Near-source hypochlorous acid emissions from indoor bleach cleaning†
Received
6th October 2022
, Accepted 6th December 2022
First published on 5th January 2023
Abstract
Cleaning surfaces with sodium hypochlorite (NaOCl) bleach can lead to high levels of gaseous chlorine (Cl2) and hypochlorous acid (HOCl); these have high oxidative capacities and are linked to respiratory issues. We developed a novel spectral analysis procedure for a cavity ring-down spectroscopy (CRDS) hydrogen peroxide (H2O2) analyzer to enable time-resolved (3 s) HOCl quantification. We measured HOCl levels in a residential bathroom while disinfecting a bathtub and sink, with a focus on spatial and temporal trends to improve our understanding of exposure risks during bleach use. Very high (>10 ppmv) HOCl levels were detected near the bathtub, with lower levels detected further away. Hypochlorous acid concentrations plateaued in the room at a level that depended on distance from the bathtub. This steady-state concentration was maintained until the product was removed by rinsing. Mobile experiments with the analyzer inlet secured to the researcher's face were conducted to mimic potential human exposure to bleach emissions. The findings from mobile experiments were consistent with the spatial and temporal trends observed in the experiments with fixed inlet locations. This work provides insight on effective strategies to reduce exposure risk to emissions from bleach and other cleaning products.
Environmental significance
Chlorinated species including molecular chlorine (Cl2) and hypochlorous acid (HOCl) emitted during the use of sodium hypochlorite (NaOCl) bleach can spur chemical processes and pose human exposure risks. In this work, we observed HOCl levels of over 10 ppmv near a bathtub surface during disinfection with bleach, over 50 times higher than previously reported. Further, these high levels were sustained until the bleach solution was rinsed down the drain – up to 40 minutes in this work. These findings suggest that cleaning method and proximity to cleaned or disinfected surfaces can greatly affect indoor air composition and human exposure risk. The novel HOCl quantification method we developed also has environmental significance, as it provides an alternative to mass spectrometric methods.
|
1. Introduction
Sodium hypochlorite (NaOCl) bleach is a surface disinfectant commonly used in residential and non-residential buildings for its oxidizing and antimicrobial properties.1–8 Negative health effects associated with bleach cleaning have been documented; frequent use is linked to increased risk of asthma and severe respiratory issues.9–14 The misuse of bleach in domestic and business settings has led to acute health effects and fatal incidents.15,16 Even when used according to manufacturer instructions, emissions from bleach can pose health risks. Hypochlorous acid (HOCl) emitted during bleach use under controlled laboratory and simulated domestic conditions rapidly forms secondary products including molecular chlorine (Cl2),1 reactive nitrogenated and chlorinated compounds such as chloramines, chloroform and carbon tetrachloride,4–6,17–21 and aerosols.22,23 Hypochlorous acid is suspected of causing respiratory irritation, may aggravate pre-existing respiratory issues, and may cause asthma with long-term use.2,5,24,25 It can also cause protein denaturation and radical production,26–28 and can react with unsaturated biomolecules such as squalene to produce toxic chlorohydrins.24,29 Many of the secondary products have also been linked to adverse health effects.16,30–37 It is therefore important to quantify HOCl levels indoors during and following the use of bleach to perform exposure risk assessments.
The first observation of emissions of HOCl from the use of bleach-based cleaners was reported in 2017 using chemical ionization mass spectrometry (CIMS), when a laboratory floor was mopped with an aqueous solution of a commercial bleach product; room-averaged peak HOCl levels on the order of 100 parts per billion by volume (ppbv) were reported.1 Similar levels (15–370 ppbv) of HOCl were reported following mopping the floor of a test house with bleach.17 Finally, cleaning surfaces in a university athletic center with a dichlor (dichloro-s-triazinetrione) spray was reported to form HOCl and other chlorinated species.38 These studies demonstrate that cleaning surfaces with bleach and similar cleaning products can emit high levels of HOCl. However, further study is required to relate observed emissions to exposure.
It is reasonable to suspect that concentrations of emitted species from bleach and other cleaning agents are highest close to the point of use,39 and that spatial distributions within a room or building may be very heterogeneous due to slow mixing of air. In performing exposure risk assessments, it will be important to take total emissions and occupants' positions relative to the cleaned surfaces into account for the duration of elevated HOCl levels following bleach use. In this work, we quantify temporally- and spatially-resolved HOCl mixing ratios when cleaning surfaces in a residential bathroom with a commercial bathroom bleach product. This study introduces a novel cavity ring-down spectroscopy (CRDS) method to conduct time-resolved (∼3 s) HOCl quantification in a domestic setting. This work will improve our understanding of exposure risk to HOCl and secondary products during bleach use and may help establish mitigation strategies.
2. Methods
2.1. Sampling location
Measurements were performed in an apartment bathroom (7.4 m3) in Saskatoon, Saskatchewan, Canada. The unit layout is shown in the ESI (Fig. S1).† Prior to and during cleaning, all apartment windows were closed, all fans in the apartment were turned off, and all interior doors were open unless otherwise noted. Air change rates (ACR) were not measured during these experiments. We assume that the ACR is similar to the reported average of ∼0.5 per hour (h−1) for residential buildings because exterior doors and windows were closed during most experiments.40 There was also no forced air circulation or mechanical ventilation in the unit during cleaning experiments, except for a communal duct from the building constantly venting all bathrooms. Air change rates would likely be driven by indoor–outdoor temperature gradients and leaks in the building envelope and around window and door casements.40
Bathroom lighting was provided by three incandescent light bulbs that were covered by a white, plastic, top-open shade positioned approximately 117 cm above the sink. An air vent was present on the wall at the bathtub rear, 208 cm above the bottom of the bathtub. There were no windows in the bathroom; windows were located in the bedroom and living room (Fig. S1†).
2.2. Cavity ring-down spectroscopy
Gas-phase HOCl mixing ratios were measured in real-time using cavity ring-down spectroscopy (CRDS; Picarro PI2114 Hydrogen Peroxide (H2O2) Analyzer; Picarro Inc.). This instrument was designed to selectively quantify gas-phase H2O2 mixing ratios using the 2ν OH overtone stretch at ∼1400 nm. This overtone absorption is observed when the OH stretch is excited from the ground vibrational state to the 2nd vibrational excited state (ν = 0 to ν = 2). A detailed discussion of cavity-enhanced spectroscopy methods, including CRDS, is provided in Young et al.41 We have previously demonstrated the application of this instrument for indoor air quality research by measuring time-resolved H2O2 mixing ratios during surface cleaning with a commercial H2O2-based cleaning solution.39 The 2ν OH stretch of HOCl is also at ∼1400 nm.42 The software of the PI2114 analyzer was modified to include the HOCl absorption in the least-squares analysis of the absorption spectra acquired by the spectrometer in its normal operating mode.
