DOI:
10.1039/D2CE01355J
(Paper)
CrystEngComm, 2023,
25, 86-94
Crystal structures of 5-bromo-1-arylpyrazoles and their halogen bonding features†
Received
29th September 2022
, Accepted 22nd November 2022
First published on 23rd November 2022
Abstract
Single crystal X-ray diffraction analysis was employed to investigate the supramolecular properties of five 5-brominated pyrazoles in order to evaluate the role of the Br atom in the formation of halogen bonding duly recognizing that the Br atom is less polarizable than the iodine atom. Hirshfeld analysis was employed to conceive a more descriptive image of the halogen bond propensity of 5-bromopyrazoles. The compounds display Br⋯O contacts or Br⋯Br as type I or type II halogen contacts. The structural analyses revealed the isostructurality of compounds 2 and 3 while compound 5 was found to be isostructural with its iodo-analogue, suggesting the importance of the halogen contacts even if they are considered weak in nature.
Introduction
The halogen bond1–3 is currently extensively studied either in the solid state4–9 or in solution.10–15 The interest in halogen bond formation was raised due to its interesting applications or properties in directing supramolecular assemblies,16,17 in catalysis18,19 or in biochemical systems to explore drug-target interactions and in rational drug design.20–24
Using the concept of the σ-hole25–29 two main classes of halogen or inter-halogen contacts can be defined: halogen bonds established between halogen atoms and electron rich entities other than halogen atoms, and halogen bonds in which halogen atoms play both the role of donor and bond acceptor, these interactions being subdivided into three types30–32 which are presented in Fig. 1. Due to its high polarizability the iodine atom presents the highest magnitude of the σ-hole, which was defined as the positive potential surface on the end cap of the halogen atom along the bond axis.26 Thus, different classes of iodinated compounds became benchmarks in the study of halogen bonding.33–37 We also previously reported the halogen bonding properties in 5-iodopyrazoles which we found to be favorable substrates for investigating such interactions.38–40
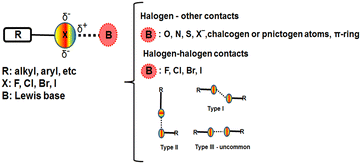 |
| Fig. 1 The halogen bond. “B” represents an electronegative moiety such as a pi-system, molecular entities containing electronegative atoms such as O, N, etc., or another halogen atom playing the role of halogen bond acceptor. These latter cases are classified by their directionality and the type of forces involved as type I (dispersive in nature interhalogen-contact), type II (electrostatic in nature, halogen bond) and an unusual type III inter-halogen contact more recently explored. | |
Proceeding further in attempting to understand halogen bonding, our attention was drawn to 5-bromopyrazoles, as we observed that crystal structures containing a pyrazole brominated at the 5 position are rather scarce (with only 17 hits in the Cambridge Structural Database (CSD))41–53 and usually represented by transition metal complexes. Another reason for pursuing these compounds is that from our previous studies we observed that even when the Br atom is not attached at the 5 position of the pyrazole, it is prone to halogen bonding.38–40 Thus, it was of interest to us to gauge what the influence of Br attached to the 5 position of the pyrazole ring on the supramolecular crystalline networks of 1-aryl-5-bromopyrazoles might be.
Herein we present the X-ray crystal structures of five 5-brominated pyrazoles and evaluate the role of the bromine atom as participant in halogen bonding, among the other potential supramolecular interactions such as hydrogen bonding and π-stacking.
Materials and methods
The best method for the synthesis of 5-bromopyrazoles is the 1,3-dipolar cycloaddition of 4-bromosydnones with acetylenic dipolarophiles. The synthesis and structural characterization of the compounds 1–5 were described by us previously.38–40,54,55
Crystals of compounds 1 and 2 were grown from ethanol–methylene chloride 1
:
1 mixture by slow evaporation and crystals for compounds 3, 4 and 5 were obtained similarly from acetonitrile as solvent.
X-ray diffraction
Single-crystal X-ray diffraction data were collected on an Oxford-Diffraction XCALIBUR EOS CCD diffractometer with graphite-monochromated Mo-Kα radiation. The unit cell determination and data integration were carried out using the CrysAlisPro package from Oxford Diffraction.56 Multi-scan correction for absorption was applied. The structures were solved with program ShelXT using the intrinsic phasing method and refined by the full-matrix least-squares method on F2 with ShelXL.57,58 Crystal 2 was refined as a non-merohedral twin and the twin component ratio was estimated at 0.85/0.15. Olex2 was used as an interface to the ShelX programs.59 Non-hydrogen atoms were refined anisotropically. Aromatic hydrogen atoms were added in idealized positions and refined using a riding model. Detailed crystallographic data for 1–5 are provided in Table 1. Specific details of each refinement are given in the crystallographic information files (CIF-files).
