DOI:
10.1039/D2MA00975G
(Paper)
Mater. Adv., 2023,
4, 881-889
Transparent and flexible high-surface area nanoporous crystalline PPO films
Received
13th October 2022
, Accepted 15th November 2022
First published on 16th November 2022
Abstract
Guest and processing conditions leading to high surface area (SA) nanoporous-crystalline (NC) poly(2,6-dimethyl-1,4-phenylene)oxide (PPO) films are explored. In this framework, we found crystallization conditions leading to unprecedented high-SA films (with SA up to 450 m2 g−1) with transparency and toughness comparable with those for amorphous PPO films. Suitable preparation procedures require (i) guest-induced co-crystallization by large uptake of a highly boiling guest molecule; (ii) guest sorption from the vapor phase at a temperature close to the guest boiling point; and (iii) subsequent guest removal by sorption/desorption of a volatile guest. This high-SA, transparent, and tough NC PPO films are expected to be suitable for organic pollution remediation, molecular separations, and molecular sensing.
Introduction
Two thermoplastic and commercially available polymers, poly(2,6-dimethyl-1,4-phenylene)oxide (PPO)1–7 and syndiotactic polystyrene (sPS),7–18 can be easily co-crystallized with low molecular-mass guest molecules. Moreover, PPO19–26 and sPS27–34 presently are the only two polymers, whose co-crystalline forms can lead to nanoporous-crystalline (NC) forms after suitable guest removal procedures.
The main feature of NC forms is their lower density than the corresponding amorphous phases, which leads to the high uptake of organic guest molecules, even when they are present only in traces. Uptake of guest molecules is particularly fast for PPO35–37 and sPS37–44 NC aerogels, due to their high surface area (SA; in the range of 500–750 m2 g−1 and 200–350 m2 g−1 for PPO and sPS, respectively). However, these very low-density aerogels present high per unit mass pollutant uptake, but relatively poor uptake per unit volume.
In recent papers,45,46 we have shown that pollutant uptake per unit volume is much higher for low SA NC PPO films compared to for aerogels, provided that the crystalline phase chain axes, and hence, their intrahelical empty channels23 are preferentially perpendicular to the film plane (c⊥ orientation).47,48 Moreover, for PPO and sPS NC polymer films with SA higher than 600 m2 g−1 and 120 m2 g−1, respectively, organic pollutant uptake is faster than for corresponding aerogels, even if evaluated per unit mass as was recently described.49,50 Known preparation procedures for high-SA NC films require fast crystallization of the amorphous films, which is induced by high uptake (typically in the range 70–90 wt%) of non-volatile liquid guests (carvone or dibenzyl ether), followed by a guest exchange with a volatile guest. However, due to the presence of mesopores beside the nanopores of their crystalline phases, the described high-SA NC films are opaque and exhibit poor toughness, and hence, are unsuitable for many applications.
In this paper, we explore other possible guests and processing conditions leading to the formation of high-SA NC PPO films. In this framework, we found crystallization conditions that lead to high SA (with SA up to 450 m2 g−1) with transparency and toughness comparable with those of NC PPO films, exhibiting negligible SA.
Experimental section
Materials and sample preparation
Poly(2,6-dimethyl-1,4-phenylene) oxide with Mw = 350 kg mol−1 (Ultra High P6130 grade) was supplied by Sabic, the Netherlands. Toluene, methyl benzoate, 1,3,5-trimethylbenzene (mesitylene), carvone, limonene, eugenol, carvacrol, dibenzyl ether, chloroform (CHCl3), acetonitrile (ACN), perchloroethylene (PCE), methanol, and CaCl2 were purchased from Sigma-Aldrich, Italy.
Amorphous film preparation
Amorphous PPO films with 20–200 μm thickness were obtained by casting 0.1–1 wt% CHCl3 solution at T = 60 °C. CHCl3 was extracted from the cast amorphous PPO films by ACN guest sorption/desorption at room temperature.
Guest-induced crystallization
Liquid guest-induced crystallization.
Liquid guest-induced crystallization of amorphous PPO films (20–100 μm) was conducted by sorption of liquids at temperatures in the range of 4–80 °C (Table 1).47
Table 1 The BET surface area (m2 g−1) and degree of crystallinity (%) of NC PPO films obtained by liquid phase guest-induced crystallization of amorphous PPO films (≈20 microns) at different temperatures. Guest molecules are ordered based on their diffusivities in amorphous PPO films (D, cm2 sec−1) at room temperature
Guest molecule |
Guest diffusivity (D) at rt (cm2 s−1) |
Liquid phase guest-induced crystallization temperature (°C) |
4 °C |
30 °C |
65 °C |
BET surface area (m2 g−1) |
Crystallinity (%) |
BET surface area (m2 g−1) |
Crystallinity (%) |
BET surface area (m2 g−1) |
Crystallinity (%) |
Toluene |
High |
185 |
43 |
Film dissolution |
Mesitylene |
1.9 × 10−9 |
175 |
45 |
165 |
44 |
Film dissolution |
Dibenzyl ether |
9.8 × 10−10 |
Negligible |
38 |
560 |
46 |
Film dissolution |
Carvone |
4.4 × 10−10 |
Negligible |
40 |
620 |
44 |
Film dissolution |
Limonene |
5.5 × 10−11 |
Negligible |
19 |
Negligible |
33 |
490 |
54 |
Eugenol |
2.6 × 10−11 |
Negligible |
18 |
Negligible |
33 |
440 |
56 |
Carvacrol |
6.4 × 10−12 |
Negligible |
10 |
Negligible |
24 |
330 |
48 |
Vapor guest-induced crystallization.
Vapor guest-induced crystallization was studied for the guest molecules listed in Table 2. Typically, the vapor phase guest-induced crystallization was conducted by laying amorphous PPO films (20–100 μm) on the surface of the liquid guest for 5–15 minutes at a temperature close to the guest boiling point.
