DOI:
10.1039/D2MD00351A
(Research Article)
RSC Med. Chem., 2023,
14, 367-377
Expanding the structure–activity relationships of alkynyl diphenylurea scaffold as promising antibacterial agents†
Received
26th September 2022
, Accepted 25th November 2022
First published on 30th November 2022
Abstract
With the continuous and alarming threat of exhausting the current antimicrobial arsenals, efforts are urgently needed to develop new effective ones. In this study, the antibacterial efficacy of a set of structurally related acetylenic-diphenylurea derivatives carrying the aminoguanidine moiety was tested against a panel of multidrug-resistant Gram-positive clinical isolates. Compound 18 was identified with a superior bacteriological profile than the lead compound I. Compound 18 demonstrated an excellent antibacterial profile in vitro: low MIC values, extended post-antibiotic effect, refractory ability to resistance development upon extended repeated exposure, and high tolerability towards mammalian cells. Finally, when assessed in a MRSA skin infection animal model, compound 18 showed considerable healing and less inflammation, decrease in the bacterial loads in skin lesions, and it surpassed fusidic acid in controlling the systemic dissemination of S. aureus. Collectively, compound 18 represents a promising lead anti-MRSA agent that merits further investigation for the development of new anti-staphylococcal therapeutics.
1. Introduction
The introduction of antibiotics into clinical use has had a huge impact as it elevated the possibility of treating life-threatening infectious diseases and controlling post-operative infections.1 Unfortunately, almost every new antibiotic introduced to the market was followed by the reported emergence of bacterial resistance against it. More seriously, pathogens have not only acquired resistance to single antibiotics but also to multiple antibiotics and to the majority of antibiotic classes discovered to date.2 Bacteria acquire resistance through multiple mechanisms, including the natural, acquired, and cross ones, leading to the emergence of multidrug-resistant (MDR) bacterial strains.3 It was reported that the frequency of life-threatening infectious diseases caused by MDR bacteria has reached alarming levels worldwide.4 It was estimated that by the year 2050, more than 10 million people would die annually as a result of drug-resistant bacterial infections. Therefore, if the situation remains as it is, infections that were previously treated with an antibiotic course in few days could become untreatable.5
Staphylococcus aureus is one of the leading causes of skin, wounds, and hospital acquired infections (HAIs). It is a leading cause of bacteremia, endocarditis, skin and soft tissue infections, and medical device-related infections.6 A successful treatment of infections caused by this resilient organism has become a daunting challenge with the emergence of clinical methicillin-resistant S. aureus (MRSA) isolates, which are currently a leading cause of both hospital-acquired and community-acquired infections worldwide.7,8 According to the Center for Disease Control and Prevention (CDC), MRSA infects about 323
700 patients yearly in the United States alone, resulting in 10
600 deaths and $1.7 billion healthcare costs.9 Linezolid and vancomycin are still the frontline drugs for the treatment of MRSA infections; however, resistance to both antibiotics is rising, highlighting the threat of upcoming non-treatable S. aureus strains.10,11 Consequently, there is a pressing need for the development of new effective anti-staphylococcal therapeutics, preferably with chemical characteristics that vastly differ from those of the existing ones to combat the rapid spread of drug resistance.12
Accordingly, our work is directed towards the synthesis, optimization, and characterization of new anti-MRSA compounds in an effort to uncover and investigate potential new therapeutic candidates targeting this problematic organism.13–24 Previously, we screened a library of aminoguanidine-containing compounds for their anti-MRSA activity.25 The diphenylurea compound was one of the best leads obtained so far with a minimum inhibitory concentration (MIC) of 10 μg mL−1 against the MRSA USA300 strain (Fig. 1). Herein, we investigated the structure–activity relationship of this unique class of antibacterial drugs by replacing the iodine atom with a large library of alicyclic, aromatic, and open-chain acetylenic moieties. The antibacterial profile of the newly synthesized compounds was assessed, and the in vivo efficacy of the most promising compound was investigated in a MRSA skin infection mouse model.
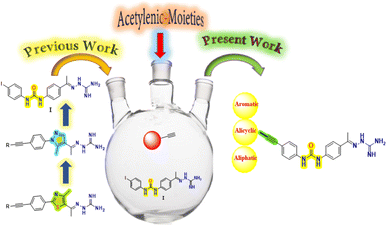 |
| Fig. 1 Previous and current work pipeline. | |
2. Results and discussion
A. Synthetic procedure
Reaction between 4-iodoaniline (1) and isocyanate 2 afforded the key starting material iododiphenylurea 3. The alkyne side chain was tethered using typical Sonogashira cross-coupling conditions, as outlined in Scheme 1. Finally, the desired aminoguanidine derivatives 5–19 were accessed through condensation reactions among 4a–o with aminoguanidine in the presence of a catalytic amount of hydrochloric acid (Scheme 1).
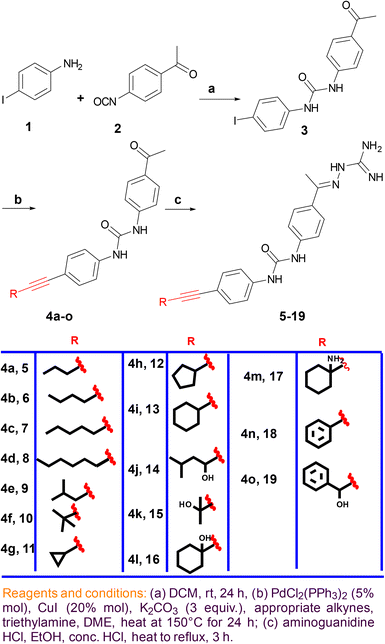 |
| Scheme 1 | |
B. Testing the synthesized compounds for their antibacterial activity
B.1. Preliminary screening of diphenylurea compounds and their structure–activity relationship assessment.
The 15 synthesized diphenylurea compounds were initially screened against three clinically relevant bacteria: two Gram-positive bacteria (MRSA USA300 and Clostridioides difficile) and one Gram-negative bacterium (Escherichia coli JW55031) (Fig. 2). Briefly, compounds with short cyclic chains, such as the cyclopropyl derivative (compound 11) were inactive (MIC ≥ 64 mg mL−1). Interestingly, increasing the ring size to the cyclopentyl and cyclohexyl derivatives provided compounds 12 and 13, with significantly increased potency against MRSA USA300 (MIC = 2 and 4 mg mL−1, respectively).
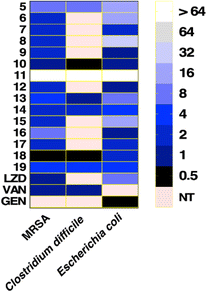 |
| Fig. 2 MICs (μg mL−1) of tested compounds and control drugs against Gram-positive and Gram-negative bacteria. A heat map representing the MICs of the tested compounds according to the color code shown in the figure key. The light red color indicates a non-tested value. Tested compounds are presented on the Y-axis. Bacterial strains tested MRSA NRS384 (USA300), C. difficile ATCC BAA 1870, and E. coli JW55031 (tolC-mutant) are shown on the X-axis. LZD: linezolid, VAN: vancomycin, GEN: gentamicin. | |
Compounds with open chain and branched substituents (5–10) showed potent anti-MRSA activity, with MIC values ranging from 0.5 μg mL−1 to 8 μg mL−1. Moreover, introducing polar groups, such as a hydroxyl group, had no significant effect on the MIC values. On the other hand, derivatives with aromatic substituents (compound 18) exhibited better anti-staphylococcal activity as the MIC value was found to be as low as 0.5 μg mL−1. Introducing a polar group, like hydroxyl group, resulted in an increase in the MIC value from 0.5 to 4 μg mL−1 (Fig. 2).
B.2. Diphenylurea compounds show remarkable anti-staphylococcal activity.
Based on the preliminary screening, ten compounds were picked to further assess their antibacterial activity against a panel of clinical isolates of methicillin-sensitive S. aureus (MSSA), methicillin-resistant S. aureus (MRSA), and vancomycin-resistant S. aureus (VRSA). We also tested the activity of the compounds against vancomycin-resistant Enterococcus faecalis and Enterococcus faecium. Enterococci are the third common cause of nosocomial infections and health-care-related bloodstream infections,26,27 and they are responsible for around 25–50% of the mortality rate among hospitalized patients.28S. epidermidis, a common member of the human epithelial microflora and one of the most frequent nosocomial pathogens, was also included among the tested strains.29 In addition, we tested compounds against Streptococcus pneumoniae, S. pyogenes and S. mutans. Finally, we added Listeria monocytogenes30 to complement the whole set, and we obtained a holistic overview regarding the spectrum of activity of our compounds against a panel of clinically important Gram-positive bacteria.
All of the tested compounds suppressed the growth of the tested strains (MSSA, MRSA, and VRSA) at concentrations as low as 0.5 μg mL−1 (Fig. 3). Compound 18 was the most potent antibacterial compound in this series with MIC values ranging from 0.5 to 2 μg mL−1. Similarly, compounds 6 and 9 inhibited the strains tested with MIC values ranging from 0.5 μg mL−1 to 2 μg mL−1. Interestingly, the antibacterial effect of the diphenylurea scaffold was extended to include linezolid-resistant and vancomycin-resistant staphylococcal strains, suggesting that they do not share the same resistance mechanism as linezolid or vancomycin.