We are not aware of any quantitative spectroscopy of HOCl in the near infrared. Cavazza et al.42 have analyzed the frequency spectrum of the 2ν1 band, presenting spectroscopic parameters and lists of assigned transitions, but they do not report any line intensities. We therefore acquired our own spectra of HOCl in the frequency region probed by the Picarro PI2114. The work proceeded in two steps. In the first step, a PI2114 instrument was used to acquire high resolution spectra of a vapour sample from the headspace over a solution of sodium hypochlorite in water, as well as spectra of pure water in the same frequency region. Removing the known empty-cavity base line and the absorption due to water vapour yields the HOCl spectrum shown in Fig. S2 in the ESI.† The H2O2 spectrum is also shown in Fig. S2† for comparison. All the strong absorption features of the HOCl spectrum align with frequencies reported by Cavazza et al.42 One sees that the HOCl and H2O2 spectra have some overlapping absorption, but also very distinct features, so it is straightforward to distinguish the absorption of these two compounds by least-squares curve fitting.
The second calibration step enabled HOCl quantitation. Zero air was bubbled through a sodium hypochlorite buffer solution at 50 standard cubic centimetres per minute (sccm) and the flow was diluted with dry zero air to vary the HOCl concentration. Detailed calibration information is provided in the ESI.† The method limit of detection (LOD), calculated as 3σ above the mean signal when well-mixed ambient apartment air was not influenced by cleaning activities and HOCl was assumed to be absent, was determined to be 20 ppbv for unaveraged 3 s resolution data and 10 ppbv for 16 s averages.
This CRDS instrument is, to our knowledge, the only instrument other than CIMS that can quantify HOCl at the ppbv level with temporal resolution of seconds to minutes. The instrument response time measured during calibrations was on the order of 4 s during increases in HOCl mixing ratios on the order of 10 ppbv and ∼10 s during decreases. When adding our PFA inlet in this work, partitioning of HOCl was expected to potentially impact the response time based on the high Henry's Law constant (KH) for HOCl (∼660 M atm−1),43 which would theoretically result in a tubing-driven delay of ∼5–10 s in response time. Our observations of ∼18 s response to the removal of >1 ppmv HOCl from ambient air (see Table 2) are consistent with this, and are similar to predicted CIMS response times for species with similar KH.44 While CIMS has the advantage of being able to speciate numerous chlorine-containing species associated with bleach use, its applicability to indoor measurements is limited by several factors. These include safety concerns due to radioactive ion sources, high expense, and the need for specialized training for operation, maintenance, and data analysis. The CRDS instrument does not suffer from any of these limitations. Further, it is portable, quiet, and can operate with infrequent calibration and maintenance over weeks or months at a time. This modified CRDS instrument is ideal for indoor measurements where minimizing disruption to occupants is desirable. This manuscript demonstrates its applicability to indoor air quality measurements.
2.3. Sampling conditions for hypochlorous acid measurements
The analyzer was located outside of the bathroom, approximately 2.1 m from the open bathroom door (Fig. S1†). Bathroom air was sampled through a 4.7 m PFA tubing inlet with 3/16′′ ID at a flow rate of 1 standard litre per minute (slpm). The sampling inlet was positioned at three different locations in the bathroom for stationary measurements (Table 1) and secured to a pair of glasses worn by the researcher for mobile measurements. When secured to the glasses, the sampling inlet was positioned ∼6 cm in front of the face of the researcher. During mobile measurements, the sampling inlet was secured to the face of the researcher at all times while they were in the bathroom. Reported HOCl mixing ratios during mobile measurements are assumed to be representative of those in inhaled air. When the researcher exited the bathroom during mobile cleaning events, the sampling inlet was placed on the analyzer outside the bathroom.
Table 1 Inlet placement for stationary measurements
Location |
Distance from bathtub floor (cm) |
Inlet location description |
Near-ceiling |
234 |
Approximate centre of bathroom, 203 cm above the floor, 20 cm below the ceiling |
Mid-room |
165 |
Approximate centre of bathroom, 104 cm above the floor, 119 cm below the ceiling |
Near-tub |
33 |
Directly over bathtub, 39 cm above the floor; 184 cm below the ceiling |
2.4. Cleaning activities
A commercial bleach cleaner containing 2.0% w/w sodium hypochlorite (NaOCl) was used to clean bathroom surfaces, with the main focus on the surface of a porcelain bathtub (∼2 m2) in this work. A sufficient volume of cleaning solution was sprayed into the bathtub to fully coat the surface, and additional cleaning solution was applied to areas with visible soiling. The volume applied ranged from 40–85 mL, achieved with 80–170 pumps of the spray bottle. The product was left on the bathtub surface for a minimum of ten minutes, as per the product manufacturer's instructions. The bathtub was then scrubbed with a water-dampened brush which was occasionally rewetted. Scrubbing lasted 4–7 min; the duration was determined by the level of visible soiling of the bathtub surface. After scrubbing, the bathtub was rinsed with tap water (∼2 min) and the resulting solution entered the drain.
Bleach cleaner was also used to clean the sink during stationary and mobile measurements. The sink was cleaned similarly to the bathtub except that smaller volumes of bleach product were used. The entire sink was sprayed with approximately 25–35 mL achieved by 50–70 pumps of bleach product. The solution was left to sit for a minimum of ten minutes. Then the sink was scrubbed with a water-dampened brush and rinsed with tap water. Scrubbing and rinsing lasted ∼4 min, with the resulting solution entered the drain.
