DOI:
10.1039/D3FO02832A
(Paper)
Food Funct., 2023,
14, 9194-9203
Designing sustainable weaning foods for developing countries: not only a matter of nutrients†
Received
12th July 2023
, Accepted 24th September 2023
First published on 25th September 2023
Abstract
Blended complementary foods from cereals and high-protein sources are used worldwide to cope with infants’ malnutrition. However, the usefulness of the food matrix during traditional processes reaches suboptimal effectiveness due to cereal gelatinization and viscosity, which reduce consumption. The interplay between nutritional and physical qualities needed for weaning children presents further significant constraints. A combination of processing methods can improve and optimize the overall product quality. This paper investigated the nutritional, functional, and anti-nutritional factors of a complementary infant porridge made by combining fermented sorghum flour with germinated bottle gourd seed flour. Overall, the combination improved the functional and physical properties of the porridge suitable for children of 10 months and over. A serving of 100 g would contribute 115–145% and 23–31% of the recommended nutritional intake of protein and energy, respectively, for low breast milk energy between 6–24 months. The results demonstrate that a combination of strategies and technologies are needed to balance nutritional and physical quality.
1 Introduction
Climate resilient crops (CRCs) in the small grains family, such as sorghum, millets, and amaranth, are often used to make weaning and complementary foods in Sub-Saharan Africa (SSA). These crops deserve special research attention in view of the current climate change. Within the group of CRCs, sorghum is particularly promising due to its nutritional superiority and functional qualities, which include bioactive compounds, dietary fibre, vitamin B, and minerals (potassium, iron, zinc).1,2 Sorghum is an energy-dense cereal but is limited in essential amino acids and protein digestibility. Hence, its monotonous consumption has been associated with protein-energy (PEM) malnutrition in SSA.3 In PEM, consumption of both energy and protein is insufficient leading to a form of hidden hunger and stunted growth in other cases kwashiorkor or marasmus.4
The supply of animal protein is insufficient to meet the protein needs in resource-limited communities, stimulating the research to efficiently combat PEM via cost-effective strategies. Using protein from locally available underutilized high-protein oilseeds and legumes is the most pragmatic choice. Food-to-food fortification (FtFF) by blending nutrient-limited cereal-based traditional complementary foods (TCFs) with nutrient-dense ingredients, has been advocated as such a cost-effective strategy.5 In this respect,6 suggested adding oil to cereal-based complementary foods to improve energy and nutrient density. Furthermore, oil reduces the high glutinous nature of the porridges.7 So far, many reports investigated the effect of legume incorporation in complementary food but studies on promising oil-seed protein have received little attention, especially regarding the ubiquitous cucurbits, belonging to the Cucurbitaceae family and have good potential for food blending. To illustrate, seeds of the bottle gourd (Lagenaria siceraria), also known as calabash, bottle or white-flowered gourd, contain essential amino acids, i.e., threonine, valine, leucine, lysine, methionine and tyrosine, at levels higher than the Food and Agriculture Organization recommendations.8 Additionally, palmitic acid, linoleic acid, oleic acid and stearic acid have been identified in bottle gourd seeds.9 Besides the protein value, adding bottle gourd seed flour to porridge is a practical option for increasing its fat content. Repurposing bottle gourd seeds by integrating them into nutrient-limiting complementary foods has the potential to enhance product functionality and nutritional value.
A weaning cereal porridge with 20% dry matter can supply the Recommended Daily Intake (RDI) of 3000 kJ of energy in four feedings of 250 ml each.10 Unfortunately, concentrated weaning cereal porridges become highly viscous, making them difficult to swallow and digest for infants aged 6 to 12 months.11 To enable consumption, water is added to the viscous product, resulting in over-dilution and hence loss in energy-protein density causing subsequent malnutrition.12
Other cost-effective strategies to efficiently combat malnutrition are based on food processing methods. Traditional food bioprocessing methods such as steeping, germination, malting and fermentation can improve the nutritional value, bio-accessibility of nutrients, and reduce viscosity and antinutritional compound content.13,14 The use of optimized methods has the potential to further enhance nutrition and physical product quality and could be effective in the processing of complementary infant foods. Especially germination and fermentation are widely used in sorghum-producing regions in processing of weaning foods.15 Combinations of fermentation and germination, when applied to different ingredients of the same product, are expected to improve product quality even more.
To date, a global framework of the pathway of optimization of cereal-legume complementary food exists and the nutritional, functional and microbial characteristics of sorghum porridges have been widely investigated.15,16 Still unexplored is how a fermentation and germination combination on more than one ingredient impacts the nutritional density and the corresponding functionality of a traditionally processed complementary food. Therefore, this research seeks to answer these questions: (i) what is the synergistic effect of processing technologies (i.e., fermentation and germination) on nutrient density and physical properties of traditional complementary foods, and (ii) can complementary foods formulated using treated oilseed ingredients and state-of-the-art food processing methods meet the complementary food age recommendation?
2 Methodology
2.1 Sample preparation
2.1.1 Raw materials.
Hulled, finely milled sorghum meal and matured bottle gourd seeds were purchased from a local market in Zimbabwe. Fig. 1 schematically shows the sample preparation.
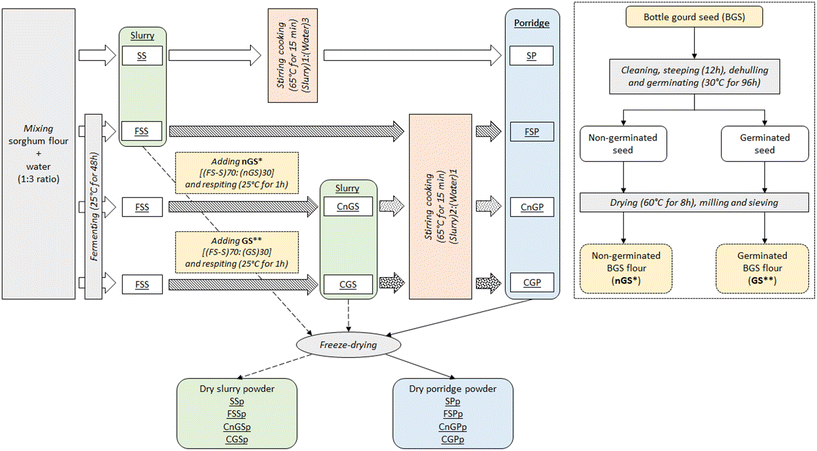 |
| Fig. 1 Ingredient treatment and experimental design showing the different process combinations: germination and fermentation from sorghum and bottle gourd seed. *The process for obtaining BGS flour is shown in the lower left box. SS: sorghum slurry, FSS: fermented sorghum slurry; SP: sorghum porridge, FSP: fermented sorghum porridge, CnGP: fermented and blended with non-germinated seed porridge, CGP: fermented and blended with germinated seed porridge, CnGS: blended sorghum & non-germinated seed and CGS: blended sorghum & germinated seed. | |
2.1.2 Fermentation.
Sorghum flour was mixed with lukewarm tap water at a 1
:
3 ratio using a regular cooking spoon until uniform consistency. Next, the slurry was incubated at 25 °C for 48 h in the dark for spontaneous fermentation.
