DOI:
10.1039/D3FO00728F
(Paper)
Food Funct., 2023,
14, 6828-6839
Effect of Lacticaseibacillus paracasei strain Shirota supplementation on clinical responses and gut microbiome in Parkinson's disease†
Received
22nd February 2023
, Accepted 21st June 2023
First published on 12th July 2023
Abstract
Parkinson's disease (PD) is a common neurodegenerative disease characterized by motor issues and a range of non-motor symptoms. Microbial therapy may be a useful approach for the treatment of PD. However, comprehensive analyses of the impact of probiotic supplementation on motor and non-motor symptoms are still lacking and the mechanisms whereby the treatment works remain unclear. This study investigated Lacticaseibacillus paracasei strain Shirota (LcS) supplementation on clinical responses, gut microbiota and faecal metabolites in PD patients. Patients (n = 128) were randomised to receive either probiotics (LcS-fermented milk, containing 1 × 1010 living LcS cells) or placebo for 12 weeks. All participants were examined and the basic clinical features were recorded using questionnaires. Fecal and blood samples were collected at the baseline and after 12 weeks for further omics analysis. We found that LcS intervention significantly alleviated patients’ constipation-related symptoms and non-motor symptoms. We found no significant shifts in the composition of gut microbiota or faecal metabolites. Several taxa were differentially abundant between the groups, especially with regard to LcS intake, which increased the abundance of the genus Lacticaseibacillus in the probiotic group compared with those at the baseline and in the placebo group. The faecal concentration of L-tyrosine was significantly decreased and the plasma concentration of L-tyrosine was increased in the probiotic group compared with the placebo group. Our study demonstrated that although supplementation with LcS did not induce major changes in the global gut microbiome, the probiotic had favorable effects in managing constipation and other non-motor symptoms in PD patients. This study was registered at the Chinese Clinical Trial Registry: ChiCTR1800016795.
1. Introduction
Parkinson's disease (PD) is a common neurodegenerative disease characterized by motor symptoms, including bradykinesia, resting tremors and rigidity. Non-motor symptoms (NMSs), such as neuropsychiatric symptoms, sleep disorders, wakefulness, and constipation, have gained relevance in recent years. Of these symptoms, constipation is among the most common NMSs of PD, with a prevalence of 70–80%. Many non-motor features are intricately related to each other and may overlap in symptomatology and treatments. NMSs may develop at any stage of the disease and severely affect the overall quality of life of PD patients; effective management options have been recognized as an important unmet need in the clinical setting.1 However, limited therapies are available for non-motor deficits. The current standard interventions, such as dopamine replacement therapy, are limited in the treatment of non-motor dysfunctions; in fact, some aspects of non-motor dysfunctions can even be worsened by dopamine treatment.2
There has been a surge in awareness of the association between gut microbiota and PD.3–6 As evidence is accumulating on the effects of gut microbiota on disease development and progression, another course of action has been recognized in relation to the potential usage of microbiota-based therapeutic strategies in PD. Consequently, microbiota-targeted interventions, including the administration of probiotics, have increasingly been evaluated. Studies have found that the intake of fermented milk, which contains fibre and multiple strains of probiotics or multi-strain probiotic capsules (including strains from Bifidobacterium, Lactobacillus and so on) for 4 weeks, can improve the symptoms associated with constipation in PD patients.7–9 However, one study reported that probiotic treatment might relieve abdominal pain and bloating as much as trimebutine treatment, with less improvement in constipation.10 Frankly, there are still many questions about the proper use of probiotic therapy for PD. First, in theory, the therapeutic effects of a single-strain probiotic and its effects on gastrointestinal symptoms are more straightforward to assess than multi-species probiotic supplements. Moreover, a recent review did not find convincing evidence that multi-species probiotic supplements were superior to single-strain probiotic supplements.11 It would be necessary to perform individual trials to confirm the effect of a specific probiotic. Second, as the duration of intervention in both studies was only 4 weeks, further studies on the long-term efficacy and safety profile of probiotic administration in PD are needed. Indeed, Bifidobacterium and Lactobacillus are included in most probiotic formulations, but are two of the genera that have been commonly elevated in PD patients.12,13 Third, a comprehensive analysis of the impact of probiotic supplementation on motor and non-motor symptoms and how probiotics contribute to homeostasis or symptom improvement is still lacking, and the mechanisms remain unclear.
Lacticaseibacillus paracasei strain Shirota (L. paracasei YIT 9029; LcS), formerly classified as Lactobacillus casei strain Shirota, has been used in the production of fermented milk for more than 80 years. Many studies have found that LcS has many beneficial effects, including protection against infection, enhanced immunoregulation, a reduced risk of carcinogenesis and enhanced intestinal motility.14,15 One pilot study, for the first time, showed improvements in stool consistency and defecation habits in PD patients who used LcS as a single-strain supplement.16
In this study, we designed a randomized, double-blind, placebo-controlled trial to investigate the effect of LcS supplementation on clinical responses and gut microbial homeostasis in PD patients.
2. Materials and methods
2.1. Study design and participants
We performed a randomized, double-blind, placebo-controlled trial at the Movement Disorder Clinic of the Department of Neurology, Ruijin Hospital, Shanghai Jiao Tong University School of Medicine. The patients were assessed for eligibility when they were diagnosed with PD according to the Parkinson's disease Queen Square Brain Bank criteria17 and were assessed on whether they met the Rome III criteria for functional constipation.18 Other inclusion and exclusion criteria are described in the ESI Methods.† Eligible patients were randomized to receive LcS fermented milk (100 mL, containing 1 × 1010 living LcS cells) or a placebo (manufactured by Yakult (China) Corp., Shanghai, China) once daily at breakfast for 12 weeks. The placebo was an acidified milk without LcS, with the same nutritional content, colour, flavour, taste, and pH, made using the same ingredients as the LcS-fermented milk, with the addition of lactic acid. The trial consisted of a baseline period of two weeks, a 12-week intervention period and two visits (V1 and V2) (Fig. S1†). The beverages were distributed to each subject every two weeks via a refrigerated parcel and stored at 0–10 °C. Compliance was monitored by asking the participants to return the beverage containers. The study was conducted following the guidelines laid down in the Declaration of Helsinki and approved by the Ethics Committee of Ruijin Hospital, Shanghai Jiao Tong University School of Medicine. All subjects consented to participate in this study and provided their written informed consent. This study was registered at the Chinese Clinical Trial Registry: ChiCTR1800016795. All experiments were performed in compliance with China's national laws and guidelines.
2.2. Randomization
Eligible patients were randomized to either the probiotic or placebo arm using a computer-generated randomization sequence by an independent statistician who was not involved in the data management or statistical analyses. The size of the blocks was not disclosed to the investigators. The patients and investigators were all blinded to the treatment allocation. Unblinding for statistical analyses was performed after database lock.