2.5. Data processing
The data collected from the CRDS was processed and graphed using IGOR Pro 8.04 (WaveMetrics, Inc.; Portland, USA). All time series presented in this work depict data collected at a time resolution of 3 s. The HOCl decay rate constant (k) was determined by fitting a single exponential decay to the period of decreasing HOCl mixing ratios following their peak for each cleaning event (eqn (1)), where [HOCl]min is the measured background mixing ratio of HOCl, [HOCl]max is the peak mixing ratio, t is time, and tmax is the time at which the peak mixing ratio was observed. | [HOCl]t = [HOCl]min + [HOCl]max exp(k(tmax − t)) | (1) |
3. Results & discussion
3.1. Stationary measurements during bathtub cleaning
Hypochlorous acid mixing ratios increased during bathtub cleaning events regardless of inlet location. Fig. 1 shows representative time series for cleaning events with the inlet at near-ceiling, mid-room, and near-tub locations (Table 1). Irrespective of inlet location, HOCl levels increased and then plateaued following bleach application to the bathtub surface. The liquid coating on the bathtub surface dried over the course of ∼5–10 min, leaving a visible residue. Elevated HOCl levels persisted until the bleach product was removed from the bathtub by scrubbing and rinsing. A sharp increase in HOCl mixing ratios was consistently observed during scrubbing for the near-ceiling and mid-room locations, while no significant increase was observed at the near-tub level. These peak HOCl levels began decreasing within 2 min via a first order exponential decay to below the LOD. These temporal profiles are quite different from those reported in the literature for bleach cleaning; other studies uniformly report a rapid increase of HOCl following bleach application followed by a rapid decrease to below the LOD, with no plateau.1,17 The different temporal profiles may be due to several factors. Previous studies mopped the floor with aqueous sodium hypochlorite solutions and researchers had no further physical interactions with the cleaned surface, whereas we sprayed the solution onto the surface. The composition of the cleaning solutions was also somewhat different. While NaOCl was the active ingredient, additives may affect the partitioning of HOCl. For example, the bleach cleaner used in this work was deposited as a foam that evaporated to leave a thin residue. We hypothesize that the bleach product used in this work left a long-lasting HOCl reservoir on the bathtub surface, and that the observed plateau reflects the steady-state HOCl mixing ratio (with loss terms including dilution, surface partitioning, and removal via air change). The spike in HOCl mixing ratios during scrubbing is likely due to rapid transfer of HOCl to the gas phase. The act of scrubbing mechanically generates increased air movement and high surface area droplets, both promoting HOCl transfer to the gas phase. Cleaning solution is also moved across more of the tub surface during scrubbing, particularly onto the bathtub walls where thin films can form that would undergo rapid evaporation and release of HOCl. The rapid decay in HOCl following scrubbing is due to the removal of the reservoir as the bleach was washed down the drain.
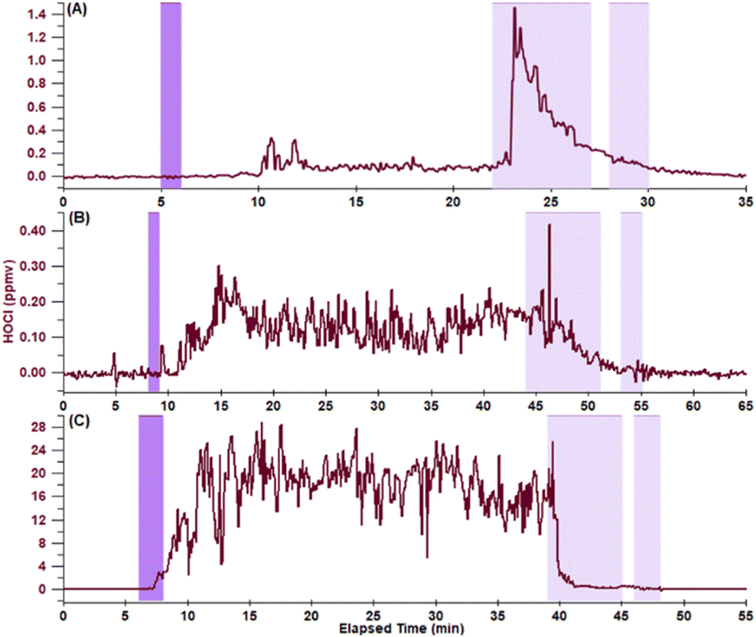 |
| Fig. 1 Representative time series of HOCl mixing ratios during stationary measurements of bathtub cleaning events with the inlet at (A) near-ceiling, (B) mid-room, and (C) near-tub positions. Time intervals during which the cleaner was sprayed onto the bathtub surface are denoted by dark purple, and subsequent time intervals for scrubbing and rinsing of the bathtub (always in that sequence) are denoted by light purple. | |
Assuming that HOCl at the mid-room and near-ceiling locations is well-mixed (see discussion below), we used the rate constant for HOCl loss and the steady-state concentrations (shown in Table 2) to calculate an HOCl emission rate of 0.8 ppbv s−1. The total number of emitted HOCl molecules depends on the duration of the experiment; if the tub is rinsed 10 minutes following application, 8.8 × 1019 molecules will be emitted, while 3.5 × 1020 molecules will be emitted over 40 minutes of exposure. Wong et al.1 reported that 4.2 × 1021 HOCl molecules were emitted following mopping 37 m2 of a floor with ∼200 mL of a bleach solution. As discussed above, the temporal profiles in the two studies were quite different, with no sustained steady-state observed by Wong et al.1 Based on our calculations, ∼4% of total HOCl molecules partition to the air over 40 min exposure in our experiments. This assumes that all OCl− is converted to HOCl due to exposure to CO2 in the air and interaction with acidic species at the air–solid interface. The extent of protonation following application to surfaces is not known, although it has been reported to be much more extensive than in the headspace of an aqueous NaOCl solution.1 For comparison, Wong et al.1 predicted that ∼60% of available HOCl molecules were emitted to the gas phase in their study. In that work, the number of HOCl molecules emitted increased with each consecutive mopping experiment.1 The authors hypothesized that each cleaning event removed reactive materials that acted as HOCl sinks.1 Emitted HOCl levels did not depend on the order of cleaning experiments in the current study. This is likely due to the longer period of at least 9.5 h between cleaning leading to random variations in the level of soiling on the bathtub surface for each experiment.