Table 2 The BET surface area (m2 g−1) and degree of crystallinity (%) of NC PPO films obtained by vapor phase guest-induced crystallization of amorphous PPO films (≈20 microns) at temperatures close to the guest boiling point. Guest molecules are ordered based on their boiling temperatures (2nd column)
Guest molecule |
Boiling point (°C) |
BET surface area (m2 g−1) |
Degree of crystallinity (%) |
Toluene |
110 |
Film dissolution |
Mesitylene |
164 |
325 |
44 |
Limonene |
176 |
170 |
35 |
Carvone |
231 |
450 |
56 |
Carvacrol |
237 |
305 |
47 |
Eugenol |
254 |
205 |
51 |
Dibenzyl ether |
298 |
T
b (guest) > Tm (polymer host) |
Guest removal from the CC films was conducted by supercritical carbon dioxide (scCO2) extraction. General extraction conditions comprised temperature = 40 °C, pressure = 250 bar, and time = 4 hours. The thickness of amorphous films after full guest removal increased by a factor of 2.1–2.2 and 2.9–3.3 for liquid-induced crystallization at 4 °C and room temperature, respectively. An intermediate increase of thickness (factor in the range 2.4–2.7) was observed for vapor-induced crystallization (with carvone at 220 °C).
Characterization techniques
Wide-angle X-ray diffraction (WAXD) patterns were collected with a Bruker D8 diffractometer (at an operating step size of 0.03° and a rate of 0.2 s/step with nickel-filtered Cu Kα radiation). Two-dimensional wide-angle X-ray diffraction (2D-WAXD) patterns obtained by sending the X-ray beam parallel or perpendicular to the film surface are called EDGE or THROUGH patterns in the discussion onwards. The degree of planar orientation (fc) of the PPO films was evaluated by using Hermans’ orientation function: | 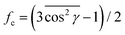 | (1) |
where cos2
γ is determined by the azimuthal distribution of 001 reflection intensity for the 2D-WAXD EDGE patterns. When fc(001) is equal to −0.5 or 1, the c axes of all crystallites are perfectly perpendicular or parallel to the film plane, respectively. When fc(001) is equal to 0, a random crystallite orientation occurs.
The correlation length of the crystalline domains was evaluated for the intense and rather isolated 210 equatorial reflections, which were located at a diffraction angle of 2θ = 11.2° by using the Scherrer's formula:
| D210 = (kλ)/(β cos θ210) | (2) |
where
λ is the wavelength of the X-ray (0.154 nm);
k is the shape factor assumed to be 0.9;
β is the full width at half-maximum (FWHM) of the 210 reflections as taken from radial profiles of 2D-WAXD THROUGH patterns.
Nitrogen adsorption measurements were performed at 77 K on a Nova Quantachrome 4200e instrument. The specific SA of the NC PPO films was calculated using the Brunauer–Emmett–Teller (BET) method in the 0.05 < p/p0 < 0.2 pressure range. The surface morphology of the PPO films was analyzed by scanning electron microscopy (Carl Zeiss SMT AG, Oberkochen, Germany). Before imaging, all PPO film samples were coated with gold (Agar Auto Sputter Coater model 108 A, Stansted, UK) at 30 mA for 5 min. Thermogravimetric analysis (TGA, TA Q500 equipment) was used to quantify the guest content in the co-crystalline PPO films.
Differential scanning calorimetry (DSC, TA Q2000 equipment) was used to measure the melting enthalpies of the NC PPO films. The heating and cooling cycles were conducted at a rate of 10 °C min−1. Degrees of crystallinity (Xc) of NC PPO films were estimated based on the following equation:
|  | (3) |
Where Δ
Hf and Δ
H°
f are melting enthalpies of semicrystalline and fully crystalline PPO samples, respectively. The melting enthalpy of the fully crystalline PPO samples is assumed as 42 ± 2 J g
−1.
50
Because the high-SA films exhibit macroporosity, their densities cannot be evaluated by the standard floatation method (generally conducted in aqueous solutions of methanol or CaCl2). For this reason, the density of our films (ρ) was simply obtained as the mass/volume ratio of rectangular homogeneous samples. We verified that the two film density evaluation methods lead to close values, both for amorphous (1.04 g cm−3) and for NC c⊥ oriented (0.98 g cm−3) films.
The density of the polymer (ρp) that is present in the high-SA films was calculated based on the degree of crystallinity (Xc, obtained from DSC) and of the density of amorphous PPO (ρam = 1.04 g cm−3) and the NC α-form (ρα = 0.93 g cm−3), by using the formula:20
| ρp = ραXc + ρam(1 − Xc) | (4) |
The porosity (
P) of NC films was evaluated as follows:
| 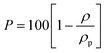 | (5) |
Vapor sorption measurements (0.05 ≥
p/
p0 ≥ 0.01) were performed at 35 °C with a VTI-SA symmetrical vapor sorption analyzer (TA instruments). The diffusion coefficient (
D) of PCE in PPO films was evaluated based on the following equation:
| 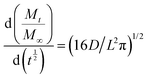 | (6) |
where
L is the film thickness, while
Mt and
M∞ are the masses of PCE absorbed at the time
t at equilibrium, respectively.
Results and discussion
Liquid guest-induced crystallization
The known procedure of guest-induced crystallization leading to high-SA NC PPO films requires fast crystallization of amorphous films induced by high uptake of non-volatile guests, followed by a guest exchange with a volatile guest.48 The literature data refer to the sorption of liquid carvone and dibenzyl ether at room temperature.48 This procedure is now extended to lower (4 °C) and higher (65 °C) temperatures and to other non-volatile guests of CC phases of PPO (e.g., toluene, mesitylene, limonene, eugenol, and carvacrol). The BET SA and the degree of crystallinity (as evaluated by DSC measurements) of the obtained films are summarized in Table 1, where the guests are ordered based on their room temperature diffusivities in amorphous PPO films (2nd column).
