DOI:
10.1039/D2SC01700H
(Edge Article)
Chem. Sci., 2022,
13, 8759-8765
Recyclable fluorous-tag assisted two-directional oligosaccharide synthesis enabled by interrupted Pummerer reaction mediated glycosylation†
Received
24th March 2022
, Accepted 21st June 2022
First published on 22nd June 2022
Abstract
Herein, we report a novel fluorous-tag assisted two-directional oligosaccharide assembly strategy, which maintained the high coupling efficiency of solution-phase synthesis and featured the advantage of an easy purification process comparable to solid-phase synthesis. A well-designed fluorous tag was decorated on the latent anomeric leaving group in interrupted Pummerer reaction mediated (IPRm) glycosylation. The high efficiency of the in-solution phase glycosylation and the unique affinity of the fluorous tag towards polytetrafluoroethylene (PTFE) particles allowed flexible assembly from the reducing end to the non-reducing end and fast purification by PTFE-assisted filtration. Moreover, the fluorous-tagged latent anomeric leaving group could be activated by oxidation and cleaved by IPRm glycosylation, thus enabling the elongation of the carbohydrate chain from the non-reducing end to the reducing end as well as the recovery of the fluorous tag. The present two-directional synthetic strategy is used to assemble the repeating unit of Streptococcus pneumoniae type 14 capsular polysaccharide.
Introduction
Saccharides are one of the most abundant biomolecules in nature.1 However, the exploration of their various physiological functions is hampered by their extraordinarily complex chemical structures and poor availabilities. Consequently, the development of efficient oligosaccharide and polysaccharide assembly strategies is of great demand. Significant progress has been made in the past few decades2 as exemplified by the access to many complex oligosaccharides and polysaccharides, especially the remarkable studies of Ye's 92-mer branched arabinogalactan,3 Yu's 128-mer liner glycan in solution-phase synthesis4 and Seeberger's 151-mer glycan in automated solid-phase synthesis.5 Despite these achievements, huge challenges are still encountered in carbohydrate synthesis, which largely encumbered the development of glycoscience. Among these challenges, one of the major obstacles is the tedious purification process.2g Time-consuming and eco-unfriendly column chromatography is always required in conventional solution-phase synthesis (Fig. 1A(a)). Solid-phase synthesis is one of the most representative state of the art solutions to this problem,6–8 however, at the expense of consuming a large excess of carbohydrate building blocks due to the heterogeneous reaction manner, while the carbohydrate building blocks are much more expensive compared to amino acids and nucleotides in solid-phase polypeptide and polynucleotide synthesis (Fig. 1A(b)).
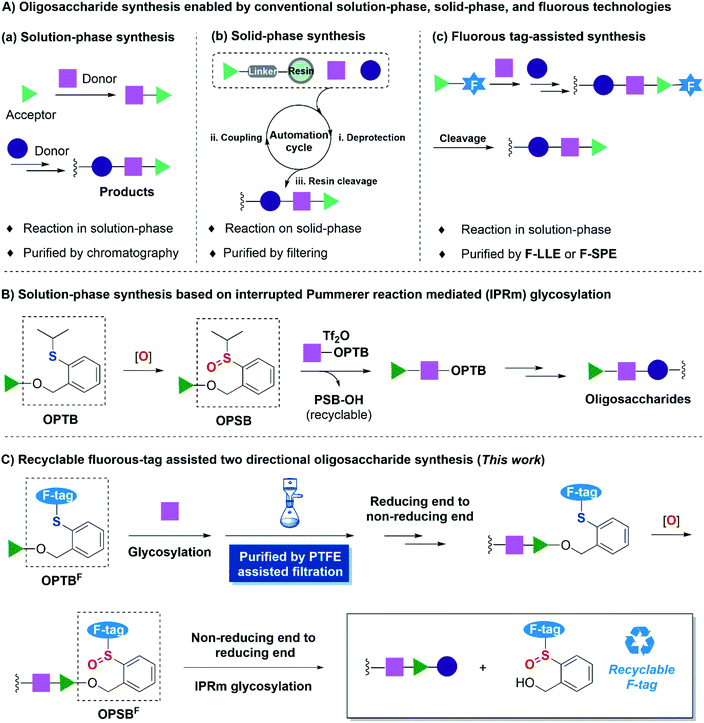 |
| Fig. 1 Prior arts in oligosaccharide assembly and this work. | |
As a complementary type of solution-phase synthesis and solid-phase synthesis, fluorous synthesis pioneered by Zhu,9 Horváth10 and Curran11et al. began to flourish in organic synthesis from the middle 1990s.12,13 Fluorous techniques retain the advantages of solution-phase synthesis and solid-phase purification. In this strategy (Fig. 1A(c)), a fluorous tag was installed on substrates, which allowed the reaction to be performed in a homogenous system. Upon completion of the reaction, fluorous solid-phase extraction (F-SPE)14 or fluorous liquid–liquid extraction (F-LLE)11b,15 was implemented for the purification by virtue of the unique interactions between fluorous-tag labeled substrates and fluorous silica gel or fluorous solvent, thus significantly simplifying the purification processes.16 This superiority has also enriched the carbohydrate synthesis. Since Curran firstly introduced a benzyl type fluorous protecting group as a tag for disaccharide synthesis,17 Seeberger,18 