Table 2 HOCl levels, detection time delays, and HOCl decay kinetics for stationary measurements. “N” is the number of compiled cleaning experiments
Metric |
Near-ceiling (N = 3) |
Mid-room (N = 3) |
Near-tub (N = 2) |
6 data point (16 s) averages centred on the maximum signal.
|
Mean steady-state HOCl (ppbv) |
100 ± 70 |
150 ± 20 |
21 000 ± 4000 |
Peak HOCl during scrubbing (ppbv)a |
910 ± 690 |
220 ± 10 |
23 000 ± 7000 |
Time between spraying bathtub and HOCl detection (min) |
7.3 ± 5.9 |
2.1 ± 0.9 |
2.0 ± 1.0 |
Time between scrubbing bathtub and decrease in HOCl (min) |
2.0 ± 1.7 |
1.9 ± 0.2 |
0.3 ± 0.4 |
HOCl decay rate constant during scrubbing (h−1) |
20 ± 9 |
26 ± 7 |
204 ± 4 |
While the general shapes of the HOCl temporal profiles are similar at each inlet location, Table 2 shows that there were differences in magnitude and timing. Mean steady-state HOCl mixing ratios were the same at the mid-room and near-ceiling positions within experimental uncertainty based on comparison by paired Student's t-test, and were within the range of peak mixing ratios reported elsewhere following floor mopping with bleach solutions (15–370 ppbv).1,17 The mean steady-state mixing ratio of 21 ppmv measured near the bathtub exceeds all prior reported mixing ratios following bleach surface cleaning by at least a factor of 50, and is larger than the mean steady-state mixing ratios measured at the other inlet locations in this work by over a factor of 100. The high HOCl steady-state mixing ratios at the near-tub inlet location suggest that HOCl emitted from the bathtub surface is not well-mixed at this distance. The high decay rate constant of 204 h−1 measured at the near-tub inlet location is likely dominated by dilution, compared to lower rate constants of ∼23 h−1 at the mid-room and near-ceiling locations. Loss at these locations is likely dominated by air change and surface partitioning and/or reaction. The similar steady-state mixing ratios and decay kinetics at the mid-room and near-ceiling locations lead us to hypothesize that air in the bathroom is relatively well-mixed within 2.1 min following cleaning.
While steady-state HOCl mixing ratios were similar at the mid-room and near-ceiling locations, differences were noted in the spikes observed during scrubbing (“peak HOCl during scrubbing” in Table 2). The magnitude of the peak was generally larger at the near-ceiling location with a mean of 910 ppbv compared to 220 ppbv at the mid-room location. The relative increase in HOCl mixing ratio – the peak level compared to the mean steady-state level – was a factor of 8.5 larger at near-ceiling compared to a factor of 1.5 at mid-room. These differences may be due to air being drawn toward the vent mounted near the ceiling, but we do not wish to speculate further here due to the large variability in the magnitude of the peaks at the near-ceiling location (76% relative uncertainty). In comparison, peak HOCl levels agree at the 95% confidence level for the mid-room and near-ceiling locations based on comparison by paired Student's t-test.
3.2. Mobile measurements during bathtub cleaning
To investigate HOCl exposure during cleaning, mobile measurements with the inlet attached to glasses worn by the researcher were performed to measure near-face HOCl mixing ratios (Fig. 2 and Table 3). The HOCl levels were sometimes below the LOD after bleach cleaner application because the researcher left the bathroom within ∼1 min, such that the HOCl could not fully mix in the bathroom air. The HOCl levels were below the LOD when the inlet was outside the bathroom for all mobile experiments. The researcher re-entered the bathroom ∼3 min after bleach application during one experiment; near-face levels of 300 ± 160 ppbv were observed during this period (Fig. 2). Given that only one mid-room measurement was conducted during mobile experiments, we do not speculate on potential differences between mobile and stationary measurements. With that said, it is worth discussing ways in which the presence and actions of a person could affect indoor HOCl concentrations, especially in a relatively small volume of air. Reactions of oxidants, including HOCl, with skin oil can result in a concentration gradient with the lowest oxidant levels near the skin.24,45–47 Other human-related factors could have the opposite effect of increasing near-face concentrations. For example, human thermal plumes facilitate transport from the lower regions of the room towards the face,48 and human movement can increase the extent of local air mixing, reducing mixing time from minutes to seconds and potentially promoting transport of HOCl from the source to more distant regions of the room.49–51 Further study of near-face concentrations of cleaning product emissions should be performed, as they could be quite different (either higher or lower) than concentrations at similar locations in the absence of people. This could be an important consideration in assessing risks of dermal and inhalation exposure.
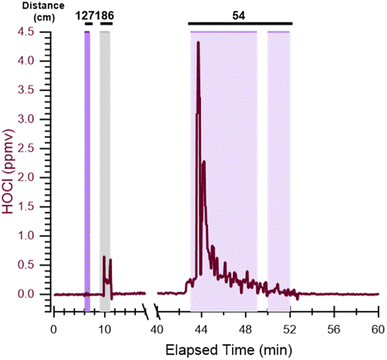 |
| Fig. 2 Representative time series for a mobile bathtub cleaning event. Dark purple denotes when bleach cleaner was sprayed in the bathtub, grey when the researcher entered the bathroom but did not perform cleaning activities, and light purple when the bathtub was scrubbed and then rinsed. The distances (cm) above the highlighted activity indicate the mean distance of the mobile inlet to the bathtub surface. Unshaded regions are when the researcher and inlet were outside the bathroom. | |
Table 3 Mean HOCl mixing ratios during mobile measurements
Activity/location |
Distance from bathtub floor (cm) |
Replicatesa |
HOCl (ppbv) |
Number of data points collectedb |
Numbers in parentheses denote the number of trials in which HOCl levels exceeded the LOD.
Numbers in parentheses denote the total duration (in minutes) of measurements.
Reported HOCl levels are peak levels, calculated the same as in Table 2.
|
Out of bathroom |
>200 |
25 (0) |
<LOD |
2265 (117.8) |
Spraying |
127 |
4 (0) |
<LOD |
96 (4.8) |
Mid-room |
186 |
1 (1) |
300 ± 160 |
26 (1.3) |
Scrubbing and rinsingc |
54 |
4 (4) |
2600 ± 1000 |
24 (1.1) |
As with stationary measurements, the highest near-face HOCl levels were measured during bathtub scrubbing, when HOCl levels spiked to approximately 2.6 ppmv before decaying on the order of 10 min. Peak near-face HOCl levels during scrubbing were approximately 9 times lower than peak HOCl for stationary near-tub measurements. The lower peak HOCl levels measured during mobile experiments are likely due to a combination of the inlet being ∼50 cm above the bathtub surface for mobile measurements compared to 30 cm for stationary measurements, and the inter-experiment heterogeneity observed in the latter (Table 2). These observations suggest that HOCl exposure will depend on the person's proximity to the bleach source, time spent inside the space where bleach is applied, the release of HOCl during scrubbing, and loss to surfaces and rinsing the solution down the drain.
