Occurrence and removal of four artificial sweeteners in wastewater treatment plants of China†
Received
25th August 2022
, Accepted 3rd November 2022
First published on 4th November 2022
Abstract
Artificial sweeteners discharged into aquatic environments have raised concern because of their ubiquitous occurrence and potential biological effect. And some of them, such as sucralose (SUC) and acesulfame (ACE), have been identified as emerging contaminants. Wastewater treatment plants (WWTPs) are considered as important sources and sinks of artificial sweeteners discharged into the environment. In this study, the occurrence and removal of four representative artificial sweeteners in 12 WWTPs located in different provinces of China were investigated. The results showed that artificial sweeteners were detected widely in the investigated WWTPs. The median concentrations of the four target artificial sweeteners were detected in influents at levels of 0.03–3.85 μg L−1 and decreased in the order of SUC > ACE > aspartame (APM) > neotame (NTM). Additionally, the per capita mass loads of total artificial sweeteners in the WWTPs could be affected by the location of the WWTPs and were higher in southern cities than in northern cities. It was also found that there was a distinct linear correlation between the per capita mass load of ACE in influents and population density. During the treatment of WWTPs, the overall removal efficiency of artificial sweeteners ranged from −116% to 99.1%. Among the target artificial sweeteners, SUC and ACE might have potential risk to aquatic environments based on the calculation of the risk quotient. Thus, advanced treatment processes were carried to further remove SUC and ACE to reduce their long-term cumulative effect. Overall, UV/H2O2 and UV/PDS showed a better effect than granular activated carbon (GAC) adsorption in the removal of artificial sweeteners. The reaction constants of ACE by UV/H2O2 and UV/PDS were higher than those of SUC, which is related to molar extinction coefficients. Meanwhile, the adsorption ability of GAC adsorption for SUC was better than that of ACE, which is in correlation with the octanol–water partition coefficient. By comparison of removal efficiency, UV/PDS was considered as the most suitable advanced treatment process to remove ACE and SUC.
Environmental significance
Artificial sweeteners are recognized as emerging contaminants in aquatic environments because of their ubiquitous occurrence and potential biological effect. In this study, the occurrence and removal efficiency of four representative artificial sweeteners in 12 WWTPs located in different provinces of China were investigated. Moreover, the risk quotient of the four artificial sweeteners to aquatic environment was calculated and 3 advanced treatment processes were carried out to further remove them to reduce their long-term cumulative effect. These results would provide a basis to assess their potential ecological risks and search for a suitable way of removing artificial sweeteners effectively.
|
1 Introduction
Artificial sweeteners, as sugar substitutes, are consumed prevalently in foods, beverages, confectionary, pharmaceuticals and sanitary products.1,2 Among them, sucralose (SUC), aspartame (APM), acesulfame (ACE) and neotame (NTM) are the most popular artificial sweeteners around the world and are consumed the most in China.3–5 The consumption of APM is the most (18.5 × 103 metric tons (Mt)) in food and beverage products, followed by ACE (6.8 × 103 Mt) and SUC (3.3 × 103 Mt).6 And NTM has been recognized by the public as a second-generation sweetener.3 Owing to the huge consumption in daily life, artificial sweeteners have been detected frequently with high concentrations in environmental media globally.7–9 Additionally, ACE and SUC were proved to be persistent during WWTPs. Due to the ubiquitous occurrence and recalcitrant nature of some artificial sweeteners, they are usually regarded as chemical markers for tracers of wastewater discharged to environmental water.10
Wastewater treatment plants (WWTPs) are considered as important sources and sinks of artificial sweeteners discharged into the environment. Previous studies have described the fate of artificial sweeteners in different environmental media globally in recent years. For instance, in the New York State, America, SUC, APM, and ACE were detected at average concentrations ranging from 0.13 to 29.4 μg L−1 in WWTP influents, from 0.11 to 29.6 μg L−1 in effluents, and from 0.08 to 0.65 μg g−1 (dry weight, dw) in sludge, respectively.2 In contrast, NTM was less frequently detected in WWTPs with concentrations ranging from below method detection limits to 0.01 μg L−1 in Tianjin WWTPs, China.11 Besides, SUC and ACE in nine WWTPs located in the Pearl River Delta region have been investigated.12 Nonetheless, comprehensive research of artificial sweeteners in WWTPs at different cities on a national scale in China has not been reported yet. The removal of artificial sweeteners is the key to preventing and controlling artificial sweetener pollution in downstream water. Generally, the removal of artificial sweeteners was quite diverse owing to the different microbial communities in activated sludge in various WWTPs. It's very important and meaningful to investigate the behaviors of artificial sweeteners during WWTP treatment processes.
Although the negative impacts of artificial sweeteners on human health have been ignored and still unclear in previous research,5 their long-term cumulative effect in the environment still caused considerable concern. For instance, SUC may affect the behavior of cladocerans at environmentally relevant concentrations of 0.5–500 μg L−1.13 Analogously, ACE with the concentration of no observed effect concentrations (NOECs) was found to remarkably alter the neuro-behavioral profiles of zebrafish.14 Therefore, knowing the potential ecological risks of artificial sweeteners from WWTPs discharged into the environment is urgently needed.
Due to the limited ability of conventional WWTP wastewater treatment technologies to remove chemically stable artificial sweeteners, advanced treatment technology for eliminating them is required.11,15 Advanced oxidation processes (AOPs), such as UV/hydrogen peroxide (UV/H2O2) and UV/peroxydisulfate (UV/PDS) based on the production of hydroxyl radicals (HO˙) and sulfate radicals (SO
−), can contribute to the degradation of recalcitrant trace organic chemicals in water effectively.16,17 Apart from them, granular active carbon (GAC) adsorption, as a kind of common advanced treatment technology, is frequently used to adsorb micropollutants.17 GAC was suggested to be the most promising adsorptive medium to improve the removal of artificial sweeteners in on-site WWTPs.18 Additionally, the residual concentrations and chemical characteristics of artificial sweeteners in effluents are different. Thus, it is necessary to find a suitable advanced treatment to reduce the risk of artificial sweeteners in effluents.