Pohl,19 Mizuno20 and others21 have developed diversified fluorous tags for carbohydrate chemical synthesis in the past few decades. To address the problem of the requirement of expensive fluorous solvent and fluorous silica gel in purification steps, Chai and co-workers introduced cheap and reusable polytetrafluoroethylene (PTFE) particles for F-SPE, which allowed purification by simple filtration, namely PTFE assisted filtration. This process significantly simplified the purification step and made the isolation efficiency comparable to that of solid-phase synthesis.22 Among the various fluorous-tag assisted carbohydrate synthesis methods, the installation of a fluorous tag at the reducing end displayed exceptional advantages due to the stability and the flexibility. It also facilitated the synthesis of carbohydrate microarrays19a and automated solution-phase iterative synthesis19c advanced by Pohl and co-workers. The final cleavage of the fluorous tags in this strategy was generally relied on alcoholysis,21f olefin metathesis,18b,19e oxidative cleavage,20a,b reductive hydrogenation22a,b and so on, and consequently only allowed single directional assembly from the reducing end to the non-reducing end and very few of the cleaved fluorous tags were reported to be recovered and recycled.20a,21f,22a
We have recently developed a solution-phase oligosaccharide synthesis strategy based on interrupted Pummerer reaction mediated (IPRm) glycosylation.23 In this strategy, we comprehensively applicated latent O-2-(2-propylthiol)-benzyl (OPTB) glycosides and its active counterpart O-2-[(propan-2-yl)sulfinyl]benzyl (OPSB) glycosides as glycosyl donors in glycosylation. It enables efficient and rapid assembly of complex oligosaccharides and also allows the recovery and regeneration of the anomeric leaving group (Fig. 1B).24–27 In view of these advantages, we envisioned that the installation of fluorous side chains on anomeric OPTB and OPSB functionalities (OPTBF and OPSBF respectively) would further facilitate the reducing end to non-reducing end assembly sequence by virtue of the PTFE assisted filtration technology. Most importantly, the fluorous tagged anomeric leaving group would be amenable to be cleaved and recovered by IPRm glycosylation and thus allow the non-reducing end to reducing end assembly and overall, provide a two-directional oligosaccharide synthesis (Fig. 1C).
Results and discussion
Our study commenced on the design of a fluorous tagged anomeric leaving group by installing appropriate fluorous chains on the anomeric PTB group at appropriate positions. Inspired by Chai's work,22b we intended to directly fix two perfluoroalkane chains on the terminal position of PTB-OH by ether linkages aimed at simplifying the synthesis and minimizing the impact of the fluorous chains on the glycosylation efficiency (Scheme 1). In addition, the two perfluoroalkane chains would maintain the high affinity of the extended sugar chains to the PTFE particles. To this purpose, known alcohol 1 bearing two perfluoroalkyl ether chains was selected as the starting material.22a The tosylation of alcohol 1 followed by a SN2 displacement with methyl thiosalicylate under basic conditions furnished 3 in good yield. The subsequent reduction of the ester group of 3 produced PTBF-OH 4 in 97% yield. It's worth noting that all the purification steps in this synthetic procedure were performed by PTFE assisted filtration, that is the adsorption of the reaction residues on PTFE powder (200 μm particle size) in a solvent mixture of acetone/H2O (6
:
4, v/v), followed by filtration with a sand core funnel and successive washing with acetone/H2O and dichloromethane (Scheme 1). Each purification could be finished within half an hour and the synthesis was easy to scale up to 5 grams in one batch. This newly designed fluorous-tagged PTBF-OH has great stability and is amenable to be stored on shelf at least for 10 months without detectable decomposition.
