DOI:
10.1039/D2MD00439A
(Research Article)
RSC Med. Chem., 2023,
14, 745-756
Inhibition and eradication of Pseudomonas aeruginosa biofilms by secondary metabolites of Nocardiopsis lucentensis EMB25†
Received
14th December 2022
, Accepted 2nd March 2023
First published on 7th March 2023
Abstract
Millions of people worldwide have been impacted by biofilm-associated disorders, which are impregnable owing to frequent changes in surface antigens and gene expression. Globally, about 11% of nosocomial infections, including cystic fibrosis, chronic wound infections, and post-surgical infections, are caused by Pseudomonas aeruginosa, the most prevalent Gram-negative bacterial species. Moreover, biofilms are highly resistant to the host's immune system, and exhibit increased tolerance to stress factors such as starvation, dehydration, and antimicrobials. Here, we have isolated a rare halophilic actinobacteria, Nocardiopsis lucentensis EMB25, and utilized the secondary metabolites for inhibition and eradication of P. aeruginosa biofilm. For the first time, N. lucentensis EMB25 bacteria was explored to study the anti-effect of secondary metabolites on pre-established biofilm. The secondary metabolites targeted the quorum sensing pathway and were found to bind to LasR and RhlR, as confirmed via molecular docking. Also, the reduction in virulence factors, rhamnolipids and pyocyanin further supported the study as these two are regulated by LasR and RhlR. In addition, the downregulation of various QS system genes lasA, lasB, rhlA, rhlB, and pqsA confirmed that the secondary metabolites act on two main regulators of the quorum sensing pathway, LasR, and RhlR. The findings of this study support the bioprospecting of previously unknown and extreme-condition actinobacteria as a rich source of novel bioactives against infections caused by bacterial biofilms.
1. Introduction
In nature, biofilms are believed to be the most prevalent form of bacteria accounting for at least two-thirds of all human infections, and merely 0.1% (ref. 1) of the total microbial biomass on Earth is considered to exist in the planktonic state.2 Biofilms are surface-associated microbial communities of bacteria residing in a matrix called extracellular polymeric substance (EPS), mainly consisting of polysaccharides, proteins, and extracellular DNA (eDNA).3 In the early stages of biofilm development, bacteria attach to surfaces and form microcolonies. Subsequently, these colonies develop into mature biofilms depending on numerous factors, including the density of the bacterial cells and the availability of nutrients.4 Biofilm development is considered a vital contributing factor to the exponentially-growing antimicrobial resistance (AMR) among all the other factors. Almost all available antibiotics act on metabolically active bacteria. However, bacteria residing deep in the biofilm are metabolically inactive due to the low oxygen levels, conferring up to 1000-fold resistance to antibiotics.5 Also, the thick layer of EPS causes reduced penetration and quenching of antibiotics due to negatively charged eDNA. Additionally, the transfer of resistant genes to susceptible bacteria occurs within the biofilms.4
Biofilm formation is well-understood in P. aeruginosa, a Gram-negative opportunistic and nosocomial pathogen that affects immuno-compromised patients including those suffering from cystic fibrosis.6P. aeruginosa is often found in diverse natural and man-made environments due to its metabolic versatility, such as natural water bodies, soil, skin, and a variety of medical devices.7 Infections caused by P. aeruginosa pose difficulties due to its intrinsic and acquired resistance, often leading to multi-drug resistance (MDR) or extensively drug-resistant (XDR) strains. In addition, tolerance to antibiotics by P. aeruginosa is further enhanced by biofilm formation, which acts as a protective layer against antibiotics and hosts immune response. P. aeruginosa has thus become a model organism for biofilm research due to its clinical relevance and relative ease of biofilm formation.3
P. aeruginosa strains synthesize primarily three types of exopolysaccharides, Pel, Psl, and alginate, which play a critical role in surface attachment, biofilm formation, and maintaining the biofilm architecture.8,9 The cell–cell communication within bacterial communities is mediated through a quorum sensing (QS) network, which enables bacteria to collectively control behaviour by monitoring the levels of small signal molecules known as autoinducers (AI).10 The three interconnected QS systems involved in biofilm formation in P. aeruginosa are las, rhl and pqs, producing and recognizing their cognate AI molecules: N-3-oxo-dodecanoyl-ACP (ACP-acyl carrier protein), N-butyryl-ACP, and 2-heptyl-3-hydroxy-4-quinolone (PQS) respectively.11 The three QS systems are involved in the production of diverse virulence factors such as pyocyanin, hydrogen cyanide, polysaccharides, lectins, rhamnolipids, pyoverdine, and factors necessary for immune evasion. Moreover, QS plays an essential role in modulating biofilm formation and biofilm-mediated resistance to antibiotics.12,13
P. aeruginosa uses LasI and RhlI to synthesize the acyl-homoserine lactone (AHL) signaling molecules and LuxR-type regulatory proteins LasR and RhlR respectively to recognize them. These two regulatory proteins contain both, a DNA binding domains and a ligand-binding domains and control the expression of various downstream genes.14 LasR with bound 3OC12HL acts upon lasA, and lasB genes which upon activation produces exoprotease and elastase respectively.15 RhlR in complex with C4-HSL acts on pyocyanin syntheses and rhlABR where rhlAB encodes rhamosyl transferase, and rhlB regulates the rhamnolipids synthesis.16 The PQS system regulates the synthesis of pyocyanin, rhamnolipids, PA-IL lectin, and elastase.17,18 Rhamnolipids assist in maintaining the biofilm channels, form microcolonies, facilitate the 3-D mushroom-shaped structure, and aid biofilm dispersal.19,20 Pyocyanin is a phenazine molecule whose production is controlled by PQS secondary molecule that regulates the expression of phzA-G operons. Pyocyanin being a redox-active pigment promotes virulence by interfering with the cellular pathways such as respiration, energy metabolism, electron transfer and innate immune mechanisms.21,22 Hence, pyocyanin has been recognized as a critical virulent factor. It is worth emphasizing that the QS system regulates several biological processes important to P. aeruginosa infection, including motility, the development of virulence factors, and biofilm formation.19 Indeed, inhibiting the QS system would impair the biofilm formation and reduce the virulence of P. aeruginosa. While both the synthases and regulatory proteins are exciting targets for inhibition, much of the research has focused on designing small-molecule antagonists that can inhibit the LasR and RhlR transcription factor.14,23