Table 1 Crystallographic parameters of compounds 1–5
Compound |
1
|
2
|
3
|
4
|
5
|
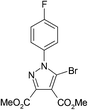
|

|

|

|
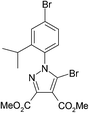
|
CCDC no. |
2156676
|
2156677
|
2156678
|
2156679
|
2156680
|
Emp. formula |
C13H10BrFN2O4 |
C14H12BrClN2O4 |
C15H15BrN2O4 |
C16H17BrN2O4 |
C16H16Br2N2O4 |
F
w
|
357.14 |
387.62 |
367.20 |
381.22 |
460.13 |
T [K] |
293 |
297 |
293 |
294 |
250 |
Space group |
P21/c |
P21/n |
P21/n |
P21/c |
Pbca
|
a [Å] |
11.2936(6) |
5.3058(5) |
5.6908(2) |
11.1782(9) |
14.0434(8) |
b [Å] |
4.4341(2) |
10.0452(9) |
10.0921(4) |
10.3856(6) |
12.0064(8) |
c [Å] |
27.9340(14) |
28.782(2) |
27.4423(9) |
15.2063(16) |
21.0629(13) |
α [°] |
90 |
90 |
90 |
90 |
90 |
β [°] |
93.314(5) |
91.144(7) |
84.574(3) |
107.016(9) |
90 |
γ [°] |
90 |
90 |
90 |
90 |
90 |
V [Å3] |
1396.50(12) |
1533.7(2) |
1569.00(10) |
1688.1(3) |
3551.4(4) |
Z
|
4 |
4 |
4 |
4 |
8 |
ρ
calcd [g cm−3] |
1.699 |
1.679 |
1.554 |
1.500 |
1.721 |
μ [mm−1] |
2.972 |
2.873 |
2.639 |
2.456 |
4.587 |
Crystal size [mm] |
0.20 × 0.10 × 0.10 |
0.30 × 0.20 × 0.20 |
0.25 × 0.10 × 0.08 |
0.25 × 0.20 × 0.15 |
0.18 × 0.18 × 0.15 |
2θ range |
3.612 to 52.744 |
4.294 to 50.052 |
4.302 to 50.048 |
3.81 to 50.054 |
3.868 to 50.048 |
Refls. collected |
8561 |
2682 |
12 050 |
9999 |
11 205 |
Indep. refls |
2864 [Rint = 0.0394] |
2682 [Rint = 0.094] |
2767 [Rint = 0.0364] |
2984 [Rint = 0.0501] |
3133 [Rint = 0.0635] |
Data/rests./params. |
2864/0/192 |
2682/0/203 |
2767/0/203 |
2984/0/213 |
3133/0/221 |
GOF |
1.058 |
1.078 |
1.058 |
1.043 |
1.019 |
R
1
|
0.0488, |
0.0964 |
0.0377 |
0.0467 |
0.0483 |
wR2 |
0.0923 |
0.2253 |
0.0560 |
0.0773, |
0.0596 |
Hirshfeld analysis was employed as implemented in the program CrystalExplorer21.60,61
All the non-covalent contacts were referenced to the van der Waals distances listed by Bondi.62
Results and discussion
A quick search in the CSD revealed 17 hits41–53 for 5-bromopyrazole structures, 14 of them presenting short halogen contacts involving 5-Br-pyrazole. These 14 structures are discussed in 10 articles and to date, the remaining structures49,52,53 appear in the CSD as communications only, without associated articles. However, of the 10 published papers only three make clear reference to the presence of halogen bonding involving the 5-Br atom without any further study.41,47,48
As we had previously investigated the halogen bond propensity in 5-iodinated pyrazoles, we hypothesized that 5-bromopyrazoles should possess similar features, despite the relatively smaller magnitude of the σ-hole of the Br atom compared with that of the I atom. Having this in mind we have synthesized and obtained single crystals of five 5-bromopyrazoles which displayed good diffraction quality. These are presented in Table 1 below, together with their data-collection and refinement parameters.
X-ray diffraction analysis
The crystal structures as well as the chemical compositions of the compounds 1–5 have been elucidated by the single crystal X-ray diffraction method. The results of this investigation are depicted in Fig. 2, while the bond distances and angles for each molecule are summarized in Table S1.† According to X-ray crystallography, in the crystal structures of all the compounds the asymmetric unit (ASU) comprises one neutral molecule. There are no co-crystallized solvent molecules in any of the crystals.
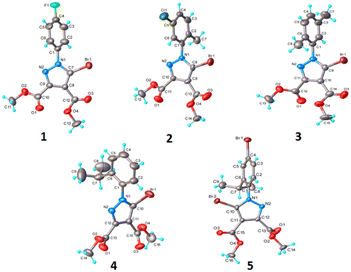 |
| Fig. 2 Single crystal X-ray molecular structures with atom labelling and thermal ellipsoids drawn at the 50% probability level. | |
The analysis of the crystal packing of 1 has revealed the presence of numerous intermolecular hydrogen and halogen bonds that ensure the interconnection of each asymmetric unit with four adjacent molecules, as shown in Fig. 3. On the whole, the main crystal structure motif for 1 is characterized as a 3D supramolecular network. A careful inspection of this structure reveals trifurcated halogen bonding, which can best be appreciated when viewed as a stereoscopic figure (Fig. S1†) which shows just two pairs of molecules of 1 which are related by translation along the crystal b-axis (somewhat offset from the view direction for clarity). It is evident that three interactions emanate from each of the equivalent Br atoms. This trifurcated halogen bond consists of two Br⋯Br contacts of 3.622 Å which are symmetry-related and a Br⋯O short contact of 3.055 Å (Fig. S1†). This infinite pattern created by the halogen contacts is interconnected via C–H⋯O hydrogen bonding, which extends the aggregation to 3D architecture.