From Table 1, it is apparent that the highly diffusive toluene guest leads to high-SA NC films only at low sorption temperatures, while it dissolves amorphous PPO films at 30 °C and 65 °C. Mesitylene leads to high-SA NC films for sorption at 4 °C and 30 °C, while it dissolves amorphous PPO films at 65 °C. Dibenzyl ether and carvone have a similar behavior: both lead to high-SA NC films for sorption at 30 °C, while they dissolve amorphous PPO films at 65 °C. Moreover, both guests when absorbed at 4 °C led to low SA NC films due to very slow guest sorption (and guest-induced crystallization) kinetics.48,49 The three guests presented in Table 1 with the lowest diffusivities (limonene, eugenol, and carvacrol) lead to high-SA NC films only at the highest sorption temperature (65 °C). Guest-sorption procedures conducted at lower temperatures lead to low-SA NC films, with c⊥ orientations.44–47
In summary, the results presented in Table 1 indicate that non-volatile guests of CC phases of PPO can give high-SA NC PPO films, provided that suitable guest sorption temperatures are chosen. It is necessary to avoid very high sorption kinetics, which may lead to the dissolution of amorphous PPO films (upper right part of Table 1), as well as very low sorption kinetics, which may lead to low-SA NC PPO films (lower left part of Table 1).
Vapor guest-induced crystallization
In this section, guest-induced crystallization of PPO amorphous films is explored for the guests in Table 1 by using high-temperature vapors rather than low-temperature liquids. Our experiments on vapor-induced crystallization were conducted by exposing amorphous PPO films to vapors at temperatures close to the guest boiling point (2nd column in Table 2).
This new route to get high-SA NC PPO films was effective with most of the guests assessed here. It was not only effective for toluene, which is a very strong solvent for PPO (first line of Table 2), and for dibenzyl ether, whose boiling point (as well as treatment temperature) was higher than the melting temperature of PPO51–54 (last row in Table 2). For the other entries in Table 2, the guest content after the vapor sorption procedure was in the range of 65–80 wt% and became null after the acetonitrile sorption/desorption procedure.
The most relevant feature of the high-SA NC PPO films presented in Table 2 (that were obtained by vapor guest-induced crystallization) was that they were all transparent and tough, whereas all the high-SA NC PPO films in Table 1 obtained by liquid guest-induced crystallization, were opaque and brittle.
A comparison of structure, morphology, transparency, toughness, and guest uptake of high-SA NC PPO films obtained by liquid or vapor guest-induced crystallization, is presented in the next three sections.
Structure and morphology of high-surface area NC PPO Films
In this section, as well as in the next two sections, a comparison between three NC PPO films with similar thickness is presented (≈50 μm): (i) a transparent high-SA obtained by vapor guest-induced crystallization (red lines); (ii) an opaque high-SA obtained by liquid guest-induced crystallization (blue lines); and (iii) a highly diffusive low SA with c⊥ orientation (black lines). The main quantitative results are summarized in Table 3.
Table 3 Characterization of NC PPO α-form films obtained by carvone-induced crystallization of amorphous films: (2nd row) transparent high-SA NC PPO film by vapor induced crystallization; (3rd row) opaque high-SA NC PPO by liquid induced crystallization; (4th row) c⊥ NC PPO by low-temperature liquid induced crystallization; (5th row) amorphous PPO. Correlation length (D210, nm), degree of crystallinity (Xc, %, by DSC), measured film density (ρ, g cm−3), percent of porosity (P), crystallite size based on SEM images (nm), BET surface area (m2 g−1), light transmittance at 600 nm (%), PCE diffusivity at 35 °C and p/p0 = 0.01 (D, cm2 s−1)
PPO film |
D
210 (nm) |
Degree of crystallinity (%) |
Density (ρ, g cm−3) |
% of porosity based on the density (%) |
Crystallite sizes based on SEM images (nm) |
BET surface area (m2 g−1) |
Transmittance at 600 nm (%) |
PCE diffusivity at 35 °C, p/p0 = 0.01 (cm2 s−1) |
Transparent high-SA NC film |
5.6 |
56 |
0.91 |
7 |
40–60 |
450 |
74 |
2.8 × 10−9 |
Opaque high-SA NC film |
5.4 |
45 |
0.82 |
16.5 |
40–60 |
620 |
0 |
3.3 × 10−9 |
c
⊥ NC film |
5.5 |
40 |
0.98 |
0 |
35–45 |
<4 |
86 |
6.6 × 10−10 |
Amorphous film |
— |
0 |
1.04 |
0 |
— |
<4 |
90 |
8.2× 10−12 |
The WAXD patterns (in particular, radial profiles of 2D THROUGH patterns) of the three films are shown in Fig. 1. All these patterns show intense equatorial 100, 010, 210, and 310 reflections that are typical of the NC α-form.20,22 The broadness of these diffraction peaks, being similar for the three films considered here, indicates the occurrence of similar correlation lengths of the polymer crystallites. For instance, the correlation length as calculated for the intense and rather isolated 210 reflections (D210) is in the narrow range of 5.4–5.6 nm.