2.1.3 Germination.
Bottle gourd seeds were spread evenly on a wet layer of cotton wool in a Petri dish and incubated at 30 °C for 96 h in the dark. The seeds were watered every 12 h with MilliQ water. Next, the germinated bottle gourd seeds were dried in an electric oven at 60 °C, milled using a cryomiller (SPEX SamplePrep Freezer/Cryo Mill 6875D), after which the resulting flour was passed through an 80-mesh sieve. The flour fraction <80 μm was retained and stored in an air-tight container at −20 °C until further use.
2.1.4 Sorghum porridge preparation.
Sorghum porridge was produced by mixing sorghum flour and lukewarm water in a 1
:
3 ratio (16.7% solids) to obtain a homogenous, lump-free slurry. The slurry was then either immediately cooked (unfermented porridge) or subjected to spontaneous fermentation (as described in section 2.1.2) and then cooked. To obtain blended samples, either non-germinated or germinated bottle gourd seed (BGS) flour was added to the fermented slurry. The four slurries (i.e., unfermented, fermented, fermented with non-germinated BGS, and fermented with germinated BGS) were heated to 65 °C on a heating element at 300 °C while stirring using a magnetic and an automatic stirrer (IKA eurostar 20 digital) rotating at 200 and 65 rpm, respectively, to ensure proper mixing of the whole porridge. Part of the cooked porridge was then cooled in a water bath to 40 °C and directly analysed for viscosity and nutritional properties, while the remainder was instantly freeze-dried for further analysis of functional properties (2 samples per analysis with 3 replicates per sample).
2.2 Characterization of freeze-dried sorghum porridge
2.2.1 Functional properties.
The freeze-dried porridge was milled to a powder and kept at 4 °C before analysis of functional properties. Bulk density (BD), water absorption capacity (WAC), water solubility capacity (WSC) and oil absorption capacity (OAC) were determined using the methods previously described.17
2.2.2 Alpha-amylase activity.
The α-amylase activity was determined using a colourimetric method developed by Megazyme (Wicklow, Ireland), which consists of hydrolysis of sample extracts for grains with the α-amylase. Alpha-amylase activity is expressed in Ceralpha units per gram of dry matter (U g−1 DM; ICC Standard No. 303, Megazyme International, Ireland).
2.2.3 Phytic acid content.
Phytic acid content was measured using a Megazyme kit (Phytic acid (phytate)/total phosphorus). The extraction process was carried out starting from 1 g of freeze-dried sample, followed by enzymatic dephosphorylation and the colourimetric determination of phosphorus.
2.3 Porridge biochemical analysis
2.3.1 Proximate composition.
Moisture content was analyzed using method 925.09 (AOAC, 2000). The pH was measured with a pH meter (pH1002 VWR phenomenal) before and after cooking all samples. Protein content (N × 6.25) was determined by the Dumas combustion method using an analyzer (EA 112 NC, Thermo Fisher Scientific Inc., Waltman, USA) following the manufacturer's protocol. Cellulose and D-methionine were used to prepare the control and calibration curves, respectively. Lipid contents were determined by the method of extraction with the Soxhlet method with petroleum ether as the extraction solvent. Total dietary fibre was measured using a commercial Megazyme kit (K-TDFR, Megazyme Int., Wicklow, Ireland); ash and protein residues were corrected for corresponding soluble dietary fibre (SDF) and insoluble dietary fibre (IDF) values. Total dietary fibre (TDF) was calculated as the sum of SDF and IDF. The carbohydrate content (g per 100 g) was calculated by difference. Energy values were then calculated using Atwater's conversion factors,18 as follows:
2.4 Viscosity
Viscosity was measured using a GmbH viscometer (Anton Paar, GmbH, Austria) equipped with a 50 mm measuring plate and a 1 × 0.5, Groove probe (A-8054 Graz, Anton Paar, GmbH, Austria). Approximately 10 g of porridge was placed on the rheometer plate, gently squeezed to 1 mm thickness and subjected at variable rates increasing from 0.1 to 1000 s−1 at a controlled temperature of 40 °C using a Peltier plate. The test, shear rate range, plate and probe settings were chosen following previous methods.12
2.5 Simulated in vitro gastrointestinal digestion
Simulated gastric fluid (SGF) and simulated intestinal fluid (SIF) were used to carry out the in vitro digestion according to previous methods.19 Aliquots were taken at different time points of gastric and intestinal digestion, i.e., SGP: 0, 2 h and SIP at 15, 30, 60 and 120 min. Enzyme inactivation was performed by the addition of absolute ethanol for starch digestion then rested for 30 min at room temperature before centrifugation at 10
000g for 10 min. Enzyme inactivation for protein digestion was performed by the addition of 25 μL pefabloc© (0.1 M). Samples were left for 30 min and at room temperature, after which 0.83 mL 5% TCA was added, and then centrifuged at 10
000g for 10 min. Supernatants were collected and stored at (−20° C) for further analysis. As a blank, 5 ml MilliQ water was digested.
2.5.1 Determination of starch hydrolysis.
An aliquot of 0.1 ml of the supernatant obtained after the addition of ethanol and centrifugation was mixed with an amyloglucosidase solution (27.17 U ml−1) in 0.1 M sodium acetate buffer (pH 4.8) and incubated at 37 °C for 1 h. The amount of glucose was then quantified using a Megazyme D-glucose assay kit (GOPOD FORMAT, K-GLUC, Megazyme Inc., Bray, Ireland). The glucose content was multiplied by a factor of 0.9 to obtain the corresponding amount of starch, and the results were expressed as g of hydrolyzed starch per 100 g of dry starch.