3.3. HOCl measurements during sink cleaning
Mixing ratios of HOCl were also measured during the cleaning of a 0.3 m2 porcelain sink (Table S3†). One stationary measurement with the inlet at the near-ceiling position (180 cm from the sink) was performed during which the HOCl mixing ratios remained below the LOD. Three mobile measurements were performed, with the inlet 60–90 cm from the sink surface during spraying and scrubbing. The mean mixing ratio during spraying was 17 ± 15 ppbv (over a total of 76 data points), which was below the LOD of 20 ppbv. However, HOCl levels frequently exceeded the LOD, indicating that HOCl was emitted from the sink surface. The low levels emitted from the sink compared to the bathtub are likely due to the smaller surface area being cleaned and the smaller volume of bleach product applied. Mixing ratios increased during scrubbing with a peak level of 370 ± 330 ppbv. This is much smaller than peak levels of 2 ppmv observed during mobile bathtub cleaning experiments. These results suggest that exposure risk can be substantially reduced by cleaning smaller surface areas at any one time and/or leaving the room.
4. Implications
We have modified a portable H2O2 CRDS to quantify time resolved HOCl levels with a detection limit of 20 ppbv at 3 s time resolution and 10 ppbv for 16 s averages. This instrument is simple to use, easily transportable, and requires little attention over weeks or months of continuous measurements. The small footprint and low noise generated from the vacuum pump also make this instrument ideal for use in occupied spaces. The presented method provides unprecedented capabilities to study indoor atmospheric processes in occupied spaces where bleach solutions are used.
Measurements in an occupied residence provide insight into exposure during common cleaning practices with a bleach-based product. Measurements at three distances from the bathtub illustrate spatial and temporal heterogeneity of HOCl levels within the bathroom. After bleach application, HOCl mixing ratios remained elevated at all measured distances for up to 40 minutes until the bathtub was scrubbed and rinsed. Mixing ratios measured more than 1 m from the bathtub surface were consistent with reported concentrations in well-mixed rooms.1,17 However, HOCl levels closest to the point of application reached peak levels of approximately 20 ppmv, more than 50 times higher than those previously reported. Similar results were obtained during mobile measurements during which the instrument inlet was affixed near the researcher's face, with HOCl levels of ∼3 ppmv detected when the researcher's face was within 1 m of the bottom of the bathtub. These results demonstrate that exposure to HOCl and other potentially harmful reaction products from bleach use can vary greatly even within a small room. People who spend time in close proximity to surfaces to which bleach is applied, such as janitorial and cleaning staff, will be exposed to much higher levels compared to other people in the same room, even if they are only separated by a few metres. Our results suggest that the largest exposures will be during scrubbing of bleached surfaces due to close proximity of the person cleaning the surface combined with increased HOCl levels during scrubbing.
This work demonstrates the importance of cleaning methods on exposure outcomes. Previous studies, in which floors were mopped with a dilute bleach solution, showed a sharp rise in HOCl levels followed by a rapid decay.1,17 In those experiments, the total duration of elevated HOCl levels was on the order of 5–20 min, whether the experiment took place in a laboratory (ACR = 13 h−1) or a test house (ACR = 0.6 h−1).1,17 The protocol for disinfecting (rather than cleaning) with bleach, as denoted by the Centers for Disease Control and Prevention (CDC) recommends a surface contact time of at least 1 min, while the manufacturer of the product used in this work recommended a minimum of 10 min.52 Our results demonstrate that high steady-state HOCl levels will be maintained until the bleach solution is removed from the surface through scrubbing, rinsing, and/or evaporation. In the bathroom used in these experiments, the steady-state HOCl levels observed imply a balance between emission and loss processes, such as surface deposition/reaction and ventilation over time periods of at least 40 min.
This work provides important insight into HOCl exposure during common cleaning practices. It will be important to relate exposure to health risk to determine the urgency of implementing harm mitigation strategies. The effects of HOCl exposure on human health are not well known. Hypochlorous acid is suspected of causing skin irritation, and has been reported to react with squalene, forming toxic chlorohydrins at 600 ppbv, which further suggests its potential for skin irritation.24 Inhalation risks for this species have not yet been reported.2,53 Based on our observations, we suggest that more research into the health effects of gas-phase HOCl should be undertaken and that occupational long- and short-term exposure limits should be established. It is also important to note that while HOCl was the only analyte quantified in this study, numerous other chlorinated and nitrogenated compounds and volatile organic compounds (VOCs) are emitted when bleach is applied to surfaces.4,6,17–21 A concerning concomitant emission is Cl2, which has been reported to attain peak mixing ratios around 20% of those observed simultaneously for HOCl following mopping floors with bleach.1,17 Chlorine gas can be lethal after ∼1 h exposure at >30 ppmv, generating corrosive compounds capable of dissolving lung tissue; it can also severely damage the eyes and skin.16,54 Recommended/permissible acute 15 minute exposure limits for Cl2 range from 0.4–1 ppmv.54 Assuming similar temporal and spatial trends for Cl2 as HOCl in this work,1,17,55 we might expect sustained Cl2 levels as high as 4 ppmv near surfaces on which bleach has been applied. This could easily result in 15-minute exposure exceedances when bleach is used as a disinfectant. While further study into exposure risks is needed, we suggest reducing exposure to bleach emissions by limiting the time such solutions reside on surfaces (e.g., 1 min CDC recommendation for disinfection), using products in well ventilated spaces as recommended by the manufacturer, and limiting time spent in rooms where bleach has been applied to surfaces.
Conflicts of interest
There are no conflicts to declare.
Acknowledgements
We thank Jay Dave for assistance with data visualization. This work was funded by the Alfred P. Sloan Foundation Chemistry of Indoor Environments (CIE) Program (Awards G-2018-11062, G-2018-11051, and G-2019-11404) and by National Science and Engineering Research Council of Canada (NSERC) Discovery Grants (RGPIN-2019-04868, RGPIN-2020-06166, and RGPIN-2017-05972). Dr Kahan is a Canada Research Chair in Environmental Analytical Chemistry. This research was undertaken, in part, thanks to funding from the Canada Research Chairs Program.
References
- J. P. S. Wong, N. Carslaw, R. Zhao, S. Zhou and J. P. D. Abbatt, Observations and Impacts of Bleach Washing on Indoor Chlorine Chemistry, Indoor Air, 2017, 27(6), 1082–1090 CrossRef PubMed.
- F. Racioppi, P. A. Daskaleros, N. Besbelli, A. Borges, C. Deraemaeker, S. I. Magalini, R. Martinez Arrifta, C. Pulce, M. L. Ruggerone and P. Vlachos, Household Bleaches Based on Sodium Hypochlorite: Review of Acute Toxicology and Poison Control Center Experience, Food Chem. Toxicol., 1994, 32(9), 845–861 CrossRef CAS PubMed.