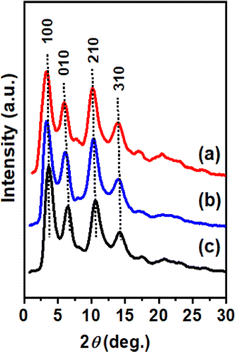 |
| Fig. 1 Radial profiles of 2D-WAXD THROUGH patterns of NC PPO films obtained by guest extraction from CC PPO films: (a) high-SA transparent (from vapor phase carvone-induced crystallization); (b) high-SA opaque (from liquid phase carvone-induced crystallization at 30 °C); and (c) low SA transparent c⊥ oriented (from liquid phase carvone-induced crystallization at 4 °C). | |
The degree of crystallinity of these films cannot be easily compared by WAXD patterns due to the occurrence of c⊥ orientation in the film (Fig. 1c). The degree of crystallinity of these films can instead be safely evaluated by melting enthalpies obtained from the DSC scans.50 The DSC scans of the three films at a heating rate of 10 °C min−1 have been compared in Fig. 2, where the melting enthalpies and melting temperatures are also indicated.
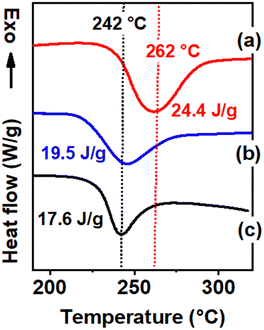 |
| Fig. 2 The DSC scans of NC PPO films obtained by carvone-induced crystallization: (a) vapor guest sorption; (b) liquid guest sorption at 30 °C, and (c) liquid guest sorption at 4 °C. Melting enthalpies and temperatures are indicated close to the curves. | |
The transparent film from vapor carvone-induced crystallization exhibits melting enthalpy (and the related degree of crystallinity, Xc ≈ 56%) and melting temperature (Tm ≈ 262 °C) much higher than for the other two films (40% < Xc < 45% and 242 °C < Tm < 245 °C). This indicates that the crystallization of amorphous PPO films induced by high-temperature vapor guest sorption not only leads to higher degrees of crystallinity but also to higher crystalline phase perfection.
The measured film densities (ρ) are compared in the 4th column of Table 3. Film porosity calculated based on these density values by using eqn (4) is reported in the 5th column of Table 3. The porosity of the vapor guest crystallized film was much lower than that of the liquid guest crystallized film (at 4 °C).
The SEM images of the three films at different magnifications are compared in Fig. 3(a–c) and (a′–c′). Macroporosity was observed in the film crystallized by sorption of liquid carvone at room temperature (Fig. 3b and b′), while no macroporosity were observed in the film crystallized by sorption of carvone vapor (Fig. 3a and a′).
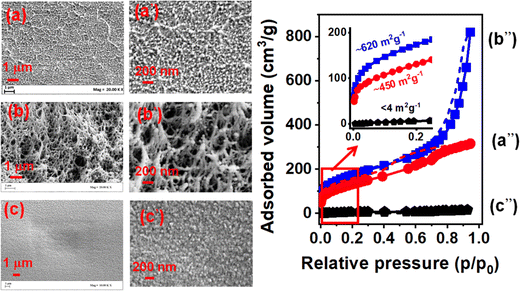 |
| Fig. 3 (a–c and a′–c′) SEM images with different magnifications and (a′′–c′′) volumetric N2 adsorption/desorption isotherms measured at 77 K for the NC PPO films, whose crystallization was induced by carvone sorption: (a and a′) from vapor phase; (b and b′) from the liquid phase at room temperature; (c and c′) from the liquid phase at 4 °C. Thick and dotted isotherms represent adsorption and desorption curves, respectively. The inset shows the enlarged low-pressure adsorption curves with the BET SA. | |
N2 sorption isotherms measured at 77 K for the three NC films are shown in Fig. 3(a′′–c′′). The sorption behavior observed for p/p0 < 0.2, which is shown as an inset in Fig. 3, is particularly interesting. The BET SA of the transparent (and highly diffusive)48c⊥ oriented film that was obtained from liquid carvone-induced crystallization at 4 °C was negligible (Fig. 3c′′), while the BET SA of the opaque film that was obtained from liquid carvone-induced crystallization at room temperature was very high (Fig. 3b′′, 620 m2 g−1). Surprisingly, the BET SA of the transparent film, from vapor-induced crystallization at the carvone boiling point, was quite high (Fig. 3a′′, 450 m2 g−1).
Transparency and toughness of high-surface area NC PPO films
All the high-SA films of Table 1, as those described in ref. 48 and 49, were completely opaque. Their density was always in the range of 0.80–0.85 g cm−3, i.e., also lower than for the NC α-form (0.93 g cm−3), clearly indicating the presence of a large number of voids (20–10 vol%) in the semi-crystalline films. The occurrence of large amounts of macropores, which were apparent in the SEM images shown in Fig. 3b and b′, was confirmed by optical and mechanical characterizations of the films.
Digital photos of films laying on the monomer unit chemical formula of PPO are shown in Fig. 4. As expected, the highest transparency occurs for the fully amorphous film (Fig. 4d), while slightly reduced transparency was observed for the NC film with c⊥ orientation (Fig. 4c),55 as well as for the NC film, crystallized by carvone sorption from the vapor phase (Fig. 4a). NC films crystallized at room temperature by liquid carvone sorption were completely opaque (Fig. 4b). This information was quantified by UV-Visible spectra for films with a thickness of nearly 50 μm and 100 μm (Fig. 4e).
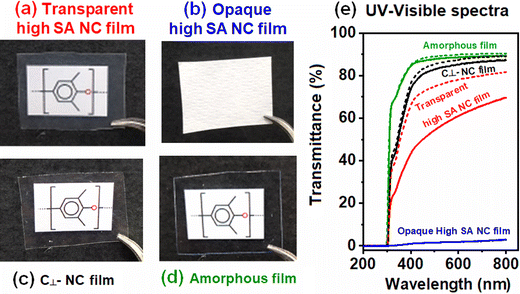 |
| Fig. 4 (a–d) Digital photos of PPO films with a thickness of ∼50 μm. To show possible transparency, the molecular formula of PPO is laying below the film. (a–c) NC obtained by carvone-induced crystallization: (a) from the vapor phase (red label and curves); (b) from the liquid phase at room temperature (blue label and curves); (c) from the liquid phase at 4 °C (black label and curves). (d) amorphous (green label and curves). (e) UV-Visible spectra of (a–d) PPO films. Dashed and continuous lines indicate transmittance for films with thicknesses of ≈50 μm and ≈100 μm, respectively. | |
The toughness of films, such as those listed in Table 3 (and shown in Fig. 4), was explored by bending tests, which evaluated the opening of a gauge at film breaking, as shown by digital photos in Fig. 5a and b.