2.3. Clinical evaluation and sample collection
Study visits were conducted for each participant at the end of the baseline period and at the end of the intervention. Demographic data (age, sex and body mass index (BMI)), the use of anti-parkinsonism medications, the age of onset, and disease duration were recorded. Disease severity was evaluated using the Movement Disorder Society–Unified Parkinson's Disease Rating Scale (MDS-UPDRS) and Hoehn and Yahr scale (H&Y scale) during the “ON” condition. Non-motor symptoms, depression, anxiety, and quality of life were assessed using the Non-motor Symptoms Scale (NMSS), Hamilton Depression Scale (HAMD-17), Hamilton Anxiety Scale (HAMA), and 39-item Parkinson's Disease Questionnaire (PDQ-39), respectively. Cognitive function was evaluated by the Mini Mental State Examination (MMSE) and Montreal Cognitive Assessment (MoCA). The levodopa equivalent daily dosage (LEDD) was calculated according to a previously published study.19 Constipation severity was assessed using the Wexner constipation score. Quality of life related to constipation, including physical discomfort, psychosocial discomfort, worries/concerns, and satisfaction, was measured using the Patient Assessment of Constipation Quality of Life (PACQoL) questionnaire. Study participants were trained in completing the stool diary at 2 weeks before study entry and weeks 11–12 of the intervention period (the mean numbers were calculated), including the number of bowel movements (BMs), stool consistency, and the use of laxatives. Stool consistency was assessed by the Bristol Stool Form Scale (BSFS, e.g., 1 = hard lumps; 2 = lumpy sausage; 3 = cracked sausage; 4 = smooth sausage; 5 = soft lumps; 6 = mushy; 7 = watery).20 Faecal and blood samples were collected for each participant at the end of the baseline period and at the end of the intervention. The faecal samples were freshly collected by the patients in the morning using sterile faecal collection containers, immediately transported on ice to the hospital, and then stored at −80 °C prior to testing. The blood samples were collected in tubes containing ethylenediaminetetraacetic acid (1 mg mL−1) and glutathione (1 mg mL−1) and immediately centrifuged (3000 rpm for 10 min at 4 °C). Plasma was extracted from the blood samples, transferred to a new centrifuge tube, and stored at −80 °C prior to processing.
2.4. 16S rRNA sequencing and the measurement of metabolomics
The V3–V4 regions of the 16S rRNA gene were amplified and sequenced on an Illumina MiSeq platform (Illumina Inc., CA, USA). A targeted metabolomics approach based on a validated method was used to analyse the faecal samples, with a total of 111 metabolites quantified. Plasma L-tyrosine was measured using a high-performance liquid chromatograph with a fluorescence detector (HPLC-FLD). Details of sample extraction and the detection methods are described in the ESI Methods.†
2.5. Statistical analysis
The sample size of 92 patients was calculated to achieve a two-sided type I error of 5% and a power of 90%, based on a mean difference of 1.1 (SD = 1.6) in the number of complete bowel movements (CBMs) per week between the probiotic group and placebo group according to a previously published RCT.7 Assuming a 20% dropout rate, we planned to enroll a total of 112 patients. All data analyses were performed following the intention-to-treat principles. Missing data were imputed with the last value carried forward. Quantitative data are presented as the mean ± standard deviation (SD), and qualitative data are presented as the frequency (percentage). The paired Wilcoxon rank test was used to analyse the differences in clinical characteristics between the baseline and week 12 in the probiotic group and placebo group. Differences in clinical characteristics between the randomization groups were analysed by the Mann–Whitney U test for quantitative data or the chi-square test for qualitative data. The effect of treatment on a continuous scale was analysed using a general linear regression model, adjusting for potential confounders as appropriate. The correlation was calculated using Spearman's rank-correlation analysis. Statistical analysis was performed using SPSS software (version 22.0; SPSS Inc., Chicago, IL) and R software (ver. 3.1.0, the R Project for Statistical Computing). The P values for multiple testing were corrected by the Benjamini–Hochberg method for the false discovery rate (FDR). The significance levels, including the P value and FDR-P value, were set at 0.05 (two-tailed).
3. Results
3.1. Characteristics of study participants
Between August 2018 and January 2019, a total of 163 patients were recruited for screening, and 35 patients were excluded due to not meeting the inclusion criteria (n = 35). A total of 128 selected patients were randomized and allocated to the probiotic group (n = 65) or the placebo group (n = 63). Eleven patients in the placebo group could not complete the intervention due to a delay in the placebo supply at week 10. The placebo intervention for those patients was terminated at the end of 10 weeks, and faecal and blood samples were collected and clinical evaluation was performed at that time. The data obtained from these patients were included in the intention-to-treat analysis as data for week 12. Overall, no harmful events related to the test beverage intake were reported. A flow diagram of the enrolment and randomization process is presented in Fig. 1. All analyses were performed using an intention-to-treat procedure. The baseline clinical characteristics were similar between the two study groups (Table 1).
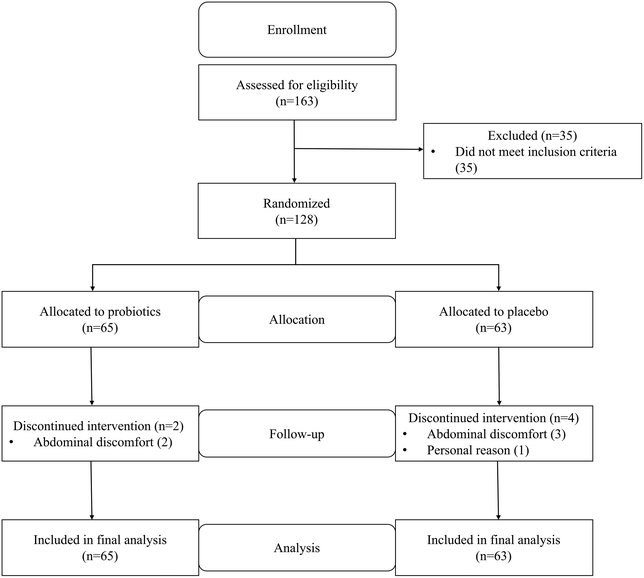 |
| Fig. 1 Trial profile. | |
Table 1 Baseline clinical characteristics according to the randomized groups
|
Probiotic group |
Placebo group |
p
|
Data are presented as mean ± standard deviation (SD) or frequency (percentage). Differences between groups were assessed using the χ2 test for categorical data and the Mann–Whitney U test for numerical data. BMI, body mass index; H&Y stage, Hoehn and Yahr stage. |
Number |
65 |
63 |
|
Gender (male/female) |
31/34 |
42/21 |
0.030 |
Age (years) |
67.22 ± 6.46 |
69.64 ± 6.41 |
0.057 |
BMI (kg m−2) |
22.58 ± 3.17 |
22.94 ± 2.95 |
0.545 |
Age of onset (years) |
61.00 ± 7.77 |
63.13 ± 8.27 |
0.150 |
Disease duration (years) |
6.29 ± 4.47 |
6.51 ± 4.92 |
0.956 |
H&Y stage (n, %) |
|
|
0.757 |
1–2.5 |
46 (70.8) |
43 (68.3) |
|
3–5 |
19 (29.2) |
20 (31.7) |
|
3.2. Influence of the intervention on clinical parameters
Clinical profiles at the baseline and after 12 weeks of intervention are shown in Table 2. After 12 weeks of intervention, the constipation-related symptoms were noticeably improved compared with those at the baseline in the probiotic group (Wexner score: 10.88 ± 4.44 vs. 13.52 ± 4.05, p < 0.001; BSFS score: 3.32 ± 1.02 vs. 2.90 ± 1.01, p < 0.001; BMs: 3.99 ± 2.20 vs. 2.70 ± 1.87, p < 0.001; PACQoL: 49.65 ± 13.44 vs. 62.65 ± 12.48, p < 0.001). We also found that people in the probiotic group decreased their use of laxatives (2.13 ± 2.44 vs. 2.50 ± 2.52, p = 0.045). Moreover, 12 weeks of LcS supplementation improved the non-motor symptoms based on the NMSS (37.60 ± 21.41 vs. 46.22 ± 22.82, p < 0.001), HAMD-17 (5.80 ± 4.31 vs. 7.11 ± 4.43, p < 0.001) and HAMA (11.65 ± 5.55 vs. 13.66 ± 5.47, p < 0.001). The LcS intervention improved quality of life scores as measured on the PDQ-39 (19.45 ± 17.29 vs. 21.03 ± 16.62, p < 0.001) compared with the baseline scores. Finally, there was a significant change in the NMSS and HAMA scores in the placebo group before and after the intervention (NMSS: 52.10 ± 33.48 vs. 54.56 ± 33.75, p = 0.007; HAMA: 13.48 ± 7.26 vs. 14.11 ± 7.54, p = 0.001). We hypothesized that the patients’ willingness was mainly driven by the desire to continue enjoying such food products or even due to the placebo effect.
