Critical evaluation of sample preparation for SP-ICP-MS determination of selenium nanoparticles in microorganisms – focus on yeast†
Received
5th June 2023
, Accepted 19th September 2023
First published on 21st September 2023
Abstract
The interest in biogenic SeNPs, produced accidentally or intentionally by a variety of procedures involving microorganisms or plants and their extracts, creates a need for methods for their reliable quantification in complex biological matrices. Particular interest is focused on sample preparation with its potential risks of non-quantitative recoveries as well as possible dissolution or formation of SeNPs during the analytical protocol. This relatively new topic is attracting a lot of attention from academia as well as the food and feed industry, considering aspects of industrial processing and profitability, medical use, and potential toxicity of SeNPs. Ten approaches, including enzymatic, mechanical and chemical extractions, aiming at the extraction of SeNPs from selenized yeast S. cerevisiae were compared. The efficiency of the extraction procedures was evaluated by single particle (SP) – ICP-MS using a collision/reaction cell. An enzymatic digestion resulted in the highest recoveries of SeNPs but, at the same time, the broadest size distribution. Chemical extractions, although leading to recoveries higher than those by mechanical procedures, were not considered as efficient, as they caused partial dissolution of NPs.
Introduction
Selenium is a trace essential element for animals and humans, with a recommended dietary allowance (RDA) of 55 μg and a tolerable upper intake level of 400 μg for adults.1 Its importance in animal farming is also well documented.2 The essentiality range of selenium is very narrow with both its deficit and excess resulting in adverse health effects.3 Selenium is acquired from the diet and, because it is unevenly distributed in the Earth's crust, in some regions it is considered an environmental pollutant, while in others local populations suffer from its deficiency, which has to be compensated for by supplementation.4 One of the most popular food and feed supplements is Se-enriched yeast produced by growing different yeast strains, e.g., Saccharomyces cerevisiae or Candida utilis, in the presence of selenite.5 At the same time there is growing interest in selenium nanoparticles (SeNPs) as a novel selenium food supplement.6 A number of procedures for synthesis of SeNPs published in recent years use microbial and plant extracts and living cells of fungi, yeast, algae and bacteria, to reduce ionic selenium and then stabilize the created nanoparticles.7–9 They allow lowering production cost, avoiding environmental pollution, reducing physiological toxicity and enhancing biological compatibility of produced nanoparticles in comparison with physical or chemical procedures as they do not require chemical reducing and stabilising agents which may be toxic and can hinder the utilization of synthesized SeNPs in biological systems. The nanoparticles produced in this way are reported to be more stable and active in biological systems as natural extract components act as both reducing agents and stabilizers for nanoparticles.9 The yeast Saccharomyces cerevisiae often serves as a source of biogenic SeNPs allowing synthesis in a broad size range which can be modulated by the concentration of the Se source in the medium and the time of incubation.10,11
From the above discussion it is clear that there is an urgent need for reliable methods for the determination of selenium nanoparticles (SeNPs) in complex organic matrices; this comes from growing interest in SeNPs both as novel selenium supplements and also from a demand from regulatory bodies requiring detailed control of their presence in “organic selenium” food and feed supplements derived from plants and microorganisms. In this context selenium enriched yeast is a particularly suitable model sample for the development of analytical methodologies being, at the same time, (i) an organism able to effectively produce SeNPs and also (ii) used as a basis for purely organic (thus, theoretically, not containing SeNPs) food and feed supplements.
The key point to be addressed in analytical methodologies is the stability of SeNPs during sample preparation, their quantitative isolation and separation from cell debris. It requires a careful optimization of experimental conditions in order to preserve the integrity of SeNPs (avoiding their dissolution) and prevent the formation of artefact NPs from possibly presenting residual ionic selenium.
The isolation of NPs from the biological matrix requires the disruption of the cell wall and/or membrane to release the NPs contained in the cytoplasm and/or located outside the cell (e.g. attached to the cell membrane). In particular, yeast cell disruption strategies for the recovery of intracellular bio-active compounds12 and oils13 have been reviewed; the main approaches fall into two groups: mechanical and non-mechanical.12,13 The former include ultrasonication, shaking in a bead mill and high pressure homogenization. Non-mechanical methods involve the use of enzymes selected according to the cell membrane composition or chemical agents, alkaline reagents, and organic solvents.12,13 In general, mechanical techniques provide a high recovery but a poor selectivity while non-mechanical ones are more gentle and selective.12
Table 1 summarizes the methods applied for the isolation of SeNPs from biological sample matrices and their determination. In the studies published so far, the isolation of SeNPs has been carried out under neutral or slightly basic conditions, often using Tris HCl buffer at pH 7.5.14–17 The most often used alkali reagent is tetramethylammonium hydroxide (TMAH), while proteinase K is often used in enzymatic procedures.18 The combination of enzymatic and physical methods, including the use of driselase, protease, SDS and sonication, was successfully applied for the extraction of SeNPs from yeast.16
Table 1 Methods of extraction and determination of SeNPs in fungi, plants and bacteria reported in the literature
Matrix |
SeNP size, nm |
Sample preparation |
LoD, nma (isotope) |
Analytical technique |
ICP MS detection |
Ref. |
As reported by the authors.