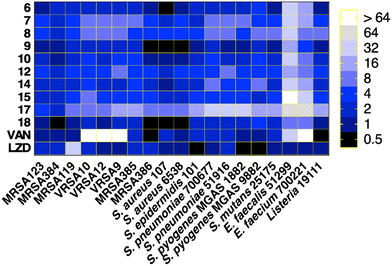 |
| Fig. 3 MICs of diphenylurea compounds against a panel of clinically relevant Gram-positive bacteria. A heat map representing the MICs of each of the tested compounds, according to the color code shown in the figure key. Tested bacteria are shown on the X-axis and compounds codes on the Y-axis. | |
B.3. All tested compounds have good tolerability towards mammalian cells.
Testing the tolerability of new antibacterial candidates is a crucial step in order to identify compounds with limited toxic effects to the host tissues. Therefore, the diphenylurea compounds were assessed for toxicity to two mammalian cells, monkey kidney epithelial cells (Vero cells) and human keratinocyte (HaCaT) cells. The eight tested compounds exhibited good tolerability against Vero cells. Compound 17 was tolerable to Vero cells at concentrations up to 128 μg mL−1, as around 100% of the cells remained viable (Fig. 4A). Compounds 6, 7, 8, 9, 12, 15 and 18 had limited tolerability at a concentration of 128 μg mL−1. However, they did not affect the cell viability at the concentration of 64 μg mL−1 (Fig. 4A). The hydroxybutyne derivative 15 displayed tolerability to HaCaT cells at concentrations up to 128 μg mL−1, where around 100% of the cells remained viable. Compounds 7, 8, 12 and 17 were tolerable to HaCaT cells at a concentration as high as 64 μg mL−1 showing ∼100% cell viability after the treatment (Fig. 4B).
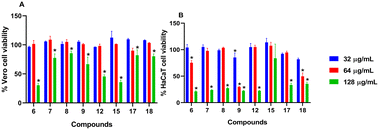 |
| Fig. 4
In vitro cytotoxicity assessment of the diphenylurea compounds using the MTS assay. Compounds were assayed (at concentrations of 32, 64 and 128 μg mL−1) against Vero cells (A) and HaCaT cells (B) to determine their potential toxic effect. Cells were incubated with the compounds at 37 °C with 5% CO2 for 24 hours. The assay reagent MTS was subsequently added, and the plate was incubated for four hours before the absorbance readings (OD490) were recorded. The data were analyzed via a two-way ANOVA with post hoc Dunnett's test for multiple comparisons. (*) denotes a statistical difference (p < 0.05) between values obtained for the compounds, as compared to DMSO. | |
B.4. Compound 18 exhibits low hemolytic activity.
Following the whole assessment of our synthesized compounds, compound 18 showed promising results. Hence, it was chosen for further testing with the hemolysis assay. The hemolytic activity was concentration-dependent and was measured as low as 2% at a concentration of 16 μg mL−1. The hemolysis increased with the increase in the concentration, yet it was significantly lower than the tested hemolysis control with Triton X-100 up to 64 μg mL−1 (Fig. 5).
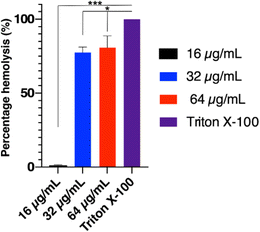 |
| Fig. 5 Hemolytic potential of compound 18. Three concentrations of compound 18 (16, 32 and 64 μg mL−1) were mixed with freshly prepared rabbit erythrocyte suspension and incubated at 37 °C for 30 min. The mixtures were centrifuged at 13 000 × g for 5 min, and the absorbance of the supernatant was measured at 540 nm. Triton X-100 represents the positive control for complete hemolysis. Results are presented as percent hemolysis relative to Triton X-100. The experiment was repeated twice for every tested concentration. Error bars represent standard deviation values. Statistical analysis was done using ordinary one-way ANOVA, followed by the Tukey's multiple comparison test. The (*) indicates a statistical difference (p ≤ 0.05). The charts were generated using GraphPad Prism (version 9.0). | |
B.5. Compound 18 demonstrates high refractory ability for resistance development following repeated multiple exposure.
After compound 18 was tested and proved to be safe on the mammalian cell lines, we aimed at testing the potential for resistance emergence against compound 18. A multi-step resistance test was conducted as reported earlier31 using the broth microdilution assay to determine the MIC of MRSA USA300 against the diphenylurea compound 18 and rifampicin for 14 consecutive passages.
The MIC values of compound 18 remained unchanged for 4 passages and then showed only a one-fold increase in the MIC for the rest of the passages (Fig. 6). In contrast, the MIC value for rifampicin against MRSA USA300 increased rapidly and surpassed the four-fold change after only four passages. The MIC continued increasing until it reached around 15-fold increase by the end of the experiment (Fig. 6). The results demonstrated the low chances of resistance development to our tested compound in comparison to the control rifampicin antibiotic.
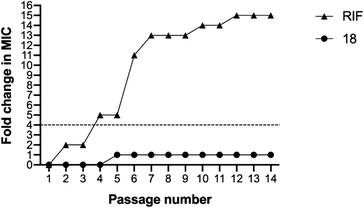 |
| Fig. 6 Stable MICs of compound 18 after passaging. MRSA USA300 was serially passed using the microdilution broth assay over a two-week period to determine the MIC of each compound after each passage. A four-fold shift in MIC would be indicative of bacterial resistance to the test agent. | |
B.6. Compound 18 demonstrates post-antibiotic effect (PAE) superior to that of vancomycin against MRSA USA300.
Bacterial growth may be inhibited following exposure to an antibiotic even after the drug concentration has fallen below the MIC value. This term is coined as the “Post-antibiotic effect” which is an extra feature that adds up to the overall advantages of a proposed compound. By definition, PAE is the time required for the bacteria exposed to each test agent to increase by one log10 cycle relative to the untreated control. Compound 18 showed an extended PAE of 5 hours, exceeding the vancomycin control by one hour (Fig. 7).
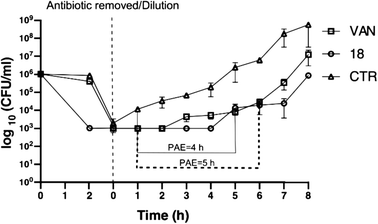 |
| Fig. 7 Post-antibiotic effect of compound 18. Regrowth curves of the MRSA USA300 strain exposed for 2 h to 5X MIC of compound 18 (open circle), 5X MIC of vancomycin (VAN; open square), or DMSO (CTR; open triangle). The duration in hours (h) of the PAE of each test culture was calculated and is indicated as a solid line for vancomycin or dotted line for compound 18. | |
B.7. Compound 18 effectively inhibits MRSA in a murine model of skin infection.
The ability of compound 18 to control an in vivo S. aureus skin infection was assessed in comparison to fusidic acid (FA) as a positive control and the petroleum jelly vehicle (PJ). Mice infected subcutaneously with the MRSA USA300 developed skin lesions within 72-hour post-infection. The group treated with just the vehicle (PJ) suffered from extensive skin damage and inflammation that continued to progress until the end of the experiment (Fig. 8A). Conversely, mice treated with either compound 18 or FA showed considerable healing and less inflammation (Fig. 8A). Upon estimating the bacterial burdens in the skin lesions, it was observed that compound 18 resulted in a decrease in the MRSA burden in the mice skin lesions by about 0.5
log10. Yet, this reduction was not significant as compared to the FA treatment, which generated around 1.5
log10 as compared to the vehicle-treated mice (Fig. 8B). On the other hand, compound 18 demonstrated superior activity compared to FA in controlling the systemic dissemination of the S. aureus, as evidenced by the higher reduction (>1
log10) in the bacterial burden detected in the spleens of the infected mice as compared to the vehicle-treated group (Fig. 8C).
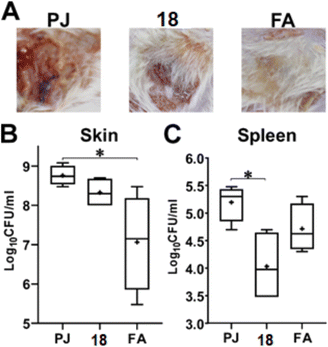 |
| Fig. 8 Compound 18 is superior in controlling the systemic dissemination of S. aureus from a skin infection. Mice infected subcutaneously with approximately 2 × 109 CFU of S. aureus USA300 developed an open wound/abscess at the site of infection within 72-hour post-infection. Topical application of petroleum jelly (PJ), 2% of compound 18 in PJ or commercial ointment with 2% fusidic acid (FA) was carried out twice daily for three more consecutive days. A. Photographs of the representative mice from the respective three groups on the last day of the course of the infection (day 7). Box plots of the bacterial burden recovered from the skin lesions (B) and the spleens (C) of the infected mice of the three groups. In both plots, the whiskers span the difference between the minimum and maximum readings, the horizontal bar represents the median, and the (+) sign represents the mean of the log10 CFU ml−1. Statistical analysis was done using the ordinary one-way ANOVA, followed by the Tukey's multiple comparison test. The (*) indicates a statistical difference (p ≤ 0.05). The charts were generated using GraphPad Prism (version 9.0). | |
3. Experimental
3.1. Chemistry
3.1.1. General.
1H NMR spectra were recorded at 400 MHz and 13C NMR spectra were recorded at 100 MHz in deuterated chloroform (CDCl3), or dimethyl sulfoxide (DMSO-d6) on a Bruker VX-400 NMR spectrometer. Chemical shifts are given in parts per million (ppm) on the delta (δ) scale. Chemical shifts were calibrated relative to those of the solvents. Flash chromatography was performed on a 230–400 mesh silica. The progress of reactions was monitored with Merck silica gel IB2-F plates (0.25 mm thickness). The infrared spectra were recorded in potassium bromide disks on a pye Unicam SP 3300 and Shimadzu FT IR 8101 PC infrared spectrophotometer. Mass spectra were recorded at 70 eV. High-resolution mass spectra for all ionization techniques were obtained from a FinniganMAT XL95. Melting points were determined using capillary tubes with a Stuart SMP30 apparatus and are uncorrected. All yields reported refer to isolated yields.