Table 2 Clinical profiles at the baseline and after the 12-week intervention in individuals who received either the probiotic or the placebo
|
Probiotic group (n = 65) |
Placebo group (n = 63) |
|
|
Baseline |
12 weeks |
Change |
p
a
|
Baseline |
12 weeks |
Change |
p
b
|
Treatment effecta |
p
c
|
Data are presented as mean ± standard deviation (SD). Differences between the baseline and at 12 weeks in the probiotic and placebo groups were assessed using the paired Wilcoxon rank test. According to the generalized linear regression model (data are reported as the mean difference between the changes of the probiotic and placebo groups), after adjusting for age, gender, BMI, disease duration, baseline MDS-UPDRS III scores, baseline Wexner score, baseline PACQoL, baseline BMs, baseline BSFS score, baseline use of laxatives, and the baseline score, respectively. MDS-UPDRS, International Parkinson and Movement Disorder Society–Unified Parkinson's Disease Rating Scale (higher scores indicate worse severity); NMSS, Non-Motor Symptoms Scale; HAMD-17, Hamilton Depression Scale; HAMA, Hamilton Anxiety Scale; MMSE, Mini Mental State Examination; MoCA, Montreal Cognitive Assessment; PACQoL, Patient Assessment of Constipation Quality Of Life; PDQ-39, 39-item Parkinson's disease Questionnaire; LEDD, Levodopa Equivalent Daily Dosage; BSFS, Bristol Stool Form Scale; BMs, Bowel Movements; n per weekpa, differences of clinical characteristics between the baseline and at 12 weeks in the probiotic group. pb, differences of clinical characteristics between the baseline and at 12 weeks in the placebo group. pc, differences in the treatment effect between the changes of the probiotic group and placebo group. |
MDS-UPDRS I |
12.26 ± 4.04 |
11.14 ± 4.05 |
−1.12 ± 1.86 |
<0.001
|
13.17 ± 5.15 |
13.06 ± 5.05 |
−0.11 ± 1.64 |
0.781 |
−0.99 (−1.62, −0.37) |
0.002
|
MDS-UPDRS II |
13.58 ± 5.61 |
13.83 ± 5.52 |
0.25 ± 1.79 |
0.222 |
14.4 ± 5.39 |
14.57 ± 5.69 |
0.17 ± 1.28 |
0.239 |
— |
— |
MDS-UPDRS III |
33.82 ± 12.38 |
34.28 ± 12.21 |
0.46 ± 3.67 |
0.404 |
33.37 ± 14.40 |
34.51 ± 14.65 |
1.14 ± 2.72 |
0.002
|
— |
— |
MDS-UPDRS IV |
1.83 ± 2.79 |
2.03 ± 3.17 |
0.20 ± 1.09 |
0.134 |
1.41 ± 2.92 |
1.30 ± 2.59 |
−0.11 ± 1.18 |
0.435 |
— |
— |
MDS-UPDRS total |
61.49 ± 19.69 |
61.28 ± 20.28 |
−0.22 ± 5.77 |
0.392 |
62.35 ± 22.85 |
63.44 ± 23.10 |
1.10 ± 3.55 |
0.040
|
— |
— |
NMSS |
46.22 ± 22.82 |
37.60 ± 21.41 |
−8.62 ± 8.33 |
<0.001
|
54.56 ± 33.75 |
52.10 ± 33.48 |
−2.46 ± 6.47 |
0.007
|
−6.50 (−9.28, −3.73) |
<0.001
|
HAMD-17 |
7.11 ± 4.43 |
5.80 ± 4.31 |
−1.31 ± 2.08 |
<0.001
|
7.27 ± 5.80 |
7.35 ± 5.49 |
0.08 ± 1.21 |
0.481 |
−1.23 (−1.80, −0.65) |
<0.001
|
HAMA |
13.66 ± 5.47 |
11.65 ± 5.55 |
−2.02 ± 2.76 |
<0.001
|
14.11 ± 7.54 |
13.48 ± 7.26 |
−0.63 ± 1.50 |
0.001
|
−1.44 (−2.20, −0.67) |
<0.001
|
MMSE |
28.20 ± 1.91 |
28.35 ± 1.91 |
0.15 ± 0.92 |
0.154 |
27.83 ± 1.98 |
28.02 ± 1.77 |
0.19 ± 0.95 |
0.142 |
— |
— |
MoCA |
25.31 ± 2.87 |
25.60 ± 2.75 |
0.29 ± 1.86 |
0.200 |
24.79 ± 3.54 |
24.76 ± 3.60 |
−0.03 ± 1.59 |
0.635 |
— |
— |
PDQ-39 |
21.03 ± 16.62 |
19.45 ± 17.29 |
−1.58 ± 4.17 |
<0.001
|
23.08 ± 21.05 |
23.43 ± 21.39 |
0.35 ± 3.09 |
0.579 |
−1.88 (−3.26, −0.50) |
0.008
|
LEDD (mg) |
505.63 ± 248.71 |
514.39 ± 258.92 |
8.76 ± 43.66 |
0.110 |
561.98 ± 310.72 |
565.59 ± 303.13 |
3.60 ± 47.52 |
0.442 |
— |
— |
Wexner score |
13.52 ± 4.05 |
10.88 ± 4.44 |
−2.65 ± 2.30 |
<0.001
|
14.44 ± 5.14 |
14.49 ± 4.90 |
0.05 ± 1.61 |
0.806 |
−2.78 (−3.51, −2.05) |
<0.001
|
BSFS score |
2.90 ± 1.01 |
3.32 ± 1.02 |
0.42 ± 0.80 |
<0.001
|
2.74 ± 1.03 |
2.76 ± 0.90 |
0.02 ± 0.62 |
0.793 |
0.42 (0.18, 0.65) |
0.001
|
BMs (n per week) |
2.70 ± 1.87 |
3.99 ± 2.20 |
1.29 ± 1.80 |
<0.001
|
2.71 ± 1.93 |
2.88 ± 2.06 |
0.17 ± 1.27 |
0.169 |
1.26 (0.72, 1.79) |
<0.001
|
Use of laxatives (n per week) |
2.50 ± 2.52 |
2.13 ± 2.44 |
−0.37 ± 1.54 |
0.045
|
2.96 ± 2.96 |
2.98 ± 2.91 |
0.02 ± 1.24 |
0.490 |
— |
— |
PACQoL total score |
62.65 ± 12.48 |
49.65 ± 13.44 |
−13.00 ± 11.15 |
<0.001
|
63.35 ± 15.53 |
61.76 ± 15.05 |
−1.59 ± 6.53 |
0.050 |
−11.72 (−14.92, −8.52) |
<0.001
|
Physical discomfort |
9.83 ± 2.64 |
7.95 ± 2.62 |
−1.88 ± 2.00 |
<0.001
|
9.83 ± 3.02 |
9.41 ± 3.15 |
−0.41 ± 1.42 |
0.033
|
−1.51 (−2.16, −0.86) |
<0.001
|
Psychosocial discomfort |
12.18 ± 3.80 |
10.71 ± 2.97 |
−1.48 ± 2.43 |
<0.001
|
12.70 ± 4.58 |
12.22 ± 4.46 |
−0.48 ± 1.64 |
0.028
|
−0.99 (−1.71, −0.27) |
0.008
|
Worries/concerns |
23.26 ± 6.66 |
18.37 ± 6.25 |
−4.89 ± 5.42 |
<0.001
|
23.84 ± 8.37 |
23.73 ± 7.68 |
−0.11 ± 3.38 |
0.504 |
−5.08 (−6.65, −3.50) |
<0.001
|
Satisfaction part |
17.32 ± 3.65 |
12.62 ± 4.16 |
−4.71 ± 3.99 |
<0.001
|
16.98 ± 4.23 |
16.40 ± 4.53 |
−0.59 ± 2.08 |
0.063 |
−4.09 (−5.26, −2.91) |
<0.001
|