|
Selenized yeast Saccharomyces cerevisiae (commercially available) |
40–200 |
Enzymatic extraction: incubation with 4% driselase (24 h) and then with 4 mg L−1 protease (24 h), and the pellet was resuspended in 4% SDS and sonicated (1 h) |
18 (80Se) |
SP-ICP-MS, SEC-HPLC/ICP-MS, and TEM |
5 mL min−1 H2; 0.1 and 5 ms dwell time |
16
|
Selenized yeast |
80–150 |
60 |
HPLC-ICP-MS, SP-ICP-MS, and XPS |
21
|
Selenized Candida utilis |
20–68 |
20 (80Se) |
SP-ICP-MS, SEC-HPLC/ICP-MS and TEM |
17
|
Selenized yeast Saccharomyces cerevisiae |
40–250 |
Mechanical extraction: sonication (10 min) and vortexing (5 min) with glass beads (500 μm); both steps were repeated; filtration (0.22 μm) |
20 |
SP-ICP -MS/MS, LC/ICP -MS/MS, iCAP™ ICP-MS/MS (HECIS), and TEM |
0.3 mL min−1 O2; mass shift 80Se → 80Se16O+; 5 and 0.1 ms dwell time |
20
|
Polymorphic fungus Aureobasidium pullulans |
20–100, median 49.7 |
Cell suspension filtered (0.45 μm), centrifuged at different speeds, and rinsed with graded EtOH series (50–100%) and with 20% SDS |
20 (78Se) |
SP-ICP-MS, AF4 coupled to MALS, UV-vis and ICP-MS, TEM, and DLS |
3.5 mL min−1 H2; 0.1 ms dwell time |
23
|
Radish plants Raphanus sativus |
26 |
Extraction with a mixture of 0.1% chitosan, 0.034 M ascorbic acid and 0.24 M acetic acid, sonication and centrifugation |
n.a. |
HPLC-ICP-MS, AF4-UV-ICP-MS, and TEM |
6 mL min−1 H2 |
14
|
Bacteria Lactobacillus bulgaricus |
100 |
Enzymatic hydrolysis of cell pellets with 0.5 mL 0.1 mg mL−1 lysozyme in TAE buffer (37 °C for 2.5 h) and then with 0.5 mL 0.1 mg mL−1 protease and sonication |
n.a. |
HPLC-ICP-MS and TEM |
6 mL min−1 H2 |
15
|
Bacteria Lactobacillus delbrueckii subsp. bulgaricus |
53–60 |
19
|
Lactic acid bacteria: Lactobacillus acidophilus, L. delbrueckii subsp. bulgaricus, and L. reuteri |
146–247 |
Sonication of bacterial cultures (2 min), centrifugation, re-suspension in 0.1% SDS with 1 M NaOH and sonication |
n.a. |
TEM-EDXS, DLS, NTA, and AF4-DAD-ICP-MS |
6 mL min−1 H2 |
22
|
Several techniques have been recently used for the characterization of SeNPs; their choice is driven by the properties to be determined. One of the most popular strategies is the on-line and off-line hyphenation of ICP-MS with separation techniques, such as high performance liquid chromatography (HPLC)14–17,19–21 or asymmetric flow field flow fractionation (AF4),14,22,23 allowing in some cases the separation of soluble chemical species and different-size NPs.24,25 Multiple detectors, such as dynamic light scattering (DLS),22,23 multiangle light scattering (MALS)23 or UV-VIS spectrophotometry14,23 are used to confirm the presence of NPs. However, DLS and MALS are not element specific. Another frequently used technique in NP analysis is transmission electron microscopy (TEM)26 which can be applied to solid samples (e.g., dried extracts). The use of energy-dispersive X-ray spectroscopy (EDS) together with TEM enables the elemental analysis of the selected region of a TEM image.22 The difficulty in providing information about SeNPs at low concentrations (μg kg−1) is the main drawback of the above-mentioned techniques.
More sensitive is single particle inductively coupled plasma mass spectrometry (SP-ICP-MS), which can provide information about the number and mass concentration of NPs as well as their size distribution.16,20,21,23 SP-ICP-MS is also capable of distinguishing and quantifying the nanoparticulate and the dissolved fraction of the element of interest.27 The NP size detection limit (LOD) is largely dependent on the solute concentration, so in order to improve the LOD, the soluble fraction is usually eliminated by repeated ultracentrifugation. The LOD for SeNPs reported by SP-ICP-MS is ca. 20 nm (Table 1), with the lowest value of 18 nm reported by Jimenez-Lamana et al. for Se-rich yeast after enzymatic digestion and multiple centrifugation steps.16
The aim of this work was to critically assess SeNP isolation procedures (enzymatic, mechanical and chemical) from selenized yeast for their accurate detection, quantification and characterization by SP-ICP-MS. The samples studied included a baker's yeast incubated in-house with selenite and a commercially available Se-enriched yeast sample.
Experimental
Reagents
Standard solutions were prepared daily by diluting the commercially available Au and Se standard solutions of 1000 mg L−1 (Sigma-Aldrich, Saint Quentin Fallavier, France) and a reference Au nanoparticle standard suspension with a nominal diameter of 50 nm (BBI Solutions, UK). A SeNP commercial suspension of 150 nm was used to verify the fate of SeNPs during extraction procedures (Glantreo, Ireland). SELM-1, CRM (NRC, Ottawa, Canada) with the certified values for total selenium, selenomethionine and methionine, was used in all procedures performed. Glucose (Sigma-Aldrich) and YPD broth (Sigma-Aldrich) were used for growth medium preparation. Ultra-pure water was obtained from a Milli-Q system (Millipore, France). Driselase from Basidiomycetes sp. (>99.9%), protease from Streptomyces griseus (>3.5 units mg−1) and Trizma base (>99.9%) purchased from Sigma-Aldrich were used for enzymatic extraction. Sodium dodecyl sulfate (SDS, >99.0%), sodium hydroxide (>98.0%) and tetramethylammonium hydroxide (TMAH, 25%) used for chemical extraction were obtained from Sigma-Aldrich. 65% HNO3 (Baker, Deventer, Netherlands) and 30% H2O2 (VWR International, Fontenay-sous-Bois, France) were used for the digestion of yeast and SeNP suspension. Acid-washed sand (200–300 μm diameter), glass beads and sand mixture (1
:
1, v:v) and metal stainless steel beads (2 mm diameter) from Sigma-Alrich were used for cell disruption.