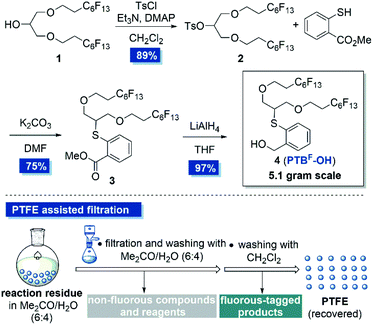 |
| Scheme 1 Synthesis of fluorous-tagged PTBF-OH via PTFE assisted filtration. | |
With fluorous-tagged PTBF-OH in hand, we embarked on proving the concept (Scheme 2). The attachment of the fluorous tag on carbohydrate was achieved by coupling of 4 with glucosyl trichloroacetimidate donor 5 in the presence of a catalytic amount of TMSOTf at −20 °C. The standard PTFE assisted filtration procedure delivered OPTBF glycoside 6 in 90% yield. Further oxidation of 6 by [bis(trifluoroacetoxy)iodo]benzene (PIFA) provided the OPSBF glycosyl donor 7 in 93% yield. Subsequently, the possibility of the cleavage of the fluorous-tagged anomeric leaving group by the IPRm glycosylation reaction was examined. Subjecting OPSBF donor 7 and acceptor 8 to a standard IPRm glycosylation procedure23 afforded the desired disaccharide 9 in 91% yield. In the meantime, the fluorous-tag derivative 10 (PSBF-OH) was isolated in 87% yield. Moreover, PSBF-OH was found to be easily reduced to PTBF-OH (4) in the presence of thiosalicylic acid (TSA) and a catalytic amount of iodine. This regenerated PTBF-OH was able to be installed as the latent leaving group again and thus was almost completely recycled. The results indicated that the attached fluorous tag is highly conducive to the IPRm glycosylation reaction and our design of the recyclable fluorous tag assisted two-directional oligosaccharide synthesis is conceptually realized.
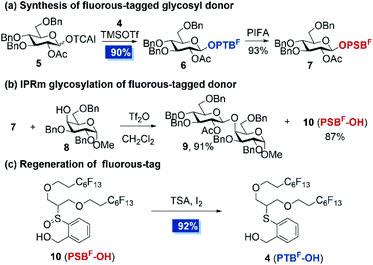 |
| Scheme 2 Proof of concept. | |
Encouraged by these results, we synthesized a series of OPTBF acceptors 12 (for details, see the ESI†) and examined their orthogonality to various classical glycosylation methods (Table 1). To our delight, the fluorous-tagged acceptors glycosylated efficiently with various types of glycosyl donors under the corresponding activation conditions, and all the reactions are highly conducive to the practical PTFE assisted filtration purification procedure. The fluorous tag was proved to be stable under acidic (13a–13d) and transition metal-catalyzed (13e) conditions, which are frequently used to activate the trichloroacetimidate glycosyl donor (5),28 OPSB/SPSB glycoside donors (11a/11b),23,29–31n-pentenyl glycosyl donor (11d),32,33 thioglycoside donor (11c)34 and o-alkynylbenzoate (Abz) glycosyl donor (11e).35,36 The high glycosylation efficiency of these reactions implied the limited restrictions of the method in the assembly of complex oligosaccharides from the reducing end to the non-reducing end, because many nice glycosylation methods are amenable to be selected to address particular coupling problems that may be encountered in the assembly sequence. In addition, the OPTBF moiety at the reducing end was able to be transformed to the active leaving group for further elongation via IPRm glycosylation. These flexibilities as well as the ease of purification processes highlighted its great potential in the oligosaccharide synthesis.
Table 1 Orthogonal glycosylations of fluorous-tagged glycosyl acceptors
Subsequently, the scope and limitations on the cleavage of the fluorous tag by IPRm glycosylation and meanwhile the elongation of the sugar chains at the reducing end were examined (Scheme 3). Firstly, various glycosides 13 possessing terminal OPTBF groups were oxidized to active OPSBF glycosides 14 by PIFA in aqueous CH3CN. These oxidation reactions proceeded smoothly and most importantly their purification by simple aqueous workup followed by extraction delivered the OPSBF glycosides in good quality, which were pure enough for next IPRm glycosylations in most cases (for details, see the ESI†).