Compared with the placebo group, the LcS intervention group's constipation-related symptoms were significantly alleviated as assessed by the Wexner score (mean difference: −2.78, 95% CI (confidence interval): −3.51, −2.05, p < 0.001), BSFS score (mean difference: 0.42, 95% CI: 0.18, 0.65, p = 0.001), BMs (mean difference: 1.26, 95% CI: 0.72, 1.79, p < 0.001) and PACQoL (mean difference: −11.72, 95% CI: −14.92, −8.52, p < 0.001). Additionally, probiotic supplementation resulted in a statistically significant reduction in NMSS (mean difference: −6.50, 95% CI: −9.28, −3.73, p < 0.001), HAMD-17 (mean difference: −1.23, 95% CI: −1.80, −0.65, p < 0.001), HAMA (mean difference: −1.44, 95% CI: −2.20, −0.67, p < 0.001), and PDQ-39 (mean difference: −1.88, 95% CI: −3.26, −0.50, p = 0.008) scores compared to the placebo. Finally, there was no change in dopaminergic therapy requirements (LEDD) between the two groups. The LcS intervention had no significant effect on MDS-UPDRS scores, except for MDS-UPDRS I (non-motor experiences of daily living) scores (mean difference: −0.99, 95% CI: −1.62, −0.37, p = 0.002).
3.3. Influence of the intervention on gut microbiota
We first compared the effects of the intervention on changes in the composition of the faecal microbiota after 12 weeks compared with the baseline in the probiotic and placebo groups. The richness and diversity of the microbial community indicated by the Chao1, Simpson and Shannon estimators showed no significant changes at the baseline or week 12 in the two groups (Fig. 2a–c). To evaluate the β-diversity changes in the gut microbiota across the groups, principal coordinate analysis (PCoA) was applied to the whole dataset of bacterial counts based on the unweighted UniFrac metrics. We found no difference in β-diversity between the baseline and follow-up for the probiotic and placebo groups (Fig. 2d).
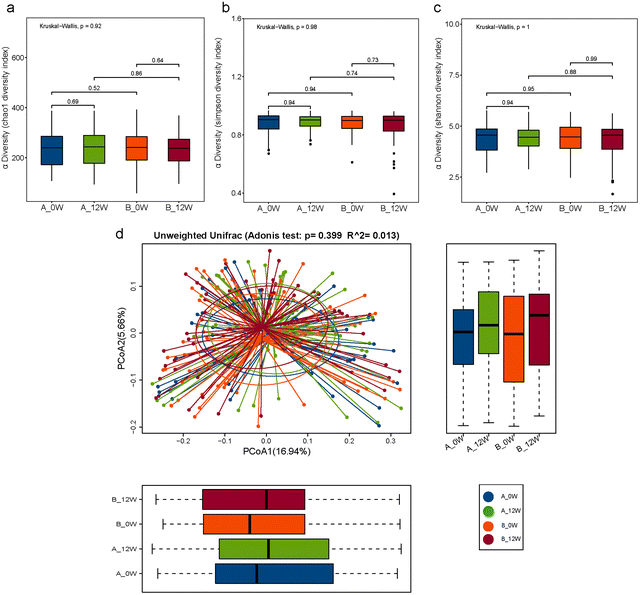 |
| Fig. 2 Changes of global gut microbiota after intervention in each group. (a–c) α-Diversity based on OTU levels estimated by the Chao1, Simpson and Shannon estimators, determined by the Kruskal–Wallis test. (d) Principal coordinate analysis (PCoA) score plots based on the unweighted UniFrac distance. A: Probiotic group, B: placebo group. | |
There were changes in the composition of the faecal microbiota of different taxonomic levels ranging from the phylum to genus levels after 12 weeks of the intervention compared with the baseline (the top 20 differences with the lowest p-value are shown in Fig. 3a and b). However, after FDR correction for multiple testing, only the relative abundance of the genus Lacticaseibacillus was significantly increased in the probiotic group (p < 0.001). We then compared the changes in the relative abundance of the faecal microbiota between the probiotic and placebo groups (the top 20 differences are shown in Fig. 3c); among them, the order Veillonellales-Selenomonadales, family Veillonellaceae and genus Lacticaseibacillus showed the same trends in the probiotic group before and after the intervention. After FDR correction, only the change in the relative abundance of the genus Lacticaseibacillus was the most significantly different (p < 0.001).
 |
| Fig. 3 Faecal microbiota with differences (top 20) after intervention in each group. (a) Changes of the relative abundance of individual taxa before and after LcS intervention, determined by the paired Wilcoxon rank test. (b) Changes of the relative abundance of individual taxa before and after placebo intervention, determined by the paired Wilcoxon rank test. (c) The changes in the relative abundance of individual taxa between the probiotic and placebo groups, determined by the Mann–Whitney U test. A: Probiotic group, B: placebo group. | |
3.4. Influence of the intervention on faecal metabolomic profiles
There were no significant shifts in the composition of faecal metabolites among the 4 groups as assessed by PCA (Fig. 4a). Comparing each metabolite, there was no difference in metabolites between the probiotic group and the placebo group at the baseline. An analysis further stratified by the major metabolite categories showed that the relative abundance of amino acid metabolites was significantly altered in the probiotic group after 12 weeks of probiotic supplementation compared with that at the baseline (40.18% vs. 37.28%, p = 0.045) (Fig. 4b). The changes in individual faecal metabolites from the baseline to the end of intervention were noted (Table 3); however, after FDR correction, none of the differences between the metabolites were significant. We then compared the changes of the metabolites between the probiotic and placebo groups. Compared with the placebo group, the probiotic group had significantly altered faecal concentrations of L-tyrosine (change: −12.50 ± 33.77 vs. −0.29 ± 31.08 ng mg−1, p = 0.020), 3,4-dihydroxyhydrocinnamic acid (change: −1.84 ± 26.91 vs. −4.52 ± 31.81 ng mg−1, p = 0.044) and glyceric acid (change: −0.22 ± 0.98 vs. 0.21 ± 1.46 ng mg−1, p = 0.045).
