. Alam, T. Vollgraff, L. Eriksson and K. J. Szabj, J. Am. Chem. Soc., 2015, 137, 11262 CrossRef CAS PubMed.
- Selected examples of constructing all-carbon quaternary centers by CDC reactions:
(a) G. Zhang, Y. Zhang and R. Wang, Angew. Chem., Int. Ed., 2011, 50, 10429 CrossRef CAS PubMed;
(b) T. Tanaka, K. Hashiguchi, T. Tanaka, R. Yazaki and T. Ohshima, ACS Catal., 2018, 8, 8430 CrossRef CAS;
(c) G. Hong, P. D. Nahide, U. K. Neelam, P. Amadeo, A. Vijeta, J. M. Curto, C. E. Hendrick, K. F. VanGelder and M. C. Kozlowski, ACS Catal., 2019, 9, 3716 CrossRef CAS;
(d) J. E. M. N. Klein, A. Perry, D. S. Pugh and R. J. K. Taylor, Org. Lett., 2010, 12, 3446 CrossRef CAS PubMed;
(e) C. L. Moody, V. Franckevičius, P. Drouhin, J. E. M. N. Klein and R. J. K. Taylor, Tetrahedron Lett., 2012, 53, 1897 CrossRef CAS;
(f) T. E. Hurst, R. M. Gorman, P. Drouhin, A. Perry and R. J. K. Taylor, Chem.–Eur. J., 2014, 20, 14063 CrossRef CAS PubMed;
(g) T. E. Hurst and R. J. K. Taylor, Eur. J. Org. Chem., 2017, 2017, 203 CrossRef CAS PubMed;
(h) Y.-X. Jia and E. P. Kündig, Angew. Chem., Int. Ed., 2009, 48, 1636 CrossRef CAS;
(i) C. Dey, E. Larionov and E. P. Kündig, Org. Biomol. Chem., 2013, 11, 6734 RSC;
(j) J. R. Donald, R. J. K. Taylor and W. F. Petersen, J. Org. Chem., 2017, 82, 11288 CrossRef CAS PubMed;
(k) Y. Liu, J. Li, X. Ye, X. Zhao and Z. Jiang, Chem. Commun., 2016, 52, 13955 RSC;
(l) Z. Tang, Z. Liu, Z. Tong, Z. Xu, C. T. Au, R. Qiu and N. Kambe, Org. Lett., 2019, 21, 5152 CrossRef CAS PubMed.
- Z. Tang, L. Peng, Y. Yuan, T. Li, R. Qiu and N. Kambe, J. Org. Chem., 2020, 85, 5300 CrossRef CAS PubMed.
- 2-Position of phenol:
(a) Z. Liu, Y. Jiang, C. Liu, L. Zhang, J. Wang, T. Li, H. Zhang, M. H. Li and X. Yang, J. Org. Chem., 2020, 85, 7386 CrossRef CAS PubMed;
(b) D. Glavač, N. Topolovčan and M. Gredičak, J. Org. Chem., 2020, 85, 14253 CrossRef PubMed;
(c) C–S construction: W. Jiang, J. Zhuge, J. Li, G. Histand and D. Lin, J. Org. Chem., 2020, 85, 2415 CrossRef CAS PubMed;
(d) A. J. Basson and M. G. McLaughlin, J. Org. Chem., 2020, 85, 5615 CrossRef CAS PubMed.
-
(a) E. M. Simmons and J. F. Hartwig, Angew. Chem., Int. Ed., 2012, 51, 3066 CrossRef CAS PubMed;
(b) P. K. Pramanick, Z. Zhou, Z.-L. Hou and B. Yao, J. Org. Chem., 2019, 84, 5684 CrossRef CAS PubMed.
-
(a) S. Jin, B. Xie, S. Lin, C. Min, R. Deng and Z. Yan, Org. Lett., 2019, 21, 3436 CrossRef CAS PubMed;
(b) H. Peng, J.-T. Yu, Y. Jiang, H. Yang and J. Cheng, J. Org. Chem., 2014, 79, 9847 CrossRef CAS PubMed;
(c) C. Pan, Q. Ni, Y. Fu and J.-T. Yu, J. Org. Chem., 2017, 82, 7683 CrossRef CAS PubMed.
|
This journal is © The Royal Society of Chemistry 2022 |
Click here to see how this site uses Cookies. View our privacy policy here.