DOI:
10.1039/D1SC05629H
(Edge Article)
Chem. Sci., 2022,
13, 775-780
Borane-catalyzed cascade Friedel–Crafts alkylation/[1,5]-hydride transfer/Mannich cyclization to afford tetrahydroquinolines†
Received
12th October 2021
, Accepted 20th December 2021
First published on 20th December 2021
Abstract
An unprecedented redox-neutral annulation reaction of tertiary anilines with electron-deficient alkynes was developed that proceeds through a cascade Friedel–Crafts alkylation/[1,5]-hydride transfer/Mannich cyclization sequence. Under B(C6F5)3 catalysis, a range of functionalized 1,2,3,4-tetrahydroquinolines were facilely constructed in moderate to good yields with exclusive 3,4-anti-stereochemistry. The commercial availability of the catalyst and the high atom and step economy of the procedure, together with metal-free and external oxidant-free conditions, make this an attractive method in organic synthesis.
Introduction
Tetrahydroquinoline (THQ) is one of the most valuable N-heterocyclic scaffolds that exists in a large number of natural products and bioactive molecules.1 Due to its importance, development of efficient methods to construct the THQ skeleton has been extensively explored in organic synthesis. Various approaches have been established, including intramolecular cyclization,2 Povarov reactions,3 hydrogenation of quinolines4 and others.5 Among all the strategies, the oxidative Povarov reaction, a dehydrogenative [4 + 2] annulation reaction between N-alkylanilines and alkenes, represents a promising protocol for the construction of THQs from simple starting materials (Scheme 1a).6 In this reaction, two new C–C bonds were formed via a cascade sp3 and sp2 C–H functionalization process. However, such a process requires stoichiometric oxidants to convert N-alkylanilines to iminium ions or α-aminoalkyl radical intermediates, which then undergo reaction with activated olefins. The development of redox-neutral [4 + 2] annulation reaction for the synthesis of THQs, which avoids the use of external oxidants, will be highly desirable. We hypothesize that the combination of N-alkylanilines and electron-deficient alkynes represents a promising approach (Scheme 1b). In addition to the attractive feature of high atom-economy, it is worth noting that THQs produced in this fashion also possess complementary substitution patterns compared to previous work. However, challenges need to be addressed to identify an effective catalytic system that promotes an efficient cascade Friedel–Crafts type addition to the alkyne followed by cyclization.
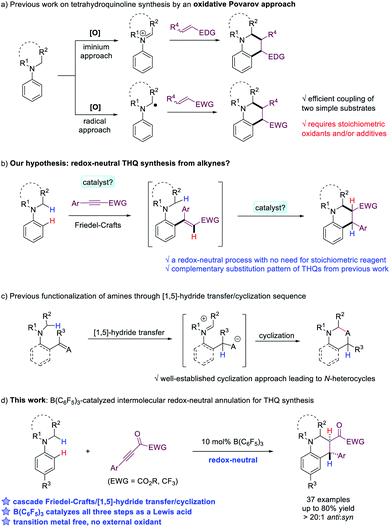 |
| Scheme 1 Different catalytic strategies for THQ synthesis. | |
For the proposed cyclization step, it is noteworthy that a [1,5]-hydride transfer/cyclization process has been developed into a powerful and versatile protocol for the synthesis of structurally diverse O- or N-heterocycles including THQs in recent years (Scheme 1c).7 In such processes, ortho-substituted functionalities such as aldehydes, ketones, imines and electron-deficient alkenes serve as hydride acceptors to construct N-heterocycles with high efficiency and fidelity.8 However, it is necessary to construct ortho-disubstituted substrates for intramolecular cyclization to proceed in literature precedents. If the substrate synthesis from readily available building blocks can be coupled with the cyclization into a cascade process, it should lead to a highly attractive and flexible synthesis of valuable heterocycles.
The last decade has witnessed an explosive development of tris(pentafluorophenyl)borane-catalyzed reactions.9 In addition, B(C6F5)3-promoted hydride transfer of amines and N-alkylanilines10 has also resulted in various step- and atom-economical transformations of these valuable substrates.11–14 In particular, B(C6F5)3-catalyzed intramolecular cyclization of ortho-substituted N,N-dialkyl arylamines via hydride transfer to afford tetrahydroquinoline derivatives was realized by the Paradies group and Wang group, respectively.15,16 Nevertheless, the synthesis of tetrahydroquinolines through B(C6F5)3-catalyzed intermolecular redox-neutral annulation still remains elusive in the literature.