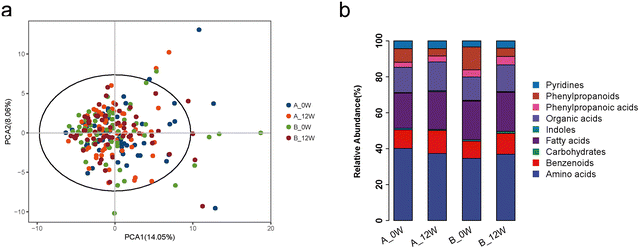 |
| Fig. 4 Change of faecal metabolomics after intervention in each group. (a) Faecal metabolites as assessed by principal component analysis (PCA). (b) Relative abundance of the metabolite categories among the groups. A: Probiotic group, B: placebo group. | |
Table 3 Faecal metabolites with differences after the intervention in each group
Metabolites (ng mg−1) |
Baseline |
12 weeks |
p
|
|
Probiotic group
|
L-Tyrosine |
57.86 ± 30.61 |
45.36 ± 22.32 |
0.006 |
Oxoadipic acid |
0.005 ± 0.003 |
0.008 ± 0.006 |
0.012 |
L-Methionine |
81.83 ± 37.98 |
69.41 ± 32.65 |
0.027 |
L-Tryptophan |
12.24 ± 6.65 |
10.24 ± 5.36 |
0.028 |
Docosapentaenoic acid, DPA |
4.94 ± 9.80 |
2.70 ± 4.42 |
0.033 |
Oxoglutaric acid |
6.15 ± 19.11 |
6.61 ± 11.21 |
0.038 |
4-Hydroxyphenylpyruvic acid |
5.14 ± 4.64 |
6.87 ± 6.29 |
0.041 |
Phenylpyruvic acid |
19.84 ± 20.23 |
29.19 ± 48.47 |
0.043 |
3,4-Dihydroxyhydrocinnamic acid |
3.76 ± 25.81 |
1.92 ± 6.97 |
0.046 |
|
Placebo group
|
Succinic acid |
7.57 ± 30.43 |
5.67 ± 7.83 |
0.015 |
Pentadecanoic acid |
32.87 ± 23.75 |
23.68 ± 22.26 |
0.029 |
Methylmalonic acid |
15.63 ± 59.74 |
12.85 ± 19.67 |
0.030 |
Oxalic acid |
4.23 ± 3.95 |
4.52 ± 2.35 |
0.048 |
We then measured the concentration of plasma L-tyrosine in fasting plasma samples. At the baseline, no differences in plasma L-tyrosine levels were observed between the groups. After treatment, the plasma L-tyrosine concentration was substantially altered solely in the probiotic group (11.20 ± 3.08 μg mL−1vs. 17.02 ± 8.97 μg mL−1, p = 0.002) (Fig. 5a). The change in the plasma concentration of L-tyrosine in the probiotic group was higher than that in the placebo group (change: 5.81 ± 8.27 vs. 1.90 ± 7.67, p = 0.016) (Fig. 5b). We specifically tested the correlation between changes in faecal and plasma L-tyrosine concentrations. The changes in the faecal concentrations of L-tyrosine were found to be negatively associated with the changes in the plasma concentrations of L-tyrosine (r = −0.27, p = 0.037) in the probiotic group (Fig. 5c), but this did not occur in the placebo group (r = −0.19, p = 0.169).
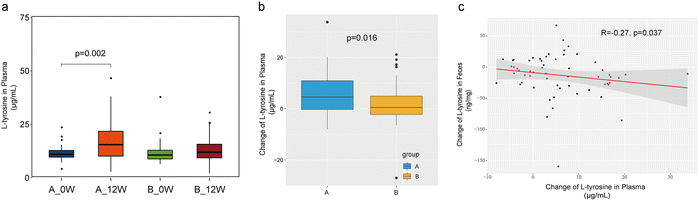 |
| Fig. 5 Effects of LcS supplementation on the concentration of plasma L-tyrosine. (a) The concentration of plasma L-tyrosine among the groups, determined by the paired Wilcoxon rank test or Mann–Whitney U test. (b) The changes in the plasma concentration of L-tyrosine between the probiotic and placebo groups, determined by the Mann–Whitney U test. (c) The correlation between changes in the faecal and plasma L-tyrosine concentrations in the probiotic group, determined by Spearman's rank-correlation analysis. A: Probiotic group, B: placebo group. | |
4. Discussion
We performed this RCT to investigate the effect of relatively long-term LcS intake on combined measures of gastrointestinal functioning and metabolic health in PD patients with constipation. Our study suggests that although LcS supplementation did not induce major changes in the global gut microbiota composition, the probiotic had favorable effects on the NMSs of PD patients.
Constipation is one of the most frequent symptoms in PD patients. The underlying causes for constipation in PD are multifaceted. In addition to physical weakness, lifestyle risks such as reduced fluid intake may significantly promote the emergence of constipation; moreover, side effects of anti-PD medications could also worsen it.21 Dysbiosis of the gut microbiota has also been hypothesized to contribute to the onset and clinical manifestation of constipation.22,23 The mismanagement of constipation in PD patients leads to intestinal occlusion, in addition to an impaired therapeutic effect of levodopa. To reduce symptom burden and improve the quality of life of PD patients, timely and effective treatment is essential. The potential use of probiotics as an alternative treatment for constipation in PD patients has been established. LcS is known to alleviate constipation in different populations. Daily LcS consumption has also been demonstrated to prevent adverse drug-associated gastrointestinal events.24 Our findings are in line with those of previous studies,16 which indicate that 12 weeks of single-strain LcS probiotic intake is an effective and safe method to alleviate constipation symptoms in PD patients. Moreover, our study is the first to demonstrate that LcS supplementation could also improve constipation-related symptoms, reduce symptom burden and improve the quality of life of PD patients as assessed by the PACQoL and PDQ-39 scores.
