DOI:
10.1039/D1SC06613G
(Edge Article)
Chem. Sci., 2022,
13, 1088-1094
Metal-free, visible-light induced enantioselective three-component dicarbofunctionalization and oxytrifluoromethylation of enamines via chiral phosphoric acid catalysis†
Received
27th November 2021
, Accepted 30th December 2021
First published on 4th January 2022
Abstract
Using diverse carbon-centered radical precursors and electron-rich (hetero)aromatics and alcohols as nucleophiles, a visible-light driven chiral phosphoric acid (CPA) catalyzed asymmetric intermolecular, three-component radical-initiated dicarbofunctionalization and oxytrifluoromethylation of enamines was developed, which provides a straightforward access to chiral arylmethylamines, aza-hemiacetals and γ-amino acid derivatives with excellent enantioselectivity. As far as we know, this is the first example of constructing a chiral C–O bond using simple alcohols via visible-light photocatalysis. Chiral phosphoric acid played multiple roles in the reaction, including controlling the reaction stereoselectivity and promoting the generation of radical intermediates by activating Togni's reagent. Mechanistic studies also suggested the importance of the N–H bond of the enamine and indole for the reactions.
Introduction
Multi-component difunctionalization of alkenes offers a simple and efficient strategy for the synthesis of complex compounds, which allows for the simultaneous construction of two C–X bonds in one-step from readily available alkenes and other starting materials.1,2 Due to the high reactivity and unique philicity, radical species have been extensively investigated in the asymmetric difunctionalization of alkenes via transition metal catalysis.3 Recently, Liu's group4 developed a dual Cu(I)/CPAs catalysis strategy for the asymmetric dicarbofunctionalization of 1,1-diarylalkenes, which proceeded via a radical generation and single-electron oxidation step catalyzed by copper(I) and enantioselective nucleophilic attack step catalyzed by CPAs (Scheme 1a). Another Liu's group also developed a copper(I) catalyzed reaction, which proceed via a mechanistically different way by trapping radical intermediates with chiral metal complexes,5 to achieve the difunctionalization of a series of alkenes6 (Scheme 1b). In both cases, although impressive results have been achieved, metals were necessary for the formation of radical intermediates. Considering green and sustainable synthetic chemistry, the exploration for the catalytic radical asymmetric difunctionalization of olefins under metal-free conditions is highly desired.
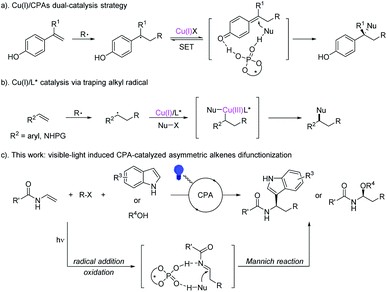 |
| Scheme 1 Asymmetric three-component radical-initiated 1,2-difunctionalization of alkenes. | |
Visible-light photoredox catalysis has been proven to be a powerful and green tool for the organic synthesis via the radical or energy transfer (ET) process.7,8 Combining with organocatalysis,9 Lewis acid catalysis,10 transition metal catalysis11 and even enzymatic catalysis,12 the development of asymmetric photocatalysis has attracted increasing attention of organic chemists. Until now, only a few examples of the photocatalytic asymmetric three-component alkene difunctionalization enabled by photoredox and metal dual catalysis have been reported,13 which deserves to be further explored. Chiral phosphoric acids (CPAs), as powerful bifunctional organic catalysts,14 have been employed in visible-light photoredox catalyzed reactions by Jiang15 and others,16 which proceeded via different enantioselective processes to control the stereoselectivity of the reactions. However, different metals or photocatalysts were required for the generation of corresponding radical species. Thereby, the development of visible-light driven chiral phosphoric acid catalysis without using photocatalysts and metals will be a highly desired supplement to asymmetric visible-light photocatalysis and traditional chiral Brønsted acid catalysis. Especially, we wondered whether or not we could achieve the visible-light induced CPAs-catalyzed asymmetric radical-initiated dicarbofunctionalization of alkenes under photocatalyst-free conditions. Based on the reported works that Brønsted acids could accelerate the reaction by activating Togni's reagent,17 we herein reported a visible-light induced asymmetric intermolecular, three-component radical-initiated dicarbofunctionalization and oxytrifluoromethylation of enamines in the presence of CPAs under the metal-free and photocatalyst-free condition (Scheme 1c).