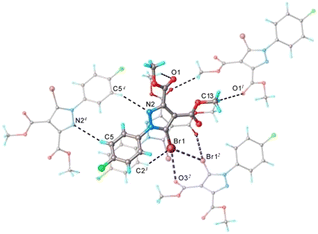 |
| Fig. 3 Intermolecular interactions in the crystal structure of 1. Symmetry generated molecules are shown with faded colors. Hydrogen and halogen bonds are shown as dotted lines with black and blue color, respectively. H-Bond parameters: C2–H⋯Br1 [C2–H 0.93 Å, H⋯Br1 (1 + x, 1 + y, z) 3.07 Å, C2⋯Br1 3.798(4) Å, ∠C2HBr1 136.2°]; C5–H⋯N2 [C5–H 0.93 Å, H⋯N2 (1 − x, 1 − y, 1 − z) 2.81 Å, C5⋯N2 3.600(5) Å, ∠C5HN2 143.2°; C11–H⋯O1 [C11–H 0.96 Å, H⋯O1 (1 + x, 1 + y, z) 2.62 Å, C11⋯O1 3.264(6) Å, ∠C11HO1 134.6°; C13–H⋯O1 [C13–H 0.96 Å, H⋯O1 (2 − x, y − 0.5, 1.5 − z) 2.61 Å, C13⋯O1 3.406(5) Å, ∠C13HO1 141.0°. Halogen bond parameters: [C7–Br1⋯O3] C7–Br1 1.843(4) Å, Br1⋯O3 (1 − x, 0.5 + y, 1.5 − z) 3.055(3) Å, ∠C7Br1O3 170.9(1)°; [C7–Br1⋯Br1] C7–Br1 1.843(4) Å, Br1⋯Br1 (1 − x, y − 0.5, 1.5 − z) 3.6215(5) Å, ∠C7Br1Br1 133.7(1)°. | |
The crystal structure of 2 is built up from discrete, weakly interacting 2D networks consolidated by C–H⋯Cl H-bonds and C–Br⋯Br–C inter-halogen contacts. A perspective view of this layer is depicted in Fig. 4 and S2.†
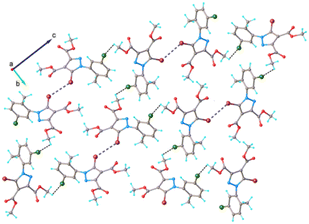 |
| Fig. 4 2D supramolecular layer in the crystal structure of 2 viewed along the a-axis. Hydrogen and inter-halogen Br⋯Br contacts are shown as dotted lines with black and blue colour, respectively. H-Bond parameters: C12–H⋯Cl1 [C12–H 0.96 Å, H⋯Cl1 (1.5 − x, y − 0.5, 1.5 − z) 2.82 Å, C12⋯Cl1 3.423(13) Å, ∠C12HCl1 121.8°]. Halogen contact parameters: [C8–Br1⋯Br1] C8–Br1 1.846(9) Å, Br1⋯Br1 (2 − x, 1 − y, 1 − z) 3.561(2) Å, ∠C8Br1Br1 128.9(3)°. | |
The crystals of 2 and 3 have very similar unit cell parameters and a common space group and their packing arrangements reveal a significant level of isostructurality. The intermolecular contacts shown by the molecule of the ASU in the crystal structure of 3 are represented in Fig. 5. They involve C–Br⋯O and C–Br⋯Br–C halogen contacts and C–H⋯Cl hydrogen bonds where those of shortest contacts occur with the participation of the CH3 groups as proton donors.