2.5.2 Determination of protein hydrolysis.
The free amino groups (NH2) in TCA samples were determined using the ortho-phthalaldehyde (OPA) method. Non-digested samples were hydrolyzed with 6 M HCl and incubated at 110 °C for 24 h, after which the free amino groups were assessed by OPA.
2.6 Statistical analysis
All results are given as means ± standard deviation of three independent determinations of each parameter. One-way analysis of variance (ANOVA) with the Tukey test was used to compare significant differences between means of triplicate readings. Pearson correlation coefficients were used to determine relationships among variables, differences were considered to be significant at p < 0.05. Statistical analyses were performed using the Statistical Package for Social Sciences (SPSS v23.0) software.
3 Results and discussion
3.1 Effect of process combinations on functional properties of freeze-dried porridges
3.1.1 Water and oil absorption capacity.
Water absorption capacity (WAC) indicates the amount of water available for gelatinization. Its value of unblended and blended flours ranged from 4.40–5.38 g g−1 DW (Table 1). The difference is marginal when considering single treatments and their combination. Only non-germinated blended flour had a significantly lower WAC. Fermentation alone and the combination with germination slightly decreased the WAC when compared to plain sorghum flour at 6.7% and 8%, respectively. These findings corroborate the reported WAC of fermented sorghum flours in other studies.20 The lower WAC of blended flours could be attributed to the reduction in hydrophilic constituents such as carbohydrates and polar amino acids than in native proteins that are used up during germination and by microorganisms during fermentation. A lower WAC is advantageous for producing lower-viscosity infant complementary porridges and extending shelf life.21
Table 1 Functional properties of blended flour samples
Parameters |
WAC (g g−1) |
WSI (g g−1) |
OAC (g g−1) |
Bulk density (g ml−1) |
pH |
α-Amylase activity |
Different superscripts in the same column denote a statistically significant difference (P < 0.05). SF: sorghum flour, FSF: fermented sorghum flour, CnG: blended with non-germinated seed, CG: blended with germinated seed. |
SF |
5.38 ± 0.75b |
3.01 ± 0.20a |
1.70 ± 0.04a |
0.86 ± 0.03b |
6.33 ± 0.03c |
0.31 ± 0.15a |
FSF |
5.02 ± 0.17a,b |
4.74 ± 1.83b |
1.92 ± 0.11a |
0.71 ± 0.02a |
3.84 ± 0.07a |
3.37 ± 1.33b |
CnG |
4.40 ± 0.33a |
5.57 ± 0.82b,c |
1.72 ± 0.04a |
0.74 ± 0.06a |
4.42 ± 0.01b |
3.12 ± 0.44b |
CG |
4.94 ± 0.26a,b |
6.94 ± 0.40c |
1.84 ± 0.08a |
0.71 ± 0.03a |
4.51 ± 0.05b |
2.84 ± 0.42b |
Oil absorption capacity (OAC) is the binding of fat by the nonpolar side chain of proteins that bind the oil hydrocarbon chains.22 OAC was not significantly different among samples. The results contrast with findings from other research.20 The rate of oil absorption was expected to be higher for the combined processed samples since the samples had higher protein contents. A possible explanation is that the protein's conformational characteristics, polarity, and degree of interaction with oil affected the absorption capacities. Due to specific fermentation and germination conditions, changes in the chemical composition may affect the spatial arrangement of molecules and therefore the functional properties of flours. A higher OAC would be useful in infant food formulation where an oil-holding property is an important consideration because it is an indication of flavor retention and increase of the mouth feel.
3.1.2 Water solubility index and bulk density.
The water solubility index (WSI) ranged from 3.01–6.94 g g−1 and the bulk density was 0.71–0.86 g ml−1. The higher values of the WSI of CnG and CG indicate a high digestibility of food, which is ideal for infant foods. Fermentation and germination significantly reduced the bulk density of the blended samples by 17% for both fermented and blended flour. The reduced bulk density could be due to the breakdown of starch and protein during fermentation and seed growth in germination. A low bulk density enables more input of the less dense flour to obtain an optimum thickness, thus rendering a more nutrient-rich product. The decrease in bulk density is advantageous for complementary infant foods.23
3.1.3 Alpha-amylase activity and pH.
Germination and fermentation significantly (P ≤ 0.05) reduced the pH and increased the α-amylase activity from 6.33 to 3.48 and 0.31 to 3.37 CU g−1, respectively. Evidently, the microbes during fermentation and the hydration activation during germination caused an increase in endogenous α-amylases. The partial hydrolysis of carbohydrates during the processes boosts the energy sources for microorganisms and yeasts, which support the growth of lactic acid bacteria and subsequently reduce the pH.24 The pH shift caused by fermentation can cause protein denaturation and impacts other functional properties such as WAC. The combination of germination and fermentation of sorghum can improve flavour and safety of infant food. This is due to the low pH levels obtained by fermentation, which are known to inhibit the proliferation of foodborne pathogens and contribute to flavour enhancement.25–27
3.2 Effect of flour blends on biochemical properties
The proximate composition and estimated protein and energy intakes of infants (6–24 months) of blended fermented sorghum porridges are shown in Table 2.