Results and discussion
We started our investigation by employing enamine 1a, Togni-II 2, and indole 3a as the model substrates (Table 1 and Table S1 in the ESI†). Initially, the reaction was examined in the presence of 5.0 mol% chiral phosphoric acid C1 as the catalyst in CH2Cl2 as the solvent and under the irradiation with a blue LED. To our delight, the expected product 4a was smoothly obtained in moderate yield with good enantioselectivity after 3 h (46% yield, 86% ee; entry 1, Table S1†). After screening of diverse CPAs, solvents and other reaction parameters (Table S1–S6†), the optimal reaction conditions were found to be: 5 mol% C1, 50 mg of 3 Å MS and THF as the solvent, affording the desired product in 54% yield with 96% ee (entry 1, Table 1). The BINOL-based CPAs with different substituents at the 2,2′-position significantly affected the yields and enantioselectivities of the reaction (entries 2 and 3, Table 1). Specifically, no product was obtained when the substituent was triphenylsilyl group (C3) (entry 1, Table 1). Some other enamines (1b–1e) were also tested, and no products or complex reaction mixtures were observed, indicating the importance of the benzoyl group for the generation of 4a (entries 4–7, Table 1). Compared with THF, the mixed solvent provided 4a with the same enantioselectivity, but lower yield (entry 8, Table 1). The addition of extra photocatalysts could not improve the yield and enantioselectivity of 4a (entries 9 and 10, Table 1). The control experiments confirmed that CPA and visible light were essential for the successful formation of 4a (entries 11 and 12, Table 1). In the absence of 3 Å MS, both the ee and yield of 4a fell (entry 13, Table 1).
Table 1 Optimization of the reaction conditionsa
With the reaction conditions optimized, the substrate scope was examined by using various N-acyl enamines. As shown in Table 2, generally, the enantioselective aryltrifluoromethylation reaction occurred smoothly in moderate yields with excellent ee with a variety of substituents tolerated, suggesting that the electronic and steric hindrance effect of the N-acyl groups did not affect the enantioselectivity of the reaction. For instance, the para-OMe, Me, halogen (Cl, F, Br) and phenyl substitution led to the expected products in 33–54% yields and all with excellent enantioselectivity (94–98% ee for 4a–4g). In addition, methyl and chloro substituents at the meta-position of the benzene ring were also well tolerated under the standard conditions and furnished the desirable products with moderate yields and excellent ee (4h, 4i). Moreover, sterically hindered ortho-substituted substrates were also suitable for this reaction, providing the aryltrifluoromethylated products in 33% and 52% yield with excellent enantioselectivity (4j and 4k). The enamine bearing 3,5-dimethyl group was capable of delivering 4l with good levels of yield and enantioselectivity. The heteroaromatic rings like thienyl and furyl groups were also tested under the reaction conditions and smoothly afforded the desired products, especially 4n in high yield with excellent enantioselectivity (66% yield, 97% ee). The enamine from cinnamic acid afforded the product 4o in 30% yield with 91% ee. Then the substrate scope of substituted indoles was evaluated. It was found that indoles with either electron-donating or electron-withdrawing substituents at the 4-, 5-, 6-, or 7-position were well tolerated, furnishing the desired products in moderate yields and all with excellent enantioselectivity (4p–4aa). Additionally, it was observed that the weaker nucleophilic electron-poor indoles exhibited better results for the enantiocontrol than electron-rich ones. Meanwhile, it was interesting to note that other aromatic ring such as 3,5-dimethoxyphenol could also participate in the reaction, furnishing the corresponding aryltrifluoromethylated adduct 4ab in 43% yield with good enantioselectivity (81% ee).
Table 2 Substrate scope of the aryltrifluoromethylation reactiona
Reaction conditions: 1a (0.1 mmol), 2 (0.15 mmol), 3 (0.2 mmol), 3 Å MS (50 mg) and C1 (5 mol%) in THF (1.0 mL) at −20 °C under irradiation of 427 nm kessil LEDs for 3 h; isolated yield after chromatography; enatioselectivity was determined by HPLC on a chiral stationary phase.
For 24 h.
|
|
Encouraged by the above results, we next explored other kinds of nucleophiles, and found that alcohols were also the suitable nucleophiles and the stable aza-hemiacetals were produced (Tables S7† and 3). After the further optimization of the reaction conditions, the corresponding products could be obtained with a higher level of ee under the optimal conditions with 5 mol% C1 as the catalyst in THF at 0 °C. Then various alcohols were tested in the reactions (Table 3). The simple and common alcohol solvents including primary (methanol and ethanol), secondary (isopropanol) and tertiary alcohols (tert-butanol) were all subjected to the reaction, yielding the corresponding oxytrifluoromethylated products (6a–6d) in moderate to good yields (42–69%) with excellent enantioselectivity (89–96% ee). More importantly, more sterically hindered alcohols proceeded with higher enantioselectivities (6dvs.6cvs.6a, 6b). The reaction using deuterated methanol proceeded well to afford the product 6e in 48% yield with 93% ee. Other alcohols with alkyl or (hetero-)aryl groups were also employed as the nucleophiles, leading to desired products in moderate yields with high enantioselectivities (6f–6h). Notably, water was also compatible with the reaction in the absence of molecular sieve, delivering the hydroxylated product 6i in 58% yield and 80% ee.