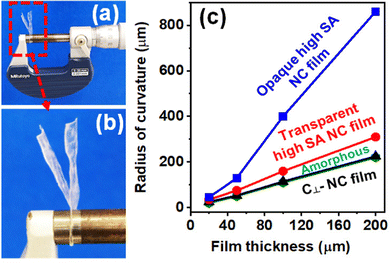 |
| Fig. 5 (a and b) Digital Photos of the used bending test for PPO films that evaluated the minimum opening of a gauge before film breaking. (c) The radius of curvature (one-half of this minimum opening) versus thickness of PPO films: (blue squares) opaque high-SA NC, (red circles) transparent high-SA NC, (black triangles) c⊥ NC, and (green pentagons) amorphous. | |
Fig. 5c shows the radius of curvature leading to film breaking (one-half of the minimum gauge opening) versus the film thickness. It is apparent that the transparent high-SA NC PPO films (from the vapor-induced crystallization) exhibit a strongly reduced brittleness as compared to the opaque high-SA NC PPO films (from liquid-induced crystallization), which was comparable to that of amorphous PPO films and the c⊥ NC PPO films.
Guest sorption kinetics from high-surface area NC PPO films
Guest diffusivities in PPO films of the four kinds listed in Table 3 were studied by guest sorption kinetics at low vapor pressures. As an example, the sorption kinetics of a relevant chlorinated pollutant (perchloroethylene, PCE)56–59 at 35 °C at a low relative pressure (p/p0 = 0.01) are shown in Fig. 6a.
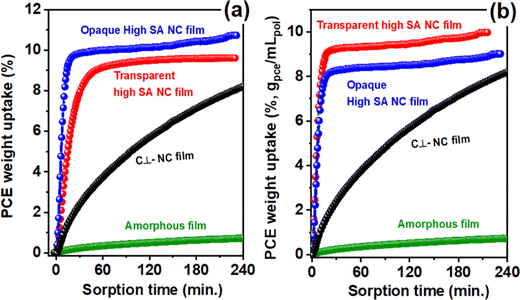 |
| Fig. 6 PCE sorption kinetics for p/p0 = 0.01 at 35 °C measured for different PPO films: (red circles) transparent high-SA NC; (blue circles) opaque high-SA NC; (black circles) c⊥ NC; (green circles) amorphous. PCE uptake is expressed as the mass of guest per mass of the polymer (a) or as the mass of guest per volume of polymer (b). | |
Amorphous PPO films, although well known for their high free-volume and molecular uptake,60–64 exhibit guest uptake much smaller and slower than for the NC film with c⊥ orientation44 and enormously smaller and slower than for the known opaque high-SA NC films.48
The most relevant result of Fig. 6a is that the new high-SA NC film obtained by vapor-induced crystallization, which has transparency and toughness comparable with those of amorphous PPO films, has guest uptake kinetics comparable with those of the already known opaque and brittle high-SA films. More interestingly, the new high-SA NC PPO films also have higher guest uptakes at equilibrium, if measured as weight uptake per unit volume (Fig. 6b), i.e., the amount that matters for most practical applications.
Conclusions
NC PPO films with transparency and toughness close to those of amorphous PPO films, as well as a surface area of up to 450 m2 g−1 and organic guest diffusivity comparable to those of opaque high-SA NC PPO films and aerogels, are presented. These high-SA polymer films have transparency and toughness close to those of NC PPO films with c⊥ orientation (i.e. the chain axes of the crystalline phase are preferentially perpendicular to the film plane) but with much higher SA and faster guest sorption kinetics. These new high-SA dense and transparent polymer films are unprecedented and can be even achieved with a high thickness (of at least up to 100 μm).
Preparation procedures leading to transparent and tough high-SA films require (i) guest-induced co-crystallization by large uptake (typically 60–80 wt%) of a highly boiling guest molecule (e.g., mesitylene, limonene, carvone, carvacrol, eugenol) in amorphous PPO films; (ii) guest sorption from vapor phase at a temperature close to the guest boiling point; and (iii) subsequent guest removal by sorption/desorption of a volatile guest, leading to the formation of NC phases.
These high-SA, transparent, and tough NC PPO films are expected to be suitable for many applications, mainly for organic pollution remediation, molecular separation, and molecular sensing. Many applications are also expected for the corresponding films exhibiting CC phases obtained by the sorption of functional guest molecules. Transparency, of course, is particularly relevant for CC films for guests with optical properties (chromophore, fluorescent, photoreactive, and chiral-optical).
Author contributions
The manuscript was written with the contributions of all authors. All authors have approved the final version of the manuscript.
Conflicts of interest
The authors declare no competing interests.
Acknowledgements
Financial support of Ministero dell’Università e della Ricerca (MUR, 0179BJNA2; Prin2020, 2020EZ8EPB) is gratefully acknowledged.
References
- J. M. Barrales-Rienda and J. M. G. Fatou, Single crystals of poly(2,6-dimethyl-1,4-phenylene) oxide, Colloid Polym. Sci., 1971, 244, 317–323 CrossRef CAS
.
- S. Horikiri, Single crystals of poly(2,6-dimethylphenylene oxide), J. Polym. Sci., Polym. Phys. Ed., 1972, 10, 1167–1170 CAS
.