Table 2 Proximate composition and estimated intakes of porridge samples consisting of sorghum flour and combinations thereof
Porridge type |
Nutritional composition (g per 100 g) |
kJ per 100 g |
Energy intake (kJ day−1) |
Protein intake (g day−1) |
Moisture |
Fibre |
Ash |
Protein |
Fat |
Carbohydrate |
Energy |
6–8 months |
9–11 months |
12–24 months |
6–8 months |
9–11 months |
12–24 months |
Different superscript in the same column denotes a statistically significant difference (P < 0.05). nGS: non-germinated seed flour, GS: germinated seed flour, SP: sorghum porridge FSP: fermented sorghum porridge (48 h fermentation), CnGP: blended sorghum porridge (48 h fermentation + non-germinated seed flour), CGP: blended sorghum porridge (48 h fermentation + germinated seed flour), BME: breast milk energy, RDI: recommended daily intake. Estimated protein and energy intakes were calculated using 16.67 g solid contents per 100 g porridge at 3 meals per day for functional gastric capacities of 249 g, 285 g and 345 g per meal for aged 6–8, 9–11 and 12–24 months children respectively.39 |
SP |
9.43 ± 1.06c |
1.92 ± 0.36a |
0.25 ± 0.13a |
10.63 ± 0.4a |
2.04 ± 0.12a |
74.37 ± 1.06c |
1497.8 ± 20.3a |
1865.1 |
2134.8 |
2584.2 |
13.24 |
15.15 |
18.35 |
FSP |
6.84 ± 1.52b |
1.91 ± 0.12a |
0.83 ± 0.99a |
9.84 ± 2.2a |
2.81 ± 0.85a |
76.40 ± 2.15c |
1547.6 ± 40.7b |
1927.2 |
2205.8 |
2670.2 |
12.25 |
14.02 |
16.97 |
CnGP |
4.18 ± 1.40a |
1.78 ± 0.19a |
1.08 ± 0.19a |
17.94 ± 0.96b |
14.86 ± 1.01c |
58.66 ± 1.35a |
1839.6 ± 44.4d |
2290.8 |
2622.0 |
3173.9 |
22.34 |
25.57 |
30.96 |
CGP |
3.69 ± 0.19a |
2.08 ± 0.75a |
1.61 ± 1.30a |
18.25 ± 0.82b |
10.68 ± 1.21b |
62.50 ± 0.74b |
1751.7 ± 28.3c |
2181.4 |
2496.7 |
3022.4 |
22.72 |
26.01 |
31.48 |
nGS |
ND
|
10.53 ± 1.54b |
ND
|
35.63 ± 0.82c |
48.55 ± 2.05d |
ND
|
ND
|
ND
|
ND
|
ND
|
ND
|
ND
|
ND
|
GS |
ND
|
14.8 ± 2.55c |
ND
|
35.18 ± 2.15c |
36.69 ± 0.82e |
ND
|
ND
|
ND
|
ND
|
ND
|
ND
|
ND
|
ND
|
RDI Low BME |
|
2310.0 |
2933.0 |
4301.0 |
5.20 |
6.70 |
9.10 |
RDI Average BME |
|
1490.0 |
2004.0 |
3230.0 |
2.00 |
3.10 |
5.00 |
3.2.1 Moisture content.
Moisture contents of the CFs ranged between 3.69–9.43 g per 100 g, with, as expected, higher protein, fat and fibre contents in the samples with lower moisture contents (Table 2). These findings are consistent with the report of,28 which shows low moisture generally increases nutrient concentration. On the other hand, high moisture contents enhance microbial growth, which in turn causes spoilage and a low nutritional quality of the food products.29 Hence, a low moisture content is recommended if the flours are to be stored for instant porridge production.
3.2.2 Ash and fibre contents.
Ash contents ranged from 0.25–1.61 g per 100 g. The blended and non-blended porridges had low fibre contents ranging from 1.91 to 2.08 g per 100 g. Ash content increased sixfold from the unblended sample to the fermentation–germination combination and threefold for the fermentation-only method. This indicates that the combination treatments significantly increase the ash content of the samples, which is expected to parallel the mineral contents, which are crucial for infant wellbeing. There was no significant difference for fibre in the formulated porridges, not even with the addition of bottle gourd seed. Fibre is not preferable for complementary infant porridge due to its dietary bulk and indigestibility.30 The recommended nutrient intake (RNI) of fibre for children below 12 months cannot be determined from the literature due to a lack of data on adverse effects in this age group and concerns about the lack of ability to manage excess amounts. High fiber levels in weaning foods can lead to increased bulk, lower caloric density, irritation of the gut mucosa, and adverse absorption efficiency of other significant nutrients.31 However, for infants aged 12 months and older, the RNI daily target range of 10–15 g per 100 g (ref. 32) is not exceeded by three times a day consumption.
3.2.3 Protein and carbohydrate contents.
The protein content of fermentation–germination combination samples was significantly higher than that of only fermented samples, while the carbohydrate content of only fermentation was higher than the fermentation–germination combination. Combination methods caused a 71% increase in protein and a 15% decrease in carbohydrates when compared to unprocessed porridge (SP). The significant protein increase is largely attributed to the complementation of highly proteinaceous germinated bottle gourd seed. The blending of cereal with high protein ingredients has been shown to improve the protein content of single cereal-based infant foods and other formulated CFs such as Ogi, rice/Bambara/groundnut/carrot porridge and commercial Cerelac.33 This is also highlighted by the corresponding estimated protein intake, which is higher than the recommended nutritional intake for both low and average breast milk for all weaning age groups (Table 2). Fermentation alone did not cause a significant crude protein or carbohydrate content change as reported for other fermented seeds and legumes.34 The reduction in carbohydrates was expected due to the activation of α-amylase.35
3.2.4 Fat content.
The fat content of the fermentation–germination combination showed a fourfold increase when compared to fermentation alone. The 36.6% reduction in crude fat due to germination was to be expected because of the biochemical and physiological changes such as increased lipolytic enzyme activity for hydrolysis of triglycerides to fatty acids and glycerol.35 Therefore, the increase in fat was mainly due to the complementation with a proportion of the high seed oil ingredient. Although the fatty acid composition was not determined in this study, we expect that the essential fatty acid content was also increased because other research reports high contents of palmitic, stearic, linoleic and oleic acids,36 which are essential for organ function and brain development in infants.
3.2.5 Energy density.
The combination method realized a 16% increase in energy content, while CnGP showed higher energy density than CGP corresponding to the higher fat content of the CnGP. Fat contains twice the energy value of protein and carbohydrates.37 The inclusion of fat through the bottle gourd seeds increased the energy density of the blended porridges significantly, which has an added benefit as a transport vehicle for fat-soluble vitamins. CnGP had more α-amylase, which hydrolyses the amylose and amylopectin to dextrins and maltose, thus causing an increase in energy density. The energy content of all the porridges fell within the recommended values for children aged 9–11 months for a once-a-day consumption. Feeding frequency is also important in determining the extent to which energy and nutrient requirements are met.38 The calculated estimates for three-times-a-day consumption (Table 2) point out that a higher frequency of servings of the porridge blend would meet the RNI for children in the weaning age group (6–24 months). This suggests that bottle gourd seed flour can be added to increase energy, protein and fat contents, at an appropriate solid content for children aged 6–24 months.
