DOI:
10.1039/D3FO00328K
(Paper)
Food Funct., 2023,
14, 5962-5976
A systematic review of the inhibitory effect of extracts from edible parts of nuts on α-glucosidase activity†
Received
24th January 2023
, Accepted 20th May 2023
First published on 12th June 2023
Abstract
An elevated postprandial glycaemic response is a risk factor for developing type 2 diabetes mellitus (T2DM). Inhibition of digestive enzymes, including membrane-bound brush-border α-glucosidases, leads to slowed carbohydrate digestion and absorption, and reduced postprandial glycaemia. Nuts are eaten widely around the world, and have the potential to inhibit α-glucosidases through their content of polyphenols and other bioactive compounds. We set out to conduct a systematic literature review exploring the inhibitory effect of extracts from edible parts of various nuts on α-glucosidase activity in vitro to ensure, as far as possible, that no papers were missed. After an initial screening, 38 studies were reviewed in full, of which 15 were suitable for the present systematic review. Notably, no studies were found which tested the inhibitory potential of nut extracts against human α-glucosidases. Two studies showed that extracts from almonds and hazelnuts inhibited rat α-glucosidase activity, but the remaining papers reported data on the yeast α-glucosidase enzyme. Where yeast and rat enzymes can be compared, it is clear that nut extracts inhibit yeast α-glucosidase more strongly than mammalian α-glucosidase, which may lead to over-estimation when predicting effects in vivo when using data from the yeast enzyme. In contrast, acarbose is a stronger inhibitor of mammalian α-glucosidase compared to the yeast enzyme. Thus, although the present review indicates that extracts from nuts inhibit yeast α-glucosidase, this cannot be extrapolated to humans in vivo. There is some evidence that extracts from almonds and hazelnuts inhibit rat α-glucosidase, but no information on human enzyme sources. Since most work has been published on the yeast enzyme, future work in vitro must utilise mammalian, and preferably human, α-glucosidases in order to be relevant to human health and disease. This systematic review was registered at INPLASY as INPLASY202280061.
1. Introduction
An elevated postprandial glycaemic response is a risk factor, and one of the initial signs, of type 2 diabetes mellitus (T2DM) that leads to increased oxidative stress and inflammation at various sites of the body.1 Lowering the postprandial glycaemic response is an important dietary strategy to reduce the risk of T2DM.2–4 Slowing down carbohydrate digestion by inhibition of salivary/pancreatic α-amylases and membrane-bound brush-border α-glucosidases has been shown to lower postprandial blood glucose responses.5 Carbohydrates are rapidly digested by α-amylases and α-glucosidases to produce monosaccharides that are absorbed in the small intestine into the blood. In the human small intestine, there are two brush border enzymes, maltase/glucoamylase and sucrase/isomaltase, exhibiting three α-glucosidase activities: maltase (hydrolysis of α-1,4-linkages between short chains of glucose), sucrase (hydrolysis of α-1,2-linkages between glucose and fructose as in sucrose) and isomaltase (hydrolysis of α-1,6-linkages between two glucose molecules). Dietary starches are hydrolysed firstly by salivary α-amylases, then by pancreatic α-amylases, and finally by intestinal brush border α-glucosidases to ultimately produce glucose.6,7 Thus, the activities of these enzymes are a critical step in determining the postprandial glycaemic response, which can be attenuated by inhibition of these enzymes. Some anti-diabetic drugs, such as acarbose, are used to inhibit α-glucosidase to control postprandial glycaemia,8,9 and it has been reported that various plant extracts can also inhibit α-glucosidase activity.10–12
Nut consumption is recommended in dietary guidelines worldwide because of health benefits.13–15 Studies have shown that nut consumption lowers the risk of developing T2DM,16 partly attributed to α-glucosidase activity inhibition.17,18 While several studies have indicated that consuming nuts is associated with a decreased risk of type 2 diabetes and metabolic syndrome,19–23 there are some other studies which did not find any correlation between consuming nuts and a risk reduction.24–26 According to a prospective cohort study,22 individuals who ate 28 g of nuts 1–4 times per week, or >5 times per week, had a 16% and 27% statistically significant lower risk of diabetes, respectively, compared to those who rarely or never consumed nuts. As reported by Asghari et al.,23 individuals who consumed 2–4 or ≥4 servings of nuts per week had a 49% and 53% lower risk of diabetes, respectively, in comparison to those who consumed <1 serving per week.
Nuts have a high total fat content (ranging from 44.4 g to 75.8 g per 100 g whole raw nut), but they are low in saturated fatty acids (3.8 g to 16.1 g per 100 g whole raw nut).27,28 They are also an excellent source of protein (7.9 to 21.2 g per 100 g raw nut)29 and dietary fibre (ranging from 4 to 11 g per 100 g whole raw nut),27 which can provide 5–10% of the daily fibre requirements in standard servings.30 Moreover, nuts have a favourable concentration of essential minerals, such as calcium, magnesium and potassium when compared to other commonly consumed foods.27,31 Nuts are also abundant in vitamins and bioactive compounds such as tocopherols and polyphenols.32–36 One action of some polyphenols, which are secondary metabolites produced by plants,37–41 is to lower postprandial blood glucose42 in the same way as acarbose.7
Certain flavonoids attenuate the activity of digestive enzymes7,11 and are the major polyphenol class in nuts.43 Data on the yeast α-glucosidase indicates that binding of the hydroxyl groups in ring A, B or C of flavonoids to the active sites of α-glucosidases results in subtle modifications of the enzyme structure44–47 and inhibition is increased by hydroxylation of the A ring at the C5 or C6 of flavonoids.11,48,49
To ensure that we captured all papers on nut extracts and inhibition of α-glucosidase activity, we performed a systematic review of the literature. As we suspected prior to the search, most of the studies used yeast α-glucosidase despite indications in other literature that indicate that the specificity of inhibition of yeast and mammalian enzymes are very different.10,11,50 This review should help shape future studies and ensure that in vitro data are better predictors on activity in human intervention studies.
2. Methods
This review was registered with the International Platform of Registered Systematic Review and Meta-analysis Protocols (INPLASY) register of systematic reviews and is reported following the Preferred Reporting Items for Systematic reviews and Meta-Analyses (PRISMA) statement.51
2.1. Search strategy
The literature was searched, using a predetermined strategy, for published original studies that presented the effect of nut extracts on α-glucosidase activity. Articles were imported into Covidence (https://www.covidence.org) and duplicates were removed. The study selection was not limited to a period of time and all studies published up to the end of May 2022 were included for screening. All articles in this review were found from four different databases (PubMed, Scopus, Web of Science, and Cochrane) by searching the following research terms: (“inhibit*”) AND (“nuts” OR “nut” OR “almond*” OR “pecan*” OR “pistachio*” OR “hazelnut*” OR “walnut*” OR “cashew*”) AND (“*glucosidase” OR “sucrase*” OR “*maltase”).
To be included, studies must have: (1) been written in English; (2) been original research; (3) tested compounds from the edible parts of nuts; and (4) measured α-glucosidase enzyme inhibition (specified as α-glucosidase, sucrase or maltase). There was no limitation on the source of α-glucosidase used and all studies that examined enzyme activity from yeast or mammalian sources were included.
2.2. Data extraction
Data were extracted independently by two authors (MF and MM) and verified by a third (MJH). Data regarding nut, test inhibitor, description of nut extraction method, number of replicates, source of enzyme and enzyme assay description, positive/negative controls, IC50/inhibition% and range of inhibition, inhibition calculations, statistical analyses, and relevant outcomes were extracted from each paper.
2.3. Quality assessment
Two authors (MF and MJH) developed a quality assessment for in vitro enzyme assay studies, based on the ToxRTool,52 and independently completed it. Briefly, the quality of the articles was evaluated against 17 criteria in five assessment domains: test substance identification, enzyme assay characterisation, study design description, study results documentation, and the plausibility of the study design and results. Each was given either a “yes” or “no” response (ESI Table 1†), however, because each criterion did not have the same significance, it was impossible to give each study an overall quality rating.
3. Results
3.1. Characteristics of the selected papers
The main objective of the present systematic review was to assess the literature on the inhibition of human α-glucosidase activity by bioactive compound extracts from nuts; however, the search included all sources of α-glucosidase and was not confined only to human enzymes. The search identified 531 papers and, after removing 163 duplicate papers, the titles and abstracts of 368 papers were screened by three reviewers (MF, MJH and MM) for inclusion. The same three reviewers independently assessed the full text of 38 articles against the inclusion criteria. Fig. 1 displays the search results and the study selection process. Full texts of all articles were obtained to retrieve detailed study characteristics. A total of 15 papers met all inclusion criteria;17,18,53–65 10 studies examined the inhibitory effect on α-glucosidase activity of polyphenol-rich extracts, four studies examined the effects of isolated protein/peptides and one study used nuts extracted in hot water with no further extraction applied (Tables 1 and 2). Of the included studies, no studies reported the effect of nut extracts on human α-glucosidase, two studies examined rat α-glucosidase activity (Table 1), and 13 articles used α-glucosidase from yeast (Table 2). All studies presented the result of enzyme activity as either percentage of inhibition for a given concentration range or reported IC50, defined as the concentration of extract necessary to inhibit enzyme activity by 50%. All studies conducted their analyses in triplicates except for Wang et al.,64 who performed 5 replicates, and also Zhu et al.,65 and Pino Ramos et al.,61 who did not specify the number of replicates.