3.1.2. Preparation of diphenylurea derivatives 3.
4-Iodoaniline (1 equiv.) was added to 4-acetylphenyl isocyante (2, 1 equiv.) in dry DCM (50 mL). The reaction mixture was stirred at room temperature for 24 hours. The precipitated was filtered off and the crude product was purified by crystallization from methanol-ethyl acetate (1
:
1) to afford the desired compound (3).
1-(4-Acetylphenyl)-3-(4-iodophenyl)urea (3).
Buff solid: mp 192–193 °C. as reported.32
3.1.3. 1-(4-Acetylphenyl)-3-(4-(pent-1-yn-1-yl)phenyl)urea 4a. General procedure.
To dry DME (25 mL) in a 75 mL sealed tube, compound 3 (500 mg, 1.3 mmol), appropriate alkynes (1.97 mmol, 1.5 equiv.), triethylamine (3 mL), and potassium carbonate (363 mg, 2.63 mmol, 2 equiv.) were added. After the reaction mixture was purged with dry nitrogen gas for 10 min, dichloro-bis(triphenylphosphine) palladium(II) (46 mg, 0.065 mmol, 5% mole) and copper(I) iodide (50 mg, 0.263 mmol, 20% mole) were added. The sealed tube was then placed in an oil bath and stirred at 65 °C for 16 h. After cooling to room temperature, the reaction mixture was passed through celite, followed by DCM (50 mL). The organic materials were then concentrated under reduced pressure. The crude materials were purified and washed via silica gel flash column chromatography using hexane-ethyl acetate (6
:
4). Yields, physical properties, and spectral data of isolated purified products are listed below:
1-(4-Acetylphenyl)-3-(4-(pent-1-yn-1-yl)phenyl)urea 4a.
White solid (120 mg, 71.2%): mp 195–193 °C. 1H NMR (DMSO-d6); δ 9.17 (brs, 1H), 8.99 (brs, 1H), 7.93 (d, J = 8.7 Hz, 2H), 7.60 (d, J = 8.7 Hz, 2H), 7.49 (d, J = 8.7 Hz, 2H), 7.33 (d, J = 8.7 Hz, 2H), 2.46 (t, J = 7.5 Hz, 2H), 2.25 (s, 3H), 1.64–1.55 (m, 2H), 1.05 (t, J = 7.1 Hz, 3H); 13C NMR (DMSO-d6); δ 196.97, 152.46, 144.59, 139.76, 132.41, 131.03, 130.12, 118.72, 117.76, 116.46, 95.35, 80.92, 25.31, 22.14, 21.15, 17.47; MS (m/z); 320.39 (M+, 78.61%).
1-(4-Acetylphenyl)-3-(4-(hex-1-yn-1-yl)phenyl)urea 4b.
Yellow oil (139 mg, 79%): 1H NMR (DMSO-d6); δ 9.84 (brs, 1H), 9.61 (brs, 1H), 7.93 (d, J = 8.8 Hz, 2H), 7.60 (d, J = 8.8 Hz, 2H), 7.49 (d, J = 8.4 Hz, 2H), 7.33 (d, J = 8.4 Hz, 2H), 2.46 (t, J = 7.9 Hz, 2H), 2.25 (s, 3H), 1.64–1.57 (m, 4H), 1.05 (t, J = 6.8 Hz, 3H); 13C NMR (DMSO-d6); δ 196.25, 152.43, 148.42, 138.43, 138.20, 137.09, 135.70, 132.02, 126.84, 117.59, 92.52, 81.00, 28.35, 25.31, 22.13, 19.17, 17.48; MS (m/z); 334.41 (M+, 62.41%).
1-(4-Acetylphenyl)-3-(4-(hept-1-yn-1-yl)phenyl)urea 4c.
Brown oil (200 mg, 76.9%): 1H NMR (DMSO-d6); δ 9.17 (brs, 1H), 8.99 (brs, 1H), 7.93 (d, J = 8.1 Hz, 2H), 7.60 (d, J = 8.5 Hz, 2H), 7.49 (d, J = 8.4 Hz, 2H), 7.33 (d, J = 8.4 Hz, 2H), 2.80 (t, J = 7.9 Hz, 2H), 2.23 (s, 3H), 1.59–141 (m, 6H), 0.96 (t, J = 6.5 Hz, 3H); 13C NMR (DMSO-d6); δ 198.51, 155.68, 148.42, 138.20, 137.09, 135.70, 132.05, 126.84, 124.05, 117.59, 92.52, 81.00, 31.04, 28.35, 25.31, 22.13, 17.48; MS (m/z); 348.44 (M+, 69.41%).
1-(4-Acetylphenyl)-3-(4-(oct-1-yn-1-yl)phenyl)urea 4d.
Brown oil (220 mg, 72%): 1H NMR (DMSO-d6); δ 9.19 (brs, 1H), 8.98 (brs, 1H), 7.93 (d, J = 8.4 Hz, 2H), 7.60 (d, J = 8.4 Hz, 2H), 7.49 (d, J = 8.4 Hz, 2H), 7.33 (d, J = 8.4 Hz, 2H), 2.48 (t, J = 7.2 Hz, 2H), 2.23 (s, 3H), 1.58–1.43 (m, 8H), 0.96 (t, J = 6.1 Hz, 3H); 13C NMR (DMSO-d6); δ 198.51, 155.68, 152.43, 148.42, 138.20, 137.09, 135.70, 132.05, 126.84, 124.05, 117.59, 92.52, 81.00, 31.04, 28.35, 25.31, 22.13, 19.17, 17.48, 14.36; MS (m/z); 348.44 (M+, 69.41%).
1-(4-Acetylphenyl)-3-(4-(4-methylpent-1-yn-1-yl)phenyl)urea 4e.
Yellowish white solid (230 mg, 87%); mp 198–199 °C. 1H NMR (DMSO-d6); δ 9.17 (brs, 1H), 9.00 (brs, 1H), 8.29 (d, J = 8.5 Hz, 2H), 7.94 (d, J = 8.5 Hz, 2H), 7.70 (d, J = 8.6 Hz, 2H), 7.51 (d, J = 8.7 Hz, 2H), 2.38 (d, J = 7.2 Hz, 2H), 2.23 (s, 3H), 1.92–1.85 (m, 1H), 1.04 (d, J = 7.2 Hz, 6H); 13C NMR (DMSO-d6); δ 200.65, 157.55, 139.24, 137.19, 132.21, 129.39, 127.46, 125.16, 123.99, 117.81, 91.98, 81.75, 29.87, 28.27, 25.24, 22.27; MS (m/z); 334.41 (M+, 92.31%).
1-(4-Acetylphenyl)-3-(4-(3,3-dimethylbut-1-yn-1-yl)phenyl)urea 4f.
Yellow oil (195 mg, 74%); 1H NMR (DMSO-d6); δ 9.17 (brs, 1H), 9.00 (brs, 1H), 8.29 (d, J = 8.4 Hz, 2H), 7.94 (d, J = 8.4 Hz, 2H), 7.70 (d, J = 8.5 Hz, 2H), 7.51 (d, J = 8.5 Hz, 2H), 2.23 (s, 3H), 1.32 (s, 9H); 13C NMR (DMSO-d6); δ 200.24, 152.34, 148.35, 138.14, 137.09, 135.73, 132.04, 126.76, 123.90, 117.49, 100.27, 79.41, 31.22, 28.14, 25.31; MS (m/z); 334.41 (M+, 92.31%).
1-(4-Acetylphenyl)-3-(4-(cyclopropylethynyl)phenyl)urea 4g.
Yellow oil (198 mg, 78.8%); 1H NMR (DMSO-d6); δ 9.16 (brs, 1H), 8.93 (brs, 1H), 7.94 (d, J = 8.5 Hz, 2H), 7.61 (d, J = 8.5 Hz, 2H), 7.49 (d, J = 8.4 Hz, 2H), 7.33 (d, J = 8.4 Hz, 2H), 2.23 (s, 3H), 1.61–1.56 (m, 1H), 0.93–0.92 (m, 2H), 0.79–0.78 (m, 2H); 13C NMR (DMSO-d6); δ 202.85, 151.53, 147.51, 137.27, 136.23, 134.86, 131.23, 125.96, 123.10, 116.73, 94.81, 75.23, 24.47, 8.11, 0.50; MS (m/z); 318.37 (M+, 78.61%).