This study investigated the overall occurrence and removal performance of artificial sweeteners in 12 municipal WWTPs located in different provinces of China, and compared the degradation efficiency of artificial sweeteners with that of different advanced treatment technologies. The results may provide an increased understanding of the overall occurrence and risk of artificial sweeteners in WWTPs of China and provide a perspective for controlling artificial sweeteners in wastewater with advanced treatment systems.
2 Materials and methods
2.1 Chemicals and consumables
NTM, APM, ACE, SUC (above 98% pure), internal standards (sucralose-d4 (SUC-d4)) and tris (hydroxymethyl) aminomethane with purity higher than 99% were purchased from Millipore Sigma (St. Louis, MO, USA). All reagents of high-performance liquid chromatography (HPLC) grade (methanol, ethyl acetate and acetonitrile) used for sample processing and analysis were provided by Merck Corporation (Merck, Darmstadt, Germany). Ultrapure water (18.2 MΩ cm) was prepared using a Milli-Q RC apparatus from Millipore Corporation (Bedford, MA, USA).
2.2 Sample collection
Wastewater and sludge samples were collected from 12 municipal WWTPs. These WWTPs with daily fluxes ranging from 100
000 to 1
000
000 m3 per day are distributed in 12 cities of China. Detailed information of each WWTP (Plant A-Plant L) is listed in Table S1.† Furthermore, the concentrations of artificial sweeteners in influents, effluents, and excess sludge of the 12 WWTPs are listed in Table S2.† All of the samples were collected under normal operating conditions and avoiding raining events during the sampling period. Twenty-four-hour composite wastewater samples were collected from each WWTP by using refrigerated automatic samplers. All the wastewater samples were taken in triplicate. While collecting, 4 mol per L H2SO4 was added to the samples to regulate the pH value of wastewater samples to suppress microbial activity. Samples were stored in pre-cleaned 1 L amber glass bottles, chilled in incubators containing a set number of ice boxes, and immediately transported to the laboratory. Wastewater samples were firstly filtered through glass microfiber filters (0.7 μm, Whatman, UK) and spiked with 100 μL internal standards (1 mg L−1), and then stored at 4 °C out of light and extracted within 24 h. Similarly, sludge samples obtained in the morning, afternoon, and evening in a WWTP were mixed together as a composite sample. And then, sludge samples were freeze-dried under vacuum (Labconco, MO, USA), homogenized, sieved (100-mesh), spiked with internal standards (200 ng g−1) and stored in darkness at −20 °C, and the extraction was carried out within 48 h.
2.3 Sample extraction
Wastewater extraction was carried out based on the method in a previous study.19 Briefly, 500 mL influent and 1 L effluent were extracted with CNW Poly-Sery PWAX cartridges (3 mL, 60 mg, CNW, ANPEL, Shanghai, China). The cartridges were dried and eluted with 6 mL methanol containing 1 mM tris (hydroxymethyl) aminomethane. The effluents were dried and then re-dissolved with 1 mL of ultrapure water. Details on samples extraction are described in Text S1.†
2.4 Laboratory-scale advanced treatment experiments
Photochemical experiments were conducted using a photoreaction operation reactor (XPA-7, Nanjing Xujiang Motor Factory, China). A low-pressure mercury lamp (22 W, 254 nm) was placed at the center of the reactor with a quartz cover, which was supplied by an electronic ballast. The fluency rate of the lamp was 2.66 × 10−6 E (L−1 s−1) based on iodide-iodate chemical actinometry.19 The UV irradiation intensity was 520 μW cm−2. 100 μg L−1 individual solutions of four artificial sweeteners (NTM, APM, ACE, and SUC) (dissolved in ultrapure water) were chosen as experimental concentrations, and 34 mg L−1 specific oxidants (PDS or H2O2) were added to the samples before UV irradiation. In order to imitate the conditions of actual wastewater, the pH of reaction solution was adjusted to 7 with phosphate buffer solution (2 mM). Samples were taken at specific time (0, 2, 5, 10, and 30 min) intervals and the experiment was repeated three times. 50 mL of 100 μg L−1 artificial sweetener solution was shaken at 130 rpm with 3 mg of GAC (JACOBI, AquaSorb PICABIOL2, 8 × 18 Mesh) to conduct the adsorption experiment. The temperature was maintained at 25 ± 0.2 °C. At specific time intervals, 1 mL of samples after solid phase extraction was transferred to an ultra-performance liquid chromatography-mass spectrometer (UPLC-MS) for analysis.
2.5 Measurement of artificial sweetener concentrations
The artificial sweeteners were analyzed using a UPLC-MS Xevo TQ-S (advanced benchtop tandem Triple quadrupole mass spectrometer from Waters Xevo TQ-S UPLC-MS, USA). The separation of artificial sweeteners was accomplished with a UPLC BEH C18 column (1.7 μm, 2.1 × 100 mm, Waters Corp, USA) at 40 °C. The mobile phases consisted of water (A) containing 0.1% (v/v) ammonium acetate and methanol (B). The injection volume was 10 μL, and gradient elution was carried out at a flow rate of 0.25 mL min−1. The system was allowed to equilibrate for 2 min before the next injection circulation with 10 μL volume. Two multi-selected reaction monitoring (MRM) transitions with individual authentic standards were set to identify and quantify artificial sweeteners during the whole experiment procedure. The physicochemical properties of artificial sweeteners are shown in Table S3.† The MRM conditions, recoveries, method limit of detection (LOD) and limit of quantification (LOQ) of the artificial sweeteners and gradient conditions of mobile phases for HPLC are listed in Tables S4 and S5.† The LOD and LOQ of each artificial sweetener were determined as the concentration with a minimum signal-to-noise (S/N) ratio of 3 and 10 under the lowest spiked concentration of wastewater and sludge samples, respectively. An instrumental blank and procedure blank were analyzed for each 10 samples, and none of the artificial sweeteners has been detected in them. The LOQ of artificial sweeteners for wastewater ranged from 12 to 100 ng L−1 and for sludge it ranged from 0.18 to 0.75 ng g−1 (dry weight, dw). To validate the accuracy of this method, a recovery experiment has been done before using this method, and the recoveries of the four artificial sweeteners are in the range of 87–95% for wastewater (n = 6) and 82–92% for sludge (n = 6). Besides, the R2 of the four artificial sweetener standard curves with concentrations ranging from 0.1 μg L−1 to 500 μg L−1 exceeded 0.99.