Herein, we report an unprecedented intermolecular redox-neutral annulation reaction of N-alkylanilines with electron-deficient alkynes catalyzed by B(C6F5)3 to deliver a range of poly-substituted tetrahydroquinolines in good to high yields (Scheme 1d). Our mechanistic studies indicate that this efficient and flexible [4 + 2] annulation reaction involves a cascade Friedel–Crafts alkylation/[1,5]-hydride transfer/Mannich cyclization sequence. In all the steps, B(C6F5)3 serves as an effective Lewis acid catalyst with the cooperative effect from TMSOTf.
Results and discussion
At the outset, we intend to study the annulation reaction of N-alkylanilines with alkynes by using B(C6F5)3 as the catalyst. To our delight, the reaction between N,N-4-trimethylaniline 1a and β,γ-alkynyl-α-ketoester 2a occurred smoothly with 10 mol% B(C6F5)3 in toluene at 80 °C. The desired 3,4-anti-tetrahydroquinoline 3a was obtained in 46% yield and > 20
:
1 dr with concomitant formation of the conjugate addition product 4a (Table 1, entry 1). Several other common Lewis acids such as Cu(OTf)2, Mg(OTf)2, In(OTf)3 and BF3·OEt2 turned out to be ineffective for the reaction (entries 2–5). Gratifyingly, the use of a catalytic amount of TMSOTf as an additive, which has been proved to improve the reaction efficiency by the Wang group15b and the Oestreich group,12g was beneficial for the [4 + 2] annulation reaction, affording the desired product 3a in 69% yield, while no byproduct 4a was observed (entry 6). Next, the solvent effect was evaluated. While dioxane, m-xylene and DCM gave slightly lower or similar yields (entries 7–9), no product or significantly decreased yield was obtained by the use of MeCN or THF as the solvent (entries 10–11). No improvement was obtained by increasing the amount of TMSOTf (entry 12). It is noteworthy that no product could be detected when TMSOTf itself was employed as the catalyst (entry 13).
Table 1 Optimization of the reaction conditionsa
With the optimal conditions in hand (Table 1, entry 6), the generality of this B(C6F5)3-catalyzed redox-neutral annulation reaction between N-alkylanilines 1 and electron-deficient alkynes 2 was investigated (Scheme 2). Various para-substituted N,N-dimethylanilines 1 were compatible in this reaction and delivered the corresponding products 3a–3g in 51–69% yields. The structure of 3c has been unambiguously confirmed by X-ray crystallographic analysis. A lower yield was obtained for 4-bromo-N,N-dimethylaniline. Disubstituted N,N-dimethylanilines underwent conjugate addition favorably at the sterically less demanding position, furnishing products 3i and 3j in 57 and 74% yields, respectively. When one of the methyl groups on the amino moiety was replaced by the benzyl group, the reaction proceeded chemoselectively at the benzyl group to form 3k in 67% yield with 4.5
:
1 dr, which indicates the superior hydride donor capability of benzylic over primary C–H bonds.8d,i,k,t The structure of 3k has been unambiguously confirmed by X-ray crystallographic analysis (see ESI†). Additionally, different functional groups on the amino moiety including alkene and alkyne were well tolerated under this catalytic system at ambient reaction temperature. The desired products 3l and 3m were obtained in moderate yields. Cyclic amine derivatives showed good reactivity in this reaction, and products 3n–3p were obtained in 44–67% yields. When studying further N,N-dialkyl arylamines, we found that N-aryl tetrahydro-isoquinoline was not compatible with the reaction conditions, and only trace amounts of product were observed. Next, the variation of β,γ-alkynyl-α-ketoesters 2 was studied. Substrates 2 containing electron-neutral, electron-donating and electron-withdrawing groups on the ortho-, meta- and para-positions on the aryl ring were well tolerated under the standard reaction conditions, giving products 3q–3ab in 56–73% yields and high anti diastereoselectivity. In addition, 2-naphthyl and 2-thienyl substituents have been tested, providing products 3ac and 3ad in 70 and 59% yields, respectively. The reaction of methyl ester with 1a led to the desired product 3ae in 70% yield.