Model yeast samples
Three batches of commercially available baker's yeast Saccharomyces cerevisiae were enriched with selenium according to procedures summarized in Table SI-1† that differed in terms of a growth medium, Se concentration added and time; the resulting preparations have been referred to as Y-A, Y-B and Y-C. The yeast growth and selenium enrichment conditions were aimed at the formation of SeNPs. Sample Y-D was a commercial Se-rich yeast where the presence of SeNPs was previously detected [unpublished].
Each of the in-house cultivated yeast samples was thoroughly washed with water prior to freeze drying to eliminate the remaining selenite ions which would impair the detection limits and could generate new SeNPs in further extraction steps.
Sample preparation procedures
The detailed procedures of sample digestion for total Se and the enzymatic procedures for SeMet, SeCys and protein-bound inorganic Se determination are described in the ESI.† The parameters of enzymatic, mechanical and chemical cell lysis procedures for the isolation of SeNPs prior to SP-ICP MS analysis are summarized in Table 2.
Table 2 Procedures for the isolation of SeNPs from yeast biomass
|
Enzymatic |
Mechanical |
Chemical |
E16 |
MR20 |
MG |
MGS |
MS |
MM2 |
MM4 |
CN |
CT |
CS |
Including sonication, vortexing and centrifugation.
|
Sample mass (mg) |
10 |
10 |
5 |
Extraction medium |
4% driselase, 4 mg L−1 protease, 4% SDS |
850 mg of glass beads |
500 mg glass beads |
500 mg sand/glass beads |
500 mg sand |
2 metal beads |
4 metal beads |
1 M NaOH |
4% TMAH |
4% SDS |
Centrifugation speed (RPM) |
10 000 |
2000 |
Final dilution |
50 000 |
100 000 |
Number of extraction stepsa |
9 |
5 |
2 |
10 |
Procedure duration [h] |
∼40 |
2 |
1 |
3 |
Enzymatic digestion.
The enzymatic extraction procedure (E) was performed as described previously by Jimenez-Lamana et al.16 Briefly, the process included 4 steps: (1) a 10 mg yeast sample was bath sonicated for 1 h and centrifuged, (2) the pellet was resuspended in 4% driselase in 30 mM Tris buffer (pH 7.5), incubated for 17 h at 25 °C and centrifuged, (3) the pellet was resuspended with 4 mg mL−1 protease in Tris buffer, incubated for 17 h at 37 °C and centrifuged, and (4) the pellet was resuspended with 4% SDS, bath sonicated for 1 h, centrifuged and diluted with water. All operations were carried out in 2 mL Eppendorf vials; the centrifugation was performed at 10
000 rpm for 10 min at 20 °C (50
000-fold final dilution).
Mechanical cell lysis.
The methods of cell lysis are based on the mechanical disruption of rigid cell walls by vigorous shaking with glass or metal beads or sand.
Six procedures, using different types of beads, were tested: glass (MR, mechanical reference, and MG) and metal (MM2 and MM4) beads and sand (MS) and a mixture of sand and glass beads (MGS). The details are given in Table 2. The sonication and vortexing steps were repeated twice. Briefly, yeast was suspended in ultrapure water (0.01
:
1, m/v) together with the beads or sand in 2 mL Eppendorf vials and shaken in a mixer mill equipped with a PTFE adapter for 5 vials (MM400, Retsch, Germany). The shaking parameters were the same for all bead types: 10 Hz oscillations for 5 min. Immediately after shaking, a 10 μL suspension aliquot was taken, diluted 200-fold with water, and centrifuged at 2000 rpm for 10 min at 20 °C to precipitate the cell debris. The supernatant was diluted with water before the analysis.
Chemical procedures.
The chemical extraction procedure was performed with the use of basic media and an anionic surfactant at various concentrations: SDS at 1%, 4% and 10% (CS); NaOH at 1; 10; 100 and 1000 mM (CN) and TMAH at 1%, 4%, 10% and 25% in ultrapure water (CT). In a 2 mL Eppendorf vial, 5 mg of yeast was mixed with 0.5 mL of extraction medium, briefly vortexed to homogenize the suspension, bath sonicated for 15 min and cooled in an ice water bath for 5 min; the process was repeated 4 times. The extract was briefly vortexed, 10 μL were taken and diluted 200-fold with ultrapure water and centrifuged at 2000 rpm for 10 min at 20 °C to precipitate the cell debris; the supernatant was diluted with the ultrapure water before the analysis (final dilution 100
000-fold).
Instrumentation
Equipment used for sample preparation and handling.
A Centrifuge 5804R (Eppendorf, Germany), Vortex2 (IKA, Germany), mixer mill equipped with a PTFE adapter for 5 vials (MM400, Retsch, Germany), and water bath OLS200 (Grant Instruments, UK) were used.
SP-ICP-MS analysis.
An Agilent 7900 ICP-MS (Agilent, Tokyo, Japan) was used in single particle mode for the SeNP characterization in the part of the study concerning the sample preparation evaluation. The sample was introduced using a Micromist concentric nebulizer and a cooled Scott spray chamber. Instrumental settings are summarized in Table SI-2 (ESI).† The 80Se isotope was monitored. Hydrogen was used as a collision gas to reduce polyatomic interference from the argon dimer. A dwell time of 100 μs and a zero settling time were set in the SP mode to register the multipoint time-resolved signal for a single NP occurrence. The transport efficiency was calculated according to Pace et al.,28 by using the particle size and the particle frequency methods, to calculate SeNP size and number concentrations, respectively. The sample flow was calculated daily, immediately before the analysis by SP-ICP-MS by measuring the mass of water aspirated using a peristaltic pump for 2 min. The process was repeated and the average value was taken for calculations. The transport efficiency in all measurements was in the range 2.4–4.4%.