1-(4-Acetylphenyl)-3-(4-(cyclopentylethynyl)phenyl)urea 4h.
Brown oil (200 mg, 73%); 1H NMR (DMSO-d6); δ 9.46 (brs, 1H), 9.17 (brs, 1H), 7.93 (d, J = 8.4 Hz, 2H), 7.60 (d, J = 8.4 Hz, 2H), 7.49 (d, J = 8.4 Hz, 2H), 7.33 (d, J = 8.4 Hz, 2H), 2.91–2.87 (m, 1H), 2.23 (s, 3H), 2.03–1.60 (m, 8H); 13C NMR (DMSO-d6); δ 200.69, 157.55, 139.29, 137.19, 132.21, 129.39, 127.41, 125.19, 123.99, 117.75, 97.07, 80.36, 33.91, 30.60, 29.87, 25.11; MS (m/z); 346.43 (M+, 62.61%).
1-(4-Acetylphenyl)-3-(4-(cyclohexylethynyl)phenyl)urea 4i.
Light brown oil (210 mg, 73.8%); 1H NMR (DMSO-d6); δ 9.17 (brs, 1H), 8.99 (brs, 1H), 7.93 (d, J = 8.7 Hz, 2H), 7.60 (d, J = 8.7 Hz, 2H), 7.49 (d, J = 8.4 Hz, 2H), 7.33 (d, J = 8.4 Hz, 2H), 2.24 (s, 3H), 1.87–1.69 (m, 1H), 1.54–1.31 (m, 10H); 13C NMR (DMSO-d6); δ 196.97, 152.46, 144.59, 139.76, 132.41, 131.03, 130.12, 118.72, 117.76, 116.46, 95.35, 80.92, 29.23, 25.75, 25.19, 22.30, 21.47; MS (m/z); 360.46 (M+, 69.61%).
1-(4-Acetylphenyl)-3-(4-(3-hydroxy-5-methylhex-1-yn-1-yl)phenyl) urea 4j.
White solid (220 mg, 76.5%); mp 201–203 °C. 1H NMR (DMSO-d6); δ 9.17 (brs, 1H), 8.99 (brs, 1H), 7.93 (d, J = 8.4 Hz, 2H), 7.60 (d, J = 8.4 Hz, 2H), 7.49 (d, J = 8.4 Hz, 2H), 7.33 (d, J = 8.4 Hz, 2H), 5.42 (brs, 1H), 2.60 (s, 3H), 2.36 1.31–1.01 (m, 11H); 13C NMR (DMSO-d6); δ 200.65, 157.55, 139.24, 137.19, 132.21, 131.54, 129.39, 127.46, 125.16, 117.81, 91.98, 81.75, 47.82, 29.87, 28.27, 28.08, 25.24; MS (m/z); 364.44 (M+, 88.31%).
1-(4-Acetylphenyl)-3-(4-(3-hydroxy-3-methylbut-1-yn-1-yl)phenyl) urea 4k.
Yellow oil (184 mg, 69.3%); 1H NMR (DMSO-d6); δ 9.27 (brs, 1H), 9.15 (brs, 1H), 7.93 (d, J = 8.4 Hz, 2H), 7.60 (d, J = 8.4 Hz, 2H), 7.50 (d, J = 8.5 Hz, 2H), 7.34 (d, J = 8.5 Hz, 2H), 5.52 (brs, 1H), 2.23 (s, 3H), 1.51 (s, 6H); 13C NMR (DMSO-d6); δ 204.00, 160.36, 155.73, 152.21, 148.32, 137.12, 135.88, 132.21, 126.90, 117.71, 89.88, 70.29, 59.29, 29.15, 25.32; MS (m/z); 336.39 (M+, 72.45%).
1-(4-Acetylphenyl)-3-(4-((1-hydroxycyclohexyl)ethynyl)phenyl) urea 4l.
Brown oil (205 mg, 69%); 1H NMR (DMSO-d6); δ 9.46 (brs, 1H), 9.17 (brs, 1H), 7.93 (d, J = 8.2 Hz, 2H), 7.60 (d, J = 8.2 Hz, 2H), 7.49 (d, J = 8.4 Hz, 2H), 7.33 (d, J = 8.4 Hz, 2H), 6.23 (brs, 1H), 2.32 (s, 3H), 2.18–1.15 (m, 10H); 13C NMR (DMSO-d6); δ 196.97, 152.46, 144.59, 139.76, 132.41, 131.03, 130.12, 118.72, 117.76, 116.46, 95.35, 80.92, 58.38, 29.23, 25.75, 25.19, 22.30, 21.47; MS (m/z); 376.45 (M+, 61.61%).
1-(4-Acetylphenyl)-3-(4-((1-aminocyclohexyl)ethynyl)phenyl)urea 4m.
Dark brown oil (196 mg, 66%); 1H NMR (DMSO-d6); δ 9.07 (brs, 1H), 8.89 (brs, 1H), 8.29 (d, J = 8.4 Hz, 2H), 8.13 (d, J = 8.4 Hz, 2H), 7.91 (brs, 2H), 7.70 (d, J = 8.4 Hz, 2H), 7.49 (d, J = 8.4 Hz, 2H), 2.67 (s, 3H), 1.72–1.56 (m, 10H); 13C NMR (DMSO-d6); δ 197.00, 153.02, 144.35, 135.03, 134.22, 132.62, 130.64, 122.16, 117,91, 91.01, 85.21, 49.41, 40.21, 27.71, 25.62, 21.90; MS (m/z); 376.45 (M+, 61.61%).
1-(4-Acetylphenyl)-3-(4-(phenylethynyl)phenyl)urea 4n.
Brown oil (215 mg, 76.8%); 1H NMR (DMSO-d6); δ 9.85 (brs, 1H), 9.61 (brs, 1H), 8.17 (d, J = 8.4 Hz, 2H), 7.82–7.75 (m, 5H), 7.72 (d, J = 8.4 Hz, 2H), 7.62 (d, J = 8.4 Hz, 2H), 7.47 (d, J = 8.4 Hz, 2H), 2.25 (s, 3H); 13C NMR (DMSO-d6); δ 201.29, 155.79, 152.25, 148.44, 139.02, 137,13, 135.85, 132.24, 129.27, 127,01, 122.92, 122.72, 117.75, 91.01, 89.84, 25.34; MS (m/z); 354.40 (M+, 48.51%).
1-(4-Acetylphenyl)-3-(4-(3-hydroxy-3-phenylprop-1-yn-1-yl)phenyl)urea 4o.
Brown oil (187 mg, 61.6%); 1H NMR (DMSO-d6); δ 9.37 (brs, 1H), 9.19 (brs, 1H), 8.62 (d, J = 8.4 Hz, 2H), 8.52 (d, J = 8.4 Hz, 2H), 8.38 (d, J = 8.4 Hz, 2H), 8.08 (d, J = 8.4 Hz, 2H), 7.44–6.99 (m, 5H), 4.92 (brs, 1H), 2.73 (s, 3H); 13C NMR (DMSO-d6); δ 200.69, 155.77, 152.22, 148.43, 139,45, 139.36, 137.14, 132.32, 128,97, 128.77, 127.74, 126.96, 122.42, 117.78, 89.72, 86.93, 63.90, 25.31; MS (m/z); 384.44 (M+, 38.31%).
3.1.4. 2-(1-(4-(3-(4-(Alkyne)phenyl)ureido)phenyl)ethylidene)hydrazine-1-carboximidamide 5–19. General procedure.
Compounds 4a–o (0.31 mmol) were dissolved in absolute ethanol (20 mL), concentrated hydrochloric acid (0.5 mL), aminoguanidine hydrochloride (69 mg, 6.2 mmol, 2 equiv.), were then added. The reaction mixture was heated at reflux for 2 h. The solvent was concentrated under reduced pressure, then poured in crushed ice and neutralized with sodium carbonate to pH 7–8, and the formed precipitated solid was collected by filtration and washed with copious amount of water. Crystallization from DCM afforded the desired products as solids.
2-(1-(4-(3-(4-(Pent-1-yn-1-yl)phenyl)ureido)phenyl)ethylidene) hydrazine-1-carboximidamide 5.
White solid (90 mg, 76.6%): mp 210–211 °C; 1H NMR (DMSO-d6); δ 9.47 (brs, 1H), 9.17 (brs, 1H), 8.10 (d, J = 8.4 Hz, 2H), 7.82 (d, J = 8.4 Hz, 2H), 7.79 (d, J = 8.2 Hz, 2H), 7.53 (d, J = 8.2 Hz, 2H), 5.72 (brs, 2H), 5.52 (brs, 2H), 2.46 (t, J = 7.1 Hz, 2H), 2.25 (s, 3H), 1.62–1.55 (m, 2H), 1.05–1.01 (t, J = 7.6 Hz, 3H); 13C NMR (DMSO-d6); δ 160.33, 155.68, 152.41, 148.38, 138.22, 137.09, 135.73, 132.04, 126.85, 124.03, 117.59, 92.34, 81.16, 25.31, 22.14, 21.15, 17.47; HRMS (EI) m/z 376.2018 M+, calc. for C21H24N6O 376.2012; anal. calc. for: C21H24N6O (376.46): C, 67.00; H, 6.43; N, 22.32%; found: C, 67.91; H, 6.98; N, 20.66%.