2.6 Environmental risk assessment
The potential risks of residual artificial sweeteners in effluents to fish were assessed using the risk quotient (RQ) approach, which is calculated by the predicted environmental concentration (PEC) dividing the predicted no-effect concentration (PNEC) of a chemical. Because the effluents will be diluted into surface water, a default dilution factor of 10 was used to obtain local surface water.20 According to the European Commission Technical Guidance Document,21 the PNEC value of a target compound was calculated using the aquatic acute median effective concentration (EC50) or the NOEC to divide by an assessment factor. In the present study, the PNEC value of a target artificial sweetener was calculated by dividing its lowest EC50 or chronic NOEC to fish by an assessment factor.
Therefore, the RQ was calculated by using eqn (1):
| 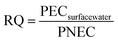 | (1) |
Classification according to the RQ values: no risk (RQ < 0.01); low risk (0.01 ≤ RQ < 0.1); medium risk (0.1 ≤ RQ < 1); high risk (RQ ≥ 1).22 This RQ ranking criterion was a good fit for this study. The detailed toxicity data used for RQ calculations are shown in Table S6.†
2.7 Data analysis
The removal efficiencies of artificial sweeteners were calculated by using eqn (2): |  | (2) |
The per capita mass loads of artificial sweeteners were calculated by using eqn (3):
|  | (3) |
Spearman's correlation coefficient (r) between the calculated per capita mass loads of artificial sweeteners and the population density in WWTP service areas was calculated using python 3.8. A statistical test (t-test) was done by using the SPSS 17.0 software program (SPSS Inc., Chicago, IL).
3 Results and discussion
3.1 Occurrence of artificial sweeteners in the 12 WWTPs
3.1.1 Concentrations and distributions of artificial sweeteners in influents.
Fig. 1 shows the occurrence and mean concentrations of artificial sweeteners in the influents of the 12 WWTPs. As shown in Fig. 1, all four artificial sweeteners in influents showed 100% detection frequencies, confirming that these artificial sweeteners were distributed in the WWTPs of China ubiquitously. The concentrations of total artificial sweeteners in WWTP influents from different regions showed evidently different distributions. Among the four target artificial sweeteners, the commonly used artificial sweeteners, SUC, ACE and APM, were 100% detected in the influents of the 12 WWTPs with median concentrations of 3.85 μg L−1, 1.29 μg L−1 and 0.56 μg L−1, respectively, while NTM, which was less produced, was detected with a lowest median concentration of 0.03 μg L−1. SUC and ACE were the predominant artificial sweeteners at the concentration levels in the influents (Fig. 1(a) and (b)), accounting for 51.08% (Plant H) to 94.58% (Plant D) and 4.98% (Plant D) to 31.48% (Plant F) of total artificial sweetener levels, respectively, which meant that ACE and SUC might be widely used in China. It was found that the concentrations of artificial sweeteners in the WWTPs showed a direct correlation with their consumption.23 Similarly, SUC accounts for the majority of the artificial sweetener market in the United States.24
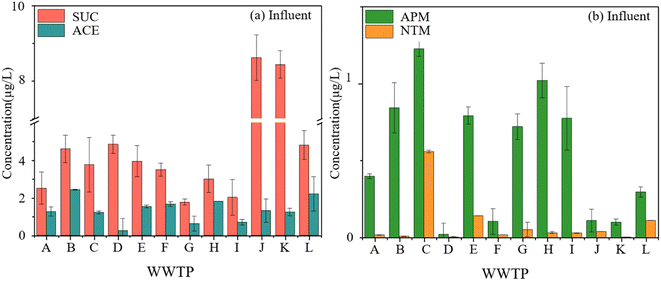 |
| Fig. 1 Occurrence and mean concentrations (±standard deviations (n = 3)) of artificial sweeteners in the (a) influents (ACE and SUC) and (b) influents (APM and NTM) from the 12 WWTPs. | |
The detected concentrations of SUC were comparable to those previously reported in WWTPs from Singapore (1.4–5.8 μg L−1). Meanwhile, the mean concentrations of ACE in the present study were similar to those at WWTPs from Singapore (6.2–9.8 μg L−1),25 USA (1.08 μg L−1) and Sweden (1.7–3.2 μg L−1).2,26 Correspondingly, the concentration of APM was generally similar to that at a WWTP from the USA (0.49 μg L−1).2 In addition, NTM is a sweetener, which was detected less frequently, and its concentrations ranged from the LOD to 0.56 μg L−1.
The concentrations and region distributions of total artificial sweeteners and chemical oxygen demand (COD) in influent samples from the 12 China's WWTPs are shown in Fig. 2(a). It shows that artificial sweeteners have regional distribution characteristics. Overall, by calculation, the per capita mass loads of total artificial sweeteners in the WWTPs could be affected by the location of the WWTPs. For instance, the per capita mass loads of total artificial sweeteners in southern cities (WWTP G-L) were higher than in northern cities (WWTP A-F) (p = 0.042 < 0.05). The geographical distribution of the per capita mass loads of total artificial sweeteners in WWTP influents can reflect the differences of the lifestyle and consumption patterns in different regions to some extent.23
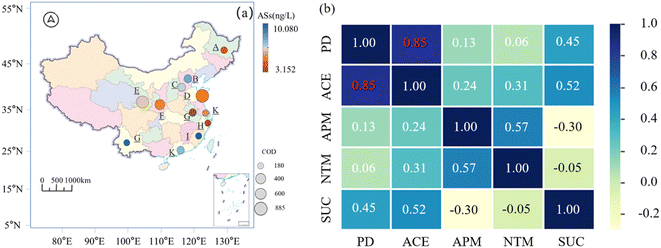 |
| Fig. 2 Distributions and correlation analysis of artificial sweeteners. (a) Concentrations and distributions of total artificial sweeteners and COD in influent samples from the 12 WWTPs. (b) Correlation analysis of the per capita mass loads of the four artificial sweeteners and population density. | |
Spearman's correlation was used to analyze the calculated per capita mass loads (Table S7†) of artificial sweeteners and the population density (Table S1†) in WWTP service areas. It was found that there was a strong linear correlation between the per capita mass loads of ACE and population density (r = 0.85), which is shown in Fig. 2(b). Similarly, Subedi et al.27 found that multiple linear regression analysis revealed that several WWTP parameters, such as treatment capacity, population equivalent and hydraulic retention time, could influence the artificial sweetener concentrations in Korean WWTPs. And Buerge et al.28 also found that the concentrations of artificial sweeteners in effluents from WWTPs in Switzerland increased with population in the catchment area. Additionally, a previous report found that artificial sweetener emissions in a region also have been closely related to consumption patterns. Neset et al.26 indicated that at present not one large source but rather several small sources contribute to the artificial sweetener mass loads coming from households, small business enterprises and industries in Sweden.