Anxiety and depression are common non-motor symptoms in PD patients, with a potential negative impact on motor disability and quality of life.25 The mechanism of mood disturbance in PD patients is not clear, and the current hypothesis includes changes in neurotransmitters (such as serotonin (5-HT), dopamine (DA), noradrenaline (NE), and acetylcholine) and lesions of the front-subcortical circuits.26 Emotional alterations could influence motor skills, and moreover, they contribute significantly and independently to worsening of rehabilitative treatment responses and great functional impairments.27 From our results, we found that adjuvant LcS treatment conferred added clinical effects on improving depression of PD patients. There was improvement in anxiety both in probiotic and placebo groups. However, a larger magnitude of improvement was observed in the probiotic group than the placebo group. The probiotic LcS has been shown to have positive psychological effects by improving mood disturbances in elderly individuals28 and reducing anxiety symptoms in patients with chronic fatigue syndrome.29 An RCT study also found that daily consumption of LcS for 9 weeks appeared to improve the potentially depressive symptoms and significantly decreased the IL-6 levels and regulated the intestinal microbiota associated with mental illness.30 Additionally, ingestion of LcS suppressed stress-induced increases in glucocorticoids and anxiety- and depression-related behaviors in human and animal models. These actions might be mediated by a direct neural communication between the gut–brain axis through stimulation of the gastric branch of the vagal afferent signaling to the brain and reduced stress reactivity in the paraventricular nucleus.31 However, limited studies have explored the use of probiotics for mental health diseases in PD patients. From our results, we demonstrated the beneficial effects of LcS probiotics in alleviating depression and anxiety in PD patients. One study found that 12 weeks of mixed probiotic consumption by individuals with PD had useful impacts on MDS-UPDRS scores.32 However, this study only assessed the UPDRS total score and did not evaluate motor or non-motor symptoms. We supposed the improvement in UPDRS total scores by probiotics in this study might mainly be due to the improvement in non-motor symptoms. Zhang et al. found that Probio-M8 intervention significantly reduced the UPDRS-III scores versus the baseline, while the scores also decreased in the placebo group after 3 months of intervention.33 With respect to safety, the 12-week LcS intervention did not induce any obvious side effects. There was no difference with respect to adverse events between the probiotic and placebo groups, suggesting that LcS probiotic treatment is safe for PD patients. Another concern is whether the efficacy of probiotic treatment is influenced by antibiotics or dopaminergic treatments. However, there was no difference in the LEDD between the two experimental groups, and there was no antibiotic use during the experiment and no mindful changes in anti-PD medications before or after the experiment. Moreover, we found that people in the probiotic group slightly decreased their use of laxatives.
Our study provided data on the effectiveness of LcS supplementation, which exerted positive effects on mood and gastrointestinal symptoms in PD patients. However, information on how probiotics contribute to homeostasis or improve symptoms is still lacking, and the mechanisms whereby the treatment works in PD patients remain unclear. Growing evidence indicates that alterations in the gut microbiota and faecal metabolites may contribute to constipation and its related symptoms.34,35 In our study, we used an integrative multi-omics analysis to investigate the gut microbiota and its metabolites in PD patients after LcS treatment. We found that daily ingestion of LcS did not result in a higher diversity and stability of the microbiota composition. However, the probiotic led to a significant increase in the relative abundance of Lacticaseibacillus between the baseline and after the intervention. Moreover, after FDR correction, Lacticaseibacillus was the only microbiota responsible for the difference between the two groups, which was not surprising since it was the administered LcS probiotic. In previous studies, it has also been suggested that the administration of LcS probably affects the gut microbiota composition indirectly, since LcS ingestion influenced the abundances of other strains, rather than solely increasing LcS concentrations. The long-term ingestion of LcS has been reported to increase the population levels of indigenous Bifidobacteria as well as the levels of the faecal organic acid concentration.36–38 Additionally, several different mechanisms explaining the effects of probiotics have been described, such as immune modulation, the production of lactic acid (consequently reducing the local pH), and the competitive adhesion or displacement of pathogenic bacteria.39,40 LcS could ameliorate depression-like behaviours in rats through the upregulation of expression levels of monoamines 5-HT, DA and NE and the activation of BDNF-TrkB signalling.39,41 In our study, we analysed a total of 111 faecal metabolites, and we found no significant shifts in the composition of faecal metabolites among the groups, especially short chain fatty acids (SCFAs) or other metabolites implicated in PD. However, we found that LcS could decrease the faecal concentration of L-tyrosine. We supposed that LcS supplementation may improve the impaired digestion and absorption of L-tyrosine in the upper digestive tract of PD patients. Indeed, we found that LcS increased the plasma concentration of L-tyrosine and that the change in plasma L-tyrosine was negatively associated with its concentration in faeces. L-Tyrosine is the biochemical precursor of catecholamines, such as DA and NE. It has been demonstrated that gut bacteria are able to increase luminal DA bioavailability through enzymes such as β-glucuronidase and tyrosine decarboxylase.42,43 DA is synthesized from L-tyrosine viaL-3,4-dihydroxyphenylalanine (L-DOPA) by tyrosine hydroxylase (TH) and aromatic L-amino acid decarboxylase (AADC).44L-Tyrosine may have the potential to enhance a variety of DA- and NE-related functions in the brain, thus ameliorating mental illness and improving the quality of life in PD patients. While the exact role of L-tyrosine in the contribution of LcS to PD symptom improvement still needs further study. Meanwhile, another RCT found the probiotic Probio-M8 could increase the serum level of DA in PD patients,33 which is similar to our results. However, it is known that only L-DOPA (but not DA) could pass through the blood–brain barrier and alleviate PD-related symptoms.
There are some limitations in this study. First, the number of patients who could not complete the intervention was different between the groups (2 in the probiotic group and 15 in the placebo group). For 11 patients in the placebo group, the intervention was terminated at week 10 due to a delay in the placebo supply. The intention-to-treat analysis was performed by incorporating the data of these 11 patients at week 10 as those at week 12. Another aspect that could also be considered a limitation was the fact that faecal sampling was carried out only at the baseline and at the end of the trial. A more complete picture of changes in the gut microbiota would have been possible with more frequent sampling. Shotgun metagenome analysis, which can provide more detailed information about the microbiota, especially in the deeper analysis of species, could provide more information about the changes in the gut microbiota composition. Diet might have a greater influence on gut microbiota. In line with this, we should acknowledge the limitation that we did not assess the differences in dietary structure levels between the two groups. The strength of this study was the thorough phenotypical characterization of participants regarding the subjective and quantitative assessment of gastrointestinal parameters, as well as the controlled randomized design with a relatively long intervention period. In contrast to previous studies, we applied 16S rRNA amplicon profiling and quantitatively targeted bacterial metabolomics, which allowed us to understand both the gut microbiota response and bacterial metabolites to gain more information about the host–gut microbiota metabolic interactions in response to LcS.
5. Conclusion
Our study demonstrated that although LcS supplementation did not induce major changes in the global gut microbiome, daily LcS intake had favourable effects on the NMSs and specific gastrointestinal symptoms in PD patients.
Author contributions
Q. X., X. Y., and Y. Q. were responsible for the design and supervision of the study and revised the manuscript. X. Y., X. H., S. X., and Y. Q. contributed to participant recruitment, clinical evaluation, and data acquisition. X. H., S. X., Y. Z., C. M., Y. L., and P. A. contributed to interpretation of data and statistics. Y. S. contributed to the randomization and masking. Z. Y. contributed to the validation of the HPLC-FLD method and the measurement of plasma L-tyrosine. X. Y. drafted the manuscript. All authors contributed to the final submitted version and agreed to be responsible for all the work.
Data availability
Sequences generated and analyzed during this study are accessible from the National Center for Biotechnology Information (NCBI) Sequence Read Archive (SRA) under the accession code PRJNA826738 after the date 31 October 2022. The key data are included in this published article. Other datasets are available from the corresponding author upon reasonable request.