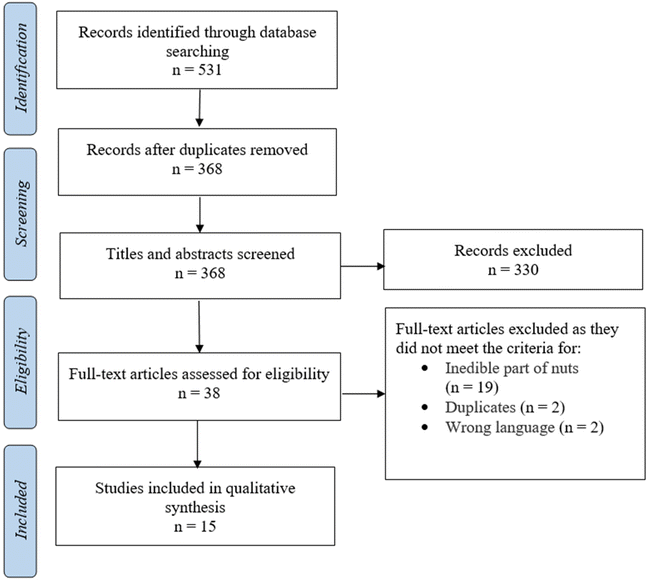 |
| Fig. 1 PRISMA flow diagram for the systematic review, detailing the database searches, the number of abstracts screened, and full texts retrieved. | |
Table 1 Characteristics of included studies which examined inhibition of rat α-glucosidase
Nut |
Extraction |
Rat enzyme assay |
Sample IC50 |
Ref. |
Name |
Part |
Conditions |
Target |
Substrate |
Positive control |
Data are expressed as mean (± standard deviation where specified) (n = 3). Abbreviations: NS, not specified; ND, no inhibition detected; PP, polyphenol. No further details. Alcalase samples (<5 kDa). Trypsin + Chymotrypsin sample (<5 kDa). Alcalase samples (>5 kDa). Trypsin + Chymotrypsin sample (>5 kDa). |
Almond (Prunus dulcis) |
Roasted seed skin |
Water, methanol, ethanol, acetone, acetonitrile |
PP-rich |
Maltose |
NS |
468 μg DW mL−1 |
18
|
Sucrose |
627 μg DW mL−1 |
Hazelnut (Corylus avellana L.) |
Meala |
Hexane, acetone, alkaline centrifugation, precipitation, alkaline protease hydrolysis, ultrafiltration |
Purified peptides |
p-Nitrophenyl-α-D-glucopyranoside |
NS |
3620 ± 720 μg DW mL−1 b |
17
|
3890 ± 120 μg DW mL−1 c |
4760 ± 120 μg DW mL−1 d |
NDe |
Table 2 Characteristics of included studies which examined inhibition of yeast α-glucosidase
Nut |
Extraction |
Yeast enzyme assay |
Sample IC50 or inhibition (%) |
Samples concentration range tested |
Ref. |
Name |
Part |
Conditions |
Target |
Substrate |
Positive control (IC50 or inhibition (%)) |
Data are expressed as mean (± standard deviation where specified). Abbreviations: ND, no inhibition detected; NS, not specified; DW, dry weight; PP, polyphenol. No information on IC50. No information on the lowest concentration tested. No further details (e.g. no details on part of nut used, no mention of skin left on seed or not, or identification of compounds present). Inhibition value of the Chilean hazelnut (Gevuina avellana), collected from Region del Bio-Bio (Contulmo) in May of 2016 and callana roasted, was not determined due to the low amount of material. Chilean hazelnut (Gevuina avellana) from Region del Maule. Chilean hazelnut (Gevuina avellana), from Region de Nuble. Chilean hazelnut (Gevuina avellana) from Yungay, Region de Nuble. Chilean hazelnut (Gevuina avellana) from Region del Bio-Bio. Chilean hazelnut (Gevuina avellana) from Region de Los Lagos. Dichloromethane fraction. Ethyl acetate fraction. N-Butanol fraction. Aqueous residue. Crude extract, dissolved dried extract with water and lyophilized then dissolved with 70% ethanol. Estimated from figure in the paper and not actually stated. “A2” fraction which was the most potent fraction after purification of 3–10 kDa walnut protein hydrosylates by gel filtration chromatography (Sephadex G-25 column) and RP-HPLC (reverse phase high-performance liquid chromatography). “B1” which is further purification of A2 on a Sephadex G-15 column. LPLLR is a novel pentapeptide, identified in fraction C1 after further purification of B1 by RP-HPLC. Hydrolysates from Prote Ax (Trp-His), 0–5 kDA fraction. 0–5 kDa fraction was purified (by gel filtration chromatography on a Sephadex G-25 column) into three subfractions, of which one (P3) had the highest inhibitory activity. Hydrolysates from Prote Ax, the P3 subfraction was further purified (RP-HPLC) into four subfractions, of which this one (P3P2) exhibited the highest inhibitory activity. P3P2 was further purified by molecular sieve and a single peptide was collected and further purified by RP-HPLC (peptide A). Hydrolysates from Protease M (Trp-Ser), 0–5 kDA fraction. 0–5 kDa fraction was purified (by gel filtration chromatography on a Sephadex G25 column) into three subfractions, of which this one (P2) had the highest inhibitory activity. Hydrolysates from Protease M, the P3 subfraction was further purified (RP-HPLC) into seven subfractions, of which P2P2 had the highest inhibitory activity. P2P2 was further purified by molecular sieve and a single peptide was collected and further purified by RP-HPLC (peptide B). |
Chilean hazelnut (Gevuina avellana)d |
Seedc |
Acetic acid, FeCl3·6H2O, acetonitrile, methanol |
PP-rich |
p-Nitrophenyl-α-D-glucopyranoside |
Acarbose (120.9 ± 2.0 μg mL−1) |
ND |
0–100 μg DW mL−1 b |
61
|
Vilches, 2016 rawe |
Vilches, 2017 rawe |
31.4 ± 1.6 μg DW mL−1 |
Vilches, 2016 roastede |
24.8 ± 0.7 μg DW mL−1 |
Los Lleques, 2017, April, rawf |
10.8 ± 0.9 μg DW mL−1 |
Los Lleuques, 2017, May, rawf |
ND |
Los Lleuques, 2016, roastedf |
79.7 ± 2.6 μg DW mL−1 |
Los Lleques, 2017, April, roastedf |
17.5 ± 0.4 μg DW mL−1 |
Los Lleques, 2017, April, roastedf |
ND |
Los Lleques, 2016, mild to medium roasted (meal)f |
66.5 ± 5.3 μg DW mL−1 |
Yungay, 2016, mild to medium roasted (meal)g |
12.3 ± 0.7 μg DW mL−1 |
Contulmo, 2017, rawh |
ND |
Contulmo, 2016, mild to medium roasted (meal)h |
ND |
Contulmo, 2016, intensively roasted (“coffee”)h |
ND |
Cotulmo, 2016, callana roastedh |
37.6 ± 2.6 μg DW mL−1 |
Contulmo, 2016, April, callana roastedh |
5.0 ± 0.4 μg DW mL−1 |
Contulmo, 2017, April, callana roastedh |
8.5 ± 0.6 μg DW mL−1 |
Contulmo, 2017, May, callana roastedh |
7.9 ± 0.4 μg DW mL−1 |
Contulmo, 2016, April, tostador roastedh |
87.4 ± 0.6 μg DW mL−1 |
Contulmo, 2016, May, tostador roastedh |
76.4 ± 0.5 μg DW mL−1 |
Contulmo, 2017, April, tostador roastedh |
3.7 ± 0.3 μg DW mL−1 |
Contulmo, 2017, May, tostador roastedh |
6.6 ± 0.3 μg DW mL−1 |
Llanada Grande, 2018, rawi |
60.9 ± 1.1% at 100 μg DW mL−1 a |
Indian almond (Terminalia catappa L.) |
Shelled drupe |
Methanol and N HCl |
PP-rich |
p-Nitrophenyl-α-D-glucopyranoside |
NS |
83.3 ± 3.5 μg DW mL−1 |
40–160 μg o |
53
|
Almond (Prunus amygdalaus, syn. Prunus dulcis) |
Cakec |
Water, hexane, alkaline protease |
PP-rich |
p-Nitrophenyl-α-D-glucopyranoside |
NS |
84.6% at 500 μg DW mL−1 a |
500–5000 μg mL−1 |
56
|
97.8% at 2000 μg DW mL−1 a |
98.9% at 5000 μg DW mL−1 a |
Almond (Prunus dulcis cv. Casteltermini) |
Seed skin |
n-Hexane, ethanol |
PP-rich |
Maltose |
Acarbose (50.1 ± 1.3 μg mL−1) |
57.1 ± 2.6 μg DW mL−1 |
NS |
58
|
Almond (Prunus amygdalus Stokes) |
Seedc |
Water |
NS |
Maltose |
Acarbose (NS) |
46% at 100 000 μg DW mL−1 a |
Single screen at 100 000 μg DW mL−1 |
55
|
Chinese nutmeg yew (Torreya grandis) |
Seedc |
Ethanol 10% |
PP-rich |
p-Nitrophenyl-α-D-glucopyranoside |
Acarbose (760 ± 10 μg mL−1) |
ND |
780–50 000 μg DW mL−1 |
65
|
Ethanol 30% |
63 450 ± 2110 μg DW mL−1 |
Ethanol 50% |
62 550 ± 750 μg DW mL−1 |
Ethanol 70% |
600 ± 30 μg DW mL−1 |
20 ± 00 μg DW mL−1 j |
100 ± 10 μg DW mL−1 k |
4650 ± 300 μg DW mL−1 l |
5680 ± 810 μg DW mL−1 m |
430 ± 20 μg DW mL−1 n |
Ethanol 90% |
2250 ± 310 μg DW mL−1 |
Terebinth (Pistacia terebinthus) |
Seed with skin |
Hexane |
PP-rich |
p-Nitrophenyl-α-D-glucopyranoside |
Acarbose (10 300 μg mL−1) |
1580 μg DW mL−1 |