3.1.2 Concentrations and distributions of artificial sweeteners in effluents and excess sludge.
The composition profiles of artificial sweeteners in the effluents are shown in Fig. 3(a) and (b). SUC and ACE were still dominant among the artificial sweeteners and accounted for 53.9% (Plant I) to 96.8% (Plant K) and 2.23% (Plant K) to 36.6% (Plant I) of total artificial sweeteners, respectively. Moreover, the median concentrations of SUC (0.98–3.47 μg L−1), ACE (0.07–3.06 μg L−1), APM (0.03–0.71 μg L−1) and NTM (<LOD-0.04 μg L−1) were 2.55, 0.52, 0.15 and 9.34 × 10−3 μg L−1, respectively. The concentrations of artificial sweeteners in effluents were of the same order as those in the WWTP influents. However, the detection frequencies of these artificial sweeteners in the effluents of the 12 WWTPs were a little bit different from those in influents, because the detection frequency of NTM could not reach up to 100% (91.7%). The concentrations of ACE were close to those in a previous study, which found that the ACE concentrations ranged from 0.97 to 19 μg L−1 in the effluents of WWTPs located in Germany and Switzerland.29 Furthermore, the concentrations of SUC in effluents were comparable to that in Guangzhou of China (0.86–17.0 μg L−1)11 and that in German (0.44–1.53 μg L−1).30 This shows that the artificial sweetener concentrations in the effluents of WWTPs in different regions are basically at the same order of magnitude. Compared to the composition profiles of artificial sweeteners in the effluents, ACE, APM and NTM had much lower detection frequencies of 41.7%, 41.7% and 25.0% in sludge (Fig. 3(c) and (d)). Meanwhile, SUC was detected in the excess sludge with a median concentration of 11.3 ng g−1 (dry weight, dw) and a detection frequency of 91.67%. The high concentrations of SUC in the excess sludge could be ascribed to the high concentrations in the influents. The result is in accordance with the sludge samples of Spain WWTP sewage, where SUC and ACE were the most frequently detected compounds.32 The concentration level found for SUC was similar to that in Sweden (7–429 ng g−1)23 and Switzerland (0.3–19 ng g−1),31 but was lower than the data obtained from WWTPs in Spain (54.0–628 ng g−1)32 and U. S. A.(80–650 ng g−1).2 In addition, SUC, ACE and APM have the highest contents in excess sludge, accounting for 0% to 100%, 0% to 88.7% and 0% to 83.4% of total artificial sweeteners, respectively (Fig. 3(d)).
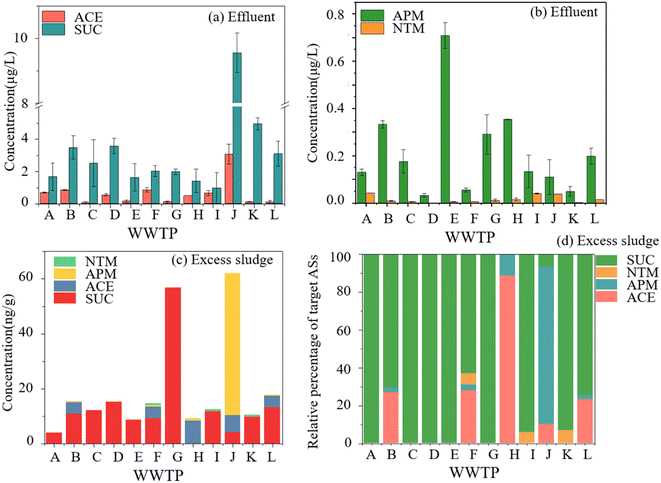 |
| Fig. 3 Composition profiles and mean concentrations (± standard deviations (n = 3)) of artificial sweeteners in the (a) effluents (ACE and SUC), (b) effluents (APM and NTM), (c) composition concentrations and (d) relative percentages of artificial sweeteners in the excess sludge from the 12 WWTPs. | |
3.2 Aqueous removal efficiency of artificial sweeteners
The removal efficiencies of artificial sweeteners are given in Fig. 4. The overall removal efficiency ranged from −116% to 99.1%, which reflected that the removal efficiency varied widely from different artificial sweeteners, and it was probably related to the differences in the composition of sludge.33 The negative removal efficiency may be caused by the release of artificial sweeteners in the mud phase to the aqueous phase and the conversion of intermediate metabolites.34 Subedi and Kannan2 also found the negative removal efficiency (−54.2%) of ACE in the WWTP of Albany Area, New York, U. S. A., and the research results of Li et al.3 showed that the lowest removal efficiency of SUC was nearly −100% in a WWTP of Australia. Among the four artificial sweeteners, the median removal of ACE, APM and NTM was more than 50%, while SUC had a much lower median removal efficiency of 34.6%. Previous studies indicated that SUC can traverse WWTPs with a nearly unchanged concentration, resulting in that the occurrence of SUC in the environment was at a much higher level than several other anthropogenic organic chemicals.10,15 It was likely due to negligible elimination efficiencies of SUC in microbial metabolism. Notably, a previous study indicated that the degradation of ACE was quite slow and inefficient, which was observed in sterile controls.28,35 However, ACE is not generally persistent and can be efficiently degraded through an anaerobic-anoxic-oxic (AAO) process in this study. The different results were probably due to the diverse microbial communities in activated sludge. APM and NTM can also be partially biodegraded during wastewater treatment processes.8,36–38