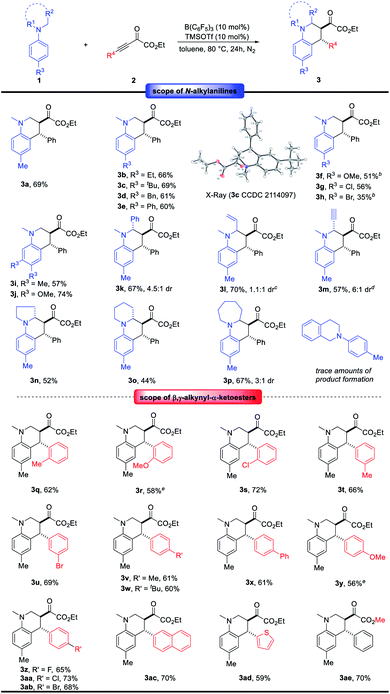 |
| Scheme 2 Scope of redox-neutral annulation between N-alkylanilines and β,γ-alkynyl-α-ketoesters. a See Table 1 and ESI† for a detailed procedure, unless otherwise noted, the dr of 3 was >20 : 1. b The reaction was performed with 10 mol% B(C6F5)3 and 20 mol% TMSOTf for 48 hours. c The reaction was performed at 25 °C for 48 hours. d The reaction was performed with 15 mol% B(C6F5)3 and 10 mol% TMSOTf at 25 °C for 72 hours. e The reaction was performed for 36 hours. | |
The redox-neutral annulation between N,N-4-trimethyl-aniline 1a and trifluoromethyl-α,β-ynones 5 was also investigated. As exemplified in Scheme 3, the reactions went smoothly under the current B(C6F5)3 catalytic system. An array of trifluoromethylated tetrahydroquinolines 6a–6f were prepared in moderate to good yields with high diastereoselectivity (>20
:
1, anti
:
syn).
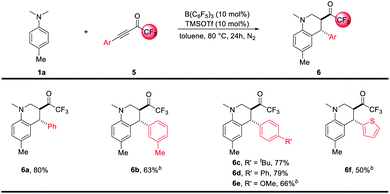 |
| Scheme 3 Scope of redox-neutral annulation between N-alkylanilines and trifluoromethyl-α,β-ynones. a See Table 1 and ESI† for a detailed procedure, the dr of 6 was >20 : 1. b The reaction was performed with 10 mol% B(C6F5)3 and 20 mol% TMSOTf for 52 hours. | |
To shed light on the mechanism of the redox-neutral annulation, a series of control experiments were performed (Scheme 4). When the reaction between 1a and 2a was performed at a slightly lower temperature (60 °C) for 24 hours, the starting material 2a was consumed and the desired product 3a was obtained in diminished yield with concomitant formation of 4a (Scheme 4a, eqn (1)). Subsequently, annulation of 4a could occur under the standard conditions to generate 3a in 74% yield (Scheme 4a, eqn (2)). These results indicated that a cascade Friedel–Crafts alkylation/intramolecular hydride transfer/Mannich cyclization sequence was included in the redox-neutral [4 + 2] annulation reaction. The hydride transfer is probably the rate-determining step. We performed the intramolecular cyclization of 4a without the addition of TMSOTf or B(C6F5)3 (Scheme 4a, eqn (2)). The reactivity of intramolecular cyclization could be enhanced in the presence of TMSOTf. However, probably due to the relatively weak Lewis acidity, TMSOTf itself was unable to promote the reaction. Next, the reaction of isotope-labelled 1a-[D6] with 2a under standard conditions was performed, giving 3a-[D6] in 56% yield with deuterium exclusively transferred to the benzylic position (Scheme 4b, eqn (3)). Besides, the reaction of 2a with a mixture of 1a-[D6] and 1e under standard conditions provided a mixture of 3a-[D6] and 3e without exchange of H/D (Scheme 4b, eqn (4)). These observations suggested that a [1,5]-hydride shift process was involved in the reaction and excluded the hydride abstraction mediated by the borane.15 A crossover experiment using 4a and 4a-[D6] was performed then in a shortened reaction time (Scheme 4c). At low conversions, a significant kinetic isotope effect (KIE) of 3.0 was observed, revealing that the [1,5]-hydride transfer could be involved in the rate-determining step.