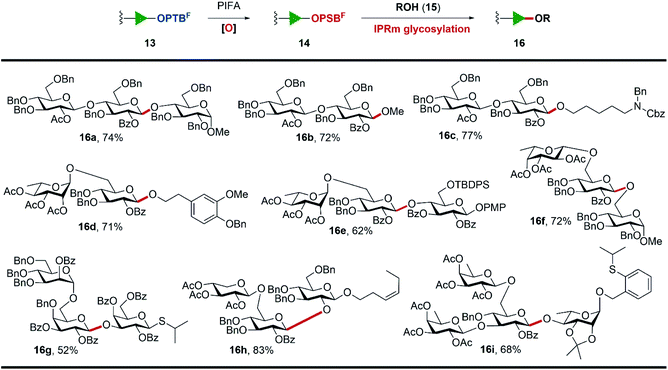 |
| Scheme 3 Synthesis of OPSBF glycosyl donors and their IPRm glycosylations. General conditions for oxidation: OPTBF glycosides 13 (1.0 equiv.), PIFA (1.2 equiv.), MeCN/H2O (9 : 1, v/v), and rt. General conditions for IPRm glycosylation: OPSBF glycosides 14 (1.0 equiv.), Tf2O (1.0 equiv.), acceptor 15 (1.2 equiv.), CH2Cl2, and −40 °C. Yield means isolated yield for two steps. | |
A variety of acceptors including simple alcohols such as MeOH, N-benzyl-N-benzyloxycarbonyl-5-aminopentanol, 3-methoxyl-4-O-benzyl phenylethanol and diversified carbohydrate acceptors were found to be effective nucleophiles in this fluorous assisted IPRm glycosylation. The standard IPRm glycosylation reaction conditions delivered glycosidic products 16a–16i in good to excellent overall yields. Among these examples, it is worth noting that: (1) p-methoxyphenyl group (16e), cis-alkene (16h), and other acid-sensitive groups such as tert-butyldiphenylsilyl group (16e) and acetonide (16i) were tolerated well under current conditions. (2) The thioglycoside acceptor (16g) and OPTB acceptor (16i) remained stable in glycosylation reactions and would be beneficial to further orthogonal glycosylations. (3) Disaccharide 16d and trisaccharide 16h were the protected forms of dictamphenosides E and (Z) 3-hexenol glycosides, which were recently isolated from Cortex Dictamni37 and Physalis alkekengi var. franchetii,38 respectively. Their good glycosylation output laid the basis for more complicated oligosaccharide assembly through the established PTFE assisted two-directional synthetic protocol.
As a final endeavor to demonstrate the utilization potential of the protocol, structurally more complex S. pneumonia type 14 (ST14) tetrasaccharide and octasaccharide were chosen as synthetic targets (Scheme 4).39,40 The tetrasaccharide repeating unit is the smallest structure to induce ST14-specific antibody effects41,42 and represents an important component for the development of synthetic or semi-synthetic pneumococcal vaccines.43 Savage44 and Seeberger42,45 have developed glycoconjugate vaccine candidates, which are advantageous to clinically used vaccines on the basis of solution-phase synthesized tetrasaccharide fragments. Besides, Salwiczak also has synthesized ST14 tetrasaccharide and octasaccharide antigens by a solid-phase method.41 We believe that the present two-directional assembly strategy has great potential to provide fast access to ST14 oligosaccharides and would be beneficial to vaccine development. With PTBF-OH, thioglycosides 17a and 17b, and OPSB glycosides 18a and 18b in hand, fluorous-tag assisted two-directional assembly was conducted. PTBF-OH was firstly glycosylated to building block 17a and provided OPTBF monosaccharide 19. Further removal of the 4-O-Fmoc protecting group, installation of galactoside 18avia IPRm glycosylation and removal of the 6-O-levulinyl group provided the disaccharide OPTBF acceptor. The subsequent introduction of glucosyl building block 17b, removal of the 4-O-Fmoc protecting group and installation of galactosyl building block 18b gave OPTBF tetrasaccharide 20 in 48% overall yield in seven steps. It's worth mentioning that tetrasaccharide 20 was obtained without column chromatography with the aid of PTFE assisted filtration. The existence of a levulinyl group at the non-reducing end allows the further elongation of the repeating polysaccharide chain. Additionally, the OPTBF moiety at the reducing end permits the elongation at the reducing end by IPRm glycosylation.