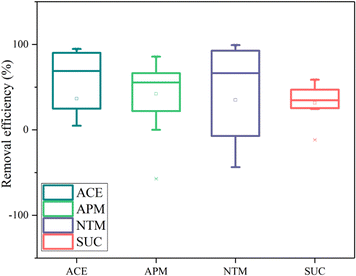 |
| Fig. 4 Removal of the four artificial sweeteners in the 12 WWTPs. | |
3.3 Daily discharge and risk assessment of artificial sweeteners
Table 1 lists the per capita mass loads of artificial sweeteners (mg (d p)−1) discharged from the effluents of the 12 WWTPs to aquatic environments. The total artificial sweetener discharge ranged from 4.53 to 63.77 mg (d p)−1. Among them, the proportions of SUC were greater than 50% (53.87–96.81%), and the sum of the proportions of SUC and ACE exceeded 70% (71.55–99.04%). Although the information about the chronic toxicity of artificial sweeteners is quite rare, considering the high concentrations and persistence of artificial sweeteners, the discharge of artificial sweeteners yet posed a significant risk to aquatic environments. Thence, necessary risk assessment of the artificial sweeteners to aquatic organisms is urgently demanded.33
Table 1 Per capita mass loads of the 4 artificial sweeteners discharged from effluents of the 12 municipal WWTPs to aquatic environments
WWTPs |
SUC (mg (d p)−1) |
ACE (mg (d p)−1) |
ASP (mg (d p)−1) |
NTM (mg (d p)−1) |
Σ Mass (mg (d p)−1) |
WWTP-A |
4.78 |
1.99 |
0.37 |
0.12 |
7.26 |
WWTP-B |
10.84 |
2.65 |
1.04 |
0.03 |
14.55 |
WWTP-C |
6.84 |
0.18 |
0.48 |
0.01 |
7.51 |
WWTP-D |
10.25 |
1.58 |
0.09 |
<LOD |
11.92 |
WWTP-E |
3.09 |
0.31 |
1.34 |
0.01 |
4.75 |
WWTP-F |
5.58 |
2.39 |
0.15 |
0.01 |
8.14 |
WWTP-G |
7.38 |
0.46 |
1.08 |
0.04 |
8.97 |
WWTP-H |
4.71 |
1.66 |
1.18 |
0.05 |
7.59 |
WWTP-I |
2.44 |
1.66 |
0.33 |
0.10 |
4.53 |
WWTP-G |
47.74 |
15.29 |
0.54 |
0.19 |
63.77 |
WWTP-K |
7.99 |
0.18 |
0.08 |
<LOD |
8.25 |
WWTP-L |
12.25 |
0.46 |
0.78 |
0.05 |
13.54 |
In the current study, by using sparse chronic toxicity data, RQ was used to assess the risk of artificial sweeteners to fish, and the results are summarized in Fig. 5. Among the target artificial sweeteners, the maximum RQ value for SUC exceeds 1.0, indicating that SUC might have a high potential risk to aquatic organisms. Previous studies also proved that SUC can have toxicological influence on fish at environmentally relevant concentrations.39 Besides, although ACE had a low risk in most WWTPs, according to the toxicity data, ACE is able to have a longer-term impact on the aquatic environment.40 As for the composite effect, it's difficult to calculate the risk and toxic effects of mixed artificial sweeteners because of the limited toxic data of artificial sweeteners.41 Meanwhile, a previous study found that there was a cumulative effect in developmental toxicity on medaka embryos while combining artificial sweeteners with caffeine.42 Despite these limitations and deficiencies, results from preliminary risk assessment of artificial sweeteners can offer a basic reference for the pollution risk of artificial sweeteners from the investigated WWTP effluents discharged to aquatic environments. Moreover, for ecosystem protection, it's important to propose further elimination for ACE and SUC.
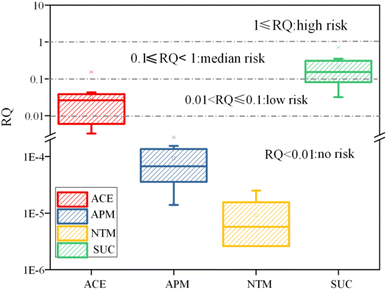 |
| Fig. 5 Risk quotients (RQs) of 4 artificial sweeteners in the 12 WWTP effluents. | |
3.4 Advanced treatments for ACE and SUC removal
In this study, SUC and ACE were considered to be further removed UV/AOPs due to their high residual and potential adverse effect (Fig. 6). As Fig. 6(a) and (b) show, comparing the degradation efficiency of target artificial sweeteners by UV/H2O2 and UV/PDS, artificial sweeteners showed similar oxidation trends during UV/H2O2 and UV/PDS. The degradation of two artificial sweeteners followed pseudo-first-order kinetics (R2 = 0.97–0.99), and the reaction constants of ACE (1.70 × 10−3 s−1 and 5.54 × 10−3 s−1) were higher than those of SUC (0.29 × 10−3 s−1 and 0.77 × 10−3 s−1) during UV/H2O2 and UV/PDS. Notably, SUC had a lower reaction rate because of its low molar extinction coefficients.43 Therefore, SUC is generally used as an ideal probe to measure the effect of UV-based AOPs.44 When artificial sweeteners were degraded by UV/PDS, HO˙ and SO
− played the dominant roles in the reaction. Moreover, compared with HO˙ (1.8–2.7 V),45 SO
− can exhibit higher redox potentials of 2.5–3.1 V over a wide pH range.46,47 Thus, UV/PDS usually shows better removal efficiency than UV/H2O2 at the same mass concentration (34 mg L−1) due to its higher redox potential.