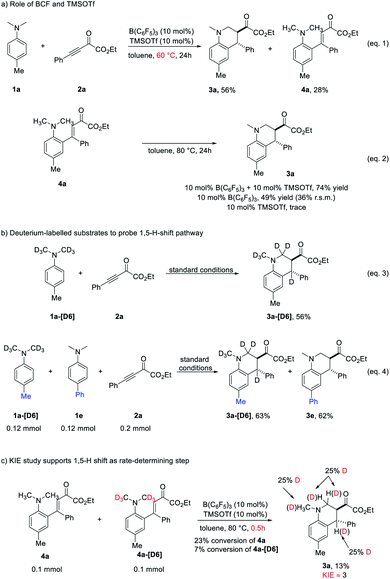 |
| Scheme 4 Control experiments. | |
Based on the experimental results and previous report,15c a plausible mechanism for the B(C6F5)3-catalyzed redox-neutral [4 + 2] annulation of N-alkylanilines and electron-deficient alkynes is proposed in Scheme 5. The reaction is initiated by the activation of β,γ-alkynyl-α-ketoester 2a with B(C6F5)3, which results in the formation of electrophilic alkyne I. N,N-4-trimethylaniline 1a reacts with intermediate I to form allenolate intermediate II. Subsequent rearomatization and protonation of the allenolate generate intermediate III. 1,5-Hydride transfer then occurs with the assistance of B(C6F5)3 to afford iminium enolate IV. Final intramolecular Mannich cyclization produced tetrahydroquinoline product 3a with the regeneration of B(C6F5)3.
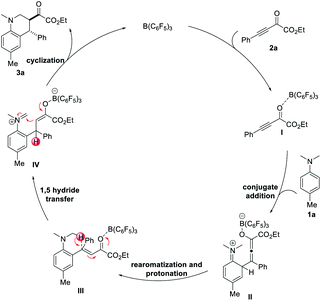 |
| Scheme 5 Proposed catalytic cycle. | |
Conclusions
In summary, we have developed a B(C6F5)3-catalyzed cascade reaction of tertiary anilines with electron-deficient alkynes to construct poly-substituted tetrahydroquinoline derivatives with excellent step and atom economy. This redox neutral reaction involves a sequential Friedel–Crafts alkylation/[1,5]-hydride transfer/Mannich cyclization sequence without using a transition metal or an external oxidant. An array of 1,2,3,4-tetrahydroquinolines were accessed in high efficiency and diastereoselectivity.
Data availability
All experimental and characterization data in this article are available in the ESI.† Crystallographic data for compounds 3c and 3k have been deposited in the Cambridge Crystallographic Data Centre (CCDC) under accession numbers CCDC 2114097 and 2114110, respectively.†
Author contributions
B.-B. Z. performed the synthetic experiments and analysed the data, with help from S. P., F. W., C. L., J. N. and Z. C.; C. M. and G. Y. directed the project and wrote the manuscript. All authors discussed the results and commented on the manuscript.
Conflicts of interest
There are no conflicts to declare.
Acknowledgements
We are grateful for the generous financial support from the National Natural Science Foundation of China (21901066 and 21702053) and the Hubei Provincial Department of Education project (Q20181003). We thank Professor Yu Zhao for helpful discussions.
Notes and references
-
(a) V. Sridharan, P. A. Suryavanshi and J. C. Menéndez, Chem. Rev., 2011, 111, 7157–7259 CrossRef CAS;
(b) I. Muthukrishnan, V. Sridharan and J. C. Menéndez, Chem. Rev., 2019, 119, 5057–5191 CrossRef CAS.