- J.-P. P. Zock, E. Plana, J. M. Antó, G. Benke, P. D. Blanc, A. Carosso, A. Dahlman-Höglund, J. Heinrich, D. Jarvis, H. Kromhout, L. L. Lillienberg, M. C. Mirabelli, D. Norbäck, M. Olivieri, M. Ponzio, K. Radon, A. Soon, M. van Sprundel, J. Sunyer, C. Svanes, K. Torén, G. Verlato, S. Villani, M. Kogevinas, J. Knox, C. Luczynska, J. Potts, S. Arinze, P. Burney, I. Cerveri, S. Chinn, R. de Marco, T. Gislason, C. Janson, N. Künzli, B. Leynaert, F. Neukirch, J. Schouten, P. Vermeire, M. Wjst, J. Weyler, V. Nelen, R. Jogi, R. Liard, M. Zureik, I. Pin, J. Ferran-Quentin, C. Frye, I. Meyer, M. Bugiani, P. Piccioni, W. Arossa, E. Caria, G. Castiglioni, E. Migliore, C. Romano, D. Fabbro, G. Ciccone, C. Magnani, P. Dalmasso, R. Bono, G. Gigli, A. Giraudo, M. C. Brussino, C. Bucca, G. Rolla, E. Zanolin, S. Accordini, A. Poli, V. LoCascio, M. Ferrari, A. Marinoni, F. Frigerio, M. Comelli, M. Grassi, A. Corsico, A. Gulsvik, E. Omenaas, B. Laerum, X. Basagana, A. Jaen, F. Burgos, J. Maldonado, A. Pereira, J. L. Sanchez, J. Martinez-Moratalla Rovira, E. Almar, N. Muniozguren, I. Urritia, F. Payo, G. Boman, D. Norback, M. Gunnbjornsdottir, K. Toren, R. Sundberg, E. Norrman, M. Soderberg, K. Franklin, B. Lundback, B. Forsberg, L. Nystrom, B. Dibbert, M. Hazenkamp, M. Brutsche, U. Ackermann-Liebrich, B. Harrison, R. Hall and D. Seaton, Domestic Use of Hypochlorite Bleach, Atopic Sensitization, and Respiratory Symptoms in Adults, J. Allergy Clin. Immunol., 2009, 124(4), 731–738 CrossRef CAS PubMed.
- W. W. Nazaroff and C. J. Weschler, Cleaning Products and Air Fresheners: Exposure to Primary and Secondary Air Pollutants, Atmos. Environ., 2004, 38(18), 2841–2865 CrossRef CAS.
- W. A. Rutala and D. J. Weber, Uses of Inorganic Hypochlorite (Bleach) in Health-Care Facilities, Clin. Microbiol. Rev., 1997, 10(4), 597–610 CrossRef CAS PubMed.
- J. P. D. Abbatt and C. Wang, The Atmospheric Chemistry of Indoor Environments, Environ. Sci.: Processes Impacts, 2020, 22(1), 25–48 RSC.
- Z. Han, E. Pappas, A. Simmons, J. Fox, C. J. Donskey and A. Deshpande, Environmental Cleaning and Disinfection of Hospital Rooms: A Nationwide Survey, Am. J. Infect. Control, 2021, 49(1), 34–39 CrossRef PubMed.
- A. D. Woolf and E. Flynn, Workplace Toxic Exposures Involving Adolescents Aged 14 to 19 Years: One Poison Center's Experience, Arch. Pediatr. Adolesc. Med., 2000, 154(3), 234–239 CrossRef CAS PubMed.
- M. Nickmilder, S. Carbonnelle and A. Bernard, House Cleaning with Chlorine Bleach and the Risks of Allergic and Respiratory Diseases in Children, Pediatr. Allergy Immunol., 2007, 18(1), 27–35 CrossRef PubMed.
- B. Matulonga, M. Rava, V. Siroux, A. Bernard, O. Dumas, I. Pin, J.-P. Zock, R. Nadif, B. Leynaert and N. Le Moual, Women Using Bleach for Home Cleaning Are at Increased Risk of Non-Allergic Asthma, Respir. Med., 2016, 117, 264–271 CrossRef PubMed.
- L. Xudong, L. Xiang Qian, C.-Y. W. Claudie, T. Lixing, Z. Zilong, W. Tze Wai, T. Lap-ah, P. S. L. Arthur and T. S. Y. Ignatius, Frequent Use of Household Cleaning Products Is Associated with Rhinitis in Chinese Children, J. Allergy Clin. Immunol., 2016, 138(3), 754–760 CrossRef PubMed.
- L. Casas, A. Espinosa, A. Borràs-Santos, J. Jacobs, E. Krop, D. Heederik, B. Nemery, J. Pekkanen, A. Hyvärinen, M. Täubel and J.-P. Zock, Domestic Use of Bleach and Infections in Children: A Multicentre Cross-Sectional Study, Occup. Environ. Med., 2015, 72(8), 602–604 CrossRef PubMed.
- I. Folletti, J.-P. Zock, G. Moscato and A. Siracusa, Asthma and Rhinitis in Cleaning Workers: A Systematic Review of Epidemiological Studies, J. Asthma, 2014, 51(1), 18–28 CrossRef CAS PubMed.
- A. N. Garrido, R. House, J. C. Lipszyc, G. M. Liss, D. L. Holness and S. M. Tarlo, Cleaning Agent Usage in Healthcare Professionals and Relationship to Lung and Skin Symptoms, J. Asthma, 2021, 59(4), 673–681 CrossRef PubMed.
- D. D. Gummin, J. B. Mowry, M. C. Beuhler, D. A. Spyker, A. C. Bronstein, L. J. Rivers, N. P. T. Pham and J. Weber, 2020 Annual Report of the American Association of Poison Control Centers' National Poison Data System (NPDS): 38th Annual Report, Clin. Toxicol., 2021, 59(12), 1282–1501 CrossRef PubMed.
-
L. K. Boerner, Accidental Mix of Bleach and Acid Kills Buffalo Wild Wings Employee, Chemical and Engineering News, vol. 97(45), 2019 Search PubMed.