Table 3 Substrate scope of the oxytrifluoromethylation reactiona
Reaction conditions: 1a (0.1 mmol), 2 (0.15 mmol), 5 (0.1 mmol), 3 Å MS (50 mg) and C1 (5 mol%) in THF (1.0 mL) at 0 °C under irradiation of 427 nm kessil LEDs for 3 h; isolated yield after chromatography; enatioselectivity was determined by HPLC on a chiral stationary phase.
No 3 Å MS.
|
|
In addition to Togni-II, the radical precursors could also be extended to a series of α-bromocarbonyl compounds with the addition of an organic-photocatalyst developed by our group,18 resulting in the direct access to the chiral γ-amino acid derivatives. The optimization of the reaction conditions was shown in the ESI.† Using the organic photocatalyst (cat-CF3Ph) from our group, the substrate scope was then examined (Table 4). The para- or meta-substituted α-bromoacetophenone were proved to be the amenable substrates under the optimal reaction conditions, giving the corresponding products in moderate to good yields (37–65%) with excellent enantioselectivities (92–96%) (8a–8j). Unfortunately, sterically hindered ortho-substituents completely inhibited the reaction. Multi-substituted phenyl and thienyl group did not obviously alter the reactivities and enantioselectivities (8k, 8l). Besides the aryl groups, an α-bromoketone with the alkyl group was also tolerated to afford the product 8m in 51% yield with excellent ee (94%). With high enantioselectivity (87–92%), the enantioselective dicarbofunctionalization could be extended to different bromo-acetate and malonate, enabling the synthesis of chiral amino acids with moderate to good yields (8n–8p). Also, different electronic and structural variated indoles were examined and showed good tolerance of functional groups. Indoles with different substitutes at the 4-, 5-, 6-, or 7-position were successfully converted to the corresponding products in 32–70% yields with excellent enantioselectivities (93–98%), indicating that the electronic effect has no obvious influence on the enantioselectivities of the products.
Table 4 Substrate scope for the synthesis chiral γ-amino acid derivativesa
Reaction conditions: 1c (0.2 mmol), 7 (0.1 mmol), 3 (0.15 mmol), 3 Å MS (100 mg), cat-CF3Ph (1 mol%) and C1 (5 mol%) in THF (0.5 mL) at r.t. under irradiation of 427 nm kessil LEDs for 12 h; isolated yield after chromatography; enatioselectivity was determined by HPLC on a chiral stationary phase.
|
|
In order to further demonstrate the practicality of this protocol in organic synthesis, the gram-scale synthesis and further transformations were carried out (Scheme 2). Using only 1 mol% of C1, the aryltrifluoromethylation reaction was performed with 1a, Togni-II and indole on a 2.5 mmol scale and the other one with α-bromoacetophenone 7a on a 5 mmol scale, offering the corresponding products 4a and 8a in 42% and 53% yield respectively, with the same 96% ee by prolonging the reaction time (Scheme 2a). After recrystallization, the optically pure 8a was obtained, which was subjected to the further transformation. Under a reflux condition, chiral γ-amino ketone 8a could react with hydroxylamine hydrochloride (NH2OH·HCl) to provide oxime 9 in excellent yield and enantioselectivity. Furthermore, 8a could be easily transformed into alkene 10 through Wittig reaction in 99% yield with 99% ee (Scheme 2b).
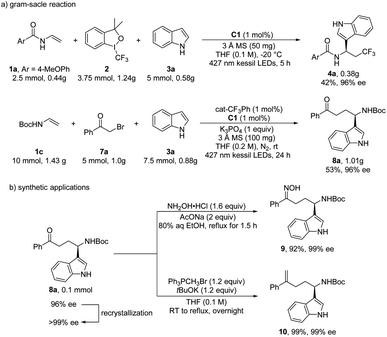 |
| Scheme 2 Gram-scale reaction and derivatizations. | |
To understand the reaction mechanism, several control experiments were performed (Scheme 3). When 2,2,6,6-tetramethyl-1-piperidinyloxy (TEMPO) was added to the reaction mixture under the standard conditions, no product 4a or 8a was generated, indicating that the reaction might involve a radical process (Scheme 3a and ESI†). Trifluoromethylated enamine 11 generated from the reaction of 1a with Togni-II was employed as the substrate to react with indole under the action of chiral phosphoric acid. After 12 h, only trace amount of the product was detected by TLC, indicating that the reaction proceeded via the imine intermediate instead of enamine (Scheme 3b). Using N-Me protected enamines or indoles in the reaction under the standard conditions, the reaction was completely inhibited, indicating that the generated imine and indole might both interact with chiral phosphoric acid by the way of hydrogen-bonding to provide the product (Scheme 3c). When either 1a or indole was not added to the reaction mixture, full conversion of Togni-II was observed, accompanied by the generation of trifluoromethylated products. Treating the Togni-II under the standard conditions, 2 also completely decomposed and the chiral phosphoric acid could accelerate this process (see ESI†). In addition, the UV/Vis absorption spectra and the 1H NMR indicated that there is an interaction between Togni-II and C1 (see Section 6.3 and 6.4 in ESI†). These results suggested that the trifluoromethyl radical was formed through the direct decomposition of Togni-II with the assistance of C1 under the irradiation of visible-light. The linear effect experiments were also performed and the results suggests that only one chiral phosphate molecule was included in the second C–C bond-forming step for the enantiomeric control (Scheme 3d).