Data analysis.
Single Nanoparticle Application Module for ICP-MS MassHunter software (Agilent) was used for raw data collection from ICP-MS and data handling in SP mode, including histogram generation. The open source software “SP/SC ICP-MS data processing platform (SPCal)” version 0.7.1 was used to create size distribution histograms from SP-ICP-MS data.29 MS Excel 2019 was used for basic statistical calculations and creation of graphs. In-house developed Visual Basic for Applications (VBA) procedures were used for task automation. Corel PaintShop Pro 2020 was used for editing the figures.
The equipment used for yeast characterization (total Se and speciation) is detailed in the ESI.†
Results and discussion
Initial characterization of model yeast samples
The initial characterization of model yeast samples involved the total selenium determination and selenium speciation analysis performed according to the procedure developed by Bierla et al.;30 the results of the determination of selenomethionine (SeMet), selenocysteine (SeCys) and protein-bound inorganic Se fraction as well as the total selenium in samples are shown in Table 3.
Table 3 Total selenium content and selenium speciation in model yeast samples. Values are reported as the average and SD of two replicates
Yeast sample |
Total Se c ± SD [μg g−1] |
Selenomethionine c ± SD [μg g−1] |
Selenocysteine c ± SD [μg g−1] |
Inorganic Se bound to the protein fraction c ± SD [μg g−1] |
Residual Se fraction [%] |
Y-A |
4500 ± 350 |
7.3 ± 0.2 |
<LOD |
1470 ± 8 |
67 |
Y-B |
22 700 ± 675 |
8.2 ± 0.3 |
<LOD |
7000 ± 260 |
69 |
Y-C |
22 000 ± 2300 |
43 ± 0.3 |
<LOD |
14 300 ± 80 |
35 |
Y-D |
2100 ± 20 |
8.8 ± 0.4 |
2.1 ± 0.1 |
650 ± 30 |
69 |
The results of speciation analysis showed, for all the selenized yeast samples studied, a significant Se fraction that could not be attributed to SeMet, SeCys or protein-bound inorganic form. The low amounts of organic forms together with the high percentage of an undetermined fraction that was not influenced by the enzymatic digestion of yeast suggest the presence of other Se forms, such as SeNPs. This hypothesis was supported by the red color of yeast biomass.
Effect of sample preparation on SeNP determination
The analysis of the biomass containing SeNPs requires an isolation step that has to be efficient and gentle, not affecting the NP properties. The extraction of SeNPs should not be performed at low pH, to avoid the oxidative dissolution of SeNPs. On the other hand, selenite ions are reduced to Se0 in the presence of certain organic molecules, such as ascorbic acid, sugars or reducing enzymes, and form NPs.8
Here, three types of extraction approaches, enzymatic, chemical and mechanical, detailed in Table 2, were investigated. The enzymatic procedure was applied as described elsewhere.16 The mechanical method was tested in 6 variants, with various types of beads and a single shaking step, including an already published method described by Álvarez-Fernández García et al.20 The three chemical approaches tested involved different extractants: NaOH, SDS and TMAH. Surfactants such as SDS or Triton X-100 affect the cell membrane by solubilizing membrane proteins and disrupting the lipid–lipid, lipid–protein and protein–protein interactions, which increases the permeability of the cell membrane and wall, leading to disintegration and lysis of the cell.31 SDS, an anionic detergent, destabilizes the cell membrane by dissolving the phospholipid and protein components. With its dual hydrophilic and hydrophobic characteristics, SDS binds to the hydrophobic sections of membrane lipids while interacting with water molecules through its hydrophilic segment. Consequently, this disturbs the lipid bilayer arrangement and ultimately causes membrane lysis. Strong base solutions such as NaOH or TMAH are highly corrosive and very reactive, causing hydrolysis and saponification of lipids and esters in the cell wall and membrane, leading to their breakdown and cell lysis.32
Extracts were appropriately diluted and further analyzed by SP-ICP-MS. The extraction conditions had a substantial impact on the amount of NPs released from biomass, the concentration of the ionic fraction, the shape of the histograms, the median NP size and the LOD values for each sample.
The analysis of insufficiently diluted sample extracts may lead to non-spectral effects from the extraction reagent (e.g. TMAH), as demonstrated elsewhere in the analysis of Ag nanoparticles in blood.18 In our study, the extracts were diluted in order to reduce these potential matrix interferences and hence reduce their impact on the selenium sensitivity. It was found that the dilution factor of yeast extracts between 104 and 105 applied throughout this study eliminated a matrix effect on the detection limits.
Comparison of enzymatic, chemical and mechanical approaches on a SeNP commercial suspension.