-
(a) M. Kretzschmar, F. Hofmann, D. Moock and C. Schneider, Angew. Chem., Int. Ed., 2018, 57, 4774–4778 CrossRef CAS PubMed;
(b) S. C. Cosgrove, J. M. C. Plane and S. P. Marsden, Chem. Sci., 2018, 9, 6647–6652 RSC;
(c) J.-S. Yu, M. Espinosa, H. Noda and M. Shibasaki, J. Am. Chem. Soc., 2019, 141, 10530–10537 CrossRef CAS PubMed;
(d) F. Mühlhaus, H. Weißbarth, T. Dahmen, G. Schnakenburg and A. Gansäuer, Angew. Chem., Int. Ed., 2019, 58, 14208–14212 CrossRef;
(e) W. Liu, P. Zhou, J. Lang, S. Dong, X. Liu and X. Feng, Chem. Commun., 2019, 55, 4479–4482 RSC;
(f) Y.-H. Wang, J.-S. Tian, P.-W. Tan, Q. Cao, X.-X. Zhang, Z.-Y. Cao, F. Zhou, X. Wang and J. Zhou, Angew. Chem., Int. Ed., 2020, 59, 1634–1643 CrossRef CAS;
(g) R. K. Tak, F. Amemiya, H. Noda and M. Shibasaki, Chem. Sci., 2021, 12, 7809–7817 RSC.
-
(a) T. S. Bush, G. P. A. Yap and W. J. Chain, Org. Lett., 2018, 20, 5406–5409 CrossRef CAS;
(b) P. Cerfontaine, F. Driessens, M.-F. Deltent and S. Petit, Org. Process Res. Dev., 2020, 24, 807–816 CrossRef CAS;
(c) J.-X. Huang, K.-Q. Hou, Q.-L. Hu, X.-P. Chen, J. Li, A. S. C. Chan and X.-F. Xiong, Org. Lett., 2020, 22, 1858–1862 CrossRef CAS;
(d) Y.-Q. Zhang, Y.-P. Zhang, Y.-X. Zheng, Z.-Y. Li and L.-W. Ye, Cell Rep. Phys. Sci., 2021, 2, 100448 CrossRef CAS.
-
(a) I. Sorribes, L. Liu, A. Doménech-Carbó and A. Corma, ACS Catal., 2018, 8, 4545–4557 CrossRef CAS;
(b) H. Wang, Y. Huang, Q. Jiang, X. Dai and F. Shi, Chem. Commun., 2019, 55, 3915–3918 RSC;
(c) C. Liu, M. Wang, S. Liu, Y. Wang, Y. Peng, Y. Lan and Q. Liu, Angew. Chem., Int. Ed., 2021, 60, 5108–5113 CrossRef CAS PubMed.
-
(a) M.-M. Zhang, Y.-N. Wang, B.-C. Wang, X.-W. Chen, L.-Q. Lu and W.-J. Xiao, Nat. Commun., 2019, 10, 2716 CrossRef;
(b) E. M. Larin, J. Loup, I. Polishchuk, R. J. Ross, A. Whyte and M. Lautens, Chem. Sci., 2020, 11, 5716–5723 RSC;
(c) K. Yuan, F. Dong, X. Yin, S.-S. Li, L. Wang and L. Xu, Org. Chem. Front., 2020, 7, 3868–3873 RSC;
(d) X. Yan, L. Ge, M. Castiñeira Reis and S. R. Harutyunyan, J. Am. Chem. Soc., 2020, 142, 20247–20256 CrossRef CAS PubMed;
(e) Q.-F. Xu-Xu, X. Zhang and S.-L. You, Org. Lett., 2020, 22, 1530–1534 CrossRef CAS;
(f) S. N. Fairuz Binte Sheikh Ismail, B. Yang and Y. Zhao, Org. Lett., 2021, 23, 2884–2889 CrossRef CAS.
-
(a) S. Murata, M. Miura and M. Nomura, J. Org. Chem., 1989, 54, 4700–4702 CrossRef CAS;
(b) L. Huang, X. Zhang and Y. Zhang, Org. Lett., 2009, 11, 3730–3733 CrossRef CAS;
(c) M. Nishino, K. Hirano, T. Satoh and M. Miura, J. Org. Chem., 2011, 76, 6447–6451 CrossRef CAS PubMed;
(d) S. Zhu, A. Das, L. Bui, H. Zhou, D. P. Curran and M. Rueping, J. Am. Chem. Soc., 2013, 135, 1823–1829 CrossRef CAS;
(e) C. Min, A. Sanchawala and D. Seidel, Org. Lett., 2014, 16, 2756–2759 CrossRef CAS PubMed;
(f) G.-Q. Xu, C.-G. Li, M.-Q. Liu, J. Cao, Y.-C. Luo and P.-F. Xu, Chem. Commun., 2016, 52, 1190–1193 RSC;
(g) M.-N. Zhao, L. Yu, R.-R. Hui, Z.-H. Ren, Y.-Y. Wang and Z.-H. Guan, ACS Catal., 2016, 6, 3473–3477 CrossRef CAS;
(h) P. Huang, P. Wang, S. Wang, S. Tang and A. Lei, Green Chem., 2018, 20, 4870–4874 RSC;
(i) X.-L. Yang, J.-D. Guo, T. Lei, B. Chen, C.-H. Tung and L.-Z. Wu, Org. Lett., 2018, 20, 2916–2920 CrossRef CAS PubMed;
(j) J. Li, Z. Gu, X. Zhao, B. Qiao and Z. Jiang, Chem. Commun., 2019, 55, 12916–12919 RSC;
(k) J. Y. Hwang, A. Y. Ji, S. H. Lee and E. J. Kang, Org. Lett., 2020, 22, 16–21 CrossRef CAS PubMed.