125–2000 μg DW mL−1 |
54
|
Acetone |
720 μg DW mL−1 |
100–1000 μg DW mL−1 |
Ethanol |
1100 μg DW mL−1 |
Pistachio (Pistacia vera L.) |
Seedc |
Methanol, ascorbic acid, hexane |
PP-rich |
p-Nitrophenyl-α-D-glucopyranoside |
NS |
<50 μg of DW mL−1 |
NS |
60
|
Aegina cultivar |
Avdat cultivar |
2490 μg of DW mL−1 |
Kastel cultivar |
5940 μg of DW mL−1 |
Kerman cultivar |
<50 μg of DW mL−1 |
Larnaka cultivar |
<50 μg of DW mL−1 |
Mateur cultivar |
<50 μg of DW mL−1 |
Napolitana cultivar |
3670 μg of DW mL−1 |
Sirora cultivar |
3690 μg of DW mL−1 |
Marula nuts (Sclerocarya birrea) |
Shelled seed |
Methanol |
PP-rich |
p-Nitrophenyl-α-D-glucopyranoside |
NS |
7800 μg DW mL−1 o |
NS |
59
|
Heckel (Ricinodendron heudelotii (Baill.)) |
Seedc |
Ethyl acetate |
PP-rich |
p-Nitrophenyl-α-D-glucopyranoside |
Acarbose (NS) |
1.3 ± 0.0 mmol acarbose equivalent per g of extract |
NS |
66
|
Methanol |
1.3 ± 0.0 mmol acarbose equivalent per g of extract |
Water |
ND |
Manchurian walnut (J. mandshurica Maxim.) |
Hydrolysed walnut peptidesc |
“Alcalase” alkaline protease hydrolysis and ultrafiltration |
Purified peptides |
p-Nitrophenyl-α-D-glucopyranoside |
Acarbose (100% at 100 000 μg mL−1) a |
56.2 ± 1.6% at 60 000 μg mL−1 (>10 kDa) a |
1000–100 000 μg DW mL−1 |
63
|
61.7 ± 1.9% at 100 000 μg mL−1 (3–10 kDa) a |
46.6 ± 11.2% at 100 000 μg mL−1 (<3 kDa) a |
Manchurian walnut (J. mandshurica Maxim.) |
Fractionated and purified hydrolysed peptidesc |
“Alcalase” alkaline protease hydrolysis and ultrafiltration and chromatography |
Purified peptides |
p-Nitrophenyl-α-D-glucopyranoside |
Acarbose (73% at 2000 μM) a |
41.6% at 2000 μg mL−1 a,p |
100–2000 μg mL−1 |
64
|
33.7% at 2000 μg mL−1 a,q |
50.1% at 2000 μM r |
100–2000 μM |
Siberian apricot (almond) (Armeniaca sibirica) |
Almond oil manufacture residuec |
Defatted protein extract enzymatically hydrolysed by “Prote Ax” or “Protease M”; ultrafiltered |
Purified peptides |
p-Nitrophenyl-α-D-glucopyranoside |
NS |
2080 ± 10 μg mL−1 s |
NS |
57
|
97 ± 4 μg mL−1 t |
7.4 ± 0.3 μg mL−1 u |
0.58 ± 0.02 μg mL−1 v |
380 ± 20 μg mL−1 w |
45 ± 3 μg mL−1 x |
19 ± 1 μg mL−1 y |
13 ± 6 μg mL−1 z |
3.2. Inhibition of mammalian α-glucosidase by nut extracts
Table 1 presents the inhibitory potential of extracts from edible parts of almond (Prunus dulcis)18 and hazelnut (Corylus avellana L.)17 on rat α-glucosidase activity. Almond skin extract showed some inhibitory activity on rat intestinal maltase and sucrase, but the data was not compared to acarbose as a positive control.18 Simsek isolated protein from hazelnut meal and hydrolysed this using two protease treatments, Alcalase or trypsin/chymotrypsin, and studied the inhibitory effect of two peptide fractions from each (<5 kDa and >5 kDa). Several of the peptide fractions inhibited rat α-glucosidase activity by 50% at a concentration of ∼4 mg mL−1. However, again due to the lack of a positive control, it is not possible to determine how hazelnut meal protein compared to acarbose.17
3.3. Inhibition of yeast α-glucosidase by nut extracts
Table 2 shows the inhibitory potential of extracts from edible parts of nuts on yeast α-glucosidase activity. Pino Ramos et al.61 examined the effects of polyphenol-enriched extracts from raw and roasted hazelnuts from different regions of Chile. The mean IC50 for the extracts tested in this study, calculated from the 15 of 22 extracts where IC50 was determined, was 31.7 ± 30.4 μg mL−1, with no apparent difference between raw or roasted, nor geographical region, while the acarbose positive control had an IC50 of 120.9 ± 2.0 μg mL−1. The researchers identified 17 polyphenols in the extracts, mostly phenolic acid derivatives, though phenolic content was relatively low, and also some phytoprostanes and phytofurans.
Five of the included studies tested the inhibitory effects on yeast α-glucosidase of extracts from different types of almond. Of the polyphenol-rich extracts, Loizzo et al.58 found the highest inhibitory potential with an extract from almond skin, similar to that of acarbose. LC-MS data revealed the almond skin extract contained at least 30 phenolics, including (+)-catechin, proanthocyanidin B, (−)-epicatechin, several quercetin and kaempferol glycosides, rosmarinic acid, and prunin. The data between the studies is difficult to compare, since different nut varieties, extraction methods, enzyme assay substrates and/or enzyme amounts were used in each. The water extract in the study by Attaallah et al.55 demonstrated the lowest inhibition of yeast α-glucosidase by almonds, while water extracts of heckle seeds (Ricinodendron heudelotii), known commonly as the African nut-tree, also exhibited low inhibitory potential.66 A fifth study on almonds involved testing peptides purified from the residue of almond oil from the Siberian apricot (Armeniaca sibirica), which belongs to a subgroup of the Prunus genus.67 Hydrolysates, after digestion by two proteases, were purified in turn by gel filtration chromatography, reverse phase high performance liquid chromatography (RP-HPLC), molecular sieve and RP-HPLC again, with the inhibitory potential reaching 0.58 ± 0.02 μg mL−1 for a single isolated peptide.57
The inhibitory effects of polyphenol-rich extracts from eight pistachio cultivars, grown on the same farm in Spain, were studied by Noguera-Artiaga et al.60 The inhibitory potential varied widely, with IC50 values ≥2.5 mg mL−1 for four of the cultivars, while the other four cultivars exhibited an IC50 value <5 μg mL−1. Without a positive control for reference, it is difficult to comment on the relative potency of pistachio components as inhibitors of yeast α-glucosidase.
Zhu et al.,65 studied the inhibitory effects of extracts from Chinese nutmeg yew (Torreya grandis), which is an evergreen and high-value medicinal plant. In this study, polyphenols were extracted from the kernels with ethanol, ranging from 10 to 90% (v/v), with 70% ethanol giving the richest phenolic content and showing the highest inhibition against yeast α-glucosidase activity (IC50: 600 ± 30 μg mL−1). This extract was purified further and the dichloromethane fraction showed the highest inhibition (IC50: 20 ± 00 μg mL−1) compared to an IC50 of 760 ± 10 μg mL−1 for acarbose. In another study, Terebinth (Pistacia terebinthus), which is a type of deciduous shrub native to the Mediterranean region, was extracted with hexane, acetone and ethanol. Again, findings indicate that these extracts had a higher inhibition potential (IC50 ≤ 1580 μg mL−1) than acarbose (IC50: 10
300 μg mL−1) on yeast α-glucosidase.54 Wang et al.,63,64 examined the inhibitory effect of protein isolated from Manchurian walnut (J. mandshurica Maxim.), in 2018 and 2020. Both studies found protein isolated from the walnuts was less effective than acarbose at inhibiting yeast α-glucosidase.