The initial experiments were performed on a 150 nm SeNP commercial suspension which was submitted to the enzymatic16 and mechanical20 procedures already reported in the literature. To separate the NPs from the dissolved Se species, the aliquots of SeNP suspension were diluted 10-fold with water and centrifuged at 10
000 rpm. After centrifugation, the supernatant was discarded and the pellet was resuspended in water. Additionally, an aliquot of the SeNP commercial suspension was analyzed directly after centrifugation and dilution as a reference. The analyses were repeated on different days; the median particle sizes obtained were in the range 118–125 nm, which was slightly lower than the nominal size (150 nm). Nevertheless, median diameters around 125–130 nm were obtained by transmission electron microscopy (data not shown), in reasonably good agreement with SP-ICP-MS data. According to the manufacturer's information, these SeNPs are not coated with organic agents or functional groups, and their nominal size refers to the Se core. The resulting histograms of the SeNP commercial suspension after the mechanical and the enzymatic procedures are shown in Fig. 1 and compared with the histogram obtained for the original suspensions. A broadening of the size histogram obtained for the sample subjected to the enzymatic procedure was observed. The median values were found to be similar both for the enzymatic (113 nm) and mechanical (120 nm) sample preparation procedures. The histogram of the SeNP commercial suspension analyzed without extraction showed a median size of 121 nm. The complexity of the enzymatic procedure, involving driselase, a mixture of laminarinase, xylanase and cellulase could promote the partial dissolution, growth or synthesis of NPs in different stages of the procedure, resulting in a significant broadening of the histograms, compared to the mechanical cell lysis. On the other hand, the mechanical procedure does not substantially alter the shape of the size distribution of the SeNPs present in the sample.
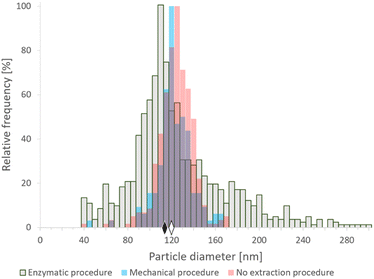 |
| Fig. 1 SP-ICP-MS histograms of size distribution of commercial SeNPs (nominal size 150 nm) after enzymatic16 (gray) and mechanical20 (blue) extraction procedures, and analyzed directly after centrifugation and dilution (pink). The diamonds represent the median size of NPs after enzymatic (black) and mechanical (white) sample preparation procedures. Bin size: 5 nm. | |
Effect on SeNP size distribution from model yeast samples.
The model yeast samples with the characteristics presented in Table 3 were subjected to 10 extraction procedures detailed in Table 2. The resulting extracts were diluted so as to obtain a total count of Se-NP events lower than 3000, in order to minimize the occurrence of simultaneous detection of more than one particle as well as to obtain a low background signal as a consequence of the dilution of the soluble fraction. The time scans obtained for the SeNP commercial suspension after enzymatic and mechanical procedures (Fig. S1A and B,† respectively) and for the Y-B sample after enzymatic and mechanical procedures (Fig. S1C and D,† respectively) showed a significant number of pulses due to the presence of SeNPs and a low background signal. The duration of a single SeNP event measured by SP-ICP-MS was affected by the intrinsic dispersion of the cloud of ions in the spectrometer, which is increased by the use of a reaction gas to median values in the range 0.4–0.8 ms. No SeNP events above the size detection limit were detected during the analysis of a CRM SELM-1 extract confirming the earlier study20 reporting only SeNPs below 10 nm in this material.
Although samples Y-A, Y-B and Y-C were prepared on the basis of the same baker's yeast, the different conditions of cultivation and enrichment with Se resulted in the generation of SeNPs in various amounts and size distributions. The size distributions obtained by SP-ICP-MS for the four examined yeast samples are presented in Fig. 2, showing different median sizes for samples Y-A, Y-B and Y-C. Among the in-house cultivated yeast samples, the lowest median size was observed for sample Y-A, which was subjected to the YPD broth and 24 h incubation, while the highest median size was obtained for sample Y-C, cultivated in the YPD broth and incubated for 72 h. Hence, a longer exposure of yeast to the selenite solution resulted in larger SeNP size. Sample Y-D showed the lowest median NP size; however, the manufacturing process of this commercial yeast is not known.
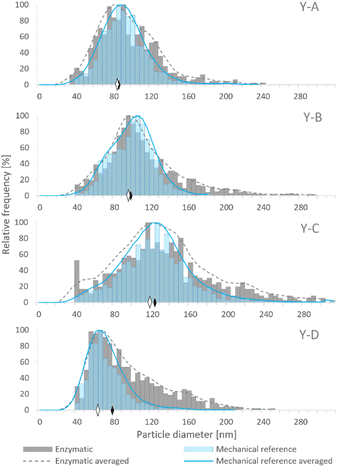 |
| Fig. 2 Histograms representing the SeNP size distribution in Se-enriched yeast samples (Y-A, Y-B, Y-C and Y-D) after enzymatic (grey columns) and mechanical (blue columns) cell lysis procedures. The solid and dashed lines represent the averaged values of the particle size distribution after the enzymatic and mechanical cell lysis procedures, respectively. The diamonds represent the median size of SeNPs after enzymatic (black) and mechanical (white) cell lysis procedures. Bin size: 5 nm. | |
These four different samples were used as models for the evaluation of the cell lysis procedures aimed at the extraction of SeNPs from the complex yeast matrix.