- For selected recent reviews, see:
(a) B. Peng and N. Maulide, Chem.–Eur. J., 2013, 19, 13274–13287 CrossRef CAS PubMed;
(b) M. C. Haibach and D. Seidel, Angew. Chem., Int. Ed., 2014, 53, 5010–5036 CrossRef CAS;
(c) S. J. Kwon and D. Y. Kim, Chem. Rec., 2016, 16, 1191–1203 CrossRef CAS;
(d) X.-D. An and J. Xiao, Org. Chem. Front., 2021, 8, 1364–1383 RSC , for a recent general review on C–H bond functionalization of amines, see:;
(e) S. Dutta, B. Li, D. R. L. Rickertsen, D. A. Valles and D. Seidel, SynOpen, 2021, 05, 173–228 CrossRef CAS PubMed.
- For pioneer work, see:
(a) W. D. Verboom, N. Reinhoudt, R. Visser and S. Harkema, J. Org. Chem., 1984, 49, 269–276 CrossRef CAS;
(b) W. H. N. Nijhuis, W. Verboom and D. N. Reinhoudt, J. Am. Chem. Soc., 1987, 109, 3136–3138 CrossRef CAS , for selected examples, see:;
(c) S. J. Pastine, K. M. McQuaid and D. Sames, J. Am. Chem. Soc., 2005, 127, 12180–12181 CrossRef CAS;
(d) S. Murarka, C. Zhang, M. D. Konieczynska and D. Seidel, Org. Lett., 2009, 11, 129–132 CrossRef CAS PubMed;
(e) P. A. Vadola and D. Sames, J. Am. Chem. Soc., 2009, 131, 16525–16528 CrossRef CAS PubMed;
(f) S. Murarka, I. Deb, C. Zhang and D. Seidel, J. Am. Chem. Soc., 2009, 131, 13226–13227 CrossRef CAS;
(g) Y. K. Kang, S. M. Kim and D. Y. Kim, J. Am. Chem. Soc., 2010, 132, 11847–11849 CrossRef CAS PubMed;
(h) G. Zhou and J. Zhang, Chem. Commun., 2010, 46, 6593–6595 RSC;
(i) W. Cao, X. Liu, W. Wang, L. Lin and X. Feng, Org. Lett., 2011, 13, 600–603 CrossRef CAS;
(j) M. C. Haibach, I. Deb, C. K. De and D. Seidel, J. Am. Chem. Soc., 2011, 133, 2100–2103 CrossRef CAS PubMed;
(k) K. Mori, K. Ehara, K. Kurihara and T. Akiyama, J. Am. Chem. Soc., 2011, 133, 6166–6169 CrossRef CAS;
(l) I. D. Jurberg, B. Peng, E. Wostefeld, M. Wasserloos and N. Maulide, Angew. Chem., Int. Ed., 2012, 51, 1950–1953 CrossRef CAS PubMed;
(m) K. Mori, T. Kawasaki and T. Akiyama, Org. Lett., 2012, 14, 1436–1439 CrossRef CAS PubMed;
(n) Y.-Y. Han, W.-Y. Han, X. Hou, X.-M. Zhang and W.-C. Yuan, Org. Lett., 2012, 14, 4054–4057 CrossRef CAS PubMed;
(o) K. Mori, K. Kurihara, S. Yabe, M. Yamanaka and T. Akiyama, J. Am. Chem. Soc., 2014, 136, 3744–3747 CrossRef CAS;
(p) K. Mori, N. Umehara and T. Akiyama, Adv. Synth. Catal., 2015, 357, 901–906 CrossRef CAS;
(q) C. W. Suh, S. J. Kwon and D. Y. Kim, Org. Lett., 2017, 19, 1334–1337 CrossRef CAS PubMed;
(r) S.-S. Li, L. Zhou, L. Wang, H. Zhao, L. Yu and J. Xiao, Org. Lett., 2018, 20, 138–141 CrossRef CAS;
(s) K. Mori, R. Isogai, Y. Kamei, M. Yamanaka and T. Akiyama, J. Am. Chem. Soc., 2018, 140, 6203–6207 CrossRef CAS PubMed;
(t) T. Yoshida and K. Mori, Chem. Commun., 2018, 54, 12686–12689 RSC;
(u) S. Zhao, X. Wang, P. Wang, G. Wang, W. Zhao, X. Tang and M. Guo, Org. Lett., 2019, 21, 3990–3993 CrossRef CAS;
(v) M. Kataoka, Y. Otawa, N. Ido and K. Mori, Org. Lett., 2019, 21, 9334–9338 CrossRef CAS;
(w) K. Yokoo and K. Mori, Org. Lett., 2020, 22, 244–248 CrossRef CAS PubMed.