3.4. Comparison of yeast and mammalian α-glucosidase inhibition
Table 3 shows the inhibitory effects of almond and hazelnut extracts on rat and yeast α-glucosidase activity. These papers were the only types of nuts for which studies had been done using both yeast and rat enzymes. Polyphenol-rich extracts from hazelnuts showed 50% inhibition of yeast α-glucosidase activity at a mean concentration of 21.1 ± 14.6 μg mL−1 for raw samples and 33.4 ± 32.2 μg mL−1 for roasted samples,61 whereas 50% inhibition of rat α-glucosidase with hazelnut meal peptides was found at much higher concentrations (3755 ± 191 μg mL−1).17 This demonstrates that hazelnut extracts are stronger inhibitors of yeast α-glucosidase compared to rat. However, it is worth noting that this is a comparison of polyphenol-rich extracts to purified peptides and the study by Simsek did not measure acarbose as a positive control,17 and neither provided specific enzyme activities. A more definitive comparison can be made for almonds, since both studies used a polyphenol-rich almond skin extract with maltase as substrate. An 8-fold stronger inhibition potential towards yeast α-glucosidase58 is evident compared to rat α-glucosidase.18
Table 3 Comparison of inhibition of rat and yeast α-glucosidase activity by nut extracts
Nut |
Part of nut |
Extract type |
Substrate |
Positive control (IC50) |
Samples IC50 (yeast enzyme) |
Sample IC50 (rat enzyme) |
Ref. |
Data are expressed as mean (± standard deviation where specified) (n = 3). Abbreviations: ND, no inhibition detected; NS, not specified; DW, dry weight; PP, polyphenol. Average of all raw samples from different regions (n = 2). Average of all roasted samples from different regions (n = 13). No further details. Average of peptide fractions <5 kDa (n = 2). |
Chilean hazelnut (Gevuina avellana), rawa |
Seeda |
PP-rich |
p-Nitrophenyl-α-D-glucopyranoside |
Acarbose (120.9 ± 2.0 μg mL−1) |
21.1 ± 14.6 μg DW mL−1a |
— |
61
|
Chilean hazelnut (Gevuina avellana), roastedb |
33.4 ± 32.2 μg DW mL−1b |
Hazelnut (Corylus avellana L.) |
Mealc |
Purified peptides |
p-Nitrophenyl-α-D-glucopyranoside |
NS |
— |
3755 ± 191 μg DW mL−1d |
17
|
Almond (Armeniaca sibirica syn. Prunus sibirica) |
Seed skin |
PP-rich |
Maltose |
Acarbose (50.1 ± 1.3 μg mL−1) |
57.1 ± 2.6 μg DW mL−1 |
— |
58
|
Almond (Prunus dulcis) |
Roasted seed skin |
PP-rich |
Maltose |
NS |
— |
468 μg DW mL−1 |
18
|
Sucrose |
627 μg DW mL−1 |
3.5. Quality assessment
ESI Table 1† shows the quality assessment of the papers included in the current review. Seventeen criteria were independently checked by two authors (MF and MJH). There were several key elements missing from the studies. Among the 15 reviewed studies, two did not specify the part of the nut from which the test substance/compounds was extracted56,60 and two more did not provide information on the purity, nor any compositional analysis (e.g., phytochemical), of the test inhibitors used.55,63 Two studies also failed to provide the nut origin/supplier,17,55 while 40% of studies were lacking this information for other solvents and reagents used throughout the extraction process and/or enzyme assay, and three studies lacked details on the nut extraction method (e.g., solvent, time and temperature).55,60,63
Surprisingly more than 66% of the studies did not provide details on the type or source of enzyme,53,56–61,63,64,66 with some requiring a search through secondary or even tertiary citations to ascertain that the yeast enzyme had been used, and 5 of 15 studies did not provide the amount/concentration of enzyme that was used.18,58,60,61,66 Almost half of the studies were lacking some or all details on the enzyme assay, including pH, buffer, incubation time and temperature, and concentration/amount of enzyme added; each of which affects enzyme activity and inhibition,17,18,55,58,60,61,66 and in three studies, the method of detection or endpoint, used to calculate enzyme activity/inhibition, was not specified.18,58,66
More than 80% of studies did not provide information on the use of positive controls, negative controls and/or the concentration of any controls used, and more than half did not mention the concentration range that was tested to calculate the IC50 value. All studies should have an inhibitor-free control in order to measure inhibition percentage or the IC50 value, but most did not provide information on substrate-free or enzyme-free controls, and half did not specify the inhibition percentage or IC50 value for the positive control.17,18,53,56,57,59,60 The description of results for all endpoints investigated was not complete in most studies. For example, authors do not describe any evaluation of endogenous sugars in the test inhibitor substance, nor do they mention specific enzyme activities or provide data relating to the controls. The statistical methods for data analysis of all studies were given and applied transparently. According to the chosen test system, enzyme assay parameters, number of doses and their range (for samples or controls), and/or inclusion of all the relevant results, all of the studies did not have an adequate and suitable study design to detect the anticipated effects. Further, the quantitative results of four of the studies are deemed not reliable due to a lack of presentation of data variability, an unusual way of calculating/presenting data, or a lack of clear dose dependent effects for putative inhibitors.18,60,65,66
Based on these assessments, we have developed a Quality Control Checklist for future studies on in vitro inhibition of enzyme activity (Table 4) which recommend future strategies to conduct and report data on inhibition of α-glucosidase activity.
Table 4 Criteria of the data reliability assessment tool for in vitro carbohydrate digestive enzyme inhibition assays
No. |
Criteria |
Explanations |
We report a modification of the ToxRTool52 to enable assessment of the quality of in vitro enzyme assays and act as a guide for researchers undertaking these types of experiment and publishing such data. |
Criteria group I: test substance identification
|
1 |
Is the test substance identified? |
Information on components and composition is expected. For plant extracts (e.g. nuts, fruits), details about the plant part used (e.g. fruit, seed, with/without skin) and processing applied (e.g. shelling, grinding, roasting, juicing) is expected |
2 |
Is the concentration and purity of the test substance given? |
Quantitative and qualitative information on the test substance purity and associated analyses are expected, including: |
- Names and amounts of bioactive compounds (e.g. specific polyphenol or peptide) and/or the total amount of bioactive compounds (e.g. total amount of polyphenols) present in the test substance |
- Type of analytical technique used to assess composition (e.g. LC-MS, Folin–Ciocalteu assay) |
- Names, grades, concentrations and volumes of solvents, enzymes and reagents used during purity assay |
- Relevant details of the purity assay, including buffer, pH, incubation time and temperature etc. provided, if applicable |
- Standards, including concentration range, where required (e.g. EGCG for Folin assay) |
3 |
Is information on the source of all substances given? |
a. Source/origin and supplier of the main test substance(s) is essential |
b. Source/supplier of all other chemicals is expected (e.g. enzyme assay substrate, enzyme, reagents, solvents, buffer, etc.) |
4 |
Is all information on the preparation of the test substance given? |
Method(s) used to extract/prepare samples, including details on solvents, times, temperatures, buffers, pH, etc., and tests used to determine yield/quality of extract (see criterion 2 for required information) must be given, unless no such additional information is needed |
|
Criteria group II: test system characterisation
|
5 |
Is the enzyme assay well described? |
Essential information about the enzyme assay includes: |
- Volume and concentration of substrate |
- Volume, concentration and pH of buffer |
- Incubation time and temperature |
- How the enzyme reaction was stopped |
6 |
Is information given on the source/origin of the enzyme? |
It is absolutely vital that details on the specific enzyme (ideally EC number) used, and the species from which it was sourced, are included with any published in vitro enzyme assay data. The enzyme may be commercially available or extracted from cultured cells, tissues or biospecimens, etc. If commercial, supplier details must be provided (see criterion 3b); if extracted by the researchers, details on the extraction process must be provided |
7 |
Is necessary information on test system properties and conditions given? |
Necessary information on the test system includes: |
a. Amount/concentration of enzyme (confirmed by measuring specific activity with no test inhibitors present and providing this value) |
b. Concentrations of positive (and negative if applicable) controls (e.g. acarbose) |
|
Criteria group III: study design description
|
8 |
Is the test substance concentration range given? |
IC50 values without information on the concentrations investigated in the assays are not considered sufficient |
9 |
Are observation time-points explained? |
This will typically apply to the time of the endpoint measurement at the end of the assay. Time points of further observations (e.g. kinetic assays) may not be mentioned when the experimental set-up makes clear that observation takes place immediately after end of assay (considered sufficient). Please also check figures and tables for respective information |
10 |
Were negative controls included? |
Negative controls are required for all in vitro studies. Not only an inhibitor-free control, since all studies must have used one to obtain IC50 or inhibition% values, but substrate-free and enzyme-free controls must have been used as well, with details provided |
11 |
Were positive controls included? |
Positive controls which are known inhibitors (e.g. acarbose) are required for all in vitro enzyme assays to confirm the test system is working as expected |
12 |
Is the number of replicates (and complete repetitions of the experiment) given? |
It is expected that assays are completed a minimum of three times, ideally on three separate days and, where enzymes are extracted from cells, tissues, animals or participants, the assay must be performed using enzymes from at least three biological replicates, with all relevant information on replicates provided |
|
Criteria group IV: study results documentation
|
13 |
Are the study endpoint(s) and their method(s) of determination clearly described? |
A description of how enzyme activity/inhibition was measured. This may be indirectly by using colorimetric methods with reducing sugars and dinitrosalicylic acid (DNSA) or chromophore-linked substrates (e.g. p-nitrophenyl-α-D-glucopyranoside) or directly by chromatography |
14 |
Is the description of the study results for all endpoints investigated transparent and complete? |
Results on all study endpoints described in the Methods section should be provided. Study results include specific enzyme activity, inhibition at each individual concentration investigated, results for positive and all negative controls, and other relevant details (e.g. when testing an extract, an enzyme-free control is necessary to check that the extract did not contain any endogenous sugars and/or enzymatic activity). Check figures and tables for relevant information |
15 |
Are the statistical analyses given and applied transparently? |
No in-depth examination and/or recalculation is expected here; rather the criterion asks for proper documentation. Only judge reported information. Where statistics are lacking, but considered indispensable, please consider this under “Plausibility of study design”, below |
|
Criteria group V: plausibility of study design and results
|
16 |
Is the chosen study design appropriate for obtaining the substance-specific data aimed for? |
Is the study design adequate and suitable to detect the anticipated effects in the test system used? Please don't give points if study design contains substantial flaws! |
Critical issues may be: |
- The chosen test system and its applicability domain |
- Number of replicates, number of concentrations/dose levels and their range and spread |
- Lack of negative control |
- Inclusion of all relevant endpoints |
- Lack of statistical evaluation |
- Source/type of enzyme |
- Concentration and amount of enzyme |
- How enzyme activity is measured |
17 |
Are the quantitative study results reliable? |
Please give points, such as if there are reasons to trust or mistrust the numerical values. Arguments here may be: |
- Was the observed variability of results, as well as that of negative/positive controls, acceptable? |
- Were control values in a reasonable range? |
Considerations will differ depending on the study type. Inherent high variability of a biological system should not be a reason to refuse making the point |
4. Discussion
This review evaluates research investigating the inhibitory effects of extracts from edible parts of nuts on α-glucosidase activity. Although the main objective was to assess the literature on human α-glucosidase inhibition, no studies were found using this enzyme source. Results demonstrate that most extracts/compounds are stronger inhibitors of yeast α-glucosidase compared to mammalian (rat) α-glucosidases. Therefore, although extracts from nuts may inhibit yeast α-glucosidase, it is never clear whether these compounds can also inhibit human (or rat) α-glucosidase unless assays have been done.