3.2.6 Phytic acid of fermented and blended porridges.
The phytic acid content of the porridge blends decreased in the following order: CnGP > CGP > SP > FSP (Fig. 2). Phytic acid contents were higher when bottle gourd seed flour was added. Up to 19.3 g per 100 g of phytate was reported in unprocessed bottle gourd seed.40 The phytic acid content decreased with germination and fermentation, confirming that these processes cause significant (p < 0.05) phytic acid reduction. The phytic acid decrease is attributed to the increased activity of polyphenol oxidase, other catabolic enzymes and leaching into the sprouting medium.41 Reductions in phytic acid content from the oil seed flours as a result of fermentation and germination have been reported in the literature.13 The reduction in phytic acid is crucial to enable adequate availability of protein and minerals, which are of paramount importance when designing CFs that can mitigate malnutrition challenges, especially hidden hunger due to micronutrient deficiencies, particularly iron, zinc, iodine and vitamin A.42 However, it should be carefully considered whether the reduction is not also due to the formation of insoluble complexes between phytate and other nutrients, such as phytate-protein-mineral or phytate-protein,43 which can negatively affect the nutritional quality of CFs. Furthermore, in vitro and in vivo availability needs to be assessed to determine whether the mineral content in the blend meets the recommended values.
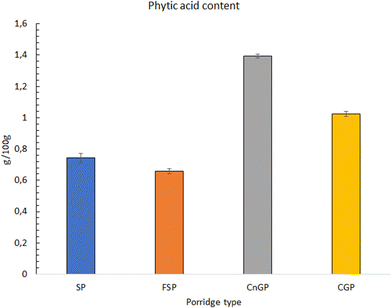 |
| Fig. 2 Phytic acid content of blended porridges. SP: sorghum porridge, FSP: fermented sorghum porridge (48 h fermentation), CnGP: blended sorghum porridge (48 h fermentation + non-germinated seed flour), CGP: blended sorghum porridge (48 h fermentation + germinated seed flour). | |
3.2.7 Viscosity.
The viscosity of all samples decreased with increasing shear rate (0.1–100 s−1), indicating that the porridges exhibited pseudo-plastic behaviour also known as shear thinning behaviour (Fig. 3). A similar trend was observed for conventionally cooked porridges of sorghum.44 At the same shear rate, the viscosity decreased in the order FSP > CnGP > CGP ∼ SP. During fermentation and germination, amylose and amylopectin are hydrolysed, resulting in significant absorption of water and reduced viscosity when preparing porridge. This is evidenced by the α-amylase content, which follows the same order. The fermented and germinated samples with higher amount of carbohydrates, are also more accessible to water and are crucial for increasing product swelling and viscosity. The reduced viscosity increases the nutrient density in weaning foods.24 However, the proper viscosity for children aged 6–9 months could not be achieved, so the current formulation is not appropriate for this age group. The shear rate in infant mouths dictates the swallowing abilities: 3–6 and 6–10 being the recommended viscosity for 6–10 months and 10–24 months respectively.45,46 The limited decrease in viscosity is suitable for children aged 10 months and older. Another way to reduce viscosity is the addition of a low pH, acidic ingredient such as fruit puree which promotes the hydrolysis of the starch granules into a thinner slurry. At lower pH, polysaccharide charges repel each other resulting in more liquid-like behaviour.47
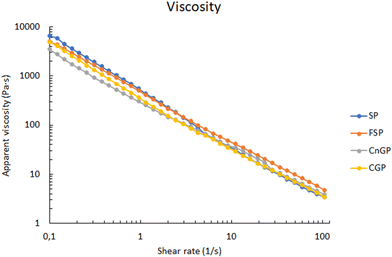 |
| Fig. 3 Viscosity of blended porridge samples. *Dry matter content 16.67%. | |
3.3
In vitro digestibility of porridges
3.3.1 Starch digestibility.
Fig. 4 reports on the kinetics of in vitro starch digestion of unfermented and fermented-blended complementary porridges. Starch in vitro digestibility was in the order FSP > SP > CnG > CGP. Data show that fermentation alone increased the starch hydrolysis, while the combination of fermentation–germination had the lowest value. CnG and CGP had the highest phytic acid content, which contributed to the reduction in digestibility of carbohydrates. Another factor that can cause disparities in starch hydrolysis and digestibility includes enzyme susceptibility due to the amylose/amylopectin ratio. A high content of amylopectin in grains renders them more enzyme-susceptible.48 Sorghum starch has more amylopectin than legumes and oil seeds.49 On the other hand, vegetable seed oil can also inhibit α-glucosidase and α-amylase due to the presence of liposoluble constituents,50 thus the limited starch hydrolysis is for blended samples. The higher protein content of the fermentation–germination samples may have affected the functional properties of starch by influencing the digestibility of both nutrients and also could have been responsible for their lower starch enzyme susceptibility. Protein bodies encapsulating starch granule surfaces can be an extra barrier to the availability of starch to amylase in vitro, thus decreasing digestibility.51 Phenolic compounds, in particular chlorogenic acid, syringic acid, quercetin and sinapic acid, have been identified in bottle gourd seed BGS.52 Chlorogenic acid reportedly inhibits enzyme activity by reducing the number of binding sites for enzymes to different extents during starch digestion.53 Though fermentation alone improved the digestibility, the levels are below the recommended values for CFs. Generally, in vitro digestibility of sorghum starch is the lowest among the cereals due to the strong association between the starch granules and proteins, and tannins.54 Sorghum has relatively high amounts of phenolic compounds; 3-deoxyanthocyanidins and tannins, which by cross-linking or binding of starch, limit starch accessibility to amylolytic enzymes.55 Varietal factors further impact sorghum digestion, namely differences in the sorghum prolamin i.e., kafirin composition and content, endosperm structure where the amorphous are more prone to enzymatic digestion than the corneous grains, particle size after milling and cooking length.56 Cooking polymerizes kafirins, which impedes starch gelatinization and digestibility.55 These findings show that further pretreatments need to be investigated to meet the required digestibility for use in complementary foods.