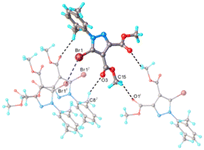 |
| Fig. 5 Intermolecular interactions in the crystal structure of 3. Symmetry generated molecules are shown with faded colors. Hydrogen and halogen contacts are shown as dotted lines with black and blue color, respectively. H-Bond parameters: C8–H⋯O3 [C8–H 0.96 Å, H⋯O3 (1 − x, 1 − y, 1 − z) 2.60 Å, C8⋯O3 3.479(5) Å, ∠C8HO3 151.7°]; C15–H⋯O1 [C15–H 0.96 Å, H⋯O1 (−x, −y, 1 − z) 2.61 Å, C15⋯O1 3.323(4) Å, ∠C15HO1 131.6°. Halogen contact parameters: [C9–Br1⋯Br1–C9] C9–Br1 1.856(3) Å, Br1⋯Br1 (2 − x, 2 − y, 1 − z) 3.6295(6) Å, ∠C9Br1Br1 148.04(9)°. | |
As with structure 2, the above-mentioned intermolecular contacts in 3 determine the formation of a two-dimensional network with the hydrophobic methyl groups oriented outside the plane, as shown in Fig. 6. The view of the packing diagram showing the arrangement of 2D architectures in the crystal is shown in Fig. S3.†
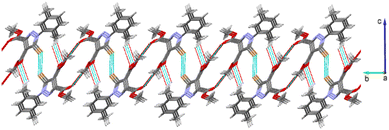 |
| Fig. 6 Two-dimensional supramolecular architecture in the crystal structure 3. Hydrogen and Br⋯Br contacts are shown as dotted lines with black and blue color, respectively. | |
As shown in Fig. 7, the short intermolecular contacts observed in the crystal of 4 involve numerous C–H⋯N and C–H⋯O hydrogen bonds as well as C–Br⋯Br′–C inter-halogen contacts. They are running in two perpendicular directions and therefore the aggregation of the molecules occurs towards the formation of a 2D supramolecular network (Fig. 8), which closely resembles that observed in the crystal 3 (Fig. 6). The packing of 2D layers in the crystals of 3 and 4 are also very similar (Fig. S3 and S4†).
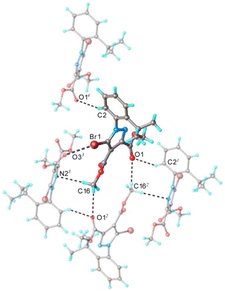 |
| Fig. 7 Intermolecular interactions in the crystal structure of 4. Symmetry generated molecules are shown with faded colors. Hydrogen and halogen contacts are shown as dotted lines with black and blue color, respectively. H-Bond parameters: C2–H⋯O1 [C2–H 0.93 Å, H⋯O1 (−x, 0.5 − y, 0.5 + z) 2.50 Å, C2⋯O1 3.337(5) Å, ∠C2HO1 150.1°]; C16–H⋯O1 [C16–H 0.96 Å, H⋯O1 (1 − x, 1 − y, 1 − z) 2.48 Å, C16⋯O1 3.299(5) Å, ∠C16HO1 143.4°; C16–H⋯N2 [C16–H 0.96 Å, H⋯N2 (1 − x, 0.5 + y, 0.5 − z) 2.59 Å, C2⋯N2 3.530(6) Å, ∠C16HN2 165.7°. Halogen contact parameters: [C10–Br1⋯O3] C10–Br1 1.855(4) Å, Br1⋯O3 (1 − x, 0.5 + y, 0.5 − z) 3.182(3) Å, ∠C9Br1O3 157.4(1)°. | |
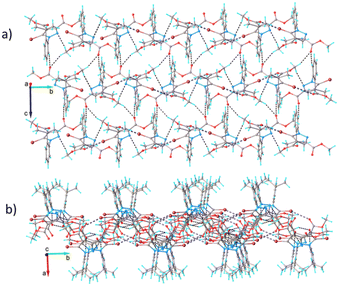 |
| Fig. 8 2D supramolecular network in the crystal of 4 viewed along the a- (a) and c- (b) axes. | |
The system of hydrogen and halogen intermolecular bonding found in crystal 5 is very similar to that observed for compounds 1–4. As illustrated in Fig. 9, each asymmetric unit is interconnected with seven adjacent molecules. Further analysis of the crystal packing shows the formation of complex and quite dense three-dimensional supramolecular aggregation, as depicted in Fig. 10. It can be characterized as being built from corrugated layers interconnected via C–H⋯O and Br⋯Br interactions.
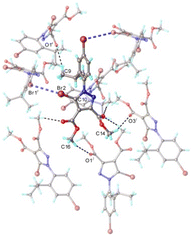 |
| Fig. 9 Intermolecular interactions in the crystal structure of 5. Symmetry generated molecules are shown with faded colors. Hydrogen and halogen contacts are shown as dotted lines with black and blue color, respectively. H-Bond parameters: C14–H⋯O3 [C14–H 0.97 Å, H⋯O3 (0.5 + x, 0.5 − y, 1 − z) 2.47 Å, C14⋯O3 3.245(5) Å, ∠C14HO3 136.3°]; C16–H⋯O1 [C16–H 0.97 Å, H⋯O1 (1 − x, 1 − y, 1 − z) 2.46 Å, C16⋯O1 3.392(5) Å, ∠C16HO1 161.4°; C9–H⋯O1 [C9–H 0.97 Å, H⋯O1 (1 − x, 0.5 + y, 0.5 − z) 2.57 Å, C9⋯O1 3.529(5) Å, ∠C9HO1 169.2°. Halogen contact parameters: [C10–Br2⋯Br1′–C4] C10–Br1 1.855(4) Å, Br1⋯Br2 (x − 0.5, y, 0.5 − z) 3.7085(7) Å, ∠C10Br1Br2 156.9(1)°, ∠C4Br2Br1 99.5(1)°. | |
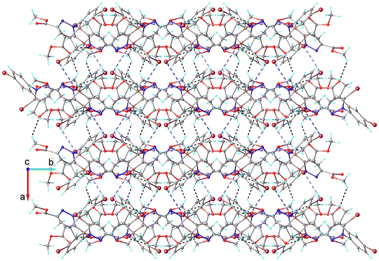 |
| Fig. 10 Partial view of the crystal packing in 5. Hydrogen and halogen contacts are shown as dotted lines with black and blue color, respectively. | |
Hirshfeld (HF) analysis
Compound 2.