4.1. Effect of nut extracts on yeast α-glucosidase
Nuts are sources of bioactive compounds including peptides and polyphenols.33–35,61 Although data obtained from yeast α-glucosidase inhibition are irrelevant to human health and disease, findings of the current review show an inhibitory potential of nut extracts on yeast α-glucosidase, which may be attributed to the polyphenol content7,12,44 or peptides.57,64 Compared to acarbose, Chinese nutmeg yew, Chilean hazelnut and Terebinth showed 38-fold, 33-fold, and 14-fold higher inhibition values, respectively. Also, almond, Pistachio, Marula nuts, and Heckel all show inhibition of yeast α-glucosidase. The findings of the present study are in line with the literature which has indicated that polyphenols and bioactive peptides may inhibit α-glucosidase.7,12,68,69 It is worth noting, however, that nut extraction and enzyme assay methods varied vastly between the studies, so differences in inhibitory potential may be due to experimental approach, rather than differences between the nuts and nut composition.
4.2 Differences between yeast and mammalian α-glucosidase
Table 5 presents the inhibition values of some other extracts and compounds for yeast and mammalian α-glucosidase activity, which serves to demonstrate the vast differences in the inhibitory activity against different sources of α-glucosidases. Four types of medical plant, including Peltophorum africanum, Manilkara mochisia, Ozoroa cf. albicans, and Cassia abbreviate, were 50-fold to 250-fold stronger inhibitors of yeast α-glucosidase compared to rat α-glucosidase.70 However, acarbose is a better inhibitor of human or rat α-glucosidases compared to the yeast enzyme. According to Table 5, all polyphenols, except quercetagetin, showed better inhibition of yeast α-glucosidase. The IC50 for quercetagetin was >8-fold lower for human sucrase activity than that of yeast, while the inhibition values for quercetin against human sucrase and yeast α-glucosidase were similar.11,71,72 A stark difference is seen for cyanidin, with almost complete inhibition of the yeast enzyme at just 4 μM, whereas the rat enzyme was not inhibited at all.48 Thus yeast and mammalian α-glucosidases have shown very different inhibition potentials.10,70,73 In agreement with the results of the present review, Babu et al.,10 observed that the methanolic extract of Himalayan rhubarb is a better inhibitor of yeast α-glucosidase than the equivalent amount of mammalian α-glucosidase. A study of various foods extracts (extracts such as green tea, chicken essence, and yogurt were used to identify the effect of naturally-occurring food components on rat and yeast α-glucosidase activity) inhibited yeast α-glucosidase whereas no inhibition was found on rat α-glucosidase.74 These differences between rat and yeast α-glucosidase inhibition are related to molecular structural differences.10,70 It has been noted that yeast and mammalian α-glucosidase are enzymically totally different and distinct. Yeast α-glucosidase is a 584 amino acid soluble protein of glycosyl hydrolase family 13, whereas human sucrase/isomaltase, for example, is a complex membrane-associated protein of 1827 amino acids of glycoside hydrolase family 1. In addition, type I (yeast) and II (mammals) enzymes even have different amino acids in their catalytic sites.73
Table 5 Comparison of inhibition of yeast and mammalian α-glucosidase activity by other compounds and extracts
Inhibitor |
IC50 or inhibition (%) of yeast enzyme |
IC50 or inhibition (%) of human (or rat where stated) enzyme |
Ref. |
Data are expressed as mean (± standard deviation where specified). |
Acarbose |
IC50 2300 μM |
Isomaltose |
IC50 39.1 ± 2.1 μM |
11, 12 and 70 |
Sucrase |
IC50 1.7 ± 0.3 μM |
IC50 12.3 ± 0.6 μM (rat) |
Maltase |
IC50 13.9 ± 2.3 μM |
IC50 0.42 ± 0.02 μM (rat) |
IC50 619.6 μM (rat) |
Peltophorum africanum
|
IC50 40 μg mL−1 |
IC50 > 2500 μg mL−1 (rat) |
70
|
Manilkara mochisia
|
IC50 50 μg mL−1 |
IC50 > 2500 μg mL−1 (rat) |
Ozoroa cf. albicans
|
IC50 50 μg mL−1 |
IC50 > 2500 μg mL−1 (rat) |
Cassia abbreviata
|
IC50 10 μg mL−1 |
IC50 > 2500 μg mL−1 (rat) |
Apigenin |
43% at >200 μM |
3% at >500 μM (rat) |
48
|
Cyanidin |
99% at 4 μM |
6% at >500 μM (rat) |
EGCG |
IC50 0.99 ± 0.07 μM |
Isomaltose |
IC50 461.9 ± 60.3 μM |
11, 12 and 75 |
Sucrase |
IC50 175.2 ± 60.1 μM |
IC50 950 ± 86 μM (rat) |
Maltase |
IC50 186.4 ± 40.4 μM |
IC50 14.0 ± 2.0 μM (rat) |
Quercetin |
IC50 163.4 ± 3.4 μM |
Sucrase |
IC50 161.9 ± 13.6 μM |
11, 71 and 72 |
IC50 364.0 μM (rat) |
Maltase |
IC50 247.3 ± 7.0 μM |
IC50 231.6 μM (rat) |
Quercetagetin |
IC50 180.1 ± 3.7 μM |
Sucrase |
IC50 21.7 ± 5.3 μM |
Gallic acid |
IC50 143.4 ± 4.1 μM |
Sucrase |
IC50 1757.6 ± 19.3 μM (rat) |
83
|
Maltase |
IC50 710.6 ± 12.3 μM (rat) |
(−)-Epicatechin |
24% at >200 μM |
Sucrase |
IC50 1080 μM (rat) |
48 and 84 |
Maltase |
IC50 770 μM (rat) |
(−)-Epigallocatechin |
IC50 75 μM |
Sucrase |
IC50 921 μM (rat) |
Maltase |
IC50 1260 μM (rat) |
(−)-Epigallocatechin-3-O-gallate |
89% at 2 μM |
Sucrase |
IC50 169 μM (rat) |
Maltase |
IC50 40 μM (rat) |
4.3. Differences between rat and human α-glucosidase
The inhibition of human sucrase activity by acarbose is greater than on rat sucrase, whereas rat maltase activity is more susceptible to inhibition by acarbose compared to human maltase.12 The same pattern can be seen for human and rat α-glucosidase inhibition by EGCG and quercetin11,12,71,75 (Table 5). Although there are some similarities between rat and human α-glucosidase, the 74% homology in sucrase-isomaltase is sufficient to give rise to different specificities of inhibition;76 therefore, it can be concluded that even the inhibitory effect of different compounds/extracts on rat and human α-glucosidase are not equivalent. One reason for this mismatch is that N-glycosylation, which can affect inhibition due to steric hindrance, is different in rat and human sucrase-isomaltase.76 The human and rat sequences contain 18 and 16 N-glycosylation sites, respectively, while only 9 of them are in the same positions76 (Fig. 2).