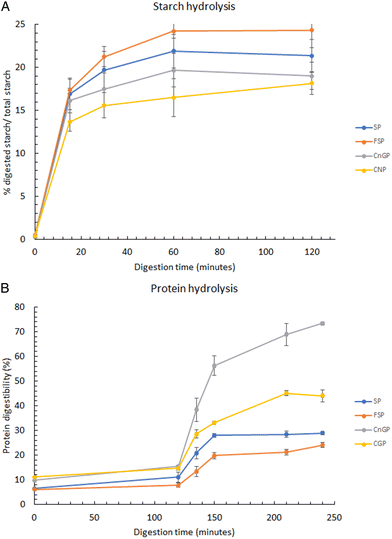 |
| Fig. 4 (A) Starch and protein (B) hydrolysis as a function of time during gastric and intestinal in vitro digestion for protein and intestinal phase only for starch. Values are mean ± SD of three independent samples. | |
3.3.2 Protein digestibility.
The combination of fermentation–germination increased protein digestibility, see Fig. 4B. In vitro protein digestibility was in the order CnGP > CGP > SP > FSP. The result that germination did not improve the protein digestibility of the seed flour in the combination blends was unexpected. In other words, the reduction of phytic acid caused by germination was expected to cause the reverse of the findings, where CGP proteins would be more digestible than CnGP. Such differences can be attributed to enzyme specificity during digestion, mode of action and conformational state of proteins.51 CnGP probably has more effective peptidases which produce amino acids than the germinated CGP, which were measured with OPA a specific method for amino acid determination, thus causing a higher output during intestinal in vitro digestion. However, the protein digestibility for CGP was higher than for SP and FSP, possibly due to increased protease hydrolysis activity, and protein solubility because of the hydrolysis of complex storage protein into more soluble amino acids by fermentation–germination and removal of protease inhibitors. Previous studies have shown that germination could activate protease hydrolytic activity leading to increased digestibility.57 Unblended sorghum samples SP and FSP had the lowest protein digestibility, probably due to the interaction of sorghum proteins with non-protein components protein-tannin complexes, enzyme inhibitors or changes in protein structural characteristics.41,58 Overall, the combination method proved to be an effective processing approach to add nutritional value for CFs.
4 Conclusion and recommendations
The combination of the traditional processing technologies of fermentation and germination improved the functional properties of the flour blend and subsequent nutritional and physical properties, cementing their applicability in infant complementary foods production. Neither fermentation nor germination alone is optimal in improving the functionalities of sorghum and bottle gourd seed flour blends. The combined effect of the “processing methods” and the “co-ingredient macronutrients” is a useful tool for further exploration of the design of affordable and diversified new complementary infant foods. Before undertaking in vivo studies, we recommend careful consideration of (i) interrelationships of processes when using highly nutritious underutilized crops from different regions, (ii) functionalities of various ingredients and target compounds – protein, fat and minerals (notably Fe and Zn) and (iii) designing a traditional complementary food using combinations of processing and raw materials to suit infants below the age of 10 months.
Author contributions
Luigi Moriconi: formal analysis, investigation, data curation, writing – review & editing. Elena Vittadini: funding acquisition, supervision, writing – review & editing. Anita R. Linnemann: conceptualization, supervision, visualization, writing – review & editing. Vincenzo Fogliano: funding acquisition, resources, supervision, writing – review & editing. Ruth T. Ngadze: project administration, conceptualization, methodology, supervision, visualization, writing – original draft, review & editing.
Conflicts of interest
The authors declare that they have no known competing financial interests or personal relationships that could have appeared to influence the work reported in this paper.
Acknowledgements
The authors would like to thank Nawa Penparkgoon for the help with the experiments.
References
- O. Ibidapo, F. Henshaw, T. Shittu and W. Afolabi, Bioactive components of malted millet (Pennisetum glaucum), Soy Residue “okara” and wheat flour and their antioxidant properties, Int. J. Food Prop., 2019, 22, 1886–1898 CrossRef CAS.
- K. N. Rai, C. L. L. Gowda, B. V. S. Reddy and S. Sehgal, Adaptation and potential uses of sorghum and pearl millet in alternative and health foods, Compr. Rev. Food Sci. Food Saf., 2008, 7, 320–396 CrossRef.
- M. A. Al-Gashanin and E. Y. Ghazwani, Knowledge, Attitude, and Practice of Weaning among Mothers in Najran Region, Saudi Arabia, 2021, J. Nutr. Metab., 2022, 6073878 Search PubMed.
- F. K. A. Nightingale, S. Sarathi, V. Hemavathy, J. A. Monisha and B. Pandian, Protein Energy Malnutrition-an Overview, Cardiometry, 2023, 585–588 Search PubMed.
- J. Kruger, J. R. N. Taylor, M. G. Ferruzzi and H. Debelo, What is food–to–food fortification? A working definition and framework for evaluation of efficiency and implementation of best practices, Compr. Rev. Food Sci. Food Saf., 2020, 19, 3618–3658 CrossRef.
- M. Arimond, S. Abbeddou, C. Kumwenda, H. Okronipa, J. Hemsworth, E. Y. Jimenez, E. Ocansey, A. Lartey, U. Ashorn, S. Adu-Afarwuah, S. A. Vosti, S. Y. Hess and K. G. Dewey, Impact of small quantity lipid-based nutrient supplements on infant and young child feeding practices at 18 months of age: results from four randomized controlled trials in Africa, Matern. Child Nutr., 2017, 13, 1–11 Search PubMed.
-
FAO/WHO/UNU, Energy and protein requirements. World Health Organization Technical Report Series 724, Geneva, Switzerland, 1991 Search PubMed.
- L. Hassan, N. Sani, S. Dangoggo and M. Ladan, Nutritional value OF Bottle Gourd (Lagenaria siceraria) Seeds, Global J. Pure Appl. Sci., 2008, 14(3), 301–306 CAS.
- C. Raza, A. Dildar, L. Inam, D. Parsa and S. Muhammad, Study of bioactivities of lipid content of fresh Lagenaria siceraria seeds pulp and identification of its chemical constituents, J. Med. Plants Res., 2014, 8, 1014–1020 CrossRef.
- M. J. R. Nout and P. O. Ngoddy, Technological aspects of preparing affordable fermented complementary foods, Food Control, 1997, 8, 279–287 CrossRef.