2-(1-(4-(3-(4-(Hex-1-yn-1-yl)phenyl)ureido)phenyl)ethylidene) hydrazine-1-carboximidamide 6.
Off-white solid (88 mg, 75%): mp 215–217 °C; 1H NMR (DMSO-d6); δ 9.17 (brs, 1H), 8.99 (brs, 1H), 7.93 (d, J = 8.4 Hz, 2H), 7.60 (d, J = 8.4 Hz, 2H), 7.49 (d, J = 8.4 Hz, 2H), 7.33 (d, J = 8.4 Hz, 2H), 5.74 (brs, 2H), 5.55 (brs, 2H), 2.45 (t, J = 7.8 Hz, 2H), 2.24 (s, 3H), 1.61–130 (m, 4H), 0.93 (t, J = 7.2 Hz, 3H); 13C NMR (DMSO-d6); δ 160.29, 155.68, 152.43, 148.42, 138.20, 137.09, 135.70, 132.05, 126.84, 124.05, 117.59, 92.52, 81.00, 31.04, 28.35, 25.31, 22.13, 17.48; HRMS (EI) m/z 390.2164 M+, calc. for C22H26N6O 390.2168; anal. calc. for: C22H26N6O (390.46): C, 67.67; H, 6.71; N, 21.52%; found: C, 67.91; H, 7.02; N, 19.99%.
2-(1-(4-(3-(4-(Hept-1-yn-1-yl)phenyl)ureido)phenyl)ethylidene) hydrazine-1-carboximidamide 7.
Light-brown solid (89 mg, 73.5%): mp 208–210 °C; 1H NMR (DMSO-d6); 9.30 (brs, 1H), 9.17 (brs, 1H), 8.10 (d, J = 8.8 Hz, 2H), 7.79 (d, J = 8.8 Hz, 2H), 7.49 (d, J = 8.6 Hz, 2H), 7.25 (d, J = 8.6 Hz, 2H), 5.71 (brs, 2H), 5.50 (brs, 2H), 2.47 (t, J = 7.2 Hz, 2H), 2.23 (s, 3H), 1.59–143 (m, 6H), 0.96 (t, J = 7.8 Hz, 3H); 13C NMR (DMSO-d6); δ 160.34, 155.68, 152.41, 148.39, 138.20, 137.73, 135.73, 132.03, 126.84, 124.04, 117.54, 92.47, 81.00, 30.74, 25,30, 21.93, 18.88, 17.96, 13.96; HRMS (EI) m/z 404.2336 M+, calc. for C23H28N6O 404.2325; anal. calc. for: C23H28N6O (404.51): C, 68.29; H, 6.98; N, 20.78%; found: C, 67.91; H, 7.38; N, 20.03%.
2-(1-(4-(3-(4-(Oct-1-yn-1-yl)phenyl)ureido)phenyl)ethylidene) hydrazine-1-carboximidamide 8.
Brown solid (80 mg, 69%): mp 211–213 °C; 1H NMR (DMSO-d6); δ 9.19 (brs, 1H), 8.98 (brs, 1H), 7.93 (d, J = 8.4 Hz, 2H), 7.60 (d, J = 8.4 Hz, 2H), 7.49 (d, J = 7.8 Hz, 2H), 7.33 (d, J = 7.8 Hz, 2H), 5.72 (brs, 2H), 5.42 (brs, 2H), 2.48 (t, J = 6.8 Hz, 2H), 2.23 (s, 3H), 1.58–143 (m, 8H), 0.96 (t, J = 7.2 Hz, 3H); 13C NMR (DMSO-d6); δ 160.35, 155.67, 152.40, 148.35, 138.21, 137.08, 135.73, 132.01, 126.85, 124.04, 117.59, 92.52, 81.01, 31.23, 28,60, 28.47, 25.32, 22.50, 17.46, 14.39; HRMS (EI) m/z 418.2495 M+, calc. for C24H30N6O 418.2481; anal. calc. for: C24H30N6O (418.58): C, 68.87; H, 7.23; N, 20.08%; found: C, 67.91; H, 7.38; N, 20.03%.
2-(1-(4-(3-(4-(4-Methylpent-1-yn-1-yl)phenyl)ureido)phenyl) ethylidene)hydrazine-1-carboximidamide 9.
Yellowish white solid (93 mg, 79.6%); mp 198–199 °C, 1H NMR (DMSO-d6); δ 9.17 (brs, 1H), 9.00 (brs, 1H), 8.29 (d, J = 8.6 Hz, 2H), 7.94 (d, J = 8.6 Hz, 2H), 7.70 (d, J = 8.7 Hz, 2H), 7.51 (d, J = 8.7 Hz, 2H), 5.71 (brs, 2H), 5.51 (brs, 2H), 2.38 (d, J = 7.6 Hz, 2H), 2.23 (s, 3H), 1.92–1.85 (m, 1H), 1.04 (d, J = 7.6 Hz, 6H); 13C NMR (DMSO-d6); δ 160.33, 155.68, 152.41, 148.37, 138.22, 137.10, 135.74, 132.04, 126.85, 124.04, 117.60, 91.36, 81.90, 28.11, 25.32, 22.31, 17.47; HRMS (EI) m/z 390.2149 M+, calc. for C22H26N6O 390.2168; anal. calc. for: C22H26N6O (390.49): C, 67.67; H, 6.71; N, 21.52%; found: C, 67.91; H, 7.55; N, 20.89%.
2-(1-(4-(3-(4-(3,3-Dimethylbut-1-yn-1-yl)phenyl)ureido)phenyl) ethylidene) hydrazine-1-carboximidamide 10.
Beige solid (96 mg, 82%); mp 201–202 °C, 1H NMR (DMSO-d6); δ 9.17 (brs, 1H), 9.00 (brs, 1H), 8.29 (d, J = 8.4 Hz, 2H), 7.94 (d, J = 8.4 Hz, 2H), 7.70 (d, J = 8.5 Hz, 2H), 7.51 (d, J = 8.5 Hz, 2H), 5.71 (brs, 2H), 5.51 (brs, 2H), 2.23 (s, 3H), 1.32 (s, 9H); 13C NMR (DMSO-d6); δ 160.36, 155.67, 152.34, 148.35, 138.14, 137.09, 135.73, 132.02, 126.79, 123.90, 117.56, 100.27, 79.41, 31.22, 28.14, 17.47; HRMS (EI) m/z 390.2170 M+, calc. for C22H26N6O 390.2168; anal. calc. for: C22H26N6O (390.49): C, 67.67; H, 6.71; N, 21.52%; found: C, 67.91; H, 7.55; N, 20.89%.
2-(1-(4-(3-(4-(Cyclopropylethynyl)phenyl)ureido)phenyl) ethylidene)hydrazine-1-carboximidamide 11.
Yellow solid (97 mg, 82%); mp 205–207 °C, 1H NMR (DMSO-d6); δ 9.27 (brs, 1H), 9.15 (brs, 1H), 7.93 (d, J = 8.4 Hz, 2H), 7.60 (d, J = 8.4 Hz, 2H), 7.49 (d, J = 8.4 Hz, 2H), 7.33 (d, J = 8.4 Hz, 2H), 5.72 (brs, 2H), 5.53 (brs, 2H), 2.23 (s, 3H), 1.61–1.56 (m, 1H), 0.93–0.92 (m, 2H), 0.79–0.78 (m, 2H); 13C NMR (DMSO-d6); δ 159.50, 154.82, 151.53, 147.51, 137.27, 136.23, 134.86, 131.23, 125.96, 123.10, 116.73, 94.81, 75.23, 24.47, 16.62, 0.50; HRMS (EI) m/z 374.1857 M+, calc. for C21H22N6O 374.1855; anal. calc. for: C21H22N6O (374.44): C, 67.36; H, 5.92; N, 22.44%; found: C, 67.81; H, 6.41; N, 21.89%.
2-(1-(4-(3-(4-(Cyclopentylethynyl)phenyl)ureido)phenyl) ethylidene)hydrazine-1-carboximidamide 12.
Yellow solid (95 mg, 81.7%); mp 215–217 °C, 1H NMR (DMSO-d6); δ 9.46 (brs, 1H), 9.17 (brs, 1H), 7.93 (d, J = 8.6 Hz, 2H), 7.60 (d, J = 8.6 Hz, 2H), 7.49 (d, J = 8.4 Hz, 2H), 7.33 (d, J = 8.4 Hz, 2H), 5.74 (brs, 1H), 5.55 (brs, 1H), 5.25 (brs, 2H), 2.91–2.87 (m, 1H), 2.23 (s, 3H), 2.03–1.60 (m, 8H); 13C NMR (DMSO-d6); δ 160.33, 155.67, 152.40, 148.39, 138.12, 137.08, 135.69, 132.00, 126.80, 124.07, 117.56, 96.45, 80.50, 33.95, 30.59, 25.10, 17.47; HRMS (EI) m/z 402.2160 M+, calc. for C23H26N6O 402.2168; anal. calc. for: C23H26N6O (402.50): C, 68.63; H, 6.51; N, 20.88%; found: C, 68.46; H, 7.11; N, 20.42%.