 |
| Fig. 2 Primary structure of human and rat sucrase-isomaltase. The black region is the N-terminal non-cleavable signal sequence, which in addition serves as the transmembrane sequence. The grey region which is the highly O-glycosylated region can be found in each isomaltase sequence. The light blue and dark blue regions are low- and high-homology regions, respectively, between the corresponding regions of sucrase-isomaltase from these species. Created with BioRender.com. | |
4.4. Limitations
There were a number of limitations in this review. The most important point to note is that there were no studies on the inhibitory effect of extracts from edible parts of nuts on human α-glucosidase and the vast majority of studies in this area used the yeast enzyme. Another significant point is that all the studies in this review estimated α-glucosidase activity indirectly by using colorimetric methods such as reducing sugars/dinitrosalicylic acid (DNSA) or chromophore-linked substrates. In most of the colorimetric methods, coloured compounds such as phenolics can directly interfere with the method and contribute to the endpoint absorbance determination.6,77,78 The DNSA reagent is used to measure the reducing ability of the sugar product and so estimate carbohydrate hydrolysis. Therefore, endogenous sugars of plant extracts can affect the reducing sugars method for enzyme activity.6 Additionally, it has been reported that in reaction mixtures containing peptides the measurement was overestimated when using DNSA.79 No study in this review has used direct chromatography, which is the most precise method to measure enzyme activity.77 Lack of consistency in reporting enzyme amount, plus using enzymes from different sources, of different types (e.g., sucrase or maltase), and from different suppliers which will have different activities, highlightsthe need to present control (i.e. inhibitor-free or substrate-free) data and the specific enzyme activity. Most studies did not mention information on negative controls, including enzyme-free control or substrate-free control, while it is crucial to measure the amount of endogenous sugars in inhibitor samples and the effect of enzyme on inhibitors without substrate. Some studies lacked details about pH, temperature, or the buffer used for the enzyme assay, all of which can affect enzyme activity and inhibition.77,80 Within the reviewed studies, there are limitations regarding nut preparation. Some papers did not specify the details of the extracted part of the nuts (e.g. removing the skin) and studies in this review lack consistency in the nut extraction method used. For example, the nut extraction solvents varied across studies and may have influenced the effectiveness of enzyme inhibition due to affecting the availability and activity of bioactive compounds within the nut extracts.81,82 Further, the studies lack consistency in the concentration units and amount or type of substrate for measuring IC50 values. Hence, the comparison between studies is not meaningful. Also, the absence of acarbose as a positive control in eight studies complicates the comparison between various nut extracts.53,55–57,59,60,66 Due to the small number of included studies for rat enzyme inhibition, it is difficult to determine whether compounds in nut extracts are effective inhibitors of mammalian α-glucosidase.
These limitations, coupled with the lack of studies on human α-glucosidase, and growing attention to nuts as an important component of a healthy diet with potential to reduce T2DM risk,16 highlight the need for future research to evaluate the inhibitory effect of extracts from nuts on human α-glucosidase activities.
5. Conclusion
Among extensive research which has been done on nuts and health outcomes, none has investigated the inhibitory effects of extracts from edible nuts on human α-glucosidase activities and most of the studies have been conducted on the yeast α-glucosidase. The yeast α-glucosidase is poorly inhibited by acarbose, a positive control which is used clinically since it is highly effective at inhibiting human α-glucosidase activities, but acarbose is always a stronger inhibitor of the mammalian α-glucosidase compared to the yeast enzyme. In order to be relevant to human health and disease, future work must utilise human, or at least mammalian, α-glucosidase enzyme sources.
Abbreviations
T2DM | Type 2 diabetes mellitus |
INPLASY | International Platform of Registered Systematic Review and Meta-analysis Protocols |
PRISMA | Preferred Reporting Items for Systematic reviews and Meta-Analyses |
RP-HPLC | Reverse phase high performance liquid chromatography |
DNSA | Dinitrosalicylic acid |
Author contributions
GW developed the concept and supervised the research. Data were extracted independently by MF and MM and verified by MJH. MF and MJH developed the quality assessment tool. MF wrote the first version of the manuscript and MM, MJH and GW edited the manuscript. All the authors contributed to the writing of the manuscript and approved the final version.
Conflicts of interest
The authors report no current relevant conflict of interest.
Acknowledgements
We thank Monash University for an International PhD Scholarship to MF.
References
- O. O. Oguntibeju, Type 2 diabetes mellitus, oxidative stress and inflammation: examining the links, Int. J. Physiol., Pathophysiol. Pharmacol., 2019, 11, 45–63 CAS
.
- S. N. Bhupathiraju, D. K. Tobias, V. S. Malik, A. Pan, A. Hruby, J. E. Manson, W. C. Willett and F. B. Hu, Glycemic index, glycemic load, and risk of type 2 diabetes: results from 3 large US cohorts and an updated meta-analysis, Am. J. Clin. Nutr., 2014, 100, 218–232 CrossRef CAS PubMed
.
- K. Marsh, A. Barclay, S. Colagiuri and J. Brand-Miller, Glycemic index and glycemic load of carbohydrates in the diabetes diet, Curr. Diabetes Rep., 2011, 11, 120–127 CrossRef CAS PubMed
.
- A. D. Baron, Postprandial hyperglycaemia and α-glucosidase inhibitors, Diabetes Res. Clin. Pract., 1998, 40, S51–S55 CrossRef CAS PubMed
.
- H. Nyambe-Silavwe and G. Williamson, Polyphenol- and fibre-rich dried fruits with green tea attenuate starch-derived postprandial blood glucose and insulin: a randomised, controlled, single-blind, cross-over intervention, Br. J. Nutr., 2016, 116, 443–450 CrossRef CAS PubMed
.
- R. Visvanathan, M. Qader, C. Jayathilake, B. C. Jayawardana, R. Liyanage and R. Sivakanesan, Critical review on conventional spectroscopic α-amylase activity detection methods: merits, demerits, and future prospects, J. Sci. Food Agric., 2020, 100, 2836–2847 CrossRef CAS PubMed
.
- G. Williamson, Possible effects of dietary polyphenols on sugar absorption and digestion, Mol. Nutr. Food Res., 2013, 57, 48–57 CrossRef CAS PubMed
.
- J. A. Balfour and D. McTavish, Acarbose, Drugs, 1993, 46, 1025–1054 CrossRef CAS PubMed
.
- K. Y. Loo, K. H. Leong, Y. Sivasothy, H. Ibrahim and K. Awang, Molecular insight and mode of inhibition of α–glucosidase and α–amylase by Pahangensin A from Alpinia pahangensis Ridl, Chem. Biodivers., 2019, 16, e1900032–e1900039 Search PubMed
.
- K. S. Babu, A. K. Tiwari, P. V. Srinivas, A. Z. Ali, B. C. Raju and J. M. Rao, Yeast and mammalian α-glucosidase inhibitory constituents from Himalayan rhubarb Rheum emodi Wall. ex Meisson, Bioorg. Med. Chem. Lett., 2004, 14, 3841–3845 CrossRef CAS PubMed
.
- E. Barber, M. J. Houghton and G. Williamson, Flavonoids as human intestinal α-glucosidase inhibitors, Foods, 2021, 10, 1939–1960 CrossRef CAS PubMed
.
- A. Pyner, H. Nyambe-Silavwe and G. Williamson, Inhibition of human and rat sucrase and maltase activities to assess antiglycemic potential: Optimization of the assay using acarbose and polyphenols, J. Agric. Food Chem., 2017, 65, 8643–8651 CrossRef CAS PubMed
.
- B. Dalile, C. Kim, A. Challinor, L. Geurts, E. R. Gibney, M. V. Galdos, G. La Fata, S. Layé, J. C. Mathers and D. Vauzour, The EAT–Lancet reference diet and cognitive function across the life course, Lancet Planet. Health, 2022, 6, e749–e759 CrossRef PubMed
.
- E. H. Haddad, J. Sabate and C. G. Whitten, Vegetarian food guide pyramid: a conceptual framework, Am. J. Clin. Nutr., 1999, 70, 615s–619s CrossRef CAS PubMed
.
- R. K. Johnson and E. Kennedy, The 2000 dietary guidelines for americans: what are the changes and why were they made?, J. Acad. Nutr. Diet., 2000, 100, 769–774 CAS
.
- J. C. Lovejoy, The impact of nuts on diabetes and diabetes risk, Curr. Diabetes Rep., 2005, 5, 379–384 CrossRef CAS PubMed
.
- S. Simsek, Angiotensin I-converting enzyme, dipeptidyl peptidase-IV, and α-glucosidase inhibitory potential of hazelnut meal protein hydrolysates, J. Food Meas. Charact., 2021, 15, 4490–4496 CrossRef
.
- T. Tsujita, T. Shintani and H. Sato, α-Amylase inhibitory activity from nut seed skin polyphenols. 1. Purification and characterization of almond seed skin polyphenols, J. Agric. Food Chem., 2013, 61, 4570–4576 CrossRef CAS PubMed
.
- C. Luo, Y. Zhang, Y. Ding, Z. Shan, S. Chen, M. Yu, F. B. Hu and L. Liu, Nut consumption and risk of type 2 diabetes, cardiovascular disease, and all-cause mortality: a systematic review and meta-analysis, Am. J. Clin. Nutr., 2014, 100, 256–269 CrossRef CAS PubMed
.
- L. C. Del Gobbo, M. C. Falk, R. Feldman, K. Lewis and D. Mozaffarian, Effects of tree nuts on blood lipids, apolipoproteins, and blood pressure: systematic review, meta-analysis, and dose-response of 61 controlled intervention trials, Am. J. Clin. Nutr., 2015, 102, 1347–1356 CrossRef CAS PubMed
.
- K. Jaceldo-Siegl, E. Haddad, K. Oda, G. E. Fraser and J. Sabaté, Tree nuts are inversely associated with metabolic syndrome and obesity: the Adventist health study-2, PLoS One, 2014, 9, e85133 CrossRef PubMed
.
- R. Jiang, J. E. Manson, M. J. Stampfer, S. Liu, W. C. Willett and F. B. Hu, Nut and peanut butter consumption and risk of type 2 diabetes in women, J. Am. Med. Assoc., 2002, 288, 2554–2560 CrossRef PubMed
.
- G. Asghari, Z. Ghorbani, P. Mirmiran and F. Azizi, Nut consumption is associated with lower incidence of type 2 diabetes: The Tehran Lipid and Glucose Study, Diabetes Metab., 2017, 43, 18–24 CrossRef CAS PubMed
.