- D. A. Oladiran and N. M. Emmambux, Locally available african complementary foods: nutritional limitations and processing technologies to improve nutritional quality—a review, Food Rev. Int., 2022, 38, 1033–1063 CrossRef CAS.
- J. Makame, H. De Kock and N. M. Emmambux, Nutrient density of common African indigenous/local complementary porridge samples, LWT, 2020, 133, 1–11 CrossRef.
- O. A. Adebo, African sorghum-based fermented foods: past, current and future prospects, Nutrients, 2020, 12, 1111 CrossRef CAS.
- F. W.-B. Tapsoba, S. Samandoulougou, H. Compaore, A. Ouedraogo, T. J. N. Yenouba, E. W. W. Bieogo, M. H. Dicko and H. Sawadogo-Lingani, Characterization of technological properties of sorghum and millet malt produced under control condition in Burkina Faso, Int. J. Biol. Chem. Sci., 2021, 15, 2261–2271 CrossRef.
- A. E. O. Elkhalifa and R. Bernhardt, Combination Effect of Germination and Fermentation on Functional Properties of Sorghum Flour, Curr. J. Appl. Sci. Technol., 2018, 30, 1–12 CrossRef.
- M. Gabaza, M. Joossens, M. Cnockaert, M. Muchuweti, K. Raes and P. Vandamme, Lactococci dominate the bacterial communities of fermented maize, sorghum and millet slurries in Zimbabwe, Int. J. Food Microbiol., 2019, 289, 77–87 CrossRef PubMed.
- K. Kaur and N. Singh, Amylose-lipid complex formation during cooking of rice flour, Food Chem., 2000, 71, 511–517 CrossRef CAS.
- R. Bazaz, W. N. Baba and F. A. Masoodi, Development and quality evaluation of hypoallergic complementary foods from rice incorporated with sprouted green gram flour, Cogent Food Agric., 2016, 2, 1154714, DOI:10.1080/23311932.2016.1154714.
- M. Minekus, M. Alminger, P. Alvito, S. Ballance, T. Bohn, C. Bourlieu, F. Carrière, R. Boutrou, M. Corredig, D. Dupont, C. Dufour, L. Egger, M. Golding, S. Karakaya, B. Kirkhus, S. Le Feunteun, U. Lesmes, A. Macierzanka, A. Mackie, S. Marze, D. J. McClements, O. Ménard, I. Recio, C. N. Santos, R. P. Singh, G. E. Vegarud, M. S. J. Wickham, W. Weitschies and A. Brodkorb, A standardised static in vitro digestion method suitable for food – an international consensus, Food Funct., 2014, 5, 1113–1124 RSC.
- A. E. O. Elkhalifa, B. Schiffler and R. Bernhardt, Effect of fermentation on the functional properties of sorghum flour, Food Chem., 2005, 92, 1–5 CrossRef CAS.
- A. E. O. Elkhalifa, B. Schiffler and R. Bernhardt, Effect of fermentation on the functional properties of sorghum flour, Food Chem., 2005, 92, 1–5 CrossRef CAS.
- C. G. Awuchi, V. S. Igwe and C. K. Echeta, The functional properties of foods and flours, Int. J. Adv. Acad. Res., 2019, 5, 139–160 Search PubMed.
- S. Chandra and S. Samsher, Assessment of functional properties of different flours, Afr. J. Agric. Res., 2013, 8, 4849–4852 Search PubMed.
- C. Chaves-López, C. Rossi, F. Maggio, A. Paparella and A. Serio, Changes Occurring in Spontaneous Maize Fermentation: An Overview, Fermentation, 2020, 6, 36 CrossRef.
- C. Mouquet-Rivier, C. Icard-Vernière, J.-P. Guyot, E. Hassane Tou, I. Rochette and S. Trèche, Consumption pattern, biochemical composition and nutritional value of fermented pearl millet gruels in Burkina Faso, Int. J. Food Sci. Nutr., 2008, 59, 716–729 CrossRef CAS PubMed.
- C. L. Ramos and R. F. Schwan, Technological and nutritional aspects of indigenous Latin America fermented foods, Curr. Opin. Food Sci., 2017, 13, 97–102 CrossRef.
- P. Mensah, B. S. Drasan, T. J. Harrison and A. M. Tomkins, Fermented Cereal Gruels: Towards a Solution of the Weanling's Dilemma, Food Nutr. Bull., 1991, 13, 1–8 Search PubMed.
- A. Morris, A. Barnett and O. Burrows, Effect of processing on nutrient content of foods, Cajanus, 2004, 37, 160–164 Search PubMed.
- I. O. Steve and O. I. Babatunde, Chemical Compositions and Nutritional Properties of Popcorn-Based Complementary Foods Supplemented With Moringa oleifera Leaves Flour, J. Food Res., 2013, 2, 117 CrossRef CAS.
- C. A. Edwards and A. M. Parrett, Dietary fibre in infancy and childhood, Proc. Nutr. Soc., 2003, 62, 17–23 CrossRef CAS.
- M. A. Asma, E. B. El Fadil and A. H. El Tinay, Development of Weaning Food from Sorghum Supplemented with Legumes and Oil Seeds, Food Nutr. Bull., 2006, 27, 26–34 CrossRef PubMed.
- I. Hojsak, M. A. Benninga, B. Hauser, A. Kansu, V. B. Kelly, A. M. Stephen, A. Morais Lopez, J. Slavin and K. Tuohy, Benefits of dietary fibre for children in health and disease, Arch. Dis. Child., 2022, 107, 973–979 CrossRef PubMed.
- S. Mariam, Nutritive Value Of Three Potential Complementary Foods Based On Cereals And Legumes, Afr. J. Food, Agric., Nutr. Dev., 2005, 5, 01–14 Search PubMed.
- B. Kouakou, K. K. S. Alexis, D. Adjéhi, D. K. Marcelin and G. Dago, Biochemical Changes Occurring During Germination and Fermentation of Millet and Effect of Technological Processes on Starch Hydrolysis by the Crude Enzymatic Extract of Millet, J. Appl. Sci. Res., 2008, 4, 1502–1510 CAS.
- J. E. Simwaka, M. V. M. Chamba, Z. Huiming, K. G. Masamba and Y. Luo, Effect of fermentation on physicochemical and antinutritional factors
of complementary foods from millet, sorghum, pumpkin and amaranth seed flours, Int. Food Res. J., 2017, 24, 1869–1879 CAS.