Many antibiotics and antimicrobials in the clinical investigation are based on their activity on the planktonic state of bacteria. As a result of the mismatch between the antimicrobial activity of current antibiotics and biofilms, recent studies show that targeting biofilm formation is a promising paradigm for overcoming resistance.3,24 Several approaches are being studied to develop antibiofilm drugs, such as structure-based designing of inhibitors against the particular target of choice, synthesis of desired drugs, high-throughput screening of chemical libraries, and mining natural products. However, from the perspective of discovering novel pharmacophores or novel classes of drugs, natural products are an obvious choice.25 Anti-QS molecules that occur naturally come from various plants, microorganisms, and higher organisms that inhabit terrestrial and marine ecosystems.26 The ability of microorganisms to produce diverse metabolites with diverse functions is particularly evident among actinobacteria. Specifically, actinomycetes originating from halophilic environments have yielded many new bioactive metabolites, revealing their potential for producing new bioactive chemical entities. In 2015, antibacterial activity of nocarbenzoxazoles A–G, from strain Nocardiopsis lucentensis DSM 44048, was reported but antibiofilm activity remain unexplored.27 In this work, we have isolated rare actinobacteria, Nocardiopsis lucentensis EMB25, that eradicated the mature biofilm and, to an extent, inhibited the biofilm formation of P. aeruginosa PseA. The organic extract used in the study was analyzed for secondary metabolites profiling and compounds were identified using the gas chromatography-mass spectrometry technique. After identification, the ligands present in the extract were docked on the LasR and RhlR. ADMET analysis of the selected metabolites shows that these can be pursued as potential drug candidates. Finally, the mechanism was elucidated through expression analysis of genes regulated by LasR and RhlR in presence and absence of organic extract. To the best of our knowledge, this is the first-time genus Nocardiopsis has been explored to inhibit and eradicate the biofilm of P. aeruginosa.
2. Materials and methods
2.1. Target bacterial strains and culture conditions
Pseudomonas aeruginosa PseA was isolated in our laboratory and used to study biofilms. The culture was revived in nutrient broth (HiMedia) media at 30 °C, 120 rpm. The culture of O.D600 0.2 (≈106 cells) was used as an inoculum throughout the biofilm studies in the present work.
2.2. Isolation of marine actinobacteria Nocardiopsis lucentensis EMB25 from environment samples
The samples were collected in sterile 50.0 mL falcon tubes from various locations of Pen, Maharashtra, India (18.7358° N, 73.0947° E), including salt pans, mangrove, creek, and dry soil at a depth of 10 m. The samples were transported under the cooling condition to the laboratory and stored at 4 °C until use. The method was followed as previously described,28 and diluted samples (100 μL) were spread onto various media, viz. M1 agar media,29 yeast malt extract (ISP-2) agar, ISP-4,30 starch casein agar, and actinomycetes isolation agar (HiMedia). The filter-sterilized antifungal (cycloheximide at 100 μg ml−1) and antibacterial (nalidixic acid at 25 μg ml−1) drug was added into the agar media to particularly select Gram-positive bacteria. The plates were incubated at 30 °C for 7 days. The actinobacteria-like colonies were selected and purified on actinomycetes isolation agar.
2.3. Quantification of biofilm formation
The crystal violet assay was used to quantify biofilm formation as described earlier with some modifications.31 Briefly, overnight cultures were diluted in tryptic soy broth (TSB) media to OD600 of 0.05 in flat-bottom polystyrene 96 well-plate. The plates were incubated statically at 30 °C for 24 h followed by removal of planktonic cells to quantify the adherent biofilm biomass. The plates were washed twice with 0.1 M phosphate buffer saline (PBS, pH 7.2) subsequently, heat fixation of the biofilm and staining with 0.3% (v/v) crystal violet dye was done for 15 min at room temperature. Afterwards the excess dye was removed, and the unbound dye was washed off with sterile water. The absorbance was taken at 590 nm after solubilization of the biofilm-bound dye with 33% (v/v) glacial acetic acid.
2.4. Screening and identification of isolated actinobacteria for biofilm eradication activity
The isolated actinobacteria were screened for the ability to eradicate 24 h mature biofilm of P. aeruginosa PseA. The cell-free supernatant of isolated 11 actinobacteria were diluted to 0.75%, 0.50%, 0.25%, and 0.125%, v/v and added on 24 h mature biofilm after removal of planktonic cells, followed by the quantification of biofilm as mentioned earlier.31 The biofilm-eradicating actinobacteria selected in the current study was identified by 16S rRNA sequencing. The sequence obtained was submitted to GenBank for accession number and the phylogenetic tree was constructed by the neighbor-joining method using MEGA software.
The ability to eradicate biofilms was further evaluated on 24 h, 48 h, and 72 h preformed biofilms of clinical pathogens procured from AIIMS, New Delhi: P. aeruginosa PseA, P. aeruginosa 25/34, P. aeruginosa 14/327, K. pneumoniae 26/366, K. pneumoniae 21/340, K. pneumoniae 16/348, E. coli 18/307, and E. coli 25/337.
2.5. Secondary metabolites extraction using organic solvent method
ISP-2 media (4 g L−1 yeast extract, 10 g L−1 malt extract, 4 g L−1 dextrose, pH 7.2) was used for the production of secondary metabolites. The seed culture was inoculated with a single colony of freshly revived actinobacteria and incubated at 30 °C, 120 rpm for 7 days. 1% inoculum was used to inoculate 12 L media for bulk production of secondary metabolites using the same condition as above. After 7 days of incubation, ethyl acetate was added to the cell-free supernatant in equal volume to extract the bioactive compounds. After overnight incubation, the organic layer was collected and dried using a rotary evaporator.26 This was done thrice to avoid any loss. The brownish colour semi-liquid was collected, weighed, and stored at 4 °C until further use.