2-(1-(4-(3-(4-(Cyclohexylethynyl)phenyl)ureido)phenyl)ethylidene) hydrazine-1-carboximidamide 13.
Brown solid (84 mg, 72.6%); mp 216–218 °C. 1H NMR (DMSO-d6); δ 9.25 (brs, 1H), 9.17 (brs, 1H), 7.93 (d, J = 8.2 Hz, 2H), 7.60 (d, J = 8.2 Hz, 2H), 7.49 (d, J = 8.4 Hz, 2H), 7.33 (d, J = 8.4 Hz, 2H), 5.77 (brs, 2H), 5.61 (brs, 2H), 2.24 (s, 3H), 1.87–1.31 (m, 11H); 13C NMR (DMSO-d6); δ 160.27, 155.68, 152.43, 148.47, 138.16, 137.09, 135.67, 132.04, 126.82, 124.02, 117.57, 96.20, 80.97, 32.66, 29.37, 25.83, 25.29, 24.79; HRMS (EI) m/z 416.2333 M+, calc. for C24H28N6O 416.2325; anal. calc. for: C24H28N6O (416.52): C, 69.21; H, 6.78; N, 20.18%; found: C, 68.91; H, 7.67; N, 19.89%.
2-(1-(4-(3-(4-(3-Hydroxy-5-methylhex-1-yn-1-yl)phenyl)ureido) phenyl)ethylidene)hydrazine-1-carboximidamide 14.
Yellow solid (89 mg, 77.2%); mp 222–224 °C. 1H NMR (DMSO-d6); δ 9.13 (brs, 1H), 8.95 (brs, 1H), 7.93 (d, J = 8.2 Hz, 2H), 7.60 (d, J = 8.2 Hz, 2H), 7.49 (d, J = 8.4 Hz, 2H), 7.33 (d, J = 8.4 Hz, 2H), 5.71 (brs, 1H), 5.51 (brs, 2H), 5.20 (brs, 2H), 2.38 (t, J = 6.4 Hz, 1H), 2.23 (s, 3H), 1.93–1.83 (m, 3H), 1.04 (d, J = 7.4 Hz, 6H); 13C NMR (DMSO-d6); δ 160.33, 155.68, 152.41, 148.37, 138.22, 137.10, 135.74, 132.04, 126.85, 124.04, 117.60, 91.36, 81.90, 51.12, 28.11, 25.32, 22.31, 17.47; HRMS (EI) m/z 420.2290 M+, calc. for C23H28N6O2 420.2274; anal. calc. for: C23H28N6O2 (420.51): C, 65.69; H, 6.71; N, 19.99%; found: C, 65.91; H, 6.77; N, 19.86%.
2-(1-(4-(3-(4-(3-Hydroxy-3-methylbut-1-yn-1-yl)phenyl)ureido) phenyl)ethylidene)hydrazine-1-carboximidamide 15.
Yellow solid (100 mg, 85.7%); mp 219–221 °C. 1H NMR (DMSO-d6); δ 9.17 (brs, 1H), 8.99 (brs, 1H), 7.93 (d, J = 8.6 Hz, 2H), 7.6 (d, J = 8.6 Hz, 2H), 7.49 (d, J = 8.4 Hz, 2H), 7.33 (d, J = 8.4 Hz, 2H), 5.72 (brs, 2H), 5.52 (brs, 2H), 3.63 (brs, 1H), 2.23 (s, 3H), 1.51 (s, 6H); 13C NMR (DMSO-d6); δ 160.36, 155.73, 152.21, 148.32, 138.12, 135.88, 132.22, 126.89, 126.89, 124.04, 117.76, 83.60, 70.29, 59.29, 29.15, 25.32; HRMS (EI) m/z 392.1957 M+, calc. for C21H24N6O2 392.1961; anal. calc. for: C21H24N6O2 (392.46): C, 64.27; H, 6.16; N, 21.41%; found: C, 64.51; H, 6.37; N, 20.22%.
2-(1-(4-(3-(4-((1-Hydroxycyclohexyl)ethynyl)phenyl)ureido)phenyl) ethylidene)hydrazine-1-carboximidamide 16.
Brown solid (85 mg, 73.9%); mp 223–224 °C. 1H NMR (DMSO-d6); δ 9.46 (brs, 1H), 9.17 (brs, 1H), 7.93 (d, J = 8.6 Hz, 2H), 7.60 (d, J = 8.7 Hz, 2H), 7.49 (d, J = 8.4 Hz, 2H), 7.33 (d, J = 8.4 Hz, 2H), 6.23 (brs, 1H), 6.01 (brs, 4H), 2.26 (s, 3H), 2.18–1.15 (m, 10H); 13C NMR (DMSO-d6); δ 159.80, 155.74, 152.58, 149.00, 137.20, 136.12, 132.23, 131.93, 126.93, 117.66, 93.05, 87.29, 58.38, 40.40, 29.23 25.75, 21.47, 17.68; HRMS (EI) m/z 432.2261 M+, calc. for C24H28N6O2 432.2274; anal. calc. for: C24H28N6O2 (416.52): C, 66.65; H, 6.53; N, 19.43%; found: C, 67.71; H, 6.67; N, 19.56%.
2-(1-(4-(3-(4-((1-Aminocyclohexyl)ethynyl)phenyl)ureido)phenyl) ethylidene)hydrazine-1-carboximidamide 17.
Dark brown solid (77 mg, 67%); mp 208–210 °C. 1H NMR (DMSO-d6); δ 9.47 (brs, 1H), 9.12 (brs, 1H), 8.14 (d, J = 8.2 Hz, 2H), 7.83 (d, J = 8.2 Hz, 2H), 7.55 (d, J = 8.4 Hz, 2H), 7.29 (d, J = 8.4 Hz, 2H), 6.23 (brs, 2H), 6.03 (brs, 2H), 2.26 (s, 3H), 2.18–1.14 (m, 10H); 13C NMR (DMSO-d6); δ 159.80, 155.74, 152.58, 149.00, 137.20, 136.12, 132.23, 131.93, 126.93, 123.96, 117.66, 93.05, 87.29, 58.38, 40.40, 29.23, 25.75, 24.73, 21.47, 17.68; HRMS (EI) m/z 431.2428 M+, calc. for C24H29N7O 431.2434; anal. calc. for: C24H29N7O (431.54): C, 66.80; H, 6.77; N, 22.72%; found: C, 66.71; H, 6.68; N, 22.57%.
2-(1-(4-(3-(4-(Phenylethynyl)phenyl)ureido)phenyl)ethylidene) hydrazine-1-carboximidamide 18.
Light brown solid (95 mg, 82%); mp 228–230 °C. 1H NMR (DMSO-d6); δ 9.11 (brs, 1H), 9.00 (brs, 1H), 8.21 (d, J = 7.6 Hz, 2H), 7.83–7.78 (m, 5H), 7.73 (d, J = 8.2 Hz, 2H), 7.53 (d, J = 8.4 Hz, 2H), 7.40 (d, J = 8.2 Hz, 2H), 5.78 (brs, 2H), 5.60 (brs, 2H), 2.26 (s, 3H); 13C NMR (DMSO-d6); δ 160.33, 155.79, 152.25, 148.44, 139.02, 137.13, 135.85, 132.22, 131.88, 129.27, 127.01, 122.92, 117.77, 91.01, 89.84, 25.34; HRMS (EI) m/z 410.1865 M+, calc. for C24H22N6O 410.1855; anal. calc. for: C24H22N6O (410.48): C, 70.23; H, 5.40; N, 20.47%; found: C, 69.91; H, 5.89; N, 20.27%.
2-(1-(4-(3-(4-(3-Hydroxy-3-phenylprop-1-yn-1-yl)phenyl)ureido) phenyl)ethylidene)hydrazine-1-carboximidamide 19.
Dark brown solid (65 mg, 56.7%); mp 165–167 °C; 1H NMR (DMSO-d6); δ 9.18 (brs, 1H), 8.97 (brs, 1H), 8.57 (d, J = 8.1 Hz, 2H), 8.41 (d, J = 8.2 Hz, 2H), 8.08 (d, J = 8.4 Hz, 2H), 7.98 (d, J = 8.2 Hz, 2H), 7.43–6.97 (m, 5H), 5.78 (brs, 2H), 5.67 (brs, 2H), 5.41 (s,1H), 2.61 (s, 3H); 13C NMR (DMSO-d6); δ 160.31, 155.77, 152.22, 148.43, 139.45, 137.14, 137.14, 135.88, 132.32, 128.97, 127.74, 126.96, 122.42, 117.78, 89.73, 86.93, 63.90, 25.31; HRMS (EI) m/z 440.1968 M+, calc. for C25H24N6O2 400.1961; anal. calc. for: C25H24N6O2 (440.50): C, 68.17; H, 5.49; N, 19.08%; found: C, 68.91; H, 5.56; N, 18.87%.