- J. Hurek and E. Turska, X-ray studies of crystallization of poly(2,6-dimethyl-1,4-phenylene oxide) on swelling in certain systems, Acta Polym., 1984, 35, 201–207 CrossRef CAS
.
- O. Tarallo, V. Petraccone, C. Daniel, G. Fasano, P. Rizzo and G. Guerra, A chiral co-crystalline form of poly(2,6-dimethyl-1,4-phenylene)oxide (PPO), J. Mater. Chem., 2012, 22, 11672–11680 RSC
.
- P. Rizzo, G. Ianniello, L. Longo and G. Guerra, Uniplanar orientations and guest exchange in PPO co-crystalline films, Macromolecules, 2013, 46, 3995–4001 CrossRef CAS
.
- M. Golla, B. Nagendra, C. Daniel, P. Rizzo and G. Guerra, Isolated and aggregated carvacrol guest molecules in cocrystalline poly(2,6-dimethyl-1,4-phenylene)oxide films, Polym. J., 2021, 53, 1093–1100 CrossRef CAS
.
- G. Guerra, C. Daniel, P. Rizzo and O. Tarallo, Advanced materials based on polymer cocrystalline forms, J. Polym. Sci., Part B: Polym. Phys., 2012, 50, 305–322 CrossRef CAS
.
- Y. Chatani, T. Inagaki, Y. Shimane and H. Shikuma, Structural study on syndiotactic polystyrene: 4. Formation and crystal structure of molecular compound with iodine, Polymer, 1993, 34, 4841–4845 CrossRef CAS
.
- Y. Chatani, Y. Shimane, T. Inagaki, T. Ijitsu, T. Yukinari and H. Shikuma, Structural study on syndiotactic polystyrene: 2. Crystal structure of molecular compound with toluene, Polymer, 1993, 34, 1620–1624 CrossRef CAS
.
- E. Bhoje Gowd, S. S. Nair and C. Ramesh, Crystalline Transitions of the Clathrate (δ) Form of Syndiotactic Polystyrene during Heating: Studies Using High-Temperature X-ray Diffraction, Macromolecules, 2002, 35, 8509–8514 CrossRef
.
- V. Venditto, G. Milano, A. De Girolamo Del Mauro, G. Guerra and J. Mochizuki, Itagaki, Orientation and microenvironment of naphthalene guest in the host nanoporous phase of syndiotactic polystyrene, Macromolecules, 2005, 38, 3696–3702 CrossRef CAS
.
- Y. Uda, F. Kaneko, N. Tanigaki and T. Kawaguchi, The first example of a polymer-crystal-organic-dye composite material: The clathrate phase of syndiotactic polystyrene with azulene, Adv. Mater., 2005, 17, 1846–1850 CrossRef CAS
.
- C. Daniel, N. Galdi, T. Montefusco and G. Guerra, Syndiotactic polystyrene clathrates with polar guest molecules, Chem. Mater., 2007, 19, 3302–3308 CrossRef CAS
.
- A. R. Albunia, C. D’Aniello, G. Guerra, D. Gatteschi, M. Mannini and L. Sorace, Ordering magnetic molecules within nanoporous crystalline polymers, Chem. Mater., 2009, 21, 4750–4752 CrossRef CAS
.
- O. Tarallo, V. Petraccone, A. R. Albunia, C. Daniel and G. Guerra, Monoclinic and triclinic δ -clathrates of syndiotactic polystyrene, Macromolecules, 2012, 43, 8549–8558 CrossRef
.
- O. Tarallo, M. M. Schiavone, V. Petraccone, C. Daniel, P. Rizzo and G. Guerra, Channel clathrate of syndiotactic polystyrene with p-nitroaniline, Macromolecules, 2010, 43, 1455–1466 CrossRef CAS
.
- T. Sano, A. Uchiyama, T. Sago and H. Itagaki, Fluorescence behavior of syndiotactic polystyrene and its derivative: Formation of a ground-state dimer in the solid state, Eur. Polym. J., 2017, 90, 114–121 CrossRef CAS
.
- A. Cozzolino, G. Monaco, C. Daniel, P. Rizzo and G. Guerra, Monomeric and dimeric carboxylic acid in crystalline cavities and channels of delta and epsilon forms of syndiotactic polystyrene, Polymers, 2021, 13, 3330 CrossRef CAS PubMed
.
- C. Daniel, S. Longo, G. Fasano, J. G. Vitillo and G. Guerra, Nanoporous crystalline phases of poly(2,6-dimethyl-1,4-phenylene)oxide, Chem. Mater., 2011, 23, 3195–3200 CrossRef CAS
.
- M. Galizia, C. Daniel, G. Fasano, G. Guerra and G. Mensitieri, Gas sorption and diffusion in amorphous and semicrystalline nanoporous poly(2,6-dimethyl-1,4-phenylene)oxide, Macromolecules, 2012, 45, 3604–3615 CrossRef CAS
.
- C. Daniel, D. Zhovner and G. Guerra, Thermal stability of nanoporous crystalline and amorphous phases of poly(2,6-dimethyl-1,4-phenylene)oxide, Macromolecules, 2013, 46, 449–454 CrossRef CAS
.
- B. Nagendra, A. Cozzolino, C. Daniel, P. Rizzo, G. Guerra, F. Auriemma, C. De Rosa, M. C. D’Alterio, O. Tarallo and A. Nuzzo, Two nanoporous crystalline forms of poly(2,6-dimethyl-1,4-phenylene)oxide and related co-crystalline forms, Macromolecules, 2019, 52, 9646–9656 CrossRef CAS
.
- M. Golla, B. Nagendra, C. Daniel, P. Rizzo, F. Auriemma, O. Tarallo and G. Guerra, Intrahelical empty channels of nanoporous-crystalline α and β forms of PPO, Eur. Polym. J., 2022, 179, 111568 CrossRef CAS
.