Fig. 2 shows the comparison of SeNP size distribution in yeast after enzymatic (E)16 and mechanical (MR)20 procedures. Mechanical cell lysis resulted in narrower and more symmetrical size distributions than the enzymatic one. The presence of larger NPs was observed after the enzymatic procedure which manifested as a long tail on the right side of the histogram. This may be caused by the agglomeration events due to the modification of the SeNP surface by the enzymes; a similar phenomenon was observed by Borowska et al.33
The SeNP size distributions obtained after the tested extraction procedures are shown in the figure. The values on the y-axis were not normalized to 100% and are presented in relation to the mass of yeast to show the extraction efficiency and the quantitative differences between the tested approaches. No significant differences were observed in the shape of the SeNP size distribution after the six mechanical cell lysis procedures. For the sake of clarity, the size distribution of only three out of six mechanical procedures is shown in Fig. 3. The size distribution of any of the samples after the enzymatic procedure was broader than that after any other extraction approach, similarly to what was observed for the SeNP commercial suspension (Fig. 1). The efficiency of enzymatic extraction was the highest among the tested approaches with the exception of sample Y-B, for which it was the lowest. This observation and the fact that the sample Y-B was cultivated in a nutrition-depleted medium give some clues to the different mechanisms of formation of SeNPs. The red color of this sample, the high efficiency of the chemical extraction using basic reagents and the poor enzymatic extraction efficiency can be due to the extracellular SeNP formation or a rapid excretion of SeNPs from the yeast cell. The mechanical procedure with metal beads showed the smallest particle number efficiency due to the small number of effective interactions of 2–4 large beads (MM2 and MM4, respectively) with the yeast cells during extraction. A slightly higher number of SeNPs were extracted with the 250–500 μm glass beads due to a substantially larger number of collisions of beads and yeast cells. For all four yeast samples the mechanical reference approach yielded a relatively large number of extracted SeNPs and a smooth, Gaussian-like, size distribution shape.
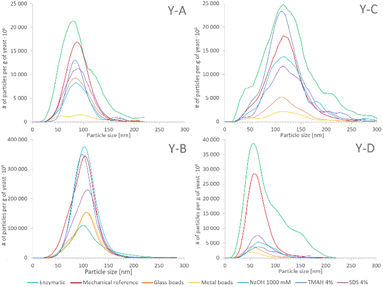 |
| Fig. 3 Averaged size distributions of the yeast samples Y-A, Y-B, Y-C and Y-D obtained after different isolation procedures: E−green, MR – red, MG – orange, MM2 – yellow, CN – blue, CT – marine blue and CS – purple. The values on the y-axis are absolute in reference to 1 g of dry yeast. | |
Effect on SeNP mass and median size from model yeast samples.
The mass of SeNPs per mass unit of yeast and the median particle size are shown in Fig. 4, while the concentration of soluble selenium in yeast and the particle size LOD are presented in Fig. SI-2.† No significant difference (±5 nm) in terms of the SeNP median size obtained by the different applied extraction procedures was observed except for sample Y-D. For the latter, the enzymatic and chemical procedures resulted in ca. 10 nm higher medians than those by the mechanical ones. In general, the lowest mass of particles per g of yeast was extracted with mechanical procedures, with the exception of the one published by Álvarez-Fernández García et al.20 The median NP sizes for all tested procedures and samples were in the ranges 84–88 nm (Y-A), 98–104 nm (Y-B), 118–127 nm (Y-C) and 60–78 nm (Y-D). The smallest SeNP median sizes were found for the commercial yeast Y-D. Among the in-house cultivated yeasts, the smallest SeNPs were found in Y-A and the largest in Y-C, which can be correlated with the time of enrichment. This observation is similar to that by Tam et al. who studied the growth mechanism of SeNPs in marine bacteria Shewanella and reported no significant impact on the average particle size for the initial concentration of selenite tested in the range 0.01–1 M.34 Also, similar to our results, SeNPs were larger after 72 h incubation than after 24 h, with an average particle size of 150 nm and 120 nm, respectively. In the work of Alvarez-Fernandez Garcia et al. the median SeNP size for the in-house cultivated S. cerevisiae was 103 nm.20 The incubation time of yeast with 25 mg L−1 Se(IV) or Se(VI) was 7 days, and the mechanical method with glass beads was used. The median size of SeNPs in selenized yeast-like fungus Aureobasidium pullulans measured by SP-ICP-MS was 49.7 nm; however, when other techniques were used, the results were substantially different: 80 nm (AF4-MALS), 167 nm (DLS) and 35.5 nm (TEM).23 This is likely because MALS and DLS are not element-specific and measure the hydrodynamic diameter, i.e. the NP core with the surface coating, while SP ICP-MS is element-specific and measures only the particle core. The enzymatic approach for the same yeast species resulted in a median size of SeNPs of 108 ± 4 nm.16 The same extraction procedure, although with a much higher LOD due to the high ionic background, resulted in a median of 100 ± 3 nm.21 Among the developed mechanical approaches the sand and metal beads were the least efficient. The chemical procedures were clearly more efficient in terms of the mass of particles measured; however, the soluble fraction was high, especially when strong bases, NaOH and TMAH, were used, leading probably to partial dissolution of SeNPs. The concentration of the ionic fraction is roughly proportional to the LOD because the background from the dissolved selenium in the time scans is the main factor influencing the LOD. Klimek-Ochab et al. tested several techniques of cell wall disintegration of fungi including ultrasound treatment, homogenization in bead mills, application of detergents and osmotic shock and reported that the mechanical disruption of fungi and yeast with glass beads was the best method.35
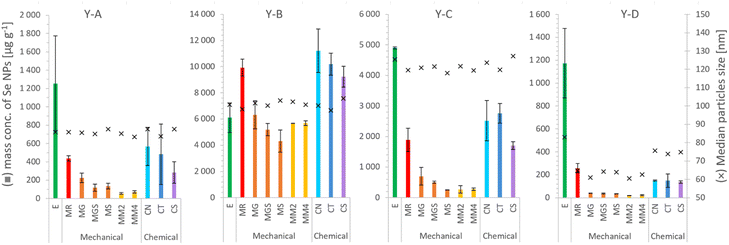 |
| Fig. 4 SeNP mass per g of selenized yeast, and the median size, after the extraction procedure: E – enzymatic,16 MR – mechanical,20 MG – glass beads, MGS – glass beads and sand, MS – sand, MM2 – 2 metal beads, MM4 – 4 metal beads, CN – 1000 mM NaOH, CT – 4% TMAH, and CS – 4% SDS. Each column and error bar represent average and SD of two replicates, respectively. | |
The mass concentrations of SeNPs determined in yeast extracts after enzymatic and mechanical procedures are shown in Table 4 together with the percentage of all determined Se species, including SeMet, SeCys and inorganic selenium bound to proteins (cf.Table 3). A satisfactory mass balance between the total selenium content and the sum of the species could be obtained for samples prepared by enzymatic procedures with the exception of a relatively low value for the sample Y-C.