2.6. Effect of organic extract on preformed biofilm and cell viability
The effect of Nocardiopsis lucentensis EMB25 extract on pre-existing biofilm of P. aeruginosa PseA was evaluated on 96 well-plates by incubating the bacteria at 30 °C for 48 h as per defined conditions mentioned earlier with the replenishment of media at every 24 h. Planktonic cells were removed by pipetting out gently, and wells were washed using 0.1 M PBS, pH 7.2. Extracts in various concentrations (25, 12.5, 6.25, 3.125, 1.56, 0.78, 0.39, 0.195, 0.098 μg μL−1) were added followed by incubation for another 24 h statically to check the effect. The crystal violet assay was performed to quantify the biofilm.
Simultaneously, XTT (sodium 3′-[1-(phenylaminocarbonyl)-3,4-tetrazolium]-bis(4-methoxy6-nitro) benzene sulfonic acid hydrate) assay was performed to check the metabolically active cells after 24 h incubation with extracts.33 After removing the planktonic cells and 0.1 M PBS, pH 7.2, XTT-menadione solution (XTT: 1 μg μL−1 and menadione: 0.4 mM mixed in 1
:
1 v/v) was added. Afterwards, plates were incubated statically at 30 °C for 5 h in dark and the absorbance was recorded at 490 nm.
2.7. Confocal laser scanning microscopic analysis (CLSM)
Biofilm eradicating effect of extract EMB25 against P. aeruginosa PseA biofilm was observed under a confocal microscope (Laser Scanning Confocal Microscope: LSM 880, Carl Zeiss). The biofilm formation was performed in 6 well-plate for 48 h at 30 °C with 18 mm coverslip added into the plate for visualization of biofilm. After following the biofilm eradication protocol, the adherent biofilm biomass was stained with the Filmtracer™ LIVE/DEAD™ Biofilm Viability Kit (Invitrogen, ThermoFisher Scientific) as per the manufacturer's protocol. This staining allows the distinguishing of live and dead cells after treatment using two dyes SYTO 9 and propidium iodide. SYTO 9 labels all bacterial cells, whereas propidium iodide stains only dead cells, causing a reduction in SYTO 9 staining in dead cells. The excess stain was removed, and the coverslips were mounted onto the glass slide. The slides were then observed under the confocal microscope.
2.8. Anti-biofilm activity and effect on the growth of planktonic cells
The activity of the extract was checked on the inhibition of biofilm using the CV assay. P. aeruginosa PseA was grown overnight in TSB media as an inoculum diluted to OD600 of 0.05 in the same media in flat-bottom polystyrene 96 well-plate and incubated statically with or without the varying concentration of Nocardiopsis lucentensis EMB25 extract (25, 12.5, 6.25, 3.125, 1.56, 0.78, 0.39, 0.195, 0.098 μg μL−1) at 30 °C for 24 h. The quantification of biofilm was done by crystal violet assay.
In addition, the effect of Nocardiopsis lucentensis EMB25 extract on the growth of P. aeruginosa PseA was evaluated in the presence of 50% (v/v) biofilm inhibitory concentration of extract and the highest concentration of 25 μg μL−1 up to 24 h at an interval of 4 h based on the absorbance at 600 nm.
2.9. Estimation of virulence factor production
The assays were performed at BIC50 and 25 μg μL−1 to estimate pyocyanin, rhamnolipid, and swarming motility in case of biofilm inhibition.
2.9.1 Pyocyanin assay.
Pyocyanin was extracted as described previously with some modifications.22,32,33P. aeruginosa PseA was grown in presence and absence of EMB25 extract for 24 h at 30 °C followed by extraction of cell-free supernatant. 3.0 mL chloroform was added to 5.0 mL cell-free supernatant, followed by vigorous vortexing for 2 min, and centrifugation for 10 min at 3000 × g and 4 °C. One mL of 0.2 N HCl was then added to partially extracted blue pyocyanin, which upon acidification turned into pink. The absorbance was taken at 520 nm. The assays were performed in triplicates.
2.9.2 Rhamnolipid production.
Rhamnolipid production was estimated using the orcinol-sulfuric acid method as described previously with some modifications.34,35 Briefly, rhamnolipids were extracted from cell-free supernatant (500 μL) using diethyl ether solvent. The samples were concentrated and to 100 μL, 900 μL orcinol solution was added containing 0.19% (w/v) orcinol in 53% H2SO4 followed by heating at 80 °C for 30 min. Then, samples were brought to room temperature for 15 min, and absorbance was measured at 421 nm. The concentration of rhamnolipids was evaluated using the standard graph of rhamnose from the range 0–50 μg mL−1.32
2.9.3 Swarming motility.
The assay was carried out as per the protocol mentioned previously.36 Briefly, swarm agar was prepared from 0.5% (w/v) agar, 0.5 g L−1 glucose, and 0.8 g L−1 nutrient broth. Additionally, the extract was added to the swarm agar media to the final concentration of 1.56 μg μL−1 and 25 μg μL−1 before pouring. The agar plates were dried for 40 min, and 3 μL of log-phase grown P. aeruginosa PseA was added at the centre of the plate and incubated for 24 h at 30 °C.
2.10. Field emission scanning electron microscopy (FESEM)
Anti-biofilm effect of EMB25 extract against P. aeruginosa PseA biofilm was observed under FESEM (FEI Company, Netherland, model: FEI Quanta 200F SEM). P. aeruginosa PseA biofilm was grown on 12 mm coverslip added into 24 well plate in the presence and absence of EMB25 extracts. After 48 h of biofilm formation, planktonic cells were removed and adhered biofilm was washed with 0.1 M PBS, pH 7.2 two times. Afterward, 3% (v/v) glutaraldehyde was added and incubated at 4 °C overnight to fix the biofilm. After incubation, the glutaraldehyde was removed and dehydration steps were followed. Biofilms were oven-dried for 15 min and mounted on aluminium stubs followed by gold coating for better visualization.