- F. Auriemma, C. Daniel, M. Golla, B. Nagendra, P. Rizzo, O. Tarallo and G. Guerra, Polymorphism of poly(2,6-dimethyl-1,4-phenylene) oxide (PPO): co-crystalline and nanoporous-crystalline phases, Polymer, 2022, 258, 125290 CrossRef CAS
.
- A. Yu Alentiev, I. S. Levin, M. I. Buzin, N. A. Belov, R. Yu Nikiforov, S. V. Chirkov, I. V. Blagodatskikh, A. S. Kechekyan, P. A. Kechekyan, V. G. Bekeshev, V. E. Ryzhikh, P. Yu and P. Yampolskii, Gas transport parameters, density and free volume of nanocrystalline poly-2,6-dimethylphenylene oxide, Polymer, 2021, 226, 123804 CrossRef
.
- A. Y. Alentiev, I. S. Levin, N. A. Belov, R. Y. Nikiforov, S. V. Chirkov, D. A. Bezgin, V. E. Ryzhikh, J. V. Kostina, V. P. Shantarovich and L.-Y. Grunin, Features of the gas-permeable crystalline phase of poly-2,6-dimethylphenylene oxide, Polymers, 2022, 14, 120 CrossRef CAS PubMed
.
- C. De Rosa, G. Guerra, V. Petraccone and B. Pirozzi, Crystal structure of the emptied clathrate form (δe form) of syndiotactic polystyrene, Macromolecules, 1997, 30, 4147–4152 CrossRef CAS
.
- Y. Uda, F. Kaneko and T. Kawaguchi, Selective guest uptake from solvent mixtures in the clathrate phase of syndiotactic polystyrene, Macromol. Rapid Commun., 2004, 25, 1900–1904 CrossRef CAS
.
- M. M. Sivakumar, T. Suzuki, Y. Yamamoto, K. P. O. Mahesh, H. Yoshimizu and Y. Tsujita, Structure and properties of the mesophase of syndiotactic polystyrene, J. Membr. Sci., 2005, 262, 11–19 CrossRef
.
- S. Malik, D. Roizard and J.-M. Guenet, Multiporous material from fibrillar syndiotactic polystyrene intercalates, Macromolecules, 2006, 39, 5957–5959 CrossRef CAS
.
- V. Petraccone, O. Ruiz de Ballesteros, O. Tarallo, P. Rizzo and G. Guerra, Nanoporous polymer crystals with cavities and channels, Chem. Mater., 2008, 20, 3663–3668 CrossRef CAS
.
- B. Bhoje Gowd, K. Tashiro and C. Ramesh, Structural phase transitions of syndiotactic polystyrene, Prog. Polym. Sci., 2009, 34, 280–315 CrossRef
.
- C. Daniel, P. Antico and G. Guerra, Etched fibers of syndiotactic polystyrene with nanoporous-crystalline phases, Macromolecules, 2018, 51, 6138–6148 CrossRef CAS
.
- Y. Tamai, Effective and efficient transport mechanism of CO2 in subnano-porous crystalline membrane of syndiotactic polystyrene, J. Membr. Sci., 2022, 646, 120202 CrossRef CAS
.
- C. Daniel, L. Longo, S. Cardea, J. G. Vitillo and G. Guerra, Monolithic nanoporous−crystalline aerogels based on PPO, RSC Adv., 2012, 2, 12011–12018 RSC
.
- C. Daniel, M. Pellegrino, V. Venditto, S. Aurucci and G. Guerra, Nanoporous-crystalline poly(2,6-dimethyl-1,4-phenylene)oxide (PPO) aerogels, Polymer, 2016, 105, 96–103 CrossRef CAS
.
- C. Daniel, S. Longo, R. Ricciardi, E. Reverchon and G. Guerra, Monolithic nanoporous crystalline aerogels, Macromol. Rapid Commun., 2013, 34, 1194–1207 CrossRef CAS PubMed
.
- C. Daniel, D. Alfano, V. Venditto, S. Cardea, E. Reverchon, D. Larobina, G. Mensitieri and G. Guerra, Aerogels with a microporous crystalline host phase, Adv. Mater., 2005, 17, 1515–1518 CrossRef CAS
.
- C. D'Aniello, C. Daniel and G. Guerra, ε Form gels and aerogels of syndiotactic polystyrene, Macromolecules, 2015, 48, 1187–1193 CrossRef
.
- X. Wang and S. C. Jana, Synergistic Hybrid Organic-Inorganic Aerogels, ACS Appl. Mater. Interfaces, 2013, 5, 6423–6429 CrossRef CAS PubMed
.
- X. Wang and S. C. Jana, Syndiotactic polystyrene aerogels containing multi-walled carbon nanotubes, Polymer, 2013, 54, 750–759 CrossRef CAS
.
- P. Raut, W. Liang, Y.-M. Chen, Y. Zhu and S. C. Jana, Syndiotactic Polystyrene-Based Ionogel Membranes for High-Temperature Electrochemical Applications, ACS Appl. Mater. Interfaces, 2017, 9, 30933–30942 CrossRef CAS PubMed
.
- V. G. Krishnan, A. M. Joseph, S. K. Peethambharan and E. Bhoje Gowd, Nanoporous Crystalline Aerogels of Syndiotactic Polystyrene: Polymorphism, Dielectric, Thermal, and Acoustic Properties, Macromolecules, 2021, 54, 10605–10615 CrossRef CAS
.
- A. Kulkarni and S. C. Jana, Surfactant-free syndiotactic polystyrene aerogel foams via Pickering emulsion, Polymer, 2021, 212, 123125 CrossRef CAS
.