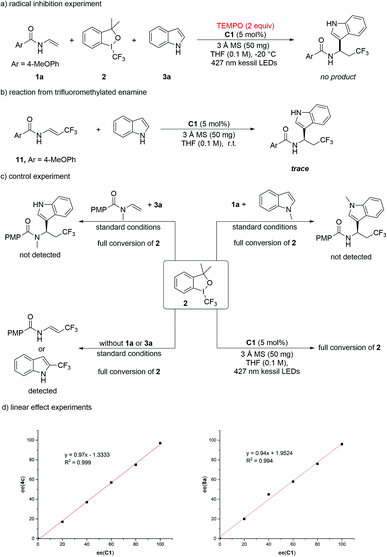 |
| Scheme 3 Mechanistic studies. | |
Based on the mechanistic studies and reported literatures, a plausible reaction mechanism is outlined in Fig. 1. Under the activation of CPA, Togni-II decomposes into the trifluoromethyl radical and related hypervalent iodine intermediate III under the direct irradiation of visible light. Then the trifluoromethyl radical would undergoes a radical addition with enamine to produce the α-amino radical IV, which is then oxidized by hypervalent iodine intermediate III to form the corresponding imine intermediate V. Under the action of the chiral phosphoric acid through the hydrogen-bonding, the imine intermediate V undergoes a Mannich reaction with indole, forming the Ts-I transition state to provide the final chiral products. For the synthesis of chiral γ-amino acid derivatives, a SET process between α-bromocarbonyl compounds and excited PC occurs to afford the corresponding carbon radical intermediate, and then the generated α-amino radical is oxidized by PC˙+ to generate the imine intermediate and complete the photocatalytic cycle.
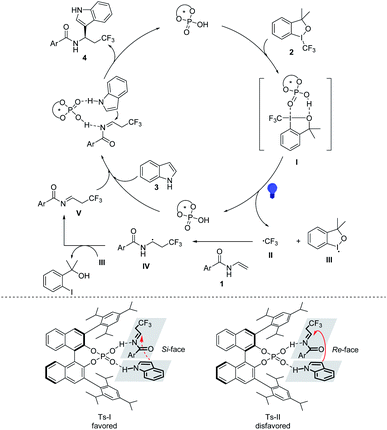 |
| Fig. 1 Proposed reaction mechanism. | |
Conclusion
In summary, we have developed a metal-free, visible-light mediated enantioselective dicarbofunctionalization and oxytrifluoromethylation of enamines in the present of chiral phosphoric acids. Compared with the previous works enabled by transition metals or photocatalysts, Togni-II underwent a direct decomposition to produce the trifluoromethyl radical under the visible-light irradiation. Using (hetero)aromatics and alcohols as nucleophiles, the corresponding chiral arylmethylamines and aza-hemiacetals were obtained in moderate to good yields with excellent enantioselectivities. Notably, for the first time, the chiral C–O bond was constructed from simple alcohols via visible-light photocatalysis. In addition, the radical precursors could also be expanded to various α-bromocarbonyl compounds by addition of an extra organic photocatalyst developed by our group, affording the γ-amino acid derivatives with excellent enantioselectivities. Mechanistic studies suggested that CPAs not only controlled the stereoselectivity but also accelerate the generation of the trifluoromethyl radical through activation of Togni's reagent.
Author contributions
H. L. planned and conducted most of the experiments; D.-S. J. conducted chiral HPLC analysis; G.-Q. X. and Y.-C. L. revised the manuscript; P.-F. X., H. Z. and H. L. directed the projects and wrote the manuscript. All authors contributed to the discussion.
Conflicts of interest
There are no conflicts to declare.
Acknowledgements
We are grateful to the NSFC (21632003, 21871116 and 22071085), the key program of Gansu province (17ZD2GC011), and the “111” program from the MOE of P. R. China, and Syngenta Company for financial support.