2.11. Gas chromatography-mass spectrometry analysis
The brownish semi-liquid was dissolved in ethyl acetate solvent, and 1.0 μL sample was injected into GC (GCMS-QP2010 Ultra) equipped with a flame thermionic detector (FTD) under split mode 1/10. The oven temperature program was as follows: the initial temperature was 100 °C (hold time: 2 min), increased to 250 °C at a rate of 6 °C min−1 (hold time: 2 min), and finally increased to 280 °C at a rate of 15 °C min−1 (hold time: 19 min). The ion source and interface temperatures were 220 °C and 270 °C, respectively. The measurements were carried out in SCAN mode with the mass range (m/z) from 40 to 650. The data obtained were compared with the standard library of mass spectra database NIST (National Institute of Standards and Technology).
2.12. Computational studies
Molecular docking of the 53 compounds identified in the extract was performed against the quorum sensing regulators LasR and RhlR. The 1.8 Å resolution crystal structure of N-terminus, ligand binding domain of LasR in complex with 3-oxo-C(12)-acylhomoserine lactone (PDB ID: 2UV0) from Pseudomonas aeruginosa was retrieved from PDB.37 Alphafold model of RhlR was retrieved from UniProt. Glide module of Schrodinger software was used for docking (Maestro v11.5.011).38 The 3D structure of LasR and RhlR was prepared by protein preparation wizard and OPSL3 force field. To determine the potential binding site for docking, the prepared proteins were used in SITEMAP program. Based upon the size, functionality and extent of solvent exposure of the protein, SITEMAP program provides site score ranking of the docking sites. Sites generated were analyzed using PYMOL to target the ligand binding pocket of the protein essential for their functioning. To identify the residues of LasR interacting with 3-oxo-C(12)-acylhomoserine lactone (PDB ID: 2UV0), the structure was analysed using CONTACT program of CCP4 suite and residues within 4 Å distance were used for grid preparation using receptor grid generation module. For RhlR, the site with best site score was used for grid generation. The grid boxes with dimension of 15 × 15 × 15 (x × y × z) Å were used further.39 Using these precalculated grid files, SP (standard precision) ligand docking was performed for both LasR and RhlR respectively. To analyze the results, ligands with lowest glide energy and lowest G score were evaluated. The drug likeness and pharmacokinetics of compounds were predicted using QikProp module of Schrodinger. This module provides the adsorption, distribution, metabolism and excretion prediction of the compounds. For the predictions, the compounds were prepared using ligPrep module using OPLS4 force field. Energy minimization and ionization states at specified pH 7 ± 2 were decided. The prepared ligands were subjected for ADME analysis predicting the various physiochemical, drug likeliness features of the compounds including number of violations of Lipinski's rule of five. The rules are: mol_MW < 500, QP log
Po/w < 5, donorHB ≤ 5, accptHB ≤ 10. It also predicted molecular weight of the compounds, predicted octanol/water partition coefficient (QP log
Po/w), determine aqueous solubility of compounds (QP log
S), Predicted brain/blood partition coefficient (QPlogBB), predicted apparent Caco-2 cell permeability in nm s−1 (QPPCaco), predicted IC50 value for blockage of HERG K+ channels (QPlogHERG) and predicted value of binding to human serum albumin (QP log
Khsa).40
2.13. Extraction of RNA and quantitative gene analysis of QS genes in P. aeruginosa
Total RNA of EMB25 culture was isolated from the stationary phase grown for 24 h in presence and absence of organic extract using RNeasy® Mini Kit (Qiagen) as per the protocol mentioned in the kit. The purity and concentration of total RNA isolated was checked on agarose gel electrophoresis and NanoDrop2000 spectrophotometer (Thermo Scientific, USA). The cDNA was prepared using the iScript™ cDNA synthesis kit with the condition mentioned in the protocol: annealing at 25 °C for 5 min, extension at 42 °C for 30 min, and inactivation at 85 °C for 5 min. Afterward, qRT-PCR was performed with the primers (Table S4†). The following conditions were set for carrying out the qRT-PCR: initial denaturation at 95 °C for 5 min, 39 cycles of 95 °C for 10 s, 52 °C for 30 s and 72 °C for 30 s (denaturation, annealing and extension) followed by 95 °C for 1 min and 60 °C for 1 min. For melt curve 65 °C to 95 °C, increment of 0.5 °C for 5 s. The rpoD gene was used as the housekeeping gene, and relative normalized expression was analyzed using the CFX Maestro software (CFX96 Touch Real-Time PCR detection system, BioRad).41
3. Results and discussion
3.1. Sample collection, identification and phylogenetic analysis
Microorganisms thriving in extreme conditions have immense potential for isolating novel drugs due to the evolution and adaptation in terms of metabolic biochemistry. In view of the biological importance as an untapped source of various secondary metabolites, halophilic actinobacteria was isolated. The salt pan in Pen, Maharashtra is rich in rare marine actinobacteria. During the time of sample collection, the recorded temperature was 39 °C and pH of the soil was 7.8. The place was explored to selectively isolate rare halophilic actinobacteria. The actinobacteria-like colonies were selected, purified and identified using 16S rRNA sequencing. The potential EMB25 16S rRNA sequence (1436 bp) was submitted to the GenBank database under the accession number MW582546.1. The evolutionary link was investigated using a similarity search against the NCBI 16s rRNA database. MEGA 7.0 software was used to build the phylogenetic tree using the neighbor-joining method. The results revealed that EMB25 is related to Nocardiopsis lucentensis and are clustered together in the phylogenetic tree (Fig. S1†). The strain has been henceforth called Nocardiopsis lucentensis EMB25.
3.2. Isolated rare actinobacteria could attenuate P. aeruginosa PseA biofilm
The isolated actinobacteria were screened for their potency for eradication of preformed biofilms of P. aeruginosa PseA. The isolates EMB24, EMB25, EMB26, EMB27, EMB28, EMB29, EMB30, EMB31, and EMB32 exhibited potent biofilm eradication activity measured at various concentration 0.75%, 0.50%, 0.25%, and 0.125% (v/v). However, the cell-free supernatant of EMB25 at 0.75% (v/v) eradicated >90% preformed biofilm of P. aeruginosa PseA (Fig. S2†).