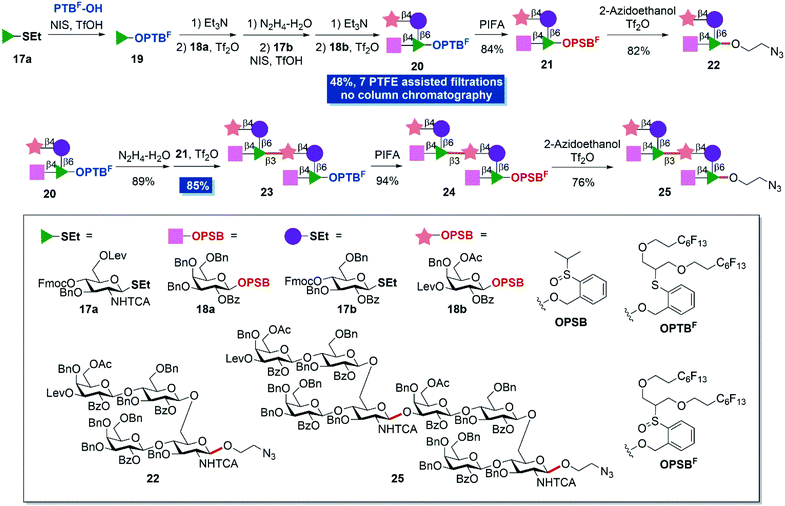 |
| Scheme 4 Fluorous-tag assisted two-directional syntheses of ST14 repeating units. | |
Simple oxidization of OPTBF tetrasaccharide 20 and coupling with 2-azidoethanol successfully provided the minimum repeating unit tetrasaccharide 22 in 82% yield with the introduction of a biological compatible tail for further elaboration in biological studies. Meanwhile the anomeric fluorous tag was cleaved. The treatment of tetrasaccharide 20 with hydrazinium hydroxide solution to remove the levulinyl group furnished the tetrasaccharide OPTBF acceptor. Next, the dimerization reaction between tetrasaccharide donor 21 (2.0 equiv.) and tetrasaccharide acceptor under standard IPRm glycosylation conditions performed very well and provided octasaccharide 23 in 85% yield with PTFE assisted filtration. Finally, the oxidation and elaboration of 2-azidoethanol accomplished the synthesis of octasaccharide dimer 25 in overall eleven steps and 26% yield from mono-saccharide building blocks. Eight PTFE assisted filtrations were adopted in the whole synthesis that largely minimized the column chromatography separation steps and simplified the purification processes.
Conclusions
In conclusion, we developed a fluorous-tag assisted two-directional oligosaccharide assembly strategy. The strategy relied on our recently developed IPRm glycosylation by designing a fluorous tagged OPTB anomeric leaving group. The upgraded IPRm glycosylation allowed flexible, rapid and efficient oligosaccharide assembly from the reducing end to the non-reducing end with the aid of PTFE assisted filtration purification technology. Upon the completion of the reducing end to non-reducing end assembly, the terminal OPTBF was able to be oxidized to the OPSBF group, which could be cleaved and recycled by another IPRm glycosylation and at the same time elongated the oligosaccharide chain at the reducing end. The efficacy was exemplified by fast access to the ST14 tetrasaccharide and octasaccharide fragments. Additionally, encouraged by recent remarkable achievements in automated fluorous-assisted solution-phase oligosaccharide synthesis46 and HPLC-based automated synthesis of glycans in solution,47 we expect to employ the developed fluorous-tag assisted synthetic strategy in an automated platform and we are currently studying on this issue.
Data availability
Experimental data including experimental procedures, characterization data, and NMR spectra for the new compounds have been uploaded as ESI material.†
Author contributions
Lei Cai and Qi Chen carried out most of the experiments, brought the project to completion and prepared the draft. Jian Guo and Zhihua Liang synthesized PTBF-OH and some fluorous-tagged glycosyl acceptors. Dengxian Fu participated in the revision of the draft. Lingkui Meng contributed to the discussion of the project and revision of the draft. Qian Wan conceived the idea. Jing Zeng and Qian Wan oversaw the project, discussed the results, and edited and reorganized the manuscript.
Conflicts of interest
There are no conflicts to declare.
Acknowledgements
This work was supported by the National Natural Science Foundation of China (22025102, 21877043, 21672077, 22077039, and 21761132014) and the State Key Laboratory of Bio-organic and Natural Products Chemistry (SKLBNPC13425). We appreciated the Analytical and Testing Centers and Medical Subcenter of Huazhong University of Science and Technology and School of Pharmacy for NMR and HRMS tests.
Notes and references
- R. Stern and M. J. Jedrzejas, Chem. Rev., 2008, 108, 5061–5085 CrossRef CAS PubMed.
- For recent reviews on glycosylation strategies:
(a) D. Crich, J. Am. Chem. Soc., 2021, 143, 17–34 CrossRef CAS PubMed;
(b) S. S. Shivatare and C.-H. Wong, J. Org. Chem., 2020, 85, 15780–15800 CrossRef CAS PubMed;
(c) C.-W. Cheng, C.-Y. Wu, W.-L. Hsu and C.-H. Wong, Biochemistry, 2020, 59, 3078–3088 CrossRef CAS PubMed;
(d) B.-H. Li and X.-S. Ye, Curr. Opin. Chem. Biol., 2020, 58, 20–27 CrossRef CAS PubMed;
(e) V. Dimakos and M. S. Taylor, Chem. Rev., 2018, 118, 11457–11517 CrossRef CAS PubMed;
(f) M. M. Nielsen and C. M. Pedersen, Chem. Rev., 2018, 118, 8285–8358 CrossRef CAS PubMed;
(g) L. Wen, G. Edmunds, C. Gibbons, J. Zhang, M. R. Gadi, H. Zhu, J. Fang, X. Liu, Y. Kong and P. G. Wang, Chem. Rev., 2018, 118, 8151–8187 CrossRef CAS PubMed;
(h) M. Panza, S. G. Pistorio, K. J. Stine and A. V. Demchenko, Chem. Rev., 2018, 118, 8105–8150 CrossRef CAS PubMed;
(i) X. Li and J. Zhu, Eur. J. Org. Chem., 2016, 4724–4767 CrossRef CAS;
(j) X. Zhu and R. R. Schmidt, Angew. Chem., Int. Ed., 2009, 48, 1900–1934 CrossRef CAS PubMed.