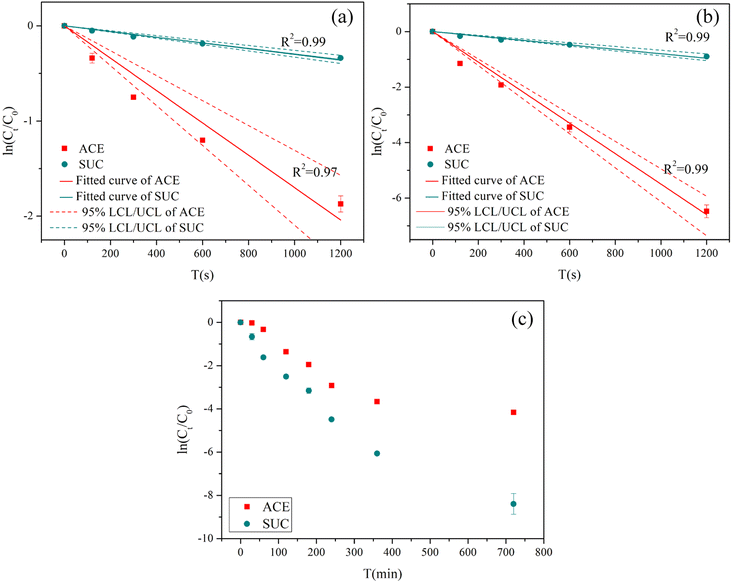 |
| Fig. 6 Degradation process of ACE and SUC by (a) UV/H2O2, (b) UV/PDS and (c) GAC adsorption. | |
Adsorption by GAC was a promising treatment process to remove micropollutants in WWTPs.18Fig. 6(c) shows the adsorption ability of ACE and SUC on GAC. The amount of artificial sweeteners adsorbed was in the order of SUC > ACE, because SUC had a higher octanol–water partition coefficient.31 Mailler et al.48 found that about 26% of SUC could be removed when using a full-scale GAC absorber. According to previous research, the Freundlich coefficient of SUC (78.6 (mg g−1) (L mg−1)1/n, 1/n = 0.364) was higher than that of ACE (51.5 (mg g−1) (L mg−1)1/n, 1/n = 0.238); therefore, the adsorption ability of SUC to GAC would be higher than that of ACE.4,10
By comparing the removal efficiency of artificial sweeteners by advanced treatments, UV/PDS was recommended as the best treatment process for ACE and SUC in wastewater.
4 Conclusion
The occurrence and removal of ACE, APM, NTM and SUC were investigated in 12 WWTPs in China. These four artificial sweeteners were almost 100% detected in the influents and effluents of WWTPs with concentrations ranging from <LOD to 8.62 μg L−1. Among them, SUC and ACE were two predominant artificial sweeteners in influents, effluents and excess sludge. And especially, the per capita mass loads of total artificial sweeteners in southern cities were higher than that in northern cities (p < 0.05). Additionally, there is a significant correlation between the per capita mass load of ACE in influents and population density. Furthermore, by calculation, the total artificial sweeteners ranging from 4.53 to 63.77 mg (d p)−1 still discharged into the environment. And SUC was considered to have a high potential risk to aquatic organisms (RQ > 1.0). UV/H2O2, UV/PDS and GAC adsorption were used to further degrade ACE and SUC. By comparison, the reaction constants and adsorption ability of ACE and SUC in UV-AOPs and GAC were in the order of ACE > SUC and SUC > ACE, respectively. And UV/PDS was recommended as the best treatment process for ACE and SUC. In the future, the mixed toxicity and long-term exposure of artificial sweeteners in WWTPs deserve research to better assess their potential ecological risks and a technology for reducing the ecological risk of artificial sweeteners needs to be developed.
Conflicts of interest
There are no conflicts to declare.
Acknowledgements
This work was supported by the National Science Foundation of China (No. 51978327) and the Jiangsu Natural Science Foundation of China (No. BK20220012 and BK20220785).
References
- H. Lin, N. Oturan, J. Wu, V. K. Sharma, H. Zhang and M. A. Oturan, Removal of Artificial Sweetener Aspartame From Aqueous Media By Electrochemical Advanced Oxidation Processes, Chemosphere, 2017, 167, 220–227 CrossRef CAS PubMed.
- B. Subedi and K. Kannan, Fate of Artificial Sweeteners in Wastewater Treatment Plants in New York State, Environ. Sci. Technol., 2014, 48, 13668–13674 CrossRef CAS PubMed.
- D. Li, J. W. O'Brien, B. J. Tscharke, P. M. Choi, Q. Zheng, F. Ahmed, J. Thompson, J. Li, J. F. Mueller, H. Suna and K. V. Thomas, National Wastewater Reconnaissance of Artificial Sweetener Consumption and Emission in Australia, Environ. Int., 2020, 143, 105963 CrossRef CAS PubMed.
- S. Li, Y. Ren, Y. Fu, X. Gao, C. Jiang, G. Wu, H. Ren and J. Geng, Fate of Artificial Sweeteners Through Wastewater Treatment Plants and Water Treatment Processes, PLoS One, 2018, 13, e0189867 CrossRef PubMed.
- S. M. Praveenaa, M. S. Cheema and H.-R. Guo, Non-Nutritive Artificial Sweeteners as an Emerging Contaminant in Environment: A Global Review and Risks Perspectives, Ecotoxicol. Environ. Saf., 2019, 170, 699–707 CrossRef PubMed.
- D. Li, J. W. O'Brien, B. J. Tscharke, P. M. Choi, F. Ahmed, J. Thompson, J. F. Mueller, H. Sun and K. V. Thomas, Trends in Artificial Sweetener Consumption: A 7-year Wastewater-Based Epidemiology Study in Queensland, Australia, Sci. Total Environ., 2021, 754, 142438 CrossRef CAS PubMed.
- M. G. Cantwell, D. R. Katz, J. Sullivan and A. Kuhn, Evaluation of The Artificial Sweetener Sucralose as a Sanitary Wastewater Tracer in Narragansett Bay, Rhode Island, USA, Mar. Pollut. Bull., 2019, 146, 711–717 CrossRef CAS PubMed.
- S. Kahl, S. Kleinsteuber, J. Nivala, M. v. Afferden and T. Reemtsma, Emerging Biodegradation of the Previously Persistent Artificial Sweetener Acesulfame in Biological Wastewater Treatment, Environ. Sci. Technol., 2018, 52, 2717–2725 CrossRef CAS PubMed.
- J. Oppenheimer, A. Eaton, M. Badruzzaman, A. W. Haghani and J. G. Jacangelo, Occurrence and Suitability of Sucralose as an Indicator Compound of Wastewater Loading to Surface Waters in Urbanized Regions, Water Res., 2011, 45, 4019–4027 CrossRef CAS PubMed.
- L. Soh, K. A. Connors, B. W. Brooks and J. Zimmerman, Fate of Sucralose Through Environmental and Water Treatment Processes and Impact on Plant Indicator Species, Environ. Sci. Technol., 2011, 45, 1363–1369 CrossRef CAS PubMed.