The cell-free extract of Nocardiopsis lucentensis EMB25 at 0.75% (v/v) was further explored for biofilm eradication activity against various clinical isolates collected from AIIMS, New Delhi, India. The results showed that the cell-free extract could attenuate >90% preformed biofilm at every condition (Fig. S3A–C†). In view of the potential of cell-free extract, fermentation and extraction of secondary metabolites was done to investigate the compounds secreted out by Nocardiopsis lucentensis EMB25 using GC-MS/MS.
3.3. Eradication of preformed P. aeruginosa PseA biofilms by organic extract
Next, we evaluated the EMB25 organic extract (0.098–25 μg μL−1) for its eradication potential on preformed P. aeruginosa PseA biofilm. The EMB25 organic extract eradicated ≥50% preformed biofilm at concentration range of 3.125–25 μg μL−1 and >80% biofilm eradication was observed at 25 μg μL−1 (Fig. 1). Overall, the data showed dose-dependent increase in eradication potential of preformed biofilm of P. aeruginosa PseA. In parallel, the XTT assay was performed to check the viability of cells after eradication of biofilm in presence of extract. At the highest concentration of extract (25 μg μL−1), percentage of metabolically active cells observed was 40% (Fig. 1). The results of CV and XTT assays inferred that removal of thick polysaccharide layer and limitation of nutrients led to killing of persister cells.
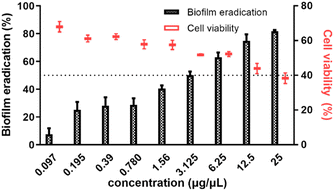 |
| Fig. 1 The effect of organic extract (25–0.097 μg μL−1) on preformed 48 h biofilm of P. aeruginosa PseA (shown in black bars) and cell viability percentage as estimated using XTT assay (shown in red symbols). | |
3.4. Disruption of the architecture of preformed biofilm by organic extract
Confocal laser scanning microscopy (CLSM) facilitates the evaluation of the architecture of biofilms. The effect of EMB25 organic extract at 3.125 μg μL−1 (BEC50) and 25 μg μL−1 on 48 h preformed biofilm of P. aeruginosa PseA was evaluated using confocal microscopy and Imaris 8.1 software. Treatment with 3.125 μg μL−1 revealed significant biofilm eradication that eventually led to death of persister cells. This is consistent with the results observed using XTT assay. In addition, treatment with 25 μg μL−1 led to >80% biofilm eradication compared to untreated control with compact and thick biofilm.
Also, the biomass of biofilm reduced in dose-dependent manner as shown in 3D projection images (Fig. 2A). The biofilm cell quantification using Imaris 8.1 software revealed bacteria cell death after treatment with EMB25 organic extract as compared to untreated control sample (Fig. 2B). These results establish that the EMB25 ethyl acetate extract led to reduced biomass and death of bacterial cells 24 h post treatment of P. aeruginosa PseA 48 h preformed biofilm.
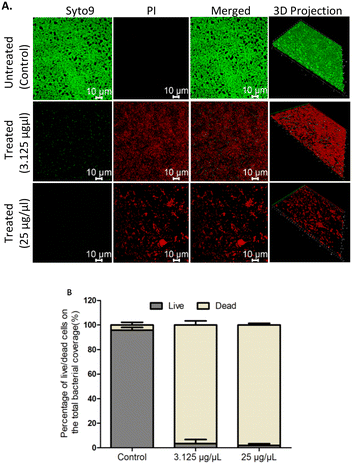 |
| Fig. 2 The effect of extract on eradication of 48 hours preformed biofilm of P. aeruginosa: (A) the CLSM images biofilm of P. aeruginosa strain untreated and after treatment for 24 hours onto glass coverslips at two different concentrations 3.125 μg μL−1 and 25 μg μL−1 in TSB media at 30 °C were evaluated. Bacterial biomass after eliminating planktonic cells were stained with the BacLight LIVE/DEAD viability kit in which live cells were labelled with Syto 9 dye, and dead cells are stained red with propidium iodide (scale bar, 10 μm) (B) the quantification of P. aeruginosa biofilms after treatment was performed by 3D imaging in Imaris 8.1 software. Data are mean from 3 independent experiments ± SD. | |
3.5. Prevention of biofilm formation and effect of organic extracts on quorum sensing mediated virulence factors
The effect of EMB25 ethyl acetate extract (0.098–25 μg μL−1) was analysed against inhibition of P. aeruginosa PseA biofilm. After 24 h incubation in absence (untreated control) and presence of extract (25–0.098 μg μL−1), CV assay was performed and significant biofilm inhibition was observed. At 1.56 μg μL−1, 50% biofilm inhibition was observed (BIC50), and >85% biofilm inhibition observed at 25 μg μL−1 (Fig. 3A). Furthermore, the time-dependent effect of EMB25 ethyl acetate extract on growth of planktonic cells was evaluated spectroscopically at concentration 1.56, 12.5 and 25 μg μL−1 (Fig. 3B). No effect on growth of planktonic bacteria was observed.