3.2. Biology
3.2.1. Bacterial strains, media, cell lines and reagents.
Clinical isolates used in this study (Table S3†) were obtained from the Biodefense and Emerging Infections Research Resources Repository (BEI Resources) and the American Type Culture Collection (ATCC). Cation-adjusted Mueller Hinton broth (CAMHB), tryptic soy broth (TSB), tryptic soy agar (TSA) and brain heart infusion (BHI) broth were purchased from Becton, Dickinson and Company (Cockeysville, MD, USA). Human keratinocyte cell line (HaCaT) was obtained from AddexBio (San Diego, CA, USA), and monkey kidney epithelial cells (Vero) was purchased from ATCC (Manassas, VA, USA). Dulbecco's Modified Eagle Medium (DMEM) was obtained from Sigma-Aldrich (St. Louis, MO, USA). Fetal bovine serum (FBS) and phosphate-buffered saline (PBS) were purchased from Corning (Manassas, VA, USA). Linezolid and vancomycin were purchased from Chem-Impex International (Wood Dale, IL, USA). Compounds were synthesized from commercial sources in our laboratory and prepared in stock solutions in DMSO.
3.2.2. MICs of diphenylurea compounds against S. aureus clinical isolates.
MICs of the diphenylureas were determined against staphylococcal clinical isolates using the broth microdilution method as described previously.32,33 Briefly, a 0.5 McFarland standard solution from each strain was prepared and diluted in CAMHB (for staphylococci and E. coli) to reach a concentration of ∼5 × 105 CFU mL−1. C. difficile was diluted in BHI supplemented with hemin and vitamin K. Enterococci and L. monocytogenes were diluted in TSB, and streptococcal strains were diluted in CAMHB supplemented with 5% lysed horse blood. Serial dilutions of test agents were incubated with bacteria before recording the MICs.
3.2.3.
In vitro cytotoxicity analysis of the diphenylurea compounds against Vero and HaCaT cells.
Cytotoxicity assessment for the diphenylurea compounds was determined as previously described.34 Briefly, cells were incubated with the compounds (in triplicates) for 24 hours. DMSO was included as a control. MTS reagent (Promega, Madison, WI, USA) was added and incubated for four hours, and the OD490 was measured. A two-way ANOVA, with post hoc Dunnett's test (p < 0.05), was used for data analysis.
3.2.4. Hemolytic activity assay.
As a final tolerability assessment step for compound 18 before being tested in the animal model of infection, the hemolytic activity was estimated using the method described before.35 Briefly, rabbit erythrocytes were washed three times with phosphate buffered saline (PBS, pH 7.4). Then, the erythrocyte pellet was resuspended in PBS and mixed 1
:
1 with a 1 % triton X-100 solution in order to induce complete hemolysis. The erythrocyte suspension was adjusted to an absorbance of 0.8 arbitrary units at 540 nm. Three tested concentrations (16, 32 and 64 μg mL−1) of compound 18 were prepared and mixed with equal volume of the freshly prepared erythrocyte suspension to reach a final concentration of (16, 32 and 64 μg mL−1) and incubated at 37 °C for 30 min. Negative control was PBS mixed with equal volume of the erythrocyte blood suspension. Finally, the mixtures were centrifuged at 13
000 × g for 5 min and the absorbance of the supernatant was measured at 540 nm.36
3.2.5. Multi-step resistance testing.
Resistance development is one of the main hampering reasons to the usage of novel antimicrobials. Hence, testing the resistance development potential towards the organism of interest is pivotal to every newly synthesized compound. Moving along with this aim in mind, a multi-step resistance experiment was done, using MRSA USA300, as reported earlier.37,38 The broth microdilution method was used to determine the MIC of both compound 18, and rifampicin, as the control antibiotic, exposed to MRSA USA300 for 14 consecutive passages. Resistance was classified as a greater than four-fold increase in the initial MIC.39
3.2.6. Post-antibiotic effect (PAE) against MRSA USA300.
The PAE refers to a period of time after complete antibiotic removal during which there is no growth of the tested organism.40 It was determined using the viable count method as previously described.41,42 Briefly, MRSA USA300 culture was adjusted to an inoculum of ∼106 CFU mL−1 and then exposed to 5× MIC of compound 18 or vancomycin for 2 h. A culture containing DMSO was included as a negative control and viable count was determined after the two hours for all the included tests. Afterwards, compound 18, vancomycin, and DMSO were removed by diluting each tube 1
:
1000 in fresh TSB media, and viable counts were determined for all cultures at this time (time 0). The antibiotic-free bacterial suspensions were incubated at 37 °C with agitation at 180 RPM, and samples were collected hourly for 8 hours. Viable count was done at each time point on mannitol salt agar (MSA) plates, and the results were read after incubation for 18–24 h at 37 °C. The PAE was defined as follows: PAE = T − C, where T is the time (h) required for the viable count in the test culture to increase one log10 cycle above the count observed immediately after dilution, and C is the corresponding time for the control.43
3.2.7. Murine skin infection model.
3.2.7.1. Ethics statement.
Animal procedures were approved by the Research Ethics Committee of the Faculty of Pharmacy, Cairo University [approval no. (MI 2868)] following the Guide for the Care and Use of Laboratory Animals published by the Institute of Laboratory Animal Research (USA).
3.2.7.2. Murine skin infection model.
The skin infection was performed as previously described.44,45 Briefly, three groups (n = 5) of six to eight-week-old female Balb/c mice, 18–20 g each, were included in the experiment. On the day prior to the infection, their backs were shaven using an electric hair clipper. On the day of the infection, the mice were anesthetized using 2,2,2-tribromoethanol (25 μg mL−1) and injected subcutaneously with 100 μL containing 2 × 109 CFU MRSA USA300, suspended in 0.5% hydroxypropyl methylcellulose (HPMC) in sterile pyrogen-free saline. The mice were kept in their cages and given food and water ad libitum. Seventy-two hours post-infection (day 3), the infection site was treated with either i) petroleum jelly (PG), ii) petroleum jelly containing 2% compound 18, or iii) commercially available topical ointment containing 2% fusidic acid (FA). The three treatments were applied twice daily for another three consecutive days (days 4 to 6). Twenty-four hours after the last dose, mice were euthanized with an overdose of the anesthesia, and the lesions were photographed using a digital camera. A skin patch equivalent to ∼1.5 cm2, surrounding the lesion site, was excised from the back of each mouse. In addition, the mice were dissected, and their spleens were excised to assess the systemic dissemination of the infection. The skin patch was shredded with a sterile surgical blade and then homogenized in 1 mL pyrogen-free saline. While the spleen was homogenized in only 0.5 mL saline. The homogenates were then serially diluted and plated on MSA. The plates were incubated at 37 °C overnight and the colonies were counted. A statistical analysis was performed using GraphPad Prism (version 9.0) (GraphPad Software, Inc., USA), applying ordinary one-way ANOVA, followed by the Tukey's multiple comparison test.
Conclusions
The SAR of alkynyldiphenylurea as new antibacterial agents was further explored against a set of clinically important Gram-positive cells. The phenylacetylene-containing derivative 18 showed the most promising antibacterial profile. Compound 18 was 20-times better than the lead compound I in terms of MIC values. In addition, compound 18 was highly tolerable to mammalian cells, and more importantly, it was comparable with fusidic acid in lowering the bacterial counts in infected mice wounds and even better in limiting systemic dissemination.
Author contributions
H. T. N. and N. S. A. conducted the microbiological tests; M. M. E. and A. M. K. synthesized all compounds; M. M. E. was responsible for all chemical characterization and writing the experimental section. Supervision was the responsibility of A. S. A., M. N. S. and A. S. M.; all authors contributed in writing.
Conflicts of interest
There are no conflicts to declare.
Acknowledgements
This paper is based upon work supported by Science, Technology & Innovation Funding Authority (STDF) under grant number: 43883.
Notes and references
- M. I. Hutchings, A. W. Truman and B. Wilkinson, Curr. Opin. Microbiol., 2019, 51, 72–80 CrossRef CAS PubMed.
- M. A. Cooper and D. Shlaes, Nature, 2011, 472, 32–32 CrossRef CAS PubMed.
- D. Ghernaout and N. Elboughdiri, Appl. Eng., 2020, 4, 1–6 Search PubMed.
- F. Prestinaci, P. Pezzotti and A. Pantosti, Pathog. Global Health, 2015, 109, 309–318 CrossRef PubMed.
- C. Antimicrobial Resistance, Lancet, 2022, 399, 629–655 CrossRef PubMed.
- S. Y. Tong, J. S. Davis, E. Eichenberger, T. L. Holland and V. G. Fowler, Jr., Clin. Microbiol. Rev., 2015, 28, 603–661 CrossRef CAS PubMed.
- S. Santajit and N. Indrawattana, BioMed Res. Int., 2016, 2016, 2475067 Search PubMed.
- E. F. Kong, J. K. Johnson and M. A. Jabra-Rizk, PLoS Pathog., 2016, 12, e1005837 CrossRef PubMed.