Table 4 Mass concentration of extracted SeNPs per g of yeast and the yields of the enzymatic and mechanical procedures reported in the literature
|
Y-A |
Y-B |
Y-C |
Y-D |
Enzymatic procedure16 |
SeNP mass conc. (μg g−1) |
1250 ± 520 |
6100 ± 1130 |
4900 ± 34 |
1800 ± 300 |
SeNP/total Se (%) |
27.7 ± 11.7 |
26.9 ± 5.0 |
22.3 ± 2.3 |
55.8 ± 14.4 |
Sum of % of all Se species |
95.1 ± 14.9 |
96.2 ± 6.3 |
56.9 ± 11.3 |
124.6 ± 14.5 |
![[thin space (1/6-em)]](https://www.rsc.org/images/entities/char_2009.gif) |
Mechanical procedure20 |
SeNP mass conc. (μg g−1) |
437 ± 28 |
9900 ± 650 |
1900 ± 380 |
257 ± 40 |
SeNP/total Se (%) |
9.6 ± 1.0 |
43.7 ± 3.1 |
8.6 ± 1.9 |
12.2 ± 1.9 |
Sum of % of all Se species |
77.0 ± 9.3 |
113.0 ± 4.9 |
43.2 ± 11.3 |
81.0 ± 2.5 |
Effect of the SeNP content in yeast.
In order to verify the potential influence of the variable content of SeNPs in yeast on the SeNP detection, an experiment was performed consisting of mixing the Y-B sample with fresh baker's yeast (Y-0) in various proportions and subjecting it to the enzymatic extraction procedure. The number and mass concentration of particles in extracts of Y-B “diluted” with Y-0 are presented in Fig. 5. The relationship between the measured number of particles and mass concentration of NPs is proportional to the mass fraction of Se-rich yeast Y-B to fresh yeast Y-0 and to the content of NPs in the analyzed sample. This study demonstrated that the extraction efficiency remains consistent in yeast samples varying in SeNP content by approximately 10-fold, confirming the robustness of the developed enzymatic procedure.
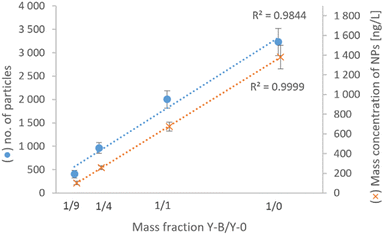 |
| Fig. 5 Number of particles and mass concentration of SeNPs in extracts of yeast Y-B mixed with fresh baker's yeast Y-0 in various mass ratios. Points and error bars represent the average and SD of two replicates. | |
Conclusions
The sample preparation procedure affects the amount of extracted SeNPs, the shape of NP size distribution, and the dissolution of SeNPs. The SeNP median size did not differ substantially between the isolation procedures for the Se-enriched yeast tested. The enzymatic procedure provided the highest recoveries in terms mass of SeNPs for 3 out of 4 studied samples, while broader size distributions were obtained, probably due to the partial agglomeration of SeNPs due to the modification of their surface by the enzymes. On the other hand, the mechanical procedure, involving two cycles of sonication and glass bead milling was faster and provided more realistic size distributions but it was less efficient in terms of mass recovery. Both methods proved to be complementary. Finally, chemical procedures did not provide satisfactory results due to the partial dissolution of SeNPs.
Author contributions
AS, KB, JS and JJL designed the study. AS, KB and JJL performed the experiments. AS and JJL analysed the data. AS, KB, JS and JJL wrote and revised the manuscript.
Conflicts of interest
There are no conflicts to declare.
Acknowledgements
The funding of the Agilent ICP-MS instrument by AQUITRACES (project no. 20131206001-1301097) by the Aquitaine Region is acknowledged.
Notes and references
-
Institute of Medicine, Dietary Reference Intakes for Vitamin C, Vitamin E, Selenium, and Carotenoids, The National Academies Press, Washington, DC, 2000 Search PubMed.
- X. Gu and C.-Q. Gao, Anim. Nutr., 2022, 11, 80–86 CrossRef CAS PubMed.
- M. P. Rayman, Lancet, 2012, 379, 1256–1268 CrossRef CAS PubMed.
- G. D. Jones, B. Droz, P. Greve, P. Gottschalk, D. Poffet, S. P. McGrath, S. I. Seneviratne, P. Smith and L. H. Winkel, Proc. Natl. Acad. Sci. U. S. A., 2017, 114, 2848–2853 CrossRef CAS PubMed.
- J. Yang and H. Yang, Crit. Rev. Food Sci. Nutr., 2023, 63, 411–425 CrossRef CAS PubMed.
- S. Skalickova, V. Milosavljevic, K. Cihalova, P. Horky, L. Richtera and V. Adam, Nutrition, 2017, 33, 83–90 CrossRef CAS PubMed.
- H. Duan, D. Wang and Y. Li, Chem. Soc. Rev., 2015, 44, 5778–5792 RSC.
- M. C. Zambonino, E. M. Quizhpe, F. E. Jaramillo, A. Rahman, N. Santiago Vispo, C. Jeffryes and S. A. Dahoumane, Int. J. Mol. Sci., 2021, 22, 989 CrossRef CAS PubMed.