- J. Kochar, J. M. Gaziano and L. Djoussé, Nut consumption and risk of type II diabetes in the Physicians’ Health Study, Eur. J. Clin. Nutr., 2010, 64, 75–79 CrossRef CAS PubMed
.
- A. Afshin, R. Micha, S. Khatibzadeh and D. Mozaffarian, Consumption of nuts and legumes and risk of incident ischemic heart disease, stroke, and diabetes: a systematic review and meta-analysis, Am. J. Clin. Nutr., 2014, 100, 278–288 CrossRef CAS PubMed
.
- D. Aune, N. Keum, E. Giovannucci, L. T. Fadnes, P. Boffetta, D. C. Greenwood, S. Tonstad, L. J. Vatten, E. Riboli and T. Norat, Nut consumption and risk of cardiovascular disease, total cancer, all-cause and cause-specific mortality: a systematic review and dose-response meta-analysis of prospective studies, BMC Med., 2016, 14, 207 CrossRef PubMed
.
- E. Ros, Health benefits of nut consumption, Nutrients, 2010, 2, 652–682 CrossRef CAS PubMed
.
- P. Hernández-Alonso, L. Camacho-Barcia, M. Bulló and J. Salas-Salvadó, Nuts and dried fruits: An update of their beneficial effects on type 2 diabetes, Nutrients, 2017, 9, 673 CrossRef PubMed
.
- G. Brufau, J. Boatella and M. Rafecas, Nuts: source of energy and macronutrients, Br. J. Nutr., 2006, 96(Suppl 2), S24–S28 CrossRef CAS PubMed
.
- R. Segura, C. Javierre, M. A. Lizarraga and E. Ros, Other relevant components of nuts: phytosterols, folate and minerals, Br. J. Nutr., 2006, 96(Suppl 2), S36–S44 CrossRef CAS PubMed
.
- A. Wojdyło, I. P. Turkiewicz, K. Tkacz, P. Nowicka and Ł. Bobak, Nuts as functional foods: Variation of nutritional and phytochemical profiles and their in vitro bioactive properties, Food Chem.: X, 2022, 15, 100418 Search PubMed
.
- R. Blomhoff, M. H. Carlsen, L. F. Andersen and D. R. Jacobs Jr., Health benefits of nuts: potential role of antioxidants, Br. J. Nutr., 2006, 96(Suppl 2), S52–S60 CrossRef CAS PubMed
.
- Y. Kim, J. B. Keogh and P. M. Clifton, Benefits of nut consumption on insulin resistance and cardiovascular risk factors: Multiple potential mechanisms of actions, Nutrients, 2017, 9, 1271–1310 CrossRef PubMed
.
- M. Kornsteiner, K.-H. Wagner and I. Elmadfa, Tocopherols and total phenolics in 10 different nut types, Food Chem., 2006, 98, 381–387 CrossRef CAS
.
- Y. Liu, J. B. Blumberg and C.-Y. O. Chen, Quantification and bioaccessibility of California pistachio bioactives, J. Agric. Food Chem., 2014, 62, 1550–1556 CrossRef CAS PubMed
.
- L. L. P. Ramos, F. Jiménez-Aspee, C. Theoduloz, A. Burgos-Edwards, R. Domínguez-Perles, C. Oger, T. Durand, Á. Gil-Izquierdo, L. Bustamante and C. Mardones, Phenolic, oxylipin and fatty acid profiles of the Chilean hazelnut (Gevuina avellana): Antioxidant activity and inhibition of pro-inflammatory and metabolic syndrome-associated enzymes, Food Chem., 2019, 298, 125026–125039 CrossRef PubMed
.
- G. Cirillo, M. Curcio, O. Vittorio, F. Iemma, D. Restuccia, U. G. Spizzirri, F. Puoci and N. Picci, Polyphenol conjugates and human health: a perspective review, Crit. Rev. Food Sci. Nutr., 2016, 56, 326–337 CrossRef CAS PubMed
.
- T. Y. Forbes-Hernandez, M. Gasparrini, S. Afrin, S. Bompadre, B. Mezzetti, J. L. Quiles, F. Giampieri and M. Battino, The healthy effects of strawberry polyphenols: which strategy behind antioxidant capacity?, Crit. Rev. Food Sci. Nutr., 2016, 56, S46–S59 CrossRef CAS PubMed
.
- Y. Ma, Y. Shang, D. Zhu, C. Wang, Z. Zhong and Z. Xu, Facile separation of 5-O-galloylquinic acid from chinese green tea extract using mesoporous zirconium phosphate, Phytochem. Anal., 2016, 27, 153–157 CrossRef CAS PubMed
.
- M. Maldini, M. Chessa, G. L. Petretto, P. Montoro, J. P. Rourke, M. Foddai, M. Nicoletti and G. Pintore, Profiling and simultaneous quantitative determination of anthocyanins in wild Myrtus communis L. berries from different geographical areas in sardinia and their comparative evaluation, Phytochem. Anal., 2016, 27, 249–256 CrossRef CAS PubMed
.
- S. A. Palma-Duran, A. Vlassopoulos, M. Lean, L. Govan and E. Combet, Nutritional intervention and impact of polyphenol on glycohemoglobin (HbA1c) in non-diabetic and type 2 diabetic subjects: Systematic review and meta-analysis, Crit. Rev. Food Sci. Nutr., 2017, 57, 975–986 CrossRef CAS PubMed
.
- L.-W. Qi, E.-H. Liu, C. Chu, Y.-B. Peng, H.-X. Cai and P. Li, Anti-diabetic agents from natural products—an update from 2004 to 2009, Curr. Top. Med. Chem., 2010, 10, 434–457 CrossRef CAS PubMed
.
- C. Aneklaphakij, T. Saigo, M. Watanabe, T. Naake, A. R. Fernie, S. Bunsupa, V. Satitpatipan and T. Tohge, Diversity of chemical structures and biosynthesis of polyphenols in uut-bearing species, Front. Plant Sci., 2021, 12, 642581–642595 CrossRef PubMed
.
- J. Xiao, G. Kai, K. Yamamoto and X. Chen, Advance in dietary polyphenols as α-glucosidases inhibitors: a review on structure-activity relationship aspect, Crit. Rev. Food Sci. Nutr., 2013, 53, 818–836 CrossRef CAS PubMed
.
- X. Peng, G. Zhang, Y. Liao and D. Gong, Inhibitory kinetics and mechanism of kaempferol on α-glucosidase, Food Chem., 2016, 190, 207–215 CrossRef CAS PubMed
.
- P. K. Mujawdiya and S. Kapur, Screening of antioxidant and α-glucosidase inhibitory activities of Indian medicinal plants, Curr. Enzyme Inhib., 2020, 16, 145–154 CrossRef CAS
.
- T. Bansode and B. Salalkar, Strategies in the Design of Antidiabetic Drugs from Terminalia Chebula Retz. Using in silico and in vitro Approach, MicroMedicine, 2016, 4, 60–67 Search PubMed
.
- K. Tadera, Y. Minami, K. Takamatsu and T. Matsuoka, Inhibition of alpha-glucosidase and alpha-amylase by flavonoids, J. Nutr. Sci. Vitaminol., 2006, 52, 149–153 CrossRef CAS PubMed
.
- E. Lo Piparo, H. Scheib, N. Frei, G. Williamson, M. Grigorov and C. J. Chou, Flavonoids for controlling starch digestion: structural requirements for inhibiting human alpha-amylase, J. Med. Chem., 2008, 51, 3555–3561 CrossRef CAS PubMed
.
- D. R. Rose, M. M. Chaudet and K. Jones, Structural studies of the intestinal α-glucosidases, maltase-glucoamylase and sucrase-isomaltase, J. Pediatr. Gastroenterol. Nutr., 2018, 66, S11–S13 CrossRef CAS PubMed
.
- D. Moher, A. Liberati, J. Tetzlaff and D. G. Altman, Preferred reporting items for systematic reviews and meta-analyses: the PRISMA statement, Ann. Intern. Med., 2009, 151, 264–269 CrossRef PubMed
.
- K. Schneider, M. Schwarz, I. Burkholder, A. Kopp-Schneider, L. Edler, A. Kinsner-Ovaskainen, T. Hartung and S. Hoffmann, “ToxRTool”, a new tool to assess the reliability of toxicological data, Toxicol. Lett., 2009, 189, 138–144 CrossRef CAS PubMed
.
- S. A. Adefegha, G. Oboh, S. I. Oyeleye and I. Ejakpovi, Erectogenic, antihypertensive, antidiabetic, anti–oxidative properties and phenolic compositions of Almond Fruit (Terminalia catappa L.) Parts (Hull and Drupe)–in vitro, J. Food Biochem., 2017, 41, e12309–e12320 CrossRef
.
- M. Akyuz, L. Yabo-Dambagi, T. Kilic and A. Cakir, Antidiabetic, neuroprotective and antioxidant potentials of different parts of Pistacia terebinthus fruits, S. Afr. J. Bot., 2022, 147, 443–456 CrossRef CAS
.
- R. Attaallah, D. Elfadil and A. Amine, Screening study of enzymatic inhibition of medicinal plants for the treatment of diabetes using a glucometer biosensor approach and optical method, J. Herb. Med., 2021, 28, 100441–100448 CrossRef
.
- T. S. de Souza, F. F. Dias, J. P. S. Oliveira, J. M. de Moura Bell and M. G. B. Koblitz, Biological properties of almond proteins produced by aqueous and enzyme-assisted aqueous extraction processes from almond cake, Sci. Rep., 2020, 10, 1–12 CrossRef PubMed
.