Notes and references
- For selected reviews, see
(a) G. Yin, X. Mu and G. Liu, Acc. Chem. Res., 2016, 49, 2413 CrossRef CAS PubMed;
(b) L. M. Wickham and R. Giri, Acc. Chem. Res., 2021, 54, 3415 CrossRef CAS PubMed;
(c) Y. Li, D. Wu, H.-G. Cheng and G. Yin, Angew. Chem., Int. Ed., 2020, 59, 7990 CrossRef CAS PubMed.
- For selected examples, see
(a) X. Liu, Y. Liang, J. Ji, J. Luo and X. Zhao, J. Am. Chem. Soc., 2018, 140, 4782 CrossRef CAS PubMed;
(b) S. Makai, E. Falk and B. Morandi, J. Am. Chem. Soc., 2020, 142, 21548 CrossRef CAS PubMed;
(c) P. Xiong, H. Long, J. Song, Y. Wang, J.-F. Li and H.-C. Xu, J. Am. Chem. Soc., 2018, 140, 16387 CrossRef CAS PubMed;
(d) J. Derosa, V. T. Tran, M. N. Boulous, J. S. Chen and K. M. Engle, J. Am. Chem. Soc., 2017, 139, 10657 CrossRef CAS PubMed;
(e) W. Shu, G. Jia and S. Ma, Angew. Chem., Int. Ed., 2009, 48, 2788 CrossRef CAS PubMed;
(f) J.-N. Mo, W.-L. Yu, J.-Q. Chen, X.-Q. Hu and P.-F. Xu, Org. Lett., 2018, 20, 4471 CrossRef CAS PubMed.
- For selected examples, see
(a) Z.-L. Li, G.-C. Fang, Q.-S. Gu and X.-Y. Liu, Chem. Soc. Rev., 2020, 49, 32 RSC;
(b) S. Sakurai, A. Matsumoto, T. Kano and K. Maruoka, J. Am. Chem. Soc., 2020, 142, 19017 CrossRef CAS PubMed;
(c) D. Anthony, Q. Lin, J. Baudet and T. Diao, Angew. Chem., Int. Ed., 2019, 58, 3198 CrossRef CAS PubMed;
(d) X. Wei, W. Shu, A. García-Domínguez, E. Merino and C. Nevado, J. Am. Chem. Soc., 2020, 142, 13515 CrossRef CAS PubMed;
(e) H.-Y. Tu, F. Wang, L. Huo, Y. Li, S. Zhu, X. Zhao, H. Li, F.-L. Qing and L. Chu, J. Am. Chem. Soc., 2020, 142, 9604 CAS;
(f) Z.-Q. Zhang, X.-Y. Meng, J. Sheng, Q. Lan and X.-S. Wang, Org. Lett., 2019, 21, 8256 CrossRef CAS PubMed;
(g) J.-S. Lin, X.-Y. Dong, T.-T. Li, N.-C. Jiang, B. Tan and X.-Y. Liu, J. Am. Chem. Soc., 2016, 138, 9357 CrossRef CAS PubMed;
(h) X.-Y. Dong, J.-T. Cheng, Y.-F. Zhang, Z.-L. Li, T.-Y. Zhan, J.-J. Chen, F.-L. Wang, N.-Y. Yang, L. Ye, Q.-S. Gu and X.-Y. Liu, J. Am. Chem. Soc., 2020, 142, 9501 CrossRef CAS PubMed.
- J.-S. Lin, T.-T. Li, J.-R. Liu, G.-Y. Jiao, Q.-S. Gu, J.-T. Cheng, Y.-L. Guo, X. Hong and X.-Y. Liu, J. Am. Chem. Soc., 2019, 141, 1074 CrossRef CAS PubMed.
- For selected reviews and examples, see
(a) C. Li, B. Chen, X. Ma, X. Mo and G. Zhang, Angew. Chem., Int. Ed., 2021, 60, 2130 CrossRef CAS PubMed;
(b) Y. Liang and G. C. Fu, J. Am. Chem. Soc., 2015, 137, 9523 CrossRef CAS PubMed;
(c) W. Zhang, F. Wang, S. D. McCann, D. Wang, P. Chen, S. S. Stahl and G. Liu, Science, 2016, 353, 1014 CrossRef CAS PubMed;
(d) J. Schmidt, J. Choi, A. T. Liu, M. Slusarczyk and G. C. Fu, Science, 2016, 354, 1265 CrossRef CAS PubMed;
(e) Z. Wang, S. Bachman, A. S. Dudnik and G. C. Fu, Angew. Chem., Int. Ed., 2018, 57, 14529 CrossRef CAS PubMed.