- Z. Gan, H. Sun, B. Feng, R. Wang and Y. Zhang, Occurrence of Seven Artificial Sweeteners in the Aquatic Environment and Precipitation of Tianjin, China, Water Res., 2013, 47, 4928–4937 CrossRef CAS PubMed.
- Y. Yang, W. Liu, Y. Liu, J. Zhao, Q. Zhang, M. Zhang, J. Zhang, Y. Jiang, L. Zhang and G. Ying, Suitability of Pharmaceuticals and Personal Care Products (PPCPs) and Artificial Sweeteners (ASs) as Wastewater Indicators in the Pearl River Delta, South China, Sci. Total Environ., 2017, 590–591, 611–619 CrossRef CAS PubMed.
- A.-K. E. Wiklund, M. Breitholtz, B.-E. Bengtsson and M. Adolfsson-Erici, Sucralose – An Ecotoxicological Challenger?, Chemosphere, 2012, 86, 50–55 CrossRef CAS PubMed.
- G. Dong, X. Li, G. Han, L. Du and M. Li, Zebrafish Neuro-Behavioral Profiles Altered by Acesulfame (ACE) Within the Range of “No Observed Effect Concentrations (NOECs)”, Chemosphere, 2020, 243, 125431 CrossRef CAS PubMed.
- C. I. Torres, S. Ramakrishna, C.-A. Chiu, K. G. Nelson, P. Westerhoff and R. Krajmalnik-Brown, Fate of Sucralose During Wastewater Treatment, Environ. Eng. Sci., 2011, 28, 325–331 CrossRef CAS.
- D. B. Miklos, W.-L. Wang, K. G. Linden, J. E. Drewes and U. Hübner, Comparison of UV-AOPs (UV/H2O2, UV/PDS and UV/Chlorine) for TOrC Removal From Municipal Wastewater Effluent and Optical Surrogate Model Evaluation, Chem. Eng. J., 2019, 362, 537–547 CrossRef CAS.
- H.-W. Yu, M. Park, S. Wu, I. J. Lopez, W. Ji, J. Scheideler and S. A. Snyder, Strategies for Selecting Indicator Compounds to Assess Attenuation of Emerging Contaminants During UV Advanced Oxidation Processes, Water Res., 2019, 166, 115030 CrossRef CAS PubMed.
- A. Rostvall, W. Zhang, W. Dürig, G. Renman, K. Wiberg, L. Ahrens and P. Gago-Ferrero, Removal of Pharmaceuticals, Perfluoroalkyl Substances and Other Micropollutants from Wastewater Using Lignite, Xylit, Sand, Granular Activated Carbon (GAC) and GAC+Polonite((R)) in Column Tests – Role of Physicochemical Properties, Water Res., 2018, 137, 97–106 CrossRef CAS PubMed.
- Y. Fu, G. Wu, J. Geng, J. Li, S. Li and H. Ren, Kinetics and Modeling of Artificial Sweeteners Degradation in Wastewater by the UV/Persulfate Process, Water Res., 2019, 150, 12–20 CrossRef CAS PubMed.
- C. Carlsson, A.-K. Johansson, G. Alvan, K. Bergman and T. Kühler, Are Pharmaceuticals Potent Environmental Pollutants? Part I: Environmental Risk Assessments of Selected Active Pharmaceutical Ingredients, Sci. Total Environ., 2006, 364, 67–87 CrossRef CAS PubMed.
-
E. TGD, Technical Guidance Document on Risk Assessment in Support of Commission Directive 93/67/EEC on Risk Assessment for New Notified Substances, Commission Regulation (EC) No 1488/94 on Risk Assessment for existing substances, and Directive 98/8/EC of the European Parliament and of the Council concerning the placing of biocidal products on the market, Part I–IV, European Chemicals Bureau (ECB), JRC-Ispra (VA), Italy, 2003 Search PubMed.
- M. D. Hernando, M. Mezcua, A. R. Fernández-Alba and D. Barceló, Environmental Risk Assessment of Pharmaceutical Residues in Wastewater Effluents, Surface Waters and Sediments, Talanta, 2006, 69, 334–342 CrossRef CAS PubMed.
- J. Luo, L. Wu, Q. Zhang, Y. Wu, F. Fang, Q. Feng, C. Li, Z. Xue and J. Cao, Review on the Determination and Distribution Patterns of a Widespread Contaminant Artificial Sweetener in the Environment, Environ. Sci. Pollut. Res., 2019, 26, 19078–19096 CrossRef PubMed.
- A. C. Sylvetsky and K. I. Rother, Trends in the Consumption of Low-Calorie Sweeteners, Physiol. Behav., 2016, 164, 446–450 CrossRef CAS PubMed.
- N. H. Tran, J. Hu and S. L. Ong, Simultaneous Determination of PPCPs, EDCs, and Artificial Sweeteners in Environmental Water Samples Using a Single-Step SPE Coupled with HPLC-MS/MS and Isotope Dilution, Talanta, 2013, 113, 82–92 CrossRef CAS PubMed.
- T.-S. S. Neset, H. Singer, P. Longrée, H.-P. Bader, R. Scheidegger, A. Wittmer and J. C. M. Andersson, Understanding Consumption-Related Sucralose Emissions – A Conceptual Approach Combining Substance-Flow Analysis with Sampling Analysis, Sci. Total Environ., 2010, 408, 3261–3269 CrossRef PubMed.
- B. Subedi, S. Lee, H.-B. Moon and K. Kannan, Emission of Artificial Sweeteners, Select Pharmaceuticals, and Personal Care Products Through Sewage Sludge From Wastewater Treatment Plants in Korea, Environ. Int., 2014, 68, 33–40 CrossRef CAS PubMed.
- I. J. Buerge, H.-R. Buser, M. Kahle, M. D. Müller and T. Poiger, Ubiquitous Occurrence of the Artificial Sweetener Acesulfame in the Aquatic Environment: An Ideal Chemical Marker of Domestic Wastewater in Groundwater, Environ. Sci. Technol., 2009, 43, 4381–4385 CrossRef CAS PubMed.
- S. Castronovo, A. Wick, M. Scheurer, K. Nodler, M. Schulz and T. A. Ternes, Biodegradation of the Artificial Sweetener Acesulfame in Biological Wastewater Treatment and Sand filters, Water Res., 2017, 110, 342–353 CrossRef CAS PubMed.