- Y. Wu, D.-C. Xiong, S.-C. Chen, Y.-S. Wang and X.-S. Ye, Nat. Commun., 2017, 8, 14851 CrossRef CAS PubMed.
- Q. Zhu, Z. Shen, F. Chiodo, S. Nicolardi, A. Molinaro, A. Silipo and B. Yu, Nat. Commun., 2020, 11, 4142 CrossRef CAS PubMed.
- A. A. Joseph, A. Pardo-Vargas and P. H. Seeberger, J. Am. Chem. Soc., 2020, 142, 8561–8564 CrossRef CAS PubMed.
- P. H. Seeberger, Acc. Chem. Res., 2015, 48, 1450–1463 CrossRef CAS PubMed.
- M. Guberman and P. H. Seeberger, J. Am. Chem. Soc., 2019, 141, 5581–5592 CrossRef CAS PubMed.
-
A. M. Downey and P. H. Seeberger, in Comprehensive Glycoscience, ed. J. J. Barchi, 2nd edn, Elsevier B.V., Oxford, 2021, pp. 561–601 Search PubMed.
- D.-W. Zhu, Synthesis, 1993, 953–954 CrossRef CAS.
- I. T. Horváth and J. Rábai, Science, 1994, 266, 72–75 CrossRef PubMed.
-
(a) D. P. Curran and S. Hadida, J. Am. Chem. Soc., 1996, 118, 2531–2532 CrossRef CAS;
(b) A. Studer, S. Hadida, R. Ferritto, S.-Y. Kim, P. Jeger, P. Wipf and D. P. Curran, Science, 1997, 275, 823–826 CrossRef CAS PubMed;
(c) D. P. Curran, Pure Appl. Chem., 2000, 72, 1649–1653 CrossRef CAS;
(d) D. P. Curran and Z. Lee, Green Chem., 2001, 3, G3–G7 RSC;
(e) Z. Luo, Q. Zhang, Y. Oderaotoshi and D. P. Curran, Science, 2001, 291, 1766–1769 CrossRef CAS PubMed.
- W. Zhang, Chem. Rev., 2009, 109, 749–795 CrossRef CAS PubMed.
- K. Goto and M. Mizuno, Trends Glycosci. Glycotechnol., 2013, 25, 203–213 CrossRef CAS.
- D. P. Curran, S. Hadida and M. He, J. Org. Chem., 1997, 62, 6714–6715 CrossRef CAS.
- D. P. Curran, S. Hadida and S.-Y. Kim, Tetrahedron, 1999, 55, 8997–9006 CrossRef CAS.
- W. Zhang and D. P. Curran, Tetrahedron, 2006, 62, 11837–11865 CrossRef CAS PubMed.
- D. P. Curran, R. Ferritto and Y. Hua, Tetrahedron Lett., 1998, 39, 4937–4940 CrossRef CAS.
-
(a) E. R. Palmacci, M. C. Hewitt and P. H. Seeberger, Angew. Chem., Int. Ed., 2001, 40, 4433–4437 CrossRef CAS;
(b) F. R. Carrel, K. Geyer, J. D. C. Codée and P. H. Seeberger, Org. Lett., 2007, 9, 2285–2288 CrossRef CAS PubMed.
-
(a) K.-S. Ko, F. A. Jaipuri and N. L. Pohl, J. Am. Chem. Soc., 2005, 127, 13162–13163 CrossRef CAS PubMed;
(b) S. K. Mamidyala, K.-S. Ko, F. A. Jaipuri, G. Park and N. L. Pohl, J. Fluor. Chem., 2006, 127, 571–579 CrossRef CAS;
(c) F. A. Jaipuri and N. L. Pohl, Org. Biomol. Chem., 2008, 6, 2686–2691 RSC;
(d) L. Liu and N. L. B. Pohl, Org. Lett., 2011, 13, 1824–1827 CrossRef CAS PubMed;
(e) S.-L. Tang and N. L. B. Pohl, Org. Lett., 2015, 17, 2642–2645 CrossRef CAS PubMed;
(f) M. K. Kern and N. L. B. Pohl, Org. Lett., 2020, 22, 4156–4159 CrossRef CAS PubMed.