Conflicts of interest
The authors have declared that no competing interests exist. None of the authors have any financial relationship with the funding body, Yakult Honsha Co., Ltd, and have no interest in the sales of this product under investigation.
Acknowledgements
This study was funded by Yakult Honsha Co., Ltd, Tokyo, Japan. We are grateful to the patients with PD and their caregivers for their participation in this research.
References
- A. H. V. Schapira, K. R. Chaudhuri and P. Jenner, Non-motor features of Parkinson disease, Nat. Rev. Neurosci., 2017, 18, 435–450 CrossRef CAS PubMed.
- K. Seppi, K. Ray Chaudhuri, M. Coelho, S. H. Fox, R. Katzenschlager, S. Perez Lloret, D. Weintraub and C. Sampaio, Update on treatments for nonmotor symptoms of Parkinson's disease-an evidence-based medicine review, Mov. Disord., 2019, 34, 180–198 CrossRef PubMed.
- M. Elfil, S. Kamel, M. Kandil, B. B. Koo and S. M. Schaefer, Implications of the Gut Microbiome in Parkinson's Disease, Mov. Disord., 2020, 35, 921–933 CrossRef CAS PubMed.
- Y. Qian, X. Yang, S. Xu, P. Huang, B. Li, J. Du, Y. He, B. Su, L. M. Xu, L. Wang, R. Huang, S. Chen and Q. Xiao, Gut metagenomics-derived genes as potential biomarkers of Parkinson's disease, Brain, 2020, 143, 2474–2489 CrossRef PubMed.
- Y. Qian, X. Yang, S. Xu, C. Wu, Y. Song, N. Qin, S. D. Chen and Q. Xiao, Alteration of the fecal microbiota in Chinese patients with Parkinson's disease, Brain, Behav., Immun., 2018, 70, 194–202 CrossRef PubMed.
- M. D. M. Teran, D. Perez Visñuk, G. Savoy de Giori, A. de Moreno de LeBlanc and J. G. LeBlanc, Neuroprotective effect of thiamine-producing lactic acid bacteria in a murine Parkinsonian model, Food Funct., 2022, 13, 8056–8067 RSC.
- M. Barichella, C. Pacchetti, C. Bolliri, E. Cassani, L. Iorio, C. Pusani, G. Pinelli, G. Privitera, I. Cesari, S. A. Faierman, R. Caccialanza, G. Pezzoli and E. Cereda, Probiotics and prebiotic fiber for constipation associated with Parkinson disease: An RCT, Neurology, 2016, 87, 1274–1280 CrossRef CAS PubMed.
- A. Ibrahim, R. A. R. Ali, M. R. A. Manaf, N. Ahmad, F. W. Tajurruddin, W. Z. Qin, S. H. M. Desa and N. M. Ibrahim, Multi-strain probiotics (Hexbio) containing MCP BCMC strains improved constipation and gut motility in Parkinson's disease: A randomised controlled trial, PLoS One, 2020, 15, e0244680 CrossRef CAS PubMed.
- A. H. Tan, S. Y. Lim, K. K. Chong, A. M. Maa, J. W. Hor, J. L. Lim, S. C. Low, C. W. Chong, S. Mahadeva and A. E. Lang, Probiotics for Constipation in Parkinson Disease: A Randomized Placebo-Controlled Study, Neurology, 2021, 96, e772–e782 CrossRef CAS PubMed.
- D. Georgescu, O. E. Ancusa, L. A. Georgescu, I. Ionita and D. Reisz, Nonmotor gastrointestinal disorders in older patients with Parkinson's disease: is there hope?, Clin. Interventions Aging, 2016, 11, 1601–1608 CrossRef CAS PubMed.
- A. C. Ouwehand, M. M. Invernici, F. A. C. Furlaneto and M. R. Messora, Effectiveness of Multistrain Versus Single-strain Probiotics: Current Status and Recommendations for the Future, J. Clin. Gastroenterol., 2018, 52, S35–S40 CrossRef CAS PubMed.
- A. H. Tan, J. W. Hor, C. W. Chong and S. Y. Lim, Probiotics for Parkinson's disease: Current evidence and future directions, JGH Open, 2021, 5, 414–419 CrossRef PubMed.
- A. H. Tan, S. Y. Lim and A. E. Lang, The microbiome-gut-brain axis in Parkinson disease - from basic research to the clinic, Nat. Rev. Neurol., 2022, 18, 476–495 CrossRef PubMed.
- E. J. Goldstein, K. L. Tyrrell and D. M. Citron, Lactobacillus species: taxonomic complexity and controversial susceptibilities, Clin. Infect. Dis, 2015, 60(Suppl 2), S98–107 CrossRef CAS PubMed.
- D. Hill, I. Sugrue, C. Tobin, C. Hill, C. Stanton and R. P. Ross, The Lactobacillus casei Group: History and Health Related Applications, Front. Microbiol., 2018, 9, 2107 CrossRef PubMed.
- E. Cassani, G. Privitera, G. Pezzoli, C. Pusani, C. Madio, L. Iorio and M. Barichella, Use of probiotics for the treatment of constipation in Parkinson's disease patients, Minerva Gastroenterol. Dietol., 2011, 57, 117–121 CAS.
- A. J. Hughes, S. E. Daniel, L. Kilford and A. J. Lees, Accuracy of clinical diagnosis of idiopathic Parkinson's disease: a clinico-pathological study of 100 cases, J. Neurol., Neurosurg. Psychiatry, 1992, 55, 181–184 CrossRef CAS PubMed.
- D. A. Drossman, The functional gastrointestinal disorders and the Rome III process, Gastroenterology, 2006, 130, 1377–1390 CrossRef PubMed.
- C. L. Tomlinson, R. Stowe, S. Patel, C. Rick, R. Gray and C. E. Clarke, Systematic review of levodopa dose equivalency reporting in Parkinson's disease, Mov. Disord., 2010, 25, 2649–2653 CrossRef PubMed.
- M. R. Blake, J. M. Raker and K. Whelan, Validity and reliability of the Bristol Stool Form Scale in healthy adults and patients with diarrhoea-predominant irritable bowel syndrome, Aliment. Pharmacol. Ther., 2016, 44, 693–703 CrossRef CAS PubMed.
- K. Knudsen, K. Krogh, K. Østergaard and P. Borghammer, Constipation in parkinson's disease: Subjective symptoms, objective markers, and new perspectives, Mov. Disord., 2017, 32, 94–105 CrossRef PubMed.
- T. H. Mertsalmi, V. T. E. Aho, P. A. B. Pereira, L. Paulin, E. Pekkonen, P. Auvinen and F. Scheperjans, More than constipation - bowel symptoms in Parkinson's disease and their connection to gut microbiota, Eur. J. Neurol., 2017, 24, 1375–1383 CrossRef CAS PubMed.
- M. S. Cirstea, A. C. Yu, E. Golz, K. Sundvick, D. Kliger, N. Radisavljevic, L. H. Foulger, M. Mackenzie, T. Huan, B. B. Finlay and S. Appel-Cresswell, Microbiota Composition and Metabolism Are Associated With Gut Function in Parkinson's Disease, Mov. Disord., 2020, 35, 1208–1217 CrossRef CAS PubMed.