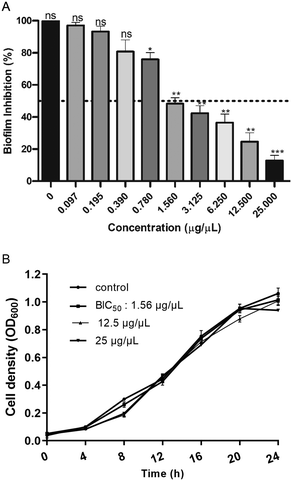 |
| Fig. 3 (A) Dose-dependent inhibition of Nocardiopsis lucentensis strain EMB25 organic extract on the formation of P. aeruginosa biofilm (B) growth curve of P. aeruginosa in presence of BIC50 (1.56 μg μL−1), 12.5 μg μL−1 and highest concentration (25 μg μL−1) used in the study (ns, non-significant, **p < 0.01; ****p < 0.0001). | |
In addition, the ethyl acetate fraction at 25 μg μL−1 concentration inhibited >85% biofilm formation of P. aeruginosa significantly higher than the inhibition (37.12%) reported earlier using Streptomyces parvulus HY026 spent medium and using endophytic Frankia sp. secondary metabolites.42,43
In continuation with the anti-biofilm study, rhamnolipid and pyocyanin, two dominant virulence factors, were quantified in presence (1.56 and 25 μg μL−1) and absence of EMB25 ethyl acetate extract. The rhamnolipid production decreased significantly in presence of 1.56 μg μL−1 concentration and four-fold reduction observed at 25 μg μL−1 (Fig. 4A). Also, pyocyanin production decreased by 68.5% at 25 μg μL−1 concentration as compared to the untreated control (Fig. 4B) suggesting that the extract is interfering with Las and Rhl QS systems of P. aeruginosa. Motility of P. aeruginosa is essential for invasion, thus the swarming motility of P. aeruginosa PseA was also evaluated in the presence and absence of the organic extract. The bacteria exhibited significant reduction (67.5%) in motility (Fig. 4C). It was concluded that the extract suppresses pyocyanin and rhamnolipid production, indicating a decrease in virulence of P. aeruginosa. The reduction in pyocyanin and rhamnolipid concentration in the presence of EMB25 extract further indicated that LasR and RhlR, regulators of the QS pathway, might be the targets of the metabolites present in the extract.
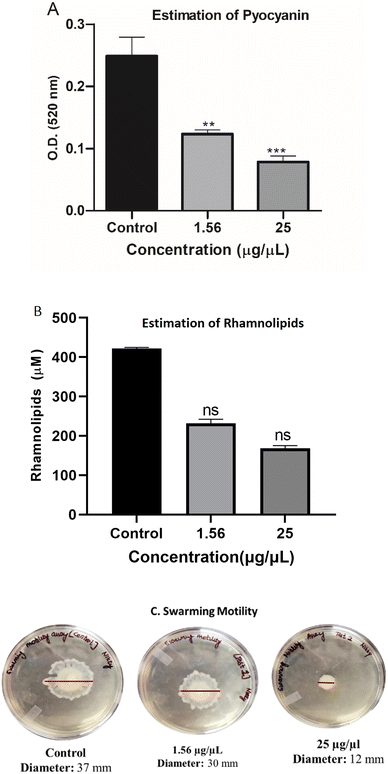 |
| Fig. 4 Estimation of virulence factor production at different organic extract concentration (1.56 μg μL−1 and 25 μg μL−1) (A) pyocyanin (B) rhamnolipid and (C) swarming motility assay. Data are mean from 3 independent experiments ± SD. Asterisks indicate the significant differences in comparison to untreated samples (**p < 0.01; ***p < 0.001; ****p < 0.0001). | |
3.6. Biofilm growth inhibition using FESEM
Scanning electron microscopy (SEM) was utilized to evaluate the morphological changes when biofilms were formed in presence of organic extract. The treated (1.56 and 25 μg μL−1) and untreated samples were observed after coating with the gold for better image visualization. Biofilm inhibition was seen at 1.56 μg μL−1 concentration and uniform distribution of non-adherent cells was observed. Complete inhibition was seen at 25 μg μL−1 concentration compared to untreated sample where thick and robust biofilm was formed (Fig. 5). The results are consistent with the biofilm inhibition assays quantified using crystal violet method.
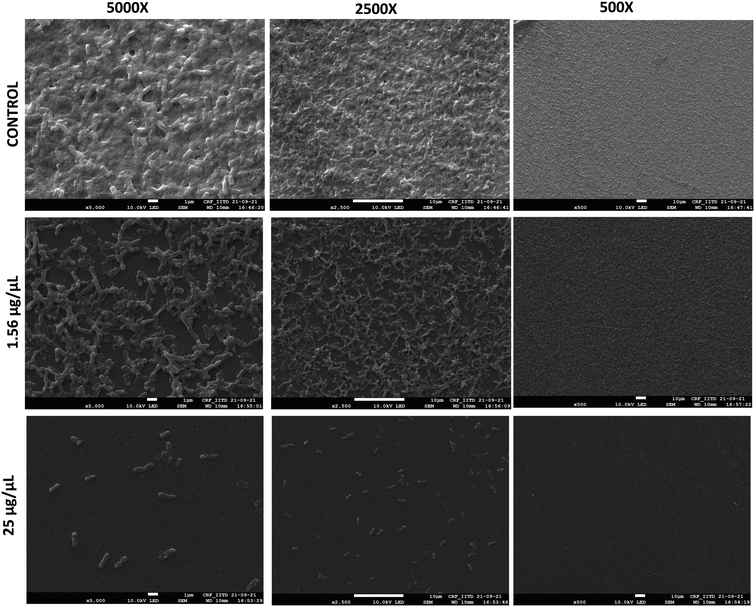 |
| Fig. 5 The effect of extract on biofilm inhibition of P. aeruginosa analyzed using FESEM at 5000X, 2500X and 500X magnification. The images were taken after treatment for 24 h onto glass coverslips at two different concentrations 1.56 μg μL−1 and 25 μg μL−1 in TSB media at 30 °C. | |
3.7. Compound identification and molecular docking with quorum sensing regulators
A total of 53 compounds were identified in ethyl acetate extract of EMB25 with the use of gas chromatography-mass spectrometry belonging to various classes including alkanes, alkanes, carboxylic acids, esters, ketones, phenols and terpenes (Table S1†). Since the secondary metabolites led to reduction in pyocyanin and rhamnolipid production, it was hypothesized that the target of the compounds could be the transcription factors LasR and RhlR that regulate the production of these virulence factors. To understand the mechanism of quorum sensing mediated inhibition of biofilm formation, molecular docking was performed on LasR and RhlR. Both the regulators belong to the LuxR family and contain two domains. An auto-inducer binding domain is present at the N-terminus and DNA binding domain is present at the C-terminus. The activity of these proteins is regulated by binding of auto-inducers at the N-terminus domain that results in their activation, consequently driving the expression of the target genes.14 Crystal structure of inducer binding domain of LasR bound to 3-oxo-C(12)-acylhomoserine lactone (PDB: 2UV0) and Alphafold model of ligand binding domain of RhlR was used for in silico docking. Sitemap program was used to find probable binding sites in LasR and RhlR. LasR structure showed one binding site with the score of 1.2, suitable for docking. This site overlaps with the binding site of the auto-inducer 3-oxo-C(12)-acylhomoserine lactone. Residues L36, L38, L40, Y47, A50, Y56, W60, R61, Y64, D65, A70, D73, T75, V76, C79, W88, F101, L110, T115, L125, G126, A127 and S129 were within 4 Å of the auto-inducer in the crystal structure were selected for receptor grid generation of LasR.