The presence of the ortho methyl group in the molecule of 2 changes the torsion angle between the phenyl plane and pyrazole plane from 56° (compound 1) to 86°. The chlorine atom also plays an important role in creating C–H⋯Cl bonds which drive the formation of layers which are interconnected in 3D by C
O⋯H contacts. Only one ester group is involved in C
O⋯H bonds. The HF surface of compound 2 (Table 2) shows however the red spot corresponding to a close contact involving the Br atom. This corresponds to a short, symmetrical Br⋯Br contact of 3.554 Å (96% of the sum of the vdW radii). The angle of 129.2° might not be so favorable but it agrees with a formal Br⋯Br inter-halogen contact of type I (Fig. 13).30 The distance Br⋯O is larger than the sum of the vdW radii and even though the atoms lie in the same plane with geometrically favorable interaction, this cannot be considered as a formal halogen interaction.
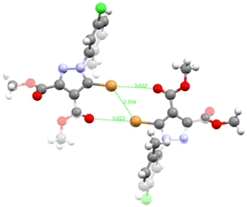 |
| Fig. 13 Formal type I Br⋯Br contact between two adjacent molecules of compound 2. | |
Compound 3.
For compound 3 the replacement of the Cl atom with a methyl group implies an increase of the contribution of H⋯H contacts from 27.7% to 40.9% (see ESI† material for fingerprint plots). However, we can observe from the Hirshfeld surface (Table 2) that the most important contacts are also for C
O⋯H bonds involving the ester groups and also the methyl group introduced to replace the chlorine atom. The Hirshfeld surface also presents a short contact for the Br atom and indeed a similar kind of dimer should be envisaged for compound 3 as for compound 2, given their isostructurality. In this case the carbonyl oxygen atom points out of the plane and forms C
O⋯H bonds with the methyl group hydrogen. In this context, the Br⋯Br contact presents a more favorable geometry with an angle of 148.0° but a greater contact distance of 3.628 Å (98% of the sum of the vdW radii). Quantitatively, the fingerprint plot shows an increase in the Br⋯Br contacts compared to 2, this being enhanced in the interactions diagram in Table 2 (ESI† for fingerprint plots). However, in this case the H-bonds appear with the same directionality as the Br⋯Br contacts (Fig. 14).
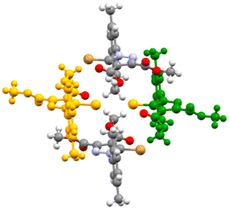 |
| Fig. 14 Formal type I Br⋯Br contact between two adjacent molecules (orange and green) of compound 3. Compared to compound 2 the C O groups are involved in H-bonds with the methyl hydrogens of two other molecules instead of pointing towards the Br atom as in compound 2. | |
Compound 4.
As with compound 3, Hirshfeld analysis and fingerprint plots for 4 reveal the highest degree of H⋯H contacts and this is due to the bulky isopropyl group in the ortho position relative to the pyrazole ring. In addition, the percentage of O⋯H contacts is also the highest, at 21.6%. The red spot at the Br atom reveals a Br⋯O bond (3.182 Å, 94.4% of the sum of the vdW radii). Interestingly, the presence of an intramolecular N⋯H bond involves the N2 atom and the CH unit of the isopropyl group. All the interactions, H⋯O, Br⋯O and H⋯N, led to the formation of infinite 3D networks (Fig. 15) with the phenyl rings pointing outwards. These networks are interpenetrated through the phenyl rings which accommodate the greater part of the H⋯H and C⋯H contacts.
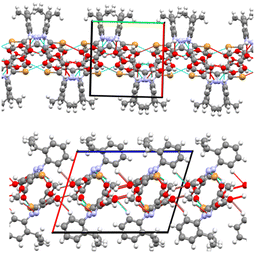 |
| Fig. 15 H⋯H and C⋯H interactions in 4 to show the interpenetration of 3D networks of strong interactions. | |
By comparison with the molecule of 4, its 5-iodo analogue (TOSCAI)38 presented a strong I⋯N bond, leading to a different configuration of the molecular packing. Given that the two analogues led to different supramolecular patterns it is clear that in this case the halogen bond strongly competes with the rest of the intermolecular contacts, at least in the case of the iodine atom.
Compound 5.