-
Centers for Disease Control and Prevention, Antibiotic resistance threats in the United States, 2019, US Department of Health and Human Services, Centres for Disease Control and Prevention, 2019 Search PubMed.
- H. T. Nour El-Din, A. S. Yassin, Y. M. Ragab and A. M. Hashem, Infect. Drug Resist., 2021, 14, 1557–1571 CrossRef PubMed.
- A. Azhar, S. Rasool, A. Haque, S. Shan, M. Saeed, B. Ehsan and A. Haque, J. Med. Microbiol., 2017, 66, 1328–1331 CrossRef CAS PubMed.
- N. Desai, A. Maheta, K. Rajpara, V. Joshi, H. Vaghani and H. Satodiya, J. Saudi Chem. Soc., 2014, 18, 963–971 CrossRef.
- H. Mohammad, A. S. Mayhoub, A. Ghafoor, M. Soofi, R. A. Alajlouni, M. Cushman and M. N. Seleem, J. Med. Chem., 2014, 57, 1609–1615 CrossRef CAS PubMed.
- I. Eid, M. M. Elsebaei, H. Mohammad, M. Hagras, C. E. Peters, Y. A. Hegazy, B. Cooper, J. Pogliano, K. Pogliano, H. S. Abulkhair, M. N. Seleem and A. S. Mayhoub, Eur. J. Med. Chem., 2017, 139, 665–673 CrossRef CAS PubMed.
- M. M. Elsebaei, H. Mohammad, M. Abouf, N. S. Abutaleb, Y. A. Hegazy, A. Ghiaty, L. Chen, J. Zhang, S. R. Malwal, E. Oldfield, M. N. Seleem and A. S. Mayhoub, Eur. J. Med. Chem., 2018, 148, 195–209 CrossRef CAS PubMed.
- M. M. Elsebaei, H. Mohammad, A. Samir, N. S. Abutaleb, A. B. Norvil, A. R. Michie, M. M. Moustafa, H. Samy, H. Gowher, M. N. Seleem and A. S. Mayhoub, Eur. J. Med. Chem., 2019, 175, 49–62 CrossRef CAS PubMed.
- M. Hagras, N. S. Abutaleb, A. O. Ali, J. A. Abdel-Aleem, M. M. Elsebaei, M. N. Seleem and A. S. Mayhoub, ACS Infect. Dis., 2018, 4, 1679–1691 CrossRef CAS PubMed.
- M. Hagras, Y. A. Hegazy, A. H. Elkabbany, H. Mohammad, A. Ghiaty, T. M. Abdelghany, M. N. Seleem and A. S. Mayhoub, Eur. J. Med. Chem., 2018, 143, 1448–1456 CrossRef CAS PubMed.
- M. Hagras, H. Mohammad, M. S. Mandour, Y. A. Hegazy, A. Ghiaty, M. N. Seleem and A. S. Mayhoub, J. Med. Chem., 2017, 60, 4074–4085 CrossRef CAS PubMed.
- A. Hammad, N. S. Abutaleb, M. M. Elsebaei, A. B. Norvil, M. Alswah, A. O. Ali, J. A. Abdel-Aleem, A. Alattar, S. A. Bayoumi, H. Gowher, M. Seleem and A. S. Mayhoub, J. Med. Chem., 2019, 62, 7998–8010 CrossRef CAS PubMed.
- Y. Hosny, N. S. Abutaleb, M. Omara, M. Alhashimi, M. M. Elsebaei, H. S. Elzahabi, M. N. Seleem and A. S. Mayhoub, Eur. J. Med. Chem., 2020, 185, 111830 CrossRef CAS PubMed.
- M. M. Elsebaei, N. S. Abutaleb, A. A. Mahgoub, D. Li, M. Hagras, H. Mohammad, M. N. Seleem and A. S. Mayhoub, Eur. J. Med. Chem., 2019, 182, 111593 CrossRef PubMed.
- A. Mancy, N. S. Abutaleb, M. M. Elsebaei, A. Y. Saad, A. Kotb, A. O. Ali, J. A. Abdel-Aleem, H. Mohammad, M. N. Seleem and A. S. Mayhoub, Eur. J. Med. Chem., 2019, 6, 80–90 Search PubMed.
- M. Hagras, N. S. Abutaleb, N. M. Elhosseiny, T. M. Abdelghany, M. Omara, M. M. Elsebaei, M. Alhashimi, A. B. Norvil, M. I. Gutay, H. Gowher, M. Seleem and A. S. Mayhoub, ACS Infect. Dis., 2020, 6, 2887–2900 CrossRef CAS PubMed.
- I. H. Eissa, H. Mohammad, O. A. Qassem, W. Younis, T. M. Abdelghany, A. Elshafeey, M. M. Abd Rabo Moustafa, M. N. Seleem and A. S. Mayhoub, Eur. J. Med. Chem., 2017, 130, 73–85 CrossRef CAS PubMed.
- K. O. Olawale, S. O. Fadiora and S. S. Taiwo, Afr. J. Infect. Dis., 2011, 5, 40–46 Search PubMed.
- S. M. Jabbari Shiadeh, A. Pormohammad, A. Hashemi and P. Lak, Infect. Drug Resist., 2019, 12, 2713–2725 CrossRef PubMed.
- E. S. Shenoy, M. L. Paras, F. Noubary, R. P. Walensky and D. C. Hooper, BMC Infect. Dis., 2014, 14, 177 CrossRef PubMed.
- M. Otto, Nat. Rev. Microbiol., 2009, 7, 555–567 CrossRef CAS PubMed.
- O. Disson, A. Moura and M. Lecuit, Trends Microbiol., 2021, 29, 811–822 CrossRef CAS PubMed.
- H. Mohammad, W. Younis, H. G. Ezzat, C. E. Peters, A. AbdelKhalek, B. Cooper, K. Pogliano, J. Pogliano, A. S. Mayhoub and M. N. Seleem, PLoS One, 2017, 12, e0182821 CrossRef PubMed.
-
CLSI, Methods for Dilution Antimicrobial Susceptibility Tests for Bacteria That Grow Aerobically, Approved Standard, 2012 Search PubMed.
- I. G. Shahin, N. S. Abutaleb, M. Alhashimi, A. E. Kassab, K. O. Mohamed, A. T. Taher, M. N. Seleem and A. S. Mayhoub, Eur. J. Med. Chem., 2020, 202, 112497 CrossRef CAS PubMed.
- Y. Hosny, N. S. Abutaleb, M. Omara, M. Alhashimi, M. M. Elsebaei, H. S. Elzahabi, M. N. Seleem and A. S. Mayhoub, Eur. J. Med. Chem., 2020, 185, 111830 CrossRef CAS PubMed.
- N. H. Mahdally, R. F. George, M. T. Kashef, M. Al-Ghobashy, F. E. Murad and A. S. Attia, Front. Microbiol., 2021, 12, 700494 CrossRef PubMed.
- A. W. Bernheimer, Methods Enzymol., 1988, 165, 213–217 CAS.
- M. A. Tag ElDein, A. S. Yassin, O. El-Tayeb and M. T. Kashef, Eur. J. Clin. Microbiol. Infect. Dis., 2021, 40, 2349–2361 CrossRef CAS PubMed.
- H. Mohammad, W. Younis, H. G. Ezzat, C. E. Peters, A. AbdelKhalek, B. Cooper, K. Pogliano, J. Pogliano, A. S. Mayhoub and M. N. Seleem, PLoS One, 2017, 12, e0182821 CrossRef PubMed.
- D. J. Farrell, M. Robbins, W. Rhys-Williams and W. G. Love, Antimicrob. Agents Chemother., 2011, 55, 1177–1181 CrossRef CAS PubMed.
- G. G. Zhanel, D. J. Hoban and G. K. Harding, DICP, Ann. Pharmacother., 1991, 25, 153–163 CAS.
- P. Gaibani, D. Lombardo, R. E. Lewis, M. Mercuri, S. Bonora, M. P. Landini and S. Ambretti, J. Antimicrob. Chemother., 2014, 69, 1856–1865 CrossRef CAS PubMed.
- M. M. Elsebaie, H. T. Nour El-Din, N. S. Abutaleb, A. A. Abuelkhir, H.-W. Liang, A. S. Attia, M. N. Seleem and A. S. Mayhoub, Eur. J. Med. Chem., 2022, 114204, DOI:10.1016/j.ejmech.2022.114204.
- J. P. Lavigne, R. Bonnet, S. Michaux-Charachon, J. Jourdan, J. Caillon and A. Sotto, J. Antimicrob. Chemother., 2004, 53, 616–619 CrossRef CAS PubMed.
- M. Hagras, N. S. Abutaleb, N. M. Elhosseiny, T. M. Abdelghany, M. Omara, M. M. Elsebaei, M. Alhashimi, A. B. Norvil, M. I. Gutay, H. Gowher, M. Seleem and A. S. Mayhoub, ACS Infect. Dis., 2020, 6, 2887–2900 CrossRef CAS PubMed.
- C. W. Tseng, M. Sanchez-Martinez, A. Arruda and G. Y. Liu, J. Visualized Exp., 2011, e2528 Search PubMed.
|
This journal is © The Royal Society of Chemistry 2023 |
Click here to see how this site uses Cookies. View our privacy policy here.