Similarly, sitemap analysis using Alphafold model of the autoinducer binding domain of RhlR gave four probable binding sites. Site 1 with a site score of 1.1 was selected for docking over other sites with scores of 0.8, 0.6 and 0.5. The residues spanning site 1 included A44, V60, Y64, W68, Y72, D81, A83, I84, W96, F101, L107, W108, A111, R112, T121, V133, L134 and Ser135 were used for grid generation. Crystal structure of full-length RhlR bound to N-butanoyl-L-homoserine lactone also became available very recently (PDB: 7R3H) which contains 75 mutations.44 Superimposition of the Alphafold model of the N-terminus domain on the crystal structure yielded rmsd of 1.2 Å indicating high structural similarity. Thus, Alphafold model was used for docking.
Molecular docking was carried out with the metabolites identified using GC-MS using the glide dock program. The top hits from the 53 compounds were ranked based on glide score, glide energy and docking score as shown in (Table 1). A total of 6 compounds each showed glide score better than −6.0 for LasR and RhlR and were selected for further analysis. 5 of them docked in both the target proteins. The superimpositions of the docked pose of the molecules and the auto-inducer on LasR and RhlR have been depicted in Fig. 6 and 7 respectively. Residues that interact with each of the top hits have been shown in (Table S2†). As can be seen, all the top ligands interact favorably with the target proteins and fit snugly into the active site utilizing similar residues as the autoinducers. They are thus capable if inhibiting the binding of the natural ligand, preventing activation of LasR and RhlR. We also performed in silico analysis of pharmacokinetics and drug-likeness of the selected metabolites using QikProp module of Schrodinger (Table S3†). The predicted ADMET (absorption, distribution, metabolism, excretion and toxicity) properties of selected compounds were present in the acceptable range. For these selected compounds, Lipinski's rule of five was also evaluated. It was found that the range of milog
P, number of hydrogen bond donor and acceptor and molecular weight of the selected molecules also falls in the acceptable range. Overall the docking study reveals that QS pathway is being targeted by the secondary metabolites present in the extract by binding to LasR and RhlR thereby inhibiting the production of pyocyanin and rhamnolipids.
Table 1 List of compounds with docking scores better than −6.0
S. no. |
Metabolites |
Docking score |
Glide score |
Glide energy |
Docking in LasR |
C30 |
Benzene, 1,1′-(1,2-cyclobutanediyl)bis-, trans- |
−7.44259 |
−7.44259 |
−52.9213 |
C15 |
Phenol, 3,5-bis(1,1-dimethylethyl)- |
−7.36119 |
−7.36159 |
−140.223 |
C12 |
Cyclododecane |
−7.35516 |
−7.35516 |
−41.4909 |
C58 |
Benzonitrile, m-phenethyl |
−6.68615 |
−6.68615 |
−100.043 |
C5 |
4,5-Heptadien-2-one, 3,3,6-trimethyl |
−6.51617 |
−6.51617 |
−106.888 |
C61 |
(2,3-Diphenylcyclopropyl)methyl phenyl sulfoxide, trans- |
−6.29516 |
−6.29516 |
−72.0301 |
Docking in RhlR |
C58 |
Benzonitrile, m-phenethyl |
−7.3542 |
−7.3542 |
−48.9187 |
C15 |
Phenol, 3,5-bis(1,1-dimethylethyl)- |
−7.2126 |
−7.213 |
−42.9753 |
C5 |
4,5-Heptadien-2-one, 3,3,6-trimethyl |
−6.77721 |
−6.77721 |
−35.3804 |
C30 |
Benzene, 1,1′-(1,2-cyclobutanediyl)bis-, trans- |
−6.67694 |
−6.67694 |
−31.7721 |
C12 |
Cyclododecane |
−6.40241 |
−6.40241 |
−20.3504 |
C10 |
Benzene, 1,1′-oxybis- |
−6.0042 |
−6.0042 |
−25.0439 |
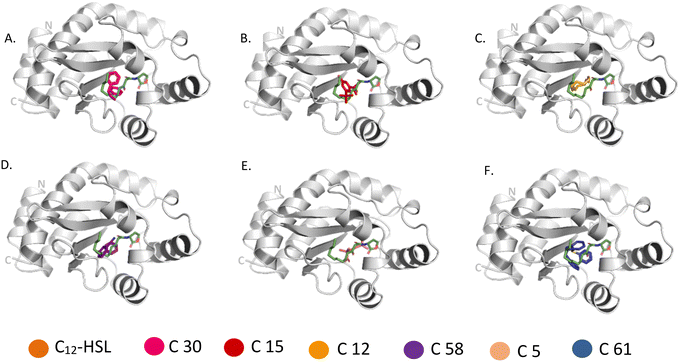 |
| Fig. 6 Comparison of binding of 3-oxo-C(12)-acylhomoserine lactone (PDB: 2UV0) with (A) C 30, (B) C 15, (C) C 12, (D) C 58, (E) C 5, (F) C 61 to LasR. Metabolites bound to the active site pocket of LasR are superimposed on autoinducer shown in green stick. LasR is shown as ribbon with its N- and C-terminus labelled and the compounds are represented in sticks of different colours. | |
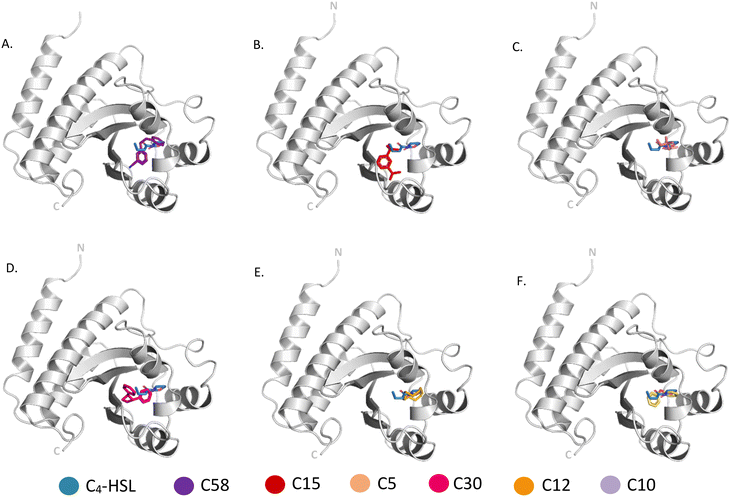 |
| Fig. 7 Comparison of binding of N-butanoyl-L-homoserine lactone with (A) C 58, (B) C 15, (C) C 5, (D) C 30, (E) C 12, (F) C 10 to Alphafold model of RhlR. Metabolites bound to the active site pocket of LasR are superimposed on auto-inducer shown in green stick. RhlR is shown as ribbon with its N- and C-terminus labelled and the compounds are represented in sticks of different colors. | |
3.8. Effect of ethyl acetate extract on quorum sensing associated genes