- For selected reviews, see:
(a) R. L. Melen, Chem. Commun., 2014, 50, 1161–1174 RSC;
(b) M. Oestreich, J. Hermeke and J. Mohr, Chem. Soc. Rev., 2015, 44, 2202–2220 RSC;
(c) D. W. Stephan, Acc. Chem. Res., 2015, 48, 306–316 CrossRef CAS;
(d) J. R. Lawson and R. L. Melen, Inorg. Chem., 2017, 56, 8627–8643 CrossRef CAS PubMed;
(e) J. Lam, K. M. Szkop, E. Mosaferi and D. W. Stephan, Chem. Soc. Rev., 2019, 48, 3592–3612 RSC;
(f) H. Fang and M. Oestreich, Chem. Sci., 2020, 11, 12604–12615 RSC;
(g) Y. Ma, S.-J. Lou and Z. Hou, Chem. Soc. Rev., 2021, 50, 1945–1967 RSC;
(h) G. Kumar, S. Roy and I. Chatterjee, Org. Biomol. Chem., 2021, 19, 1230–1267 RSC.
- For pioneer work, see:
(a) N. Millot, C. C. Santini, B. Fenet and J. M. Basset, Eur. J. Inorg. Chem., 2002, 3328–3335 CrossRef CAS , for selected reviews, see:;
(b) F. Focante, P. Mercandelli, A. Sironi and L. Resconi, Coord. Chem. Rev., 2006, 250, 170–188 CrossRef CAS;
(c) S. Basak, L. Winfrey, B. A. Kustiana, R. L. Melen, L. C. Morrill and A. P. Pulis, Chem. Soc. Rev., 2021, 50, 3720–3737 RSC.
-
(a) M. Shang, J. Z. Chan, M. Cao, Y. Chang, Q. Wang, B. Cook, S. Torker and M. Wasa, J. Am. Chem. Soc., 2018, 140, 10593–10601 CrossRef CAS PubMed;
(b) J. Z. Chan, A. Yesilcimen, M. Cao, Y. Zhang, B. Zhang and M. Wasa, J. Am. Chem. Soc., 2020, 142, 16493–16505 CrossRef CAS PubMed;
(c) Y. Aramaki, N. Imaizumi, M. Hotta, J. Kumagai and T. Ooi, Chem. Sci., 2020, 11, 4305–4311 RSC;
(d) G. Wicker, R. Schoch and J. Paradies, Org. Lett., 2021, 23, 3626–3630 CrossRef CAS PubMed.