-
(a) K. Goto and M. Mizuno, Tetrahedron Lett., 2007, 48, 5605–5608 CrossRef CAS;
(b) M. Mizuno, S. Kitazawa and K. Goto, J. Fluor. Chem., 2008, 129, 955–960 CrossRef CAS;
(c) K. Goto and M. Mizuno, Tetrahedron Lett., 2010, 51, 6539–6541 CrossRef CAS;
(d) K. Fukuda, M. Tojino, K. Goto, H. Dohi, Y. Nishida and M. Mizuno, Carbohydr. Res., 2015, 407, 122–130 CrossRef CAS PubMed.
-
(a) C. Zong, A. Venot, O. Dhamale and G.-J. Boons, Org. Lett., 2013, 15, 342–345 CrossRef CAS PubMed;
(b) W. Huang, Q. Gao and G.-J. Boons, Chem.–Eur. J., 2015, 21, 12920–12926 CrossRef CAS PubMed;
(c) C. Cai, D. M. Dickinson, L. Li, S. Masuko, M. Suflita, V. Schultz, S. D. Nelson, U. Bhaskar, J. Liu and R. J. Linhardt, Org. Lett., 2014, 16, 2240–2243 CrossRef CAS PubMed;
(d) B. Yang, Y. Jing and X. Huang, Eur. J. Org. Chem., 2010, 1290–1298 CrossRef CAS PubMed;
(e) T. Miura, Y. Hirose, M. Ohmae and T. Inazu, Org. Lett., 2001, 3, 3947–3950 CrossRef CAS PubMed;
(f) T. Miura, K. Goto, D. Hosaka and T. Inazu, Angew. Chem., Int. Ed., 2003, 42, 2047–2051 CrossRef CAS PubMed;
(g) H. Tanaka, Y. Tanimoto, T. Kawai and T. Takahashi, Tetrahedron, 2011, 67, 10011–10016 CrossRef CAS;
(h) L. Manzoni, Chem. Commun., 2003, 2930–2931 RSC;
(i) L. Manzoni and R. Castelli, Org. Lett., 2006, 8, 955–957 CrossRef CAS PubMed;
(j) K. Ikeda, H. Mori and M. Sato, Chem. Commun., 2006, 3093–3094 RSC;
(k) M. Kojima, Y. Nakamura and S. Takeuchi, Tetrahedron Lett., 2007, 48, 4431–4436 CrossRef CAS;
(l) G. Macchione, J. L. de Paz and P. M. Nieto, Carbohydr. Res., 2014, 394, 17–25 CrossRef CAS PubMed;
(m) S. Maza, N. Gandia-Aguado, J. L. de Paz and P. M. Nieto, Bioorg. Med. Chem., 2018, 26, 1076–1085 CrossRef CAS PubMed;
(n) J. L. de Paz and P. M. Nieto, Methods Mol. Biol., 2022, 2303, 37–47 CrossRef PubMed.
-
(a) Y.-H. Chai, Y.-L. Feng, J.-J. Wu, C.-Q. Deng, A.-Y. Liu and Q. Zhang, Chin. Chem. Lett., 2017, 28, 1693–1700 CrossRef CAS;
(b) Y. Feng, J. Wu, G. Chen and Y. Chai, Org. Lett., 2020, 22, 2564–2568 CrossRef CAS PubMed.
-
(a) P. Shu, X. Xiao, Y. Zhao, Y. Xu, W. Yao, J. Tao, H. Wang, G. Yao, Z. Lu, J. Zeng and Q. Wan, Angew. Chem., Int. Ed., 2015, 54, 14432–14436 CrossRef CAS PubMed;
(b) P. Shu, W. Yao, X. Xiao, J. Sun, X. Zhao, Y. Zhao, Y. Xu, J. Tao, G. Yao, J. Zeng and Q. Wan, Org. Chem. Front., 2016, 3, 177–183 RSC;
(c) L. Cai, J. Zeng, T. Li, Y. Xiao, X. Ma, X. Xiao, Q. Zhang, L. Meng and Q. Wan, Chin. J. Chem., 2020, 38, 43–49 CrossRef CAS.
- W. Chen, J. Zeng, H. Wang, X. Xiao, L. Meng and Q. Wan, Carbohydr. Res., 2017, 452, 1–5 CrossRef CAS PubMed.
- J. Fang, J. Zeng, J. Sun, S. Zhang, X. Xiao, Z. Lu, L. Meng and Q. Wan, Org. Lett., 2019, 21, 6213–6216 CrossRef CAS PubMed.
- L. Meng, J. Zeng and Q. Wan, Synlett, 2018, 29, 148–156 CrossRef CAS.