- For selected examples, see
(a) F. Wang, D. Wang, X. Wan, L. Wu, P. Chen and G. Liu, J. Am. Chem. Soc., 2016, 138(48), 15547 CrossRef CAS PubMed;
(b) L. Wu, F. Wang, X. Wan, D. Wang, P. Chen and G. Liu, J. Am. Chem. Soc., 2017, 139, 2904 CrossRef CAS PubMed;
(c) L. Wu, F. Wang, P. Chen and G. Liu, J. Am. Chem. Soc., 2019, 141, 1887 CrossRef CAS PubMed;
(d) L. Fu, S. Zhou, X. Wan, P. Chen and G. Liu, J. Am. Chem. Soc., 2018, 140, 10965 CrossRef CAS PubMed;
(e) G. Zhang, L. Fu, P. Chen, J. Zou and G. Liu, Org. Lett., 2019, 21, 5015 CrossRef CAS PubMed;
(f) G. Zhang, S. Zhou, L. Fu, P. Chen, Y. Li, J. Zou and G. Liu, Angew. Chem., Int. Ed., 2020, 59, 20439 CrossRef CAS PubMed.
- For selected reviews, see
(a) F. Strieth-Kalthoff, M. J. James, M. Teders, L. Pitzer and F. Glorius, Chem. Soc. Rev., 2018, 47, 7190 RSC;
(b) D. M. Schultz and T. P. Yoon, Science, 2014, 343, 1239176 CrossRef PubMed;
(c) C. K. Prier, D. A. Rankic and D. W. C. MacMillan, Chem. Rev., 2013, 113, 5322 CrossRef CAS PubMed.
- For selected examples, see
(a) M. T. Pirnot, D. A. Rankic, D. B. C. Martin and D. W. C. MacMillan, Science, 2013, 339, 1593 CrossRef CAS PubMed;
(b) J. B. McManus and D. A. Nicewicz, J. Am. Chem. Soc., 2017, 139, 2880 CrossRef CAS PubMed;
(c) M. A. Ischay, Z. Lu and T. P. Yoon, J. Am. Chem. Soc., 2010, 132, 8572 CrossRef CAS PubMed;
(d) A. Trowbridge, D. Reich and M. J. Gaunt, Nature, 2018, 561, 522 CrossRef CAS PubMed;
(e) G.-Q. Xu, J.-T. Xu, Z.-T. Feng, H. Liang, Z.-Y. Wang, Y. Qin and P.-F. Xu, Angew. Chem., Int. Ed., 2018, 57, 5110 CrossRef CAS PubMed;
(f) S. U. Dighe, F. Juliá, A. Luridiana, J. J. Douglas and D. Leonori, Nature, 2020, 584, 75 CrossRef PubMed;
(g) W.-L. Yu, J.-Q. Chen, Y.-L. Wei, Z.-Y. Wang and P.-F. Xu, Chem. Commun., 2018, 54, 1948 RSC;
(h) W.-L. Yu, Y.-C. Luo, L. Yan, D. Liu, Z.-Y. Wang and P.-F. Xu, Angew. Chem., Int. Ed., 2019, 58, 10941 CrossRef CAS PubMed.
-
(a) D. A. Nicewicz and D. W. C. MacMillan, Science, 2008, 322, 77 CrossRef CAS PubMed;
(b) H.-W. Shih, M. N. Vander Wal, R. L. Grange and D. W. C. MacMillan, J. Am. Chem. Soc., 2010, 132, 13600 CrossRef CAS PubMed;
(c) D. A. DiRocco and T. Rovis, J. Am. Chem. Soc., 2012, 134, 8094 CrossRef CAS PubMed;
(d) D. Uraguchi, Y. Kimura, F. Ueoka and T. Ooi, J. Am. Chem. Soc., 2020, 142, 19462 CrossRef CAS PubMed;
(e) G. Bergonzini, C. S. Schindler, C.-J. Wallentin, E. N. Jacobsen and C. R. J. Stephenson, Chem. Sci., 2014, 5, 112 RSC;
(f) F. M. Hörmann, C. Kerzig, T. S. Chung, A. Bauer, O. S. Wenger and T. Bach, Angew. Chem., Int. Ed., 2020, 59, 9659 CrossRef PubMed;
(g) J.-L. Zhang, J.-Y. Liu, G.-Q. Xu, Y.-C. Luo, H. Lu, C.-Y. Tan, X.-Q. Hu and P.-F. Xu, Org. Lett., 2021, 23, 3287 CrossRef CAS PubMed.