-
(a) J. Zhang, S. Park and S. Chang, J. Am. Chem. Soc., 2018, 140, 13209–13213 CrossRef CAS;
(b) R. Li, Y. Chen, K. Jiang, F. Wang, C. Lu, J. Nie, Z. Chen, G. Yang, Y.-C. Chen, Y. Zhao and C. Ma, Chem. Commun., 2019, 55, 1217–1220 RSC;
(c) Y. Chang, A. Yesilcimen, M. Cao, Y. Zhang, B. Zhang, J. Z. Chan and M. Wasa, J. Am. Chem. Soc., 2019, 141, 14570–14575 CrossRef CAS PubMed;
(d) M. Zhou, S. Park and L. Dang, Org. Chem. Front., 2020, 7, 944–952 RSC;
(e) Y. Chen, H.-L. Wan, Y. Huang, S. Liu, F. Wang, C. Lu, J. Nie, Z. Chen, G. Yang and C. Ma, Org. Lett., 2020, 22, 7797–7803 CrossRef CAS PubMed;
(f) Y. Chang, M. Cao, J. Z. Chan, C. Zhao, Y. Wang, R. Yang and M. Wasa, J. Am. Chem. Soc., 2021, 143, 2441–2455 CrossRef CAS PubMed;
(g) H. Fang, K. Xie, S. Kemper and M. Oestreich, Angew. Chem., Int. Ed., 2021, 60, 8542–8546 CrossRef CAS PubMed.
-
(a) A. F. G. Maier, S. Tussing, T. Schneider, U. Flörke, Z.-W. Qu, S. Grimme and J. Paradies, Angew. Chem., Int. Ed., 2016, 55, 12219–12223 CrossRef CAS PubMed;
(b) M. Kojima and M. Kanai, Angew. Chem., Int. Ed., 2016, 55, 12224–12227 CrossRef CAS.
-
(a) J. M. Farrell, Z. M. Heiden and D. W. Stephan, Organometallics, 2011, 30, 4497–4500 CrossRef CAS;
(b) Y. Han, S. Zhang, J. He and Y. Zhang, J. Am. Chem. Soc., 2017, 139, 7399–7407 CrossRef CAS;
(c) T. Wang, L. Wang, C. G. Daniliuc, K. Samigullin, M. Wagner, G. Kehr and G. Erker, Chem. Sci., 2017, 8, 2457–2463 RSC;
(d) S. Zhang, Y. Han, J. He and Y. Zhang, J. Org. Chem., 2018, 83, 1377–1386 CrossRef CAS PubMed;
(e) J. Zhang and S. Chang, J. Am. Chem. Soc., 2020, 142, 12585–12590 CrossRef CAS PubMed;
(f) S. Basak, A. Alvarez-Montoya, L. Winfrey, R. L. Melen, L. C. Morrill and A. P. Pulis, ACS Catal., 2020, 10, 4835–4840 CrossRef CAS PubMed.
-
(a) A. F. G. Maier, S. Tussing, H. Zhu, G. Wicker, P. Tzvetkova, U. Flörke, C. G. Daniliuc, S. Grimme and J. Paradies, Chem.–Eur. J., 2018, 24, 16287–16291 CrossRef CAS PubMed;
(b) J.-J. Tian, N.-N. Zeng, N. Liu, X.-S. Tu and X.-C. Wang, ACS Catal., 2019, 9, 295–300 CrossRef CAS , for B(C6F5)3 catalyzed [1,5]-hydride transfer/cyclization of ortho-substituted N-alkylanilines, see:;
(c) R. Zhou and J. Paradies, Eur. J. Org. Chem., 2021, 6334–6339 CrossRef CAS.
- For other examples on B(C6F5)3-catalyzed synthesis of tetrahydroquinolines via hydrosilylation or hydroboration, see:
(a) N. Gandhamsetty, S. Joung, S.-W. Park, S. Park and S. Chang, J. Am. Chem. Soc., 2014, 136, 16780–16783 CrossRef CAS PubMed;
(b) N. Gandhamsetty, S. Park and S. Chang, Synlett, 2017, 28, 2396–2400 CrossRef CAS;
(c) F. Ding, Y. Zhang, R. Zhao, Y. Jiang, R. L.-Y. Bao, K. Lin and L. Shi, Chem. Commun., 2017, 53, 9262–9264 RSC;
(d) E. Kim, H. J. Jeon, S. Park and S. Chang, Adv. Synth. Catal., 2020, 362, 308–313 CrossRef CAS.
Footnote |
† Electronic supplementary information (ESI) available. CCDC 2114097 and 2114110. For ESI and crystallographic data in CIF or other electronic format see DOI: 10.1039/d1sc05629h |
|
This journal is © The Royal Society of Chemistry 2022 |
Click here to see how this site uses Cookies. View our privacy policy here.