By introducing a Br atom in the para position of the phenyl ring it can be observed that the H⋯H interactions are reduced relative to compound 4 and Br⋯H interactions appear. Fingerprint plots confirm this observation quantitatively. However, the most important contacts are the C–O⋯H bonds involving the oxygen atoms in the ester groups. The structures of compounds 4 and 5 are similar to some extent, small differences being observed in the fact that the phenyl rings are slightly offset. By comparison with the 5-iodo analogue EJULON38 one can observe an identical arrangement of the molecules in the crystal packing, with similar distances and torsion angles and similar bonding patterns (Fig. 16). The halogen–halogen distances are 3.647 Å for Br⋯I in EJULON and 3.701 Å for Br⋯Br in compound 5. In the case of EJULON the distance is 96% of the sum of the vdW radii compared to 5 which is at the limit of the sum of the vdW radii. We can deduce from both examples that the two structures EJULON and compound 5 are isostructural63,64 in that they crystallize in the same space group Pbca with isostructural molecular and crystal packing arrangements, as well as corresponding fingerprint plots.
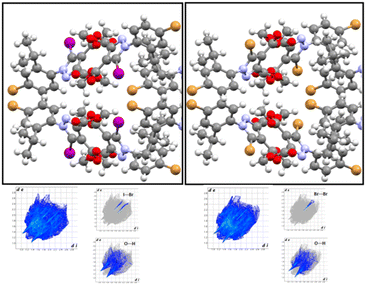 |
| Fig. 16 5-Br 5 and 5-I EJULON38 isostructural pyrazoles. Fingerprint plots present similar blueprints for I⋯Br hetero(inter)halogen bond and Br⋯Br. | |
Conclusions
In conclusion, five 5-bromo substituted pyrazoles were investigated by X-ray diffraction analysis to evaluate the influence of the Br atom as halogen bond donor/acceptor and the contribution of the halogen bonds among the other intermolecular crystal interactions. To date, the literature on studies of the supramolecular structure of 5-halogenated pyrazoles, and moreover their halogen bond propensity, is rather scarce and we have demonstrated that compounds in this class could give rise to interesting features rendering them good candidates for halogen bonding investigations. The latter can lead to an improved degree of predictability of halogen bond formation in more complex molecules. We showed that in quite complex molecules the bromine atom plays an important role, being involved in halogen bonds or inter-halogen contacts, the most interesting being the trifurcated Br contacts in molecule 1. Isostructurality or similarity of the crystal packing is another proof that even in situations where there is high competition among the intermolecular forces, halogen contacts are important directional forces. On the other hand Br atoms in 5-brominated pyrazoles showed more affinity to Br⋯O contacts or inter-halogen contacts than their iodo-analogues and no Br⋯N contact was observed. Even though the inter-halogen Br⋯Br contacts appear to be enforced by other stronger intermolecular competitive forces, their propensity becomes significant for compounds of this type.
This work continues our efforts in simplifying and standardizing halogenated 1-arylpyrazoles as important structures for halogen bonding investigation owing to their similarity with molecules relevant to the medicinal or biological field.
Conflicts of interest
There are no conflicts to declare.
Acknowledgements
MRC thanks the University of Cape Town for providing access to research facilities. SS acknowledges the support provided by the research infrastructure developed through the European Social Fund for Regional Development, Competitiveness Operational Programme 2014–2020, Axis 1, Action: 1.1.3, Project “Infra SupraChem Lab – Center for Advanced Research in Supramolecular Chemistry” (339/390015/25.02.2021, MySMIS: 108983).
Notes and references
- M. Erdely, Nat. Chem., 2014, 6, 762–764 CrossRef.
- G. R. Desiraju, P. S. Ho, L. Kloo, A. C. Legon, R. Marquardt, P. Metrangolo, P. Politzer, G. Resnati and K. Rissanen, Pure Appl. Chem., 2013, 85, 1711–1713 CrossRef CAS.
- P. Metrangolo, H. Neukirch, T. Pillati and G. Resnati, Acc. Chem. Res., 2005, 38, 386–395 CrossRef CAS PubMed.
- A. Mukherjee, S. Tothadi and G. R. Desiraju, Acc. Chem. Res., 2014, 47, 2514–2524 CrossRef CAS PubMed.
- P. Metrangolo and G. Resnati, Halogen bonding, I. impact on materials chemistry and life sciences, Top. Curr. Chem., 2015, 358 Search PubMed.
- M. Benito, Y. Roselló, M. Barceló-Oliver, A. Frontera and E. Molins, Int. J. Mol. Sci., 2021, 22, 10663 CrossRef CAS.
- A. V. Rozhkov, A. A. Eliseeva, S. V. Baykov, B. Galmes, A. Frontera and V. Yu. Kukushkin, Cryst. Growth Des., 2020, 20, 5908–5921 CrossRef CAS.
- P. Metrangolo, G. Resnati, T. Pilati and S. Biella, Halogen bonding in crystal engineering, Struct. Bonding, 2008, 126, 105–136 CrossRef CAS.
- N. Bedekovic, L. Fotovic, V. Stilinovic and D. Cincic, Cryst. Growth Des., 2022, 22, 987–992 CrossRef CAS.
- C. Draghici, M. R. Caira, D. E. Dumitrescu and F. Dumitrascu, Rev. Chim., 2018, 69, 843–845 CrossRef CAS.
- M. Erdely, Chem. Soc. Rev., 2012, 41, 3547–3557 RSC.
- R. Glasser, N. Chen, H. Wu, N. Knotts and M. Kaupp, J. Am. Chem. Soc., 2004, 126, 4412–4419 CrossRef.