- C. Daniel, P. Rizzo, B. Nagendra, A. Cozzolino and G. Guerra, High diffusivity dense films of a nanoporous-crystalline polymer, Polymer, 2021, 229, 124005 CrossRef CAS
.
- A. Cozzolino, B. Nagendra, P. Rizzo, C. Daniel and G. Guerra, Fast uptake of organic pollutants from dilute aqueous solutions by nanoporous-crystalline PPO films with c-perpendicular orientation, Eur. Polym. J., 2021, 161, 110864 CrossRef CAS
.
- P. Rizzo, C. Gallo, V. Vitale, O. Tarallo and G. Guerra, Nanoporous-crystalline films of PPO with parallel and perpendicular polymer chain orientations, Polymer, 2019, 167, 193–201 CrossRef CAS
.
- B. Nagendra, M. Golla, C. Gallo, C. Daniel, P. Rizzo, G. Guerra, L. Baldino and E. Reverchon, Mechanisms determining different planar orientations in PPO films crystallized by guest sorption, Polymer, 2021, 235, 24242 CrossRef
.
- B. Nagendra, A. Cozzolino, C. Daniel, P. Rizzo and G. Guerra, High surface area nanoporous-crystalline polymer films, Macromolecules, 2022, 55, 2983–2990 CrossRef CAS
.
- B. Nagendra, C. Daniel, P. Rizzo, G. Guerra, L. Baldino and E. Reverchon, High surface area polymer films by co-crystallization with low-molecular-mass guest molecules, Eur. Polym. J., 2022, 173, 111305 CrossRef CAS
.
- B. Nagendra, E. Vignola, C. Daniel, P. Rizzo and G. Guerra, Dependence on Film Thickness of Guest-Induced c Perpendicular Orientation in PPO Films, Polymers, 2021, 13, 4384 CrossRef CAS PubMed
.
- F. E. Karasz and J. M. O’Reilly, Thermal properties of poly(2,6-dimethylphenylene ether), J. Polym. Sci. B Polym. Lett., 1965, 3, 561–563 CrossRef CAS
.
- S. Z. D. Cheng and B. Wunderlich, Glass transition and melting behavior of poly(oxy-2,6-dimethyl-1,4-phenylene), Macromolecules, 1987, 20, 1630–1637 CrossRef CAS
.
- B. Nagendra, M. Golla, C. Daniel, P. Rizzo and G. Guerra, Melting of nanoporous-crystalline and co-crystalline solution cast films of poly(2,6-dimethyl-1,4-phenylene) oxide, Polymer, 2021, 228, 123935 CrossRef CAS
.
- B. Nagendra, P. Rizzo, C. Daniel and G. Guerra, Planar Orientation and Transparency of Nanoporous-Crystalline Polymer Films, Macromolecules, 2021, 54, 6605–6611 CrossRef CAS
.
- D. L. Freedman and J. M. Gossett, Biological reductive dechlorination of tetrachloroethylene and trichloroethylene to ethylene under methanogenic conditions, Appl. Environ. Microbiol., 1989, 55, 2144–2151 CrossRef CAS PubMed
.
- G. Chen, Reductive dehalogenation of tetrachloroethylene by microorganisms: current knowledge and application strategies, Appl. Microbiol. Biotechnol., 2004, 63, 373–377 CrossRef CAS PubMed
.
- J. A. Cichocki, K. Z. Guyton, N. Guha, W. A. Chiu, I. Rusyn and L. H. Lash, Target organ metabolism, toxicity, and mechanisms of trichloroethylene and perchloroethylene: key similarities, differences, and data gaps, J. Pharm. Exper. Therap., 2016, 359, 110–123 CrossRef CAS PubMed
.
- C. R. Doran and A. Aschengrau, Prenatal and early childhood exposure to tetrachloroethylene (PCE)-contaminated drinking water and sleep quality in adulthood, Environ. Health, 2022, 21, 15 CrossRef CAS PubMed
.
- A. Yu Alentiev, E. Drioli, M. Gokzhaev, G. Golemme, O. Ilinich, A. Lapkin, V. Volkov and Y. Yampolskii, Gas permeation properties of phenylene oxide polymers, J. Membr. Sci., 1998, 138, 99–107 CrossRef
.
- G. Chowdhury, R. Vujosevic, T. Matsuura and B. Laverty, Effects of polymer molecular weight and chemical modification on the gas transport properties of poly(2,6-dimethyl-1,4-phenylene oxide), J. Appl. Polym. Sci., 2000, 77, 1137–1143 CrossRef CAS
.
- Y.-J. Fu, C.-L. Lai, J.-T. Chen, C.-T. Liu, S.-H. Huang, W.-S. Hung, C.-C. Hu and K.-R. Lee, Hydrophobic composite membranes for separating of water-alcohol mixture by pervaporation at high temperature, Chem. Eng. Sci., 2014, 111, 203–210 CrossRef CAS
.
- M. Soniat, M. Tesfaye, A. Mafi, D. J. Brooks, N. D. Humphrey, L.-C. Weng, B. Merinov, W. A. Goddard, A. Z. Weber and F. A. Houle, Permeation of CO2 and N2 through glassy poly(dimethyl phenylene) oxide under steady- and presteady-state conditions, J. Polym. Sci., 2020, 58, 1207–1228 CrossRef CAS
.
- Y. Wu, Z. Guo, H. Wu, K. Zhu, L. Yang, L. Ren, Y. Liu, X. Wu, R. Zhao, N. AliKhan, N. M. Ahmad, M. Younas and Z. Jiang, Plasticization- and aging-resistant membranes with venation-like architecture for efficient carbon capture, J. Membr. Sci., 2020, 609, 118215 CrossRef CAS
.
|
This journal is © The Royal Society of Chemistry 2023 |
Click here to see how this site uses Cookies. View our privacy policy here.