-
(a) J. Du, K. L. Skubi, D. M. Schultz and T. P. Yoon, Science, 2014, 344, 392 CrossRef CAS PubMed;
(b) A. G. Amador, E. M. Sherbrook and T. P. Yoon, J. Am. Chem. Soc., 2016, 138, 4722 CrossRef CAS PubMed;
(c) H. Huo, X. Shen, C. Wang, L. Zhang, P. Röse, L.-A. Chen, K. Harms, M. Marsch, G. Hilt and E. Meggers, Nature, 2014, 515, 100 CrossRef CAS PubMed;
(d) C. Zhang, S. Chen, C.-X. Ye, K. Harms, L. Zhang, K. N. Houk and E. Meggers, Angew. Chem., Int. Ed., 2019, 58, 14462 CrossRef CAS PubMed;
(e) W. Ding, L.-Q. Lu, Q.-Q. Zhou, Y. Wei, J. Chen and W.-J. Xiao, J. Am. Chem. Soc., 2017, 139, 63 CrossRef CAS PubMed;
(f) H. Liang, G.-Q. Xu, Z.-T. Feng, Z.-Y. Wang and P.-F. Xu, J. Org. Chem., 2019, 84, 60 CrossRef CAS PubMed.
-
(a) E. Gandolfo, X. Tang, S. R. Roy and P. Melchiorre, Angew. Chem., Int. Ed., 2019, 58, 16854 CrossRef CAS PubMed;
(b) X. Shu, L. Huan, Q. Huang and H. Huo, J. Am. Chem. Soc., 2020, 142, 19058 CrossRef CAS PubMed;
(c) Y.-L. Li, S.-Q. Zhang, J. Chen and J.-B. Xia, J. Am. Chem. Soc., 2021, 143, 7306 CrossRef CAS PubMed;
(d) D. Wang, N. Zhu, P. Chen, Z. Lin and G. Liu, J. Am. Chem. Soc., 2017, 139, 15632 CrossRef CAS PubMed;
(e) Q. M. Kainz, C. D. Matier, A. Bartoszewicz, S. L. Zultanski, J. C. Peters and G. C. Fu, Science, 2016, 351, 681 CrossRef CAS PubMed.
-
(a) J. S. DeHovitz, Y. Y. Loh, J. A. Kautzky, K. Nagao, A. J. Meichan, M. Yamauchi, D. W. C. MacMillan and T. K. Hyster, Science, 2020, 369, 1113 CrossRef CAS PubMed;
(b) B. A. Sandoval, S. I. Kurtoic, M. M. Chung, K. F. Biegasiewicz and T. K. Hyster, Angew. Chem., Int. Ed., 2019, 58, 8714 CrossRef CAS PubMed;
(c) R. C. Betori, C. M. May and K. A. Scheidt, Angew. Chem., Int. Ed., 2019, 58, 16490 CrossRef CAS PubMed;
(d) X. Guo, Y. Okamoto, M. R. Schreier, T. R. Ward and O. S. Wenger, Chem. Sci., 2018, 9, 5052 RSC.
-
(a) F.-D. Lu, L.-Q. Lu, G.-F. He, J.-C. Bai and W.-J. Xiao, J. Am. Chem. Soc., 2021, 143, 4168 CrossRef CAS PubMed;
(b) L. Guo, M. Yuan, Y. Zhang, F. Wang, S. Zhu, O. Gutierrez and L. Chu, J. Am. Chem. Soc., 2020, 142, 20390 CrossRef CAS PubMed;
(c) J. Chen, Y.-J. Liang, P.-Z. Wang, G.-Q. Li, B. Zhang, H. Qian, X.-D. Huan, W. Guan, W.-J. Xiao and J.-R. Chen, J. Am. Chem. Soc., 2021, 143, 13382 CrossRef PubMed;
(d) P.-Z. Wang, Y. Gao, J. Chen, X.-D. Huan, W.-J. Xiao and J.-R. Chen, Nat. Commun., 2021, 12, 1815 CrossRef CAS PubMed;
(e) D. Zheng and A. Studer, Angew. Chem., Int. Ed., 2019, 58, 15803 CrossRef CAS PubMed;
(f) Y. Shen, M.-L. Shen and P.-S. Wang, ACS Catal., 2020, 10, 8247 CrossRef CAS.