- A. V. Jentzsch, Pure Appl. Chem., 2015, 87, 15–41 CrossRef.
- M. H. H. Voelkel, P. Wonner and S. M. Huber, ChemistryOpen, 2020, 9, 214–224 CrossRef CAS PubMed.
- D. Von der Heiden, A. Vanderkooy and M. Erdélyi, Coord. Chem. Rev., 2020, 407, 213147 CrossRef CAS.
- P. M. J. Szell, S. Zablotny and D. L. Bryce, Nat. Commun., 2019, 10, 916 CrossRef.
- C. Gropp, T. Husch, N. Trapp, M. Reiher and F. Diederich, J. Am. Chem. Soc., 2017, 139, 12190–12200 CrossRef CAS PubMed.
- D. Bulfield and S. M. Huber, Eur. J. Chem., 2016, 22, 14434–14450 CrossRef CAS.
- X. Liu and P. H. Toy, Adv. Synth. Catal., 2020, 362, 3437–3441 CrossRef CAS.
- M. R. Scholfield, C. M. V. Zanden, M. Carter and P. S. Ho, Protein Sci., 2013, 22, 139–152 CrossRef CAS.
- A. R. Voth and P. S. Ho, Curr. Top. Med. Chem., 2007, 7, 1336–1348 CrossRef CAS PubMed.
- J. Heidrich, L. E. Sperl and F. M. Boeckler, Front. Chem., 2019, 7, article 9 CrossRef.
- R. Kurczab, V. Canale, G. Satala, P. Zajdel and A. J. Bojarski, J. Med. Chem., 2018, 61, 8717–8733 CrossRef CAS.
- Y. Lu, Y. Liu, Z. Xu, H. Li, H. Liu and W. Zu, Expert Opin. Drug Discovery, 2012, 7, 375–383 CrossRef CAS.
- B. Mallada, A. Gallardo, M. Lamanec, B. De La Torre, V. Spirko, P. Hobza and P. Jelinek, Science, 2021, 374, 863–867 CrossRef CAS.
- T. Clark, M. Henneman, J. S. Murray and P. Politzer, J. Mol. Model., 2007, 13, 291–296 CrossRef CAS PubMed.
- M. H. Kolár and P. Hobza, Chem. Rev., 2016, 116, 5155–5187 CrossRef PubMed.
- P. Politzer, J. S. Murray, T. Clark and G. Resnati, Phys. Chem. Chem. Phys., 2017, 19, 32166–32178 RSC.
- P. Politzer and J. S. Murray, Crystals, 2017, 7, 212 CrossRef.
- P. Metrangolo and G. Resnati, IUCrJ, 2014, 5–7 CrossRef CAS.
- G. Cavallo, P. Metrangolo, R. Milani, T. Pilati, A. Priimagi, G. Resnati and G. Terraneo, Chem. Rev., 2016, 116, 2478–2601 CrossRef CAS PubMed.
- M. A. A. Ibrahim and A. M. Moussa, ACS Omega, 2020, 5(34), 21824–21835 CrossRef CAS.
- L. Happonen, J. M. Rautiainen and A. Valkonen, Cryst. Growth Des., 2021, 6, 3409–3419 CrossRef.
- N. Bedekovic, V. Stilinovic, T. Friscic and D. Cincic, New J. Chem., 2018, 42, 10584–10591 RSC.
- E. D. Speetzen, E. Bosch, M. Erdeliy and N. P. Bowling, Eur. J. Org. Chem., 2015, 1685–1695 Search PubMed.
- M. Bedin, A. Karim, M. Reitti, A.-C. C. Carlsson, F. Topic, M. Cetina, F. Pan, V. Havel, F. Al-Ameri, V. Sindelar, K. Rissanen, J. Grafenstein and M. Erdelyi, Chem. Sci., 2015, 6, 3746–3756 RSC.
- W. N. Moss and N. S. Goroff, J. Org. Chem., 2005, 70, 802–808 CrossRef CAS.
- M. M. Popa, I. C. Man, C. Draghici, S. Shova, M. R. Caira, F. Dumitrascu and D. Dumitrescu, CrystEngComm, 2019, 21, 7085–7093 RSC.
- D. Dumitrescu, S. Shova, I. C. Man, M. R. Caira, M. M. Popa and F. Dumitrascu, Crystals, 2020, 10, 1149 CrossRef CAS.
- M. M. Popa, S. Shova, M. Hrubaru, L. Barbu, C. Draghici, F. Dumitrascu and D. E. Dumitrescu, RSC Adv., 2020, 10, 15656–15664 RSC.
- P. Cabildo, R. M. Claramunt, C. Lopez, M. A. Garcia, M. Perez-Torralba, E. Pinilla, M. R. Torres, I. Alkorta and J. Elguero, J. Mol. Struct., 2011, 985, 75–81 CrossRef CAS.
- M. Corro, M. Besora, C. Maya, E. Álvarez, J. Urbano, M. R. Fructos, F. Maseras and P. J. Pérez, ACS Catal., 2014, 4, 4215–4222 CrossRef CAS.