- A. A. El-Rahman, A. Z. A. Mahmoud, A. A. Sayed and M. A. Abd El Latif, Physiochemical properties and phytochemical characteristics of bottle gourd (Lagenaria siceraria) seed oil, Egypt. J. Chem., 2022, 65(4), 269–277 Search PubMed.
- C. E. Chinma, O. Adewuyi and J. O. Abu, Effect of germination on the chemical, functional and pasting properties of flour from brown and yellow varieties of tigernut (Cyperus esculentus), Food Res. Int., 2009, 42, 1004–1009 CrossRef CAS.
- M. A. Abeshu, A. Lelisa and B. Geleta, Complementary Feeding: Review of Recommendations, Feeding Practices, and Adequacy of Homemade Complementary Food Preparations in Developing Countries – Lessons from Ethiopia, Front. Nutr., 2016, 3(41), 1–9 Search PubMed.
- K. G. Dewey and K. H. Brown, Update on Technical issues concerning Complementary Feeding of Young Children in Developing Countries and Implications for Intervention Programs, Food Nutr. Bull., 2003, 24, 5–28 CrossRef.
- A. F. Akinsola, I. Osasona, E. T. Akintayo, T. O. Siyanbola and S. O. Omosebi, Nutritional Evaluation of Calabash Gourd (Lagenaria Siceraria) Seeds and Oil, J. Culin. Sci. Technol., 2022, 1–22 Search PubMed.
- M. Rodríguez-España, C. Y. Figueroa-Hernández, J. de D. Figueroa-Cárdenas, P. Rayas-Duarte and Z. J. Hernández-Estrada, Effects of germination and lactic acid fermentation on nutritional and rheological properties of sorghum: A graphical review, Curr. Res. Food Sci., 2022, 5, 807–812 CrossRef PubMed.
- N. M. Lowe, The global challenge of hidden hunger: perspectives from the field, Proc. Nutr. Soc., 2021, 80, 283–289 CrossRef.
- P. Siddhuraju and K. Becker, Effect of Various Domestic Processing Methods on Antinutrients and in Vitro Protein and Starch Digestibility of Two Indigenous Varieties of Indian Tribal Pulse, Mucuna pruriens Var. utilis, J. Agric. Food Chem., 2001, 49, 3058–3067 CrossRef CAS.
- M. Moussa, X. Qin, L.-F. Chen, O. H. Campanella and B. R. Hamaker, High-quality instant sorghum porridge flours for the West African market using continuous processor cooking, Int. J. Food Sci. Technol., 2011, 46, 2344–2350 CrossRef CAS.
- C. M. S. Trèche, Viscosity of gruels for infants: a comparison of measurement procedures, Int. J. Food Sci. Nutr., 2001, 52, 389–400 CrossRef.
- T. Gopaldas, S. Deshpande and C. John, Studies on a wheat-based amylase-rich food, Food Nutr. Bull., 1988, 10, 55–59 Search PubMed.
- M. Dimopoulou, K. Alba, I. M. Sims and V. Kontogiorgos, Structure and rheology of pectic polysaccharides from baobab fruit and leaves, Carbohydr. Polym., 2021, 273, 118540 CrossRef CAS PubMed.
- P. Myllärinen, A. Buleon, R. Lahtinen and P. Forssell, The crystallinity of amylose and amylopectin films, Carbohydr. Polym., 2002, 48, 41–48 CrossRef.
-
S. Serna-Saldivar and L. W. Rooney, in Sorghum and Millets: Chemistry and Technology, ed. D. A. V. Dendy, American Association of Cereal Chemistry, St. Paul, MN, 1995, pp. 69–124 Search PubMed.
- H. Teng and L. Chen, α-Glucosidase and α-amylase inhibitors from seed oil: A review of liposoluble substance to treat diabetes, Crit. Rev. Food Sci. Nutr., 2017, 57, 3438–3448 CrossRef CAS.
- A. M. Rovalino-Córdova, V. Fogliano and E. Capuano, The effect of cell wall encapsulation on macronutrients digestion: A case study in kidney beans, Food Chem., 2019, 286, 557–566 CrossRef.
- S. R. Devi, T. Kumari and S. C. Deka, Extraction of dietary fiber and phytochemicals from bottle gourd seeds (Lagenaria siceraria), its physicochemical properties and application in food model, Food Chem. Adv., 2023, 2, 100252 CrossRef.
- Z. Karim, M. Holmes and C. Orfila, Inhibitory effect of chlorogenic acid on digestion of potato starch, Food Chem., 2017, 217, 498–504 CrossRef CAS.
- L. de Morais Cardoso, S. S. Pinheiro, H. S. D. Martino and H. M. Pinheiro-Sant'Ana, Sorghum (Sorghum bicolor L.): Nutrients, bioactive compounds, and potential impact on human health, Crit. Rev. Food Sci. Nutr., 2017, 57, 372–390 CrossRef CAS PubMed.
- L. I. Ezeogu, K. G. Duodu and J. R. N. Taylor, Effects of endosperm texture and cooking conditions on the in vitro starch digestibility of sorghum and maize flours, J. Cereal Sci., 2005, 42, 33–44 CrossRef CAS.
- A. Khoddami, V. Messina, K. Vadabalija Venkata, A. Farahnaky, C. L. Blanchard and T. H. Roberts, Sorghum in foods: Functionality and potential in innovative products, Crit. Rev. Food Sci. Nutr., 2023, 63, 1170–1186 CrossRef CAS PubMed.
- M. Rodríguez-España, C. Y. Figueroa-Hernández, J. de D. Figueroa-Cárdenas, P. Rayas-Duarte and Z. J. Hernández-Estrada, Effects of germination and lactic acid fermentation on nutritional and rheological properties of sorghum: A graphical review, Curr. Res. Food Sci., 2022, 5, 807–812 CrossRef PubMed.
- K. G. Duodu, J. R. N. Taylor, P. S. Belton and B. R. Hamaker, Factors affecting sorghum protein digestibility, J. Cereal Sci., 2003, 38, 117–131 CrossRef CAS.
|
This journal is © The Royal Society of Chemistry 2023 |
Click here to see how this site uses Cookies. View our privacy policy here.