- X. Gu, T. Gao, Y. Hou, D. Li and L. Fu, Identification and characterization of two novel α-glucosidase inhibitory peptides from almond (Armeniaca sibirica) oil manufacture residue, LWT–Food Sci. Technol., 2020, 134, 110215–110223 CrossRef CAS
.
- M. R. Loizzo, R. Tundis, M. Leporini, G. D'Urso, R. Gagliano Candela, T. Falco, S. Piacente, M. Bruno and F. Sottile, Almond (Prunus dulcis cv. casteltermini) skin confectionery by-products: New opportunity for the development of a functional blackberry (Rubus ulmifolius schott) jam, Antioxidants, 2021, 10, 1218–1237 CrossRef CAS PubMed
.
- V. E. Manhivi, R. M. Slabbert and D. Sivakumar, Co-ingestion of natal plums (Carissa macrocarpa) and Marula nuts (Sclerocarya birrea) in a snack bar and its effect on phenolic compounds and bioactivities, Molecules, 2022, 27, 310–324 CrossRef CAS PubMed
.
- L. Noguera-Artiaga, M. D. Salvador, G. Fregapane, J. Collado-González, A. Wojdyło, D. López-Lluch and Á. A. Carbonell-Barrachina, Functional and sensory properties of pistachio nuts as affected by cultivar, J. Sci. Food Agric., 2019, 99, 6696–6705 CrossRef CAS PubMed
.
- L. L. Pino Ramos, F. Jiménez-Aspee, C. Theoduloz, A. Burgos-Edwards, R. Domínguez-Perles, C. Oger, T. Durand, Á. Gil-Izquierdo, L. Bustamante, C. Mardones, K. Márquez, D. Contreras and G. Schmeda-Hirschmann, Phenolic, oxylipin and fatty acid profiles of the Chilean hazelnut (Gevuina avellana): Antioxidant activity and inhibition of pro-inflammatory and metabolic syndrome-associated enzymes, Food Chem., 2019, 298, 125026–125038 CrossRef CAS PubMed
.
- M. E. Rusu, I. Fizeşan, A. Pop, A.-M. Gheldiu, A. Mocan, G. Crişan, L. Vlase, F. Loghin, D.-S. Popa and I. Tomuta, Enhanced recovery of antioxidant compounds from hazelnut (Corylus avellana L.) involucre based on extraction optimization: Phytochemical profile and biological activities, Antioxidants, 2019, 8, 460–481 CrossRef CAS PubMed
.
- J. Wang, K. Du, L. Fang, C. Liu, W. Min and J. Liu, Evaluation of the antidiabetic activity of hydrolyzed peptides derived from Juglans mandshurica Maxim. fruits in insulin–resistant HepG2 cells and type 2 diabetic mice, J. Food Biochem., 2018, 42, e12518–e12526 CrossRef
.
- J. Wang, T. Wu, L. Fang, C. Liu, X. Liu, H. Li, J. Shi, M. Li and W. Min, Anti-diabetic effect by walnut (Juglans mandshurica Maxim.)-derived peptide LPLLR through inhibiting α-glucosidase and α-amylase, and alleviating insulin resistance of hepatic HepG2 cells, J. Food Biochem., 2020, 69, 103944–103956 CAS
.
- M. f. Zhu, Z. c. Tu, L. Zhang and H. Liao, Antioxidant, metabolic enzymes inhibitory ability of Torreya grandis kernels, and phytochemical profiling identified by HPLC–QTOF–MS/MS, J. Food Biochem., 2019, 43, e13043–e1342 Search PubMed
.
- S. Sut, S. Dall'Acqua, K. Bene, S. B. di Marco, K. I. Sinan, M. F. Mahomoodally, M. C. N. Picot-Allain and G. Zengin, Ricinodendron heudelotii (Baill.) Heckel stem barks and seed extracts, a native food plant from Africa: Characterization by NMR and HPLC-DAD-ESI-MSn, Food Res. Int., 2020, 129, 108877–108886 CrossRef CAS PubMed
.
- S. Shi, J. Li, J. Sun, J. Yu and S. Zhou, Phylogeny and classification of Prunus sensu lato (Rosaceae), J. Integr. Plant Biol., 2013, 55, 1069–1079 CrossRef CAS PubMed
.
- Y. Ren, K. Liang, Y. Jin, M. Zhang, Y. Chen, H. Wu and F. Lai, Identification and characterization of two novel α-glucosidase inhibitory oligopeptides from hemp (Cannabis sativa L.) seed protein, J. Funct. Foods, 2016, 26, 439–450 CrossRef CAS
.
- L. Sun and M. Miao, Dietary polyphenols modulate starch digestion and glycaemic level: a review, Crit. Rev. Food Sci. Nutr., 2020, 60, 541–555 CrossRef CAS PubMed
.
- L. Shai, S. Magano, S. Lebelo and A. Mogale, Inhibitory effects of five medicinal plants on rat alpha-glucosidase: Comparison with their effects on yeast alpha-glucosidase, J. Med. Plant Res., 2011, 5, 2863–2867 CAS
.
- S. H. Kim, S. H. Jo, Y. I. Kwon and J. K. Hwang, Effects of onion (Allium cepa L.) extract administration on intestinal α-glucosidases activities and spikes in postprandial blood glucose levels in SD rats model, Int. J. Mol. Sci., 2011, 12, 3757–3769 CrossRef CAS PubMed
.
- W. Wang, H. Xu, H. Chen, K. Tai, F. Liu and Y. Gao, In vitro antioxidant, anti-diabetic and antilipemic potentials of quercetagetin extracted from marigold (Tagetes erecta L.) inflorescence residues, J. Food Sci. Technol., 2016, 53, 2614–2624 CrossRef CAS PubMed
.
- S. Chiba, Molecular mechanism in alpha-glucosidase and glucoamylase, Biosci. Biotechnol. Biochem., 1997, 61, 1233–1239 CrossRef CAS PubMed
.
- T. Matsui, C. Yoshimoto, K. Osajima, T. Oki and Y. Osajima, In vitro survey of alpha-glucosidase inhibitory food components, Biosci. Biotechnol. Biochem., 1996, 60, 2019–2022 CrossRef CAS PubMed
.
- N. Promyos, P. Temviriyanukul and U. Suttisansanee, Evaluation of α-glucosidase inhibitory assay using different sub-classes of flavonoids, Curr. J. Appl. Sci. Technol., 2017, 17, 172–180 Search PubMed
.
- E. H. Van Beers, H. A. Büller, R. J. Grand, A. W. Einerhand and J. Dekker, Intestinal brush border glycohydrolases: structure, function, and development, Crit. Rev. Biochem. Mol. Biol., 1995, 30, 197–262 CrossRef CAS PubMed
.
- E. Barber, M. J. Houghton, R. Visvanathan and G. Williamson, Measuring key human carbohydrate digestive enzyme activities using high-performance anion-exchange chromatography with pulsed amperometric detection, Nat. Protoc., 2022, 17, 2882–2919 CrossRef CAS PubMed
.
- R. Visvanathan, M. J. Houghton and G. Williamson, Maltoheptaoside hydrolysis with chromatographic detection and starch hydrolysis with reducing sugar analysis: Comparison of assays allows assessment of the roles of direct α-amylase inhibition and starch complexation, Food Chem., 2021, 343, 128423–128436 CrossRef CAS PubMed
.
- R. S. Teixeira, A. S. da Silva, V. S. Ferreira-Leitão and E. P. da Silva Bon, Amino acids interference on the quantification of reducing sugars by the 3,5-dinitrosalicylic acid assay mislead carbohydrase activity measurements, Carbohydr. Res., 2012, 363, 33–37 CrossRef CAS PubMed
.
- C. Lankatillake, S. Luo, M. Flavel, G. B. Lenon, H. Gill, T. Huynh and D. A. Dias, Screening natural product extracts for potential enzyme inhibitors: protocols, and the standardisation of the usage of blanks in α-amylase, α-glucosidase and lipase assays, Plant Methods, 2021, 17, 3–21 CrossRef CAS PubMed
.
- M. Qasim, I. Aziz, M. Rasheed, B. Gul and M. A. Khan, Effect of extraction solvents on polyphenols and antioxidant activity of medicinal halophytes, Pak. J. Bot., 2016, 48, 621–627 CAS
.
- S. F. Sulaiman, A. A. B. Sajak, K. L. Ooi and E. M. Seow, Effect of solvents in extracting polyphenols and antioxidants of selected raw vegetables, J. Food Compos. Anal., 2011, 24, 506–515 CrossRef CAS
.
- J. Shinde, T. Taldone, M. Barletta, N. Kunaparaju, B. Hu, S. Kumar, J. Placido and S. W. Zito, Alpha-glucosidase inhibitory activity of Syzygium cumini (Linn.) Skeels seed kernel in vitro and in Goto-Kakizaki (GK) rats, Carbohydr. Res., 2008, 343, 1278–1281 CrossRef CAS PubMed
.
- T. Matsui, T. Tanaka, S. Tamura, A. Toshima, K. Tamaya, Y. Miyata, K. Tanaka and K. Matsumoto, alpha-Glucosidase inhibitory profile of catechins and theaflavins, J. Agric. Food Chem., 2007, 55, 99–105 CrossRef CAS PubMed
.
|
This journal is © The Royal Society of Chemistry 2023 |
Click here to see how this site uses Cookies. View our privacy policy here.