- S. Orbisaglia, C. Di Nicola, F. Marchetti, C. Pettinari, R. Pettinari, L. M. D. R. S. Martins, E. C. B. A. Alegria, M. F. C. G. da Silva, B. G. M. Rocha, M. L. Kuznetsov, A. J. L. Pombeiro, B. W. Skelton, A. N. Sobolev and A. H. White, Chem. – Eur. J., 2014, 20, 3689–3704 CrossRef CAS.
- M. Trose, F. Nahra, A. Poater, D. B. Cordes, A. M. Z. Slawin, L. Cavallo and C. S. J. Cazin, ACS Catal., 2017, 7, 8176 CrossRef CAS.
- E. Haldón, M. Delgado-Rebollo, A. Prieto, E. Álvarez, C. Maya, M. C. Nicasio and P. J. Pérez, Inorg. Chem., 2014, 53, 4192–4201 CrossRef.
- L. R. Kadel, J. R. Kromer, C. E. Moore and D. M. Eichhorn, Polyhedron, 2017, 125, 206–218 CrossRef CAS.
- G. J. Gainsford and G. B. Evans, Acta Crystallogr., Sect. E: Struct. Rep. Online, 2002, 58, o1021–o1022 CrossRef CAS.
- E. Nicolas, T. Cheisson, G. B. de Jong, C. G. J. Tazelaar and J. C. Slootweg, Acta Crystallogr., Sect. C: Struct. Chem., 2016, 72, 846–849 CrossRef CAS.
-
S. Trofimenko and G. P. A. Yap, CCDC 1968568: Experimental Crystal Structure Determination, 2019, DOI:10.5517/ccdc.csd.cc242g6x.
- M. Pejic, S. Popp, M. Bolte, M. Wagner and H.-W. Lerner, Z. Naturforsch. (B), 2014, 69, 83–97 CrossRef CAS.
- J. Urbano, A. A. C. Braga, F. Maseras, E. Alvarez, M. M. Diaz-Requejo and P. J. Perez, Organometallics, 2009, 28, 5968–5981 CrossRef CAS.
-
K. Kunz and M. Bolte, CSD Communication, 2019; CCDC 1941458: Experimental Crystal Structure Determination, 2019, DOI:10.5517/ccdc.csd.cc2357p7.
-
C. Pettinari, B. W. Skelton and A. H. White, CSD Communication, 2017; CCDC 945261: Experimental Crystal Structure Determination, 2017, DOI:10.5517/cc10qm9n.
- F. Dumitrascu, C. Draghici, D. Dumitrescu, L. Tarko and D. Raileanu, Liebigs Ann./Recl., 1997, 2613–2616 CrossRef CAS.
-
(a) F. Dumitrascu, C. I. Mitan, D. Dumitrescu, L. Barbu, M. Hrubaru and D. Caproiu, Rev. Chim., 2003, 54, 747 CAS;
(b) F. Dumitrascu, C. Draghici, C. Crangus, M. T. Caproiu, C. I. Mitan, D. Dumitrescu and D. Raileanu, Rev. Roum. Chim., 2002, 47, 315–318 Search PubMed;
(c) F. Dumitrascu, I. C. Mitan, D. Dumitrescu, C. Drăghici and M. T. Caproiu, ARKIVOC, 2002, ii, 80–86 Search PubMed.
-
Rigaku Oxford Diffraction, (2015), CrysAlisPro Software system, version 1.171.41.64, Rigaku Corporation, Oxford, UK Search PubMed.
- G. Sheldrick, SHELXT – Integrated space-group and crystal-structure determination, Acta Crystallogr., Sect. A: Found. Adv., 2015, 71, 3–8 Search PubMed.
- G. Sheldrick, Crystal structure refinement with SHELXL, Acta Crystallogr., Sect. C: Struct. Chem., 2015, 71, 3–8 Search PubMed.
- O. V. Dolomanov, L. J. Bourhis, R. J. Gildea, J. A. K. Howard and H. Puschmann, OLEX2: a complete structure solution, refinement and analysis program, J. Appl. Crystallogr., 2009, 42, 339–341 CrossRef CAS.
- M. A. Spackman and D. Jayatilaka, CrystEngComm, 2009, 11, 19–32 RSC.
- P. R. Spackman, M. J. Turner, J. J. McKinnon, S. K. Wolff, D. J. Grimwood, D. Jayatilaka and M. A. Spackman, J. Appl. Crystallogr., 2021, 54, 1006–1011 CrossRef CAS PubMed.
- A. Bondi, J. Phys. Chem., 1966, 70, 3006–3007 CrossRef CAS.
- D. Cincic, T. Friscic and W. Jones, Chem. Mater., 2008, 20, 6623–6626 CrossRef CAS.
- L. Fotovic, N. Bedekovic and V. Stilinovic, Cryst. Growth Des., 2022, 22, 1333–1344 CrossRef CAS PubMed.
|
This journal is © The Royal Society of Chemistry 2023 |
Click here to see how this site uses Cookies. View our privacy policy here.