- X. Zhao, J. Zeng, L. Meng and Q. Wan, Chem. Rec., 2020, 20, 743–751 CrossRef CAS PubMed.
- R. R. Schmidt and W. Kinzy, Adv. Carbohydr. Chem. Biochem., 1994, 50, 21–123 CrossRef CAS PubMed.
- X. Xiao, Y. Zhao, P. Shu, X. Zhao, Y. Liu, J. Sun, Q. Zhang, J. Zeng and Q. Wan, J. Am. Chem. Soc., 2016, 138, 13402–13407 CrossRef CAS PubMed.
- Y. Zhao, J. Zeng, Y. Liu, X. Xiao, G. Sun, J. Sun, P. Shu, D. Fu, L. Meng and Q. Wan, J. Carbohydr. Chem., 2018, 37, 471–497 CrossRef CAS.
- J. Sun, J. Fang, X. Xiao, L. Cai, X. Zhao, J. Zeng and Q. Wan, Org. Biomol. Chem., 2020, 18, 3818–3822 RSC.
- J. R. Merritt and B. Fraser-Reid, J. Am. Chem. Soc., 1992, 114, 8334–8336 CrossRef CAS.
- D. R. Mootoo, P. Konradsson, U. Udodong and B. Fraser-Reid, J. Am. Chem. Soc., 1988, 110, 5583–5584 CrossRef CAS.
- L. Meng, P. Wu, J. Fang, Y. Xiao, X. Xiao, G. Tu, X. Ma, S. Teng, J. Zeng and Q. Wan, J. Am. Chem. Soc., 2019, 141, 11775–11780 CrossRef CAS PubMed.
- Y. Li, Y. Yang and B. Yu, Tetrahedron Lett., 2008, 49, 3604–3608 CrossRef CAS.
- B. Yu, Acc. Chem. Res., 2018, 51, 507–516 CrossRef CAS PubMed.
- S. Yang, F. Sun, J. Ruan, J. Yan, P. Huang, J. Wang, L. Han, Y. Zhang and T. Wang, Fitoterapia, 2019, 134, 465–473 CrossRef CAS PubMed.
- Z. Shu, Y. Tang, Y. Yang, Z. Ding, R. Zhong, T. Xia, X. Li, C. Zheng, Z. Wen, W. Li and Y. Wang, Nat. Prod. Res., 2021, 35, 1274–1280 CrossRef CAS PubMed.
- A. Sundgren, M. Lahmann and S. Oscarson, J. Carbohydr. Chem., 2005, 24, 379–391 CrossRef CAS.
- E. V. Sukhova, D. V. Yashunsky, Y. E. Tsvetkov, E. A. Kurbatova and N. E. Nifantiev, Russ. Chem. Bull., 2014, 63, 511–521 CrossRef CAS.
- J. Louçano, P. Both, A. Marchesi, L. del Bino, R. Adamo, S. Flitsch and M. Salwiczek, RSC Adv., 2020, 10, 23668–23674 RSC.
- B. M. S. Seco, F.-F. Xu, A. Grafmüller, N. Kottari, C. L. Pereira and P. H. Seeberger, ACS Chem. Biol., 2020, 15, 2395–2405 CrossRef CAS PubMed.
- E. A. Kurbatova, N. K. Akhmatova, E. A. Akhmatova, N. B. Egorova, N. E. Yastrebova, E. V. Sukhova, D. V. Yashunsky, Y. E. Tsvetkov, M. L. Gening and N. E. Nifantiev, Front. Immunol., 2017, 8, 659 CrossRef PubMed.
- S. Deng, L. Bai, R. Reboulet, R. Matthew, D. A. Engler, L. Teyton, A. Bendelac and P. B. Savage, Chem. Sci., 2014, 5, 1437–1441 RSC.
- P. Kaplonek, N. Khan, K. Reppe, B. Schumann, M. Emmadi, M. P. Lisboa, F.-F. Xu, A. D. J. Calow, S. G. Parameswarappa, M. Witzenrath, C. L. Pereira and P. H. Seeberger, Proc. Natl. Acad. Sci. U. S. A., 2018, 115, 13353–13358 CrossRef CAS PubMed.
- R. C. Saliba, Z. J. Wooke, G. A. Nieves, A. A. Chu, C. S. Bennett and N. L. B. Pohl, Org. Lett., 2018, 20, 800–803 CrossRef CAS PubMed.
- S. Escopy, Y. Singh, K. J. Stine and A. V. Demchenko, Chem.–Eur. J., 2022, e202201180 Search PubMed.
|
This journal is © The Royal Society of Chemistry 2022 |
Click here to see how this site uses Cookies. View our privacy policy here.