- N. Bisht, P. Phalswal and P. K. Khanna, Mater. Adv.c, 2022, 3, 1415–1431 RSC.
- S. Faramarzi, Y. Anzabi and H. Jafarizadeh-Malmiri, Arch. Microbiol., 2020, 202, 1203–1209 CrossRef CAS PubMed.
- A. G. Pereira, L. G. L. Gerolis, L. S. Gonçalves, T. A. Pedrosa and M. J. Neves, Biomed. Phys. Eng. Express., 2018, 4, 035028 CrossRef.
- D. Liu, L. Ding, J. Sun, N. Boussetta and E. Vorobiev, Innovative Food Sci. Emerging Technol., 2016, 36, 181–192 CrossRef CAS.
- M. Koubaa, N. Imatoukene, L. Drévillon and E. Vorobiev, Chem. Eng. Process., 2020, 150, 107868 CrossRef CAS.
- M. Palomo-Siguero, M. I. López-Heras, C. Cámara and Y. Madrid, J. Anal. At. Spectrom., 2015, 30, 1237–1244 RSC.
- M. Palomo-Siguero, A. M. Gutiérrez, C. Pérez-Conde and Y. Madrid, Microchem. J., 2016, 126, 488–495 CrossRef CAS.
- J. Jiménez-Lamana, I. Abad-Álvaro, K. Bierla, F. Laborda, J. Szpunar and R. Lobinski, J. Anal. At. Spectrom., 2018, 33, 452–460 RSC.
- M. Kieliszek, K. Bierla, J. Jiménez-Lamana, A. M. Kot, J. Alcántara-Durán, K. Piwowarek, S. Błażejak and J. Szpunar, Int. J. Mol. Sci., 2020, 21, 5287 CrossRef CAS PubMed.
- I. Abad-Alvaro, D. Leite, D. Bartczak, S. Cuello-Nunez, B. Gomez-Gomez, Y. Madrid, M. Aramendia, M. Resano and H. Goenaga-Infante, J. Anal. At. Spectrom., 2021, 36, 1180–1192 RSC.
- M. Palomo-Siguero and Y. Madrid, Int. J. Mol. Sci., 2017, 18, 1712 CrossRef PubMed.
- R. Álvarez-Fernández García, M. Corte-Rodríguez, M. Macke, K. L. LeBlanc, Z. Mester, M. Montes-Bayón and J. Bettmer, Analyst, 2020, 145, 1457–1465 RSC.
- V. Vacchina, D. Foix, M. Menta, H. Martinez and F. Séby, Anal. Bioanal. Chem., 2021, 413, 1809–1816 CrossRef CAS PubMed.
- G. Moreno-Martin, M. Pescuma, T. Pérez-Corona, F. Mozzi and Y. Madrid, Anal. Chim. Acta, 2017, 992, 34–41 CrossRef CAS PubMed.
- K. C. Nwoko, X. Liang, M. A. M. J. Perez, E. Krupp, G. M. Gadd and J. Feldmann, J. Chromatogr. A, 2021, 1642, 462022 CrossRef CAS PubMed.
- J. Malejko, N. Świerżewska, A. Bajguz and B. Godlewska-Żyłkiewicz, Spectrochim. Acta, Part B, 2018, 142, 1–7 CrossRef CAS.
- S. López-Sanz, N. R. Fariñas, R. C. R. del Martín-Doimeadios and Á. Ríos, Anal. Chim. Acta, 2019, 1053, 178–185 CrossRef PubMed.
- E. Verleysen, T. Wagner, H. G. Lipinski, R. Kägi, R. Koeber, A. Boix-Sanfeliu, P. J. De Temmerman and J. Mast, Materials, 2019, 12, 2274 CrossRef PubMed.
- F. Laborda, J. Jiménez-Lamana, E. Bolea and J. R. Castillo, J. Anal. At. Spectrom., 2011, 26, 1362–1371 RSC.
- H. E. Pace, N. J. Rogers, C. Jarolimek, V. A. Coleman, C. P. Higgins and J. F. Ranville, Anal. Chem., 2011, 83, 9361–9369 CrossRef CAS PubMed.
- T. E. Lockwood, R. Gonzalez de Vega and D. Clases, J. Anal. At. Spectrom., 2021, 36, 2536–2544 RSC.
- K. Bierla, R. Lobinski and J. Szpunar, Int. J. Mol. Sci., 2018, 19, 543 CrossRef PubMed.
- M. S. Islam, A. Aryasomayajula and P. R. Selvaganapathy, Micromachines, 2017, 8, 1–27 CAS.
- T. A. Gomes, C. M. Zanette and M. R. Spier, Prep. Biochem. Biotechnol., 2020, 50, 635–654 CrossRef CAS PubMed.
- M. Borowska, J. Jiménez-Lamana, K. Bierla, K. Jankowski and J. Szpunar, Food Chem., 2023, 417, 135864 CrossRef CAS PubMed.
- K. Tam, C. T. Ho, J.-H. Lee, M. Lai, C. H. Chang, Y. Rheem, W. Chen, H.-G. Hur and N. V. Myung, Biosci., Biotechnol., Biochem., 2010, 74, 696–700 CrossRef CAS PubMed.
- M. Klimek-Ochab, M. Brzezińska-Rodak, E. Żymańczyk-Duda, B. Lejczak and P. Kafarski, Folia Microbiol., 2011, 56, 469–475 CrossRef CAS PubMed.
|
This journal is © The Royal Society of Chemistry 2023 |
Click here to see how this site uses Cookies. View our privacy policy here.