- M. Scheurer, F. R. u. Storck, C. Graf, H.-J. u. Brauch, W. Ruck, O. Levc and F. T. Lange, Correlation of Six Anthropogenic Markers in Wastewater, Surface Water, Bank Filtrate, and Soil Aquifer Treatment, J. Environ. Monit., 2011, 13, 966–973 RSC.
- F. T. Lange, M. Scheurer and H.-J. Brauch, Artificial Sweeteners-A Recently Recognized Class of Emerging Environmental Contaminants: A Review, Anal. Bioanal. Chem., 2012, 403, 2503–2518 CrossRef CAS PubMed.
- E. Y. Ordonez, J. B. Quintana, R. Rodil and R. Cela, Determination of Artificial Sweeteners in Sewage Sludge Samples Using Pressurised Liquid Extraction and Liquid Chromatography-Tandem Mass Spectrometry, J. Chromatogr. A., 2013, 1320, 10–16 CrossRef CAS PubMed.
- J. Luo, Q. Zhang, M. Cao, L. Wu, J. Cao, F. Fang, C. Li, Z. Xue and Q. Feng, Ecotoxicity and Environmental Fates of Newly Recognized Contaminants-Artificial Sweeteners: A Review, Sci. Total Environ., 2019, 653, 1149–1160 CrossRef CAS PubMed.
- J. Cao, B. Fu, T. Zhang, Y. Wu, Z. Zhou, J. Zhao, E. Yang, T. Qian and J. Luo, Fate of Typical Endocrine Active Compounds in Full-Scale Wastewater Treatment Plants: Distribution, Removal Efficiency and Potential Risks, Bioresour. Technol., 2020, 310, 123436 CrossRef CAS PubMed.
- T. Anumol, A. Vijayanandan, M. Park, L. Philip and S. A. Snyder, Occurrence and Fate of Emerging Trace Organic Chemicals in Wastewater Plants in Chennai, India, Environ. Int., 2016, 92–93, 33–42 CrossRef CAS PubMed.
- M. A. R. Cardenas, I. Ali, F. Y. Lai, L. Dawes, R. Thier and J. Rajapakse, Removal of Micropollutants Through a Biological Wastewater Treatment Plant in a Subtropical Climate, Queensland-Australia, J. environ. health sci. eng., 2016, 14, 14 CrossRef PubMed.
- P. Falås, A. Wick, S. Castronovo, J. Habermacher, T. A. Ternes and A. Joss, Tracing the Limits of Organic Micropollutant Removal in Biological Wastewater Treatment, Water Res., 2016, 95, 240–249 CrossRef PubMed.
- N. H. Tran, J. Hu, J. Li and S. L. Ong, Suitability of Artificial Sweeteners as Indicators of Raw Wastewater Contamination in Surface Water and Groundwater, Water Res., 2014, 48, 443–456 CrossRef CAS PubMed.
- K. Saucedo-Vence, A. Elizalde-Velázquez, O. Dublán-García, M. Galar-Martínez, H. Islas-Flores, N. SanJuan-Reyes, S. García-Medina, M. Dolores, Hernández-Navarro and L. M. Gómez-Oliván, Toxicological Hazard Induced by Sucralose to Environmentally Relevant Concentrations in Common Carp (Cyprinus carpio), Sci. Total Environ., 2017, 575, 347–357 CrossRef CAS PubMed.
- Z. Sang, Y. Jiang, Y.-K. Tsoi and K. S.-Y. Leung, Evaluating the Environmental Impact of Artificial Sweeteners: A Study of Their Distributions, Photodegradation and Toxicities, Water Res., 2014, 52, 260–274 CrossRef CAS PubMed.
- S. Stolte, S. Steudte, N. H. Schebb, I. Willenberg and P. Stepnowski, Ecotoxicity of Artificial Sweeteners and Stevioside, Environ. Int., 2013, 60, 123–127 CrossRef CAS PubMed.
- W. Lee and Y. Wang, Assessing Developmental Toxicity of Caffeine and Sweeteners in Medaka (Oryzias latipes), Springerplus, 2015, 4, 486 CrossRef PubMed.
- V. L. Grotz and I. C. Munro, An Overview of the Safety of Sucralose, Regul. Toxicol. Pharmacol., 2009, 55, 1–5 CrossRef CAS PubMed.
- Y. Lester, I. Ferrer, E. M. Thurman and K. G. Linden, Demonstrating Sucralose as a Monitor of Full-Scale UV/AOP Treatment of Trace Organic Compounds, J. Hazard. Mater., 2014, 280, 104–110 CrossRef CAS PubMed.
- H. Lin, J. Wu and H. Zhang, Degradation of Clofibric Acid in Aqueous Solution by an EC/Fe3+/PMS Process, Chem. Eng. J., 2014, 244, 514–521 CrossRef CAS.
- C. Cai, H. Zhang, X. Zhong and L. Hou, Electrochemical Enhanced Heterogeneous Activation of Peroxydisulfate by Fe-Co/SBA-15 Catalyst for the Degradation of Orange II in Water, Water Res., 2014, 66, 473–485 CrossRef CAS PubMed.
- Y. Guan, J. Ma, Y. Ren, Y. Liu, J. Xiao, L. Lin and C. Zhang, Efficient Degradation of Atrazine by Magnetic Porous Copper Ferrite Catalyzed Peroxymonosulfate Oxidation via the Formation of Hydroxyl and Sulfate Radicals, Water Res., 2013, 47, 5431–5438 CrossRef CAS PubMed.
- R. Mailler, J. Gasperi, Y. Coquet, S. Deshayes, S. Zedek, C. Cren-Olivé, N. Cartiser, V. Eudes, A. Bressy, E. Caupos, R. Moilleron, G. Chebbo and V. Rocher, Study of a Large Scale Powdered Activated Carbon Pilot: Removals of a Wide Range of Emerging and Priority Micropollutants from Wastewater Treatment Plant Effluents, Water Res., 2015, 72, 315–330 CrossRef CAS PubMed.
|
This journal is © The Royal Society of Chemistry 2023 |
Click here to see how this site uses Cookies. View our privacy policy here.