- For selected reviews and examples, see
(a) J. P. Reid, L. Simón and J. M. Goodman, Acc. Chem. Res., 2016, 49, 1029 CrossRef CAS PubMed;
(b) H. Wu, Y.-P. He and F. Shi, Synthesis, 2015, 47, 1990 CrossRef CAS;
(c) L. Schreyer, P. S. J. Kaib, V. N. Wakchaure, C. Obradors, R. Properzi, S. Lee and B. List, Science, 2018, 362, 216 CrossRef CAS PubMed;
(d) I. Čorić and B. List, Nature, 2012, 483, 315 CrossRef PubMed;
(e) J. Jin, Y. Zhao, A. Gouranourimi, A. Ariafard and P. W. H. Chan, J. Am. Chem. Soc., 2018, 140, 5834 CrossRef CAS PubMed;
(f) Y.-Y. Wang, K. Kanomata, T. Korenaga and M. Terada, Angew. Chem., Int. Ed., 2016, 55, 927 CrossRef CAS PubMed;
(g) Y.-H. Chen, H.-H. Li, X. Zhang, S.-H. Xiang, S. Li and B. Tan, Angew. Chem., Int. Ed., 2020, 59, 11374 CrossRef CAS PubMed;
(h) J. Zhang, P. Yu, S.-Y. Li, H. Sun, S.-H. Xiang, J. Wang, K. N. Houk and B. Tan, Science, 2018, 361, eaas8707 CrossRef PubMed;
(i) L. He, X.-H. Chen, D.-N. Wang, S.-W. Luo, W.-Q. Zhang, J. Yu, L. Ren and L.-Z. Gong, J. Am. Chem. Soc., 2011, 133, 13504 CrossRef CAS PubMed.
-
(a) Y. Yin, Y. Dai, H. Jia, J. Li, L. Bu, B. Qiao, X. Zhao and Z. Jiang, J. Am. Chem. Soc., 2018, 140, 6083 CrossRef CAS PubMed;
(b) L. Lin, X. Bai, X. Ye, X. Zhao, C.-H. Tan and Z. Jiang, Angew. Chem., Int. Ed., 2017, 56, 13842 CrossRef CAS PubMed;
(c) B. Qiao, C. Li, X. Zhao, Y. Yin and Z. Jiang, Chem. Commun., 2019, 55, 7534 RSC;
(d) J. Li, M. Kong, B. Qiao, R. Lee, X. Zhao and Z. Jiang, Nat. Commun., 2018, 9, 2445 CrossRef PubMed;
(e) K. Cao, S. Min Tan, R. Lee, S. Y. H. Jia, X. Zhao, B. Qiao and Z. Jiang, J. Am. Chem. Soc., 2019, 141, 5437 CrossRef CAS PubMed;
(f) Y. Yin, Y. Li, T. P. Gonçalves, Q. Zhan, G. Wang, X. Zhao, B. Qiao, K.-W. Huang and Z. Jiang, J. Am. Chem. Soc., 2020, 142, 19451 CrossRef CAS PubMed.
-
(a) R. S. J. Proctor, H. J. Davis and R. J. Phipps, Science, 2018, 360, 419 CrossRef CAS PubMed;
(b) E. C. Gentry, L. J. Rono, M. E. Hale, R. Matsuura and R. R. Knowles, J. Am. Chem. Soc., 2018, 140, 3394 CrossRef CAS PubMed;
(c) M.-L. Shen, Y. Shen and P.-S. Wang, Org. Lett., 2019, 21, 2993 CrossRef CAS PubMed;
(d) C. B. Roos, J. Demaerel, D. E. Graff and R. R. Knowles, J. Am. Chem. Soc., 2020, 142, 5974 CrossRef CAS PubMed;
(e) Z.-Y. Dai, Z.-S. Nong and P.-S. Wang, ACS Catal., 2020, 10, 4786 CrossRef CAS;
(f) Y.-Z. Cheng, Q.-R. Zhao, X. Zhang and S.-L. You, Angew. Chem., Int. Ed., 2019, 58, 18069 CrossRef CAS PubMed.
-
(a) K. Niedermann, N. Früh, E. Vinogradova, M. S. Wiehn, A. Moreno and A. Togni, Angew. Chem., Int. Ed., 2011, 50, 1059 CrossRef CAS PubMed;
(b) P. Yu, J.-S. Lin, L. Li, S.-C. Zheng, Y.-P. Xiong, L.-J. Zhao, B. Tan and X.-Y. Liu, Angew. Chem., Int. Ed., 2014, 53, 11890 CrossRef CAS PubMed;
(c) R. Koller, Q. Huchet, P. Battaglia, J. M. Welch and A. Togni, Chem. Commun., 2009, 5993 RSC.
-
(a) D. Liu, M.-J. Jiao, Z.-T. Feng, X.-Z. Wang, G.-Q. Xu and P.-F. Xu, Org. Lett., 2018, 20, 5700 CrossRef CAS PubMed;
(b) D. Liu, M.-J. Jiao, X.-Z. Wang and P.-F. Xu, Org. Lett., 2019, 21, 4745 CrossRef CAS PubMed.
Footnote |
† Electronic supplementary information (ESI) available. CCDC 2108166. For ESI and crystallographic data in CIF or other electronic format see DOI: 10.1039/d1sc06613g |
|
This journal is © The Royal Society of Chemistry 2022 |
Click here to see how this site uses Cookies. View our privacy policy here.