- J. M. Mattila, P. S. J. Lakey, M. Shiraiwa, C. Wang, J. P. D. Abbatt, C. Arata, A. H. Goldstein, L. Ampollini, E. F. Katz, P. F. Decarlo, S. Zhou, T. F. Kahan, F. J. Cardoso-Saldaña, L. H. Ruiz, A. Abeleira, E. K. Boedicker, M. E. Vance and D. K. Farmer, Multiphase Chemistry Controls Inorganic Chlorinated and Nitrogenated Compounds in Indoor Air during Bleach Cleaning, Environ. Sci. Technol., 2020, 54(3), 1730–1739 CrossRef CAS PubMed.
- M. Odabasi, Halogenated Volatile Organic Compounds from the Use of Chlorine-Bleach-Containing Household Products, Environ. Sci. Technol., 2008, 42(5), 1445–1451 CrossRef CAS PubMed.
- M. Odabasi, T. Elbir, Y. Dumanoglu and S. C. Sofuoglu, Halogenated Volatile Organic Compounds in Chlorine-Bleach-Containing Household Products and Implications for Their Use, Atmos. Environ., 2014, 92, 376–383 CrossRef CAS.
- C. Wang, J. M. Mattila, D. K. Farmer, C. Arata, A. H. Goldstein and J. P. D. Abbatt, Behavior of Isocyanic Acid and Other Nitrogen-Containing Volatile Organic Compounds in The Indoor Environment, Environ. Sci. Technol., 2022, 56(12), 7598–7607 CrossRef CAS PubMed.
- A. Moravek, T. C. VandenBoer, D. Pagonis, B. A. Nault, Z. Finewax, D. A. Day, A. V. Handschy, H. Stark, P. Ziemann, J. L. Jimenez, J. A. de Gouw and C. J. Young, Reactive Chlorine Chemistry in an Athletic Facility in the Presence and Absence of Cleaning, Environ. Sci. Technol., 2022, 56(22), 15408–15416 CrossRef CAS PubMed.
- J. M. Mattila, C. Arata, C. Wang, E. F. Katz, A. Abeleira, Y. Zhou, S. Zhou, A. H. Goldstein, J. P. D. Abbatt, P. F. Decarlo and D. K. Farmer, Dark Chemistry during Bleach Cleaning Enhances Oxidation of Organics and Secondary Organic Aerosol Production Indoors, Environ. Sci. Technol. Lett., 2020, 7(11), 795–801 CrossRef CAS.
- C. Wang, D. B. Collins and J. P. D. Abbatt, Indoor Illumination of Terpenes and Bleach Emissions Leads to Particle Formation and Growth, Environ. Sci. Technol., 2019, 53(20), 11792–11800 CrossRef CAS PubMed.
- H. Schwartz-Narbonne, C. Wang, S. Zhou, J. P. D. Abbatt and J. Faust, Heterogeneous Chlorination of Squalene and Oleic Acid, Environ. Sci. Technol., 2019, 53(3), 1217–1224 CrossRef CAS PubMed.
-
The National Institute for Occupational Safety and Health (NIOSH), High-Level Disinfectants, Health and Safety Practices Survey of Healthcare Workers, https://www.cdc.gov/niosh/topics/healthcarehsps/disinfect.html Search PubMed.
- J. M. Pullar, M. C. M. Vissers and C. C. Winterbourn, Living with a Killer: The Effects of Hypochlorous Acid on Mammalian Cells, IUBMB Life, 2000, 50(4–5), 259–266 CrossRef CAS PubMed.
- C. L. Hawkins and M. J. Davies, Hypochlorite-Induced Oxidation of Proteins in Plasma: Formation of Chloramines and Nitrogen-Centred Radicals and Their Role in Protein Fragmentation, Biochem. J., 1999, 340(Pt 2), 539–548 CrossRef CAS PubMed.
- O. M. Panasenko, I. V. Gorudko and A. V. Sokolov, Hypochlorous Acid as a Precursor of Free Radicals in Living Systems, Biochemistry, 2014, 78(13), 1466–1489 Search PubMed.
- C. C. Winterbourn, J. J. M. van den Berg, E. Roitman and F. A. Kuypers, Chlorohydrin Formation from Unsaturated Fatty Acids Reacted with Hypochlorous Acid, Arch. Biochem. Biophys., 1992, 296(2), 547–555 CrossRef CAS PubMed.
- N. Massin, A. B. Bohadana, P. Wild, M. Héry, J. P. Toamain and G. Hubert, Respiratory Symptoms and Bronchial Responsiveness in Lifeguards Exposed to Nitrogen Trichloride in Indoor Swimming Pools, Occup. Environ. Med., 1998, 55(4), 258–263 CrossRef CAS PubMed.
- K. M. Thickett, J. S. McCoach, J. M. Gerber, S. Sadhra and P. S. Burge, Occupational Asthma Caused by Chloramines in Indoor Swimming-Pool Air, Eur. Respir. J., 2002, 19(5), 827–832 CrossRef CAS PubMed.
- B. Dang, L. Chen, C. Mueller, K. H. Dunn, D. Almaguer, J. L. Roberts and C. S. Otto, Ocular and Respiratory Symptoms Among Lifeguards at a Hotel Indoor Waterpark Resort, J. Occup. Environ. Med., 2010, 52(2), 207–213 CrossRef CAS PubMed.
- A. Nel, Air Pollution-Related Illness: Effects of Particles, Science, 2005, 308(5723), 804–806 CrossRef CAS PubMed.
- M. Shiraiwa, K. Ueda, A. Pozzer, G. Lammel, C. J. Kampf, A. Fushimi, S. Enami, A. M. Arangio, J. Fröhlich-Nowoisky, Y. Fujitani, A. Furuyama, P. S. J. Lakey, J. Lelieveld, K. Lucas, Y. Morino, U. Pöschl, S. Takahama, A. Takami, H. Tong, B. Weber, A. Yoshino and K. Sato, Aerosol Health Effects from Molecular to Global Scales, Environ. Sci. Technol., 2017, 51(23), 13545–13567 CrossRef CAS PubMed.
- S. von Klot, G. Wolke, T. Tuch, J. Heinrich, D. W. Dockery, J. Schwartz, W. G. Kreyling, H. E. Wichmann and A. Peters, Increased Asthma Medication Use in Association with Ambient Fine and Ultrafine Particles, Eur. Respir. J., 2002, 20(3), 691–702 CrossRef CAS PubMed.
- C. A. Pope III, R. T. Burnett, M. J. Thun, E. E. Calle, D. Krewski, K. Ito and G. D. Thurston, Lung Cancer, Cardiopulmonary Mortality, and Long-Term Exposure to Fine Particulate Air Pollution, JAMA, 2002, 287(9), 1132–1141 CrossRef PubMed.