- S. Lin, S. Zhao, J. Liu, J. Zhang, C. Zhang, H. Hao, Y. Sun, J. Cai, Y. Yang, Y. Ma, Y. Li, J. Wang and A. Ma, Efficacy of proprietary Lactobacillus casei for anti-tuberculosis associated gastrointestinal adverse reactions in adult patients: a randomized, open-label, dose-response trial, Food Funct., 2020, 11, 370–377 RSC.
- G. M. Pontone and K. A. Mills, Optimal Treatment of Depression and Anxiety in Parkinson's Disease, Am. J. Geriatr. Psychiatry, 2021, 29, 530–540 CrossRef PubMed.
- D. Aarsland, S. Påhlhagen, C. G. Ballard, U. Ehrt and P. Svenningsson, Depression in Parkinson disease–epidemiology, mechanisms and management, Nat. Rev. Neurol., 2011, 8, 35–47 CrossRef PubMed.
- A. Schrag and R. N. Taddei, Depression and Anxiety in Parkinson's Disease, Int. Rev. Neurobiol., 2017, 133, 623–655 CrossRef PubMed.
- D. Benton, C. Williams and A. Brown, Impact of consuming a milk drink containing a probiotic on mood and cognition, Eur. J. Clin. Nutr., 2007, 61, 355–361 CrossRef CAS PubMed.
- A. V. Rao, A. C. Bested, T. M. Beaulne, M. A. Katzman, C. Iorio, J. M. Berardi and A. C. Logan, A randomized, double-blind, placebo-controlled pilot study of a probiotic in emotional symptoms of chronic fatigue syndrome, Gut Pathog., 2009, 1, 6 CrossRef.
- M. Takada, K. Nishida, A. Kataoka-Kato, Y. Gondo, H. Ishikawa and K. Suda,
et al.Probiotic Lactobacillus casei strain Shirota relieves stress-associated symptoms by modulating the gut-brain interaction in human and animal models, Neurogastroenterol. Motil., 2016, 28, 1027–1036 CrossRef CAS.
- X. Zhang, S. Chen, M. Zhang, F. Ren, Y. Ren and Y. Li,
et al., Effects of Fermented Milk Containing Lacticaseibacillus paracasei Strain Shirota on Constipation in Patients with Depression: A Randomized, Double-Blind, Placebo-Controlled Trial, Nutrients, 2021, 13, 2238 CrossRef CAS PubMed.
- O. R. Tamtaji, M. Taghizadeh, R. Daneshvar Kakhaki, E. Kouchaki, F. Bahmani, S. Borzabadi, S. Oryan, A. Mafi and Z. Asemi, Clinical and metabolic response to probiotic administration in people with Parkinson's disease: A randomized, double-blind, placebo-controlled trial, Clin. Nutr., 2019, 38, 1031–1035 CrossRef CAS PubMed.
- H. Sun, F. Zhao, Y. Liu, T. Ma, H. Jin, K. Quan, B. Leng, J. Zhao, X. Yuan, Z. Li, F. Li, L. Y. Kwok, S. Zhang, Z. Sun, J. Zhang and H. Zhang, Probiotics synergized with conventional regimen in managing Parkinson's disease, npj Parkinson's Dis., 2022, 8, 62 CrossRef CAS PubMed.
- X. Yang, P. Ai, X. He, C. Mo, Y. Zhang, S. Xu, Y. Lai, Y. Qian and Q. Xiao, Parkinson’s Disease Is Associated with Impaired Gut-Blood Barrier for Short-Chain Fatty Acids, Mov. Disord., 2022, 37, 1634–1643 CrossRef CAS PubMed.
- G. Zoppi, M. Cinquetti, A. Luciano, A. Benini, A. Muner and E. Bertazzoni Minelli, The intestinal ecosystem in chronic functional constipation, Acta Paediatr., 1998, 87, 836–841 CrossRef CAS.
- C. Wang, S. Nagata, T. Asahara, N. Yuki, K. Matsuda, H. Tsuji, T. Takahashi, K. Nomoto and Y. Yamashiro, Intestinal Microbiota Profiles of Healthy Pre-School and School-Age Children and Effects of Probiotic Supplementation, Ann. Nutr. Metab., 2015, 67, 257–266 CrossRef CAS PubMed.
- K. Matsumoto, T. Takada, K. Shimizu, K. Moriyama, K. Kawakami, K. Hirano, O. Kajimoto and K. Nomoto, Effects of a probiotic fermented milk beverage containing Lactobacillus casei strain Shirota on defecation frequency, intestinal microbiota, and the intestinal environment of healthy individuals with soft stools, J. Biosci. Bioeng., 2010, 110, 547–552 CrossRef CAS PubMed.
- S. Nagata, T. Asahara, T. Ohta, T. Yamada, S. Kondo, L. Bian, C. Wang, Y. Yamashiro and K. Nomoto, Effect of the continuous intake of probiotic-fermented milk containing Lactobacillus casei strain Shirota on fever in a mass outbreak of norovirus gastroenteritis and the faecal microflora in a health service facility for the aged, Br. J. Nutr., 2011, 106, 549–556 CrossRef CAS PubMed.
- V. De Preter, T. Vanhoutte, G. Huys, J. Swings, L. De Vuyst, P. Rutgeerts and K. Verbeke, Effects of Lactobacillus casei Shirota, Bifidobacterium breve, and oligofructose-enriched inulin on colonic nitrogen-protein metabolism in healthy humans, Am. J. Physiol.: Gastrointest. Liver Physiol., 2007, 292, G358–G368 CrossRef CAS PubMed.
- F. Nagao, M. Nakayama, T. Muto and K. Okumura, Effects of a fermented milk drink containing Lactobacillus casei strain Shirota on the immune system in healthy human subjects, Biosci., Biotechnol., Biochem., 2000, 64, 2706–2708 CrossRef CAS PubMed.
- F. Gu, Y. Wu, Y. Liu, M. Dou, Y. Jiang and H. Liang, Lactobacillus casei improves depression-like behavior in chronic unpredictable mild stress-induced rats by the BDNF-TrkB signal pathway and the intestinal microbiota, Food Funct., 2020, 11, 6148–6157 RSC.
- Y. Asano, T. Hiramoto, R. Nishino, Y. Aiba, T. Kimura, K. Yoshihara, Y. Koga and N. Sudo, Critical role of gut microbiota in the production of biologically active, free catecholamines in the gut lumen of mice, Am. J. Physiol.: Gastrointest. Liver Physiol., 2012, 303, G1288–G1295 CrossRef CAS PubMed.
- S. P. van Kessel, A. K. Frye, A. O. El-Gendy, M. Castejon, A. Keshavarzian, G. van Dijk and S. El Aidy, Gut bacterial tyrosine decarboxylases restrict levels of levodopa in the treatment of Parkinson's disease, Nat. Commun., 2019, 10, 310 CrossRef PubMed.
- T. Nagatsu, A. Nakashima, H. Ichinose and K. Kobayashi, Human tyrosine hydroxylase in Parkinson's disease and in related disorders, J. Neural Transm., 2019, 126, 397–409 CrossRef CAS PubMed.
|
This journal is © The Royal Society of Chemistry 2023 |
Click here to see how this site uses Cookies. View our privacy policy here.