- N. Li, C. Sioutas, A. Cho, D. Schmitz, C. Misra, J. Sempf, M. Wang, T. Oberley, J. Froines and A. Nel, Ultrafine Particulate Pollutants Induce Oxidative Stress and Mitochondrial Damage, Environ. Health Perspect., 2003, 111(4), 455–460 CrossRef CAS PubMed.
- Z. Finewax, D. Pagonis, M. S. Claflin, A. V. Handschy, W. L. Brown, O. Jenks, B. A. Nault, D. A. Day, B. M. Lerner, J. L. Jimenez, P. J. Ziemann and J. A. de Gouw, Quantification and Source Characterization of Volatile Organic Compounds from Exercising and Application of Chlorine-Based Cleaning Products in a University Athletic Center, Indoor Air, 2021, 31(5), 1323–1339, DOI:10.1111/ina.12781.
- S. Zhou, Z. Liu, Z. Wang, C. J. Young, T. C. Vandenboer, B. B. Guo, J. Zhang, N. Carslaw and T. F. Kahan, Hydrogen Peroxide Emission and Fate Indoors during Non-Bleach Cleaning: A Chamber and Modeling Study, Environ. Sci. Technol., 2020, 54(24), 15643–15651 CrossRef CAS PubMed.
- W. W. Nazaroff, Residential Air-change Rates: A Critical Review, Indoor Air, 2021, 31(2), 282–313 CrossRef PubMed.
-
C. J. Young, R. A. Washenfelder and S. S. Brown, Cavity Enhanced Spectroscopy: Applications Theory and Instrumentation, in Encyclopedia of Analytical Chemistry, John Wiley & Sons, Ltd, 2011 Search PubMed.
- F. Cavazza, G. Dilonardo, R. Escribano, L. Fusina, P. C. Gomez and J. Ortigoso, The 2ν1 Band of HOCl, J. Mol. Spectrosc., 1993, 159(2), 395–409 CrossRef CAS.
-
J. B. Burkholder, S. P. Sander, J. P. D. Abbatt, J. R. Barker, R. E. Huie, C. E. Kolb, M. J. Kurylo, V. L. Orkin, D. M. Wilmouth and P. H. Wine, Chemical Kinetics and Photochemical Data for Use in Atmospheric Studies: Evaluation Number 18, 6th edn, Root, https://hdl.handle.net/2014/45510 Search PubMed.
- X. Liu, B. Deming, D. Pagonis, D. A. Day, B. B. Palm, R. Talukdar, J. M. Roberts, P. R. Veres, J. E. Krechmer, J. A. Thornton, J. A. de Gouw, P. J. Ziemann and J. L. Jimenez, Effects of Gas-Wall Interactions on Measurements of Semivolatile Compounds and Small Polar Molecules, Atmos. Meas. Tech., 2019, 12(6), 3137–3149 CrossRef CAS.
- A. Wisthaler and C. J. Weschler, Reactions of Ozone with Human Skin Lipids: Sources of Carbonyls, Dicarbonyls, and Hydroxycarbonyls in Indoor Air, Proc. Natl. Acad. Sci. U. S. A., 2010, 107(15), 6568–6575 CrossRef CAS PubMed.
- S. Zhou, M. W. Forbes, Y. Katrib and J. P. D. Abbatt, Rapid Oxidation of Skin Oil by Ozone, Environ. Sci. Technol. Lett., 2016, 3(4), 170–174 CrossRef CAS.
- L. S. Pandrangi and G. C. Morrison, Ozone Interactions with Human Hair: Ozone Uptake Rates and Product Formation, Atmos. Environ., 2008, 42(20), 5079–5089 CrossRef CAS.
- S. Sun, J. Li and J. Han, How Human Thermal Plume Influences Near-Human Transport of Respiratory Droplets and Airborne Particles: A Review, Environ. Chem. Lett., 2021, 19(3), 1971–1982 CrossRef CAS PubMed.
- J. Hang, Y. Li and R. Jin, The Influence of Human Walking on the Flow and Airborne Transmission in a Six-Bed Isolation Room: Tracer Gas Simulation, Build. Environ., 2014, 77, 119–134 CrossRef PubMed.
- Z. Han, G. N. Sze To, S. C. Fu, C. Y.-H. Chao, W. Weng and Q. Huang, Effect of Human Movement on Airborne Disease Transmission in an Airplane Cabin: Study Using Numerical Modeling and Quantitative Risk Analysis, BMC Infect. Dis., 2014, 14(1), 434 CrossRef PubMed.
- A. Bhattacharya, J. Pantelic, A. Ghahramani and E. S. Mousavi, Three-Dimensional Analysis of the Effect of Human Movement on Indoor Airflow Patterns, Indoor Air, 2021, 31(2), 587–601 CrossRef PubMed.
-
Centers for Disease Control and Prevention (CDC), Cleaning and Disinfecting with Bleach and Water, https://www.cdc.gov/healthywater/hygiene/disinfecting-bleach.html Search PubMed.
- Y. Ha, Y. Kim, E. Song, H. J. Yoo and J. H. Kwon, Development of a Personal Passive Air Sampler for Estimating Exposure to Effective Chlorine While Using Chlorine-Based Disinfectants, Indoor Air, 2021, 31(2), 557–565 CrossRef PubMed.
-
Chlorine, SDS No. 001015, Airgas USA, Radnor, PA, 2021 Search PubMed.
- P. S. J. Lakey, Y. Won, D. Shaw, F. F. Østerstrøm, J. Mattila, E. Reidy, B. Bottorff, C. Rosales, C. Wang, L. Ampollini, S. Zhou, A. Novoselac, T. F. Kahan, P. F. DeCarlo, J. P. D. Abbatt, P. S. Stevens, D. K. Farmer, N. Carslaw, D. Rim and M. Shiraiwa, Spatial and Temporal Scales of Variability for Indoor Air Constituents, Commun. Chem., 2021, 4(1), 1–7 CrossRef.
Footnotes |
† Electronic supplementary information (ESI) available. See DOI: https://doi.org/10.1039/d2em00405d |
‡ ADS and ML contributed equally to this work and have the right to list themselves as first author. |
§ Currently at School of Environmental Science and Engineering, Southern University of Science and Technology, Shenzhen, Guangdong Province, 518055, China. |
|
This journal is © The Royal Society of Chemistry 2023 |
Click here to see how this site uses Cookies. View our privacy policy here.