To further validate if the underlying mechanism of biofilm inhibition, is via inhibiting the regulators LasR and RhlR of the QS system, we measured the level of expression of genes in the presence and absence of ethyl acetate extract. The real-time analysis of the expression of downstream virulence genes, lasA, lasB, rhlA, rhlB, and pqsA were quantified differentially in presence and absence of BIC50 extract concentration (Fig. 8). LasR when bound to its cognate molecule regulates the expression of lasA, and lasB gene. In the presence of extract, significant reduction in the expression of genes were observed as compared to control (without treatment). The downregulation of rhlA, and rhlB gene expression justifies the decrease in rhamnolipids production as observed while estimating the virulence factors production. Similarly, expression of pqsA, the gene responsible for pyocyanin production, decreased in presence of extract.
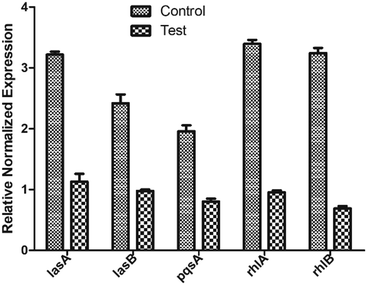 |
| Fig. 8 Relative gene expression of various QS genes involved in biofilm formation. Data is plotted as mean of three biological replicates. | |
The quantitative reduction in the concentrations and the downregulation of lasA, lasB, rhlA, rhlB, and pqsA also emphasize that the extract is acting on the two main regulators LasR and RhlR.
4. Conclusion
Resistance of microorganisms, particularly bacteria to antibiotics is a major health threat. In the current study, actinobacteria were isolated by using various physical and chemical pre-treatments. The extract of isolated rare actinobacteria acted upon the quorum sensing pathway which plays an important role in virulence and biofilm formation in P. aeruginosa. Reduction in pyocyanin and rhamnolipid formation indicated that LasR and RhlR pathways got affected in the presence of the metabolites. Docking of the identified secondary metabolites reveals that five of these are capable of binding to both the target proteins, LasR and RhlR with good energetics. ADMET analysis of the selected metabolites shows that these can be pursued as potential drug candidates. The real-time PCR study also complemented the in silico findings by measuring the gene regulation of QS system genes regulated by LasR and RhlR. To the best of our knowledge, this is the first-time genus Nocardiopsis has been explored to inhibit and eradicate the biofilm of P. aeruginosa. The limitation of the study is that the compounds could not be purified, and the results were not validated using in vivo models.
Data availability statement
The authors confirm that the data for this study findings are available within the article; ESI.†
Author contributions
Nikky Goel-conceptualization, methodology, visualization, data curation, investigation, validation, formal analysis, writing – original draft; Moumita Ghosh-writing – review & editing and computational studies; Deepti Jain: supervision, resources, writing – review & editing; Rajeshwari Sinha: review & editing; Sunil K. Khare-supervision, resources, writing – review & editing. All authors have given approval to the final version of the manuscript.
Conflicts of interest
The authors declare no competing financial interest.
Acknowledgements
NG gratefully appreciates the financial assistance provided by the Ministry of Human Resource Development (MHRD), Govt. of India and IIT Delhi. DJ acknowledges core funding received from Regional Centre for Biotechnology. DJ thanks Rajan Singh for help with confocal microscopy at the Advanced Technology Platform Centre of RCB. MG thanks Department of Science and Technology for INSPIRE fellowship.
Notes and references
- J. W. Costerton, Z. Lewandowski, D. E. Caldwell, D. R. Korber and H. M. Lappin-Scott, Microbial Biofilms, Annu. Rev. Microbiol., 1995, 49(1), 711–745 CrossRef CAS PubMed
.
- T. Bjarnsholt, O. Ciofu, S. Molin, M. Givskov and N. Høiby, Applying Insights from Biofilm Biology to Drug Development — Can a New Approach Be Developed?, Nat. Rev. Drug Discovery, 2013, 12(10), 791–808 CrossRef CAS PubMed
.
- H. Chen, R. W. Wubbolts, H. P. Haagsman and E. J. A. Veldhuizen, Inhibition and Eradication of Pseudomonas Aeruginosa Biofilms by Host Defence Peptides, Sci. Rep., 2018, 8(1), 10446 CrossRef PubMed
.
- N. Goel, S. W. Fatima, S. Kumar, R. Sinha and S. K. Khare, Antimicrobial Resistance in Biofilms: Exploring Marine Actinobacteria as a Potential Source of Antibiotics and Biofilm Inhibitors, Biotechnol. Rep., 2021, 30, e00613 CrossRef CAS PubMed
.
- N. Høiby, T. Bjarnsholt, M. Givskov, S. Molin and O. Ciofu, Antibiotic Resistance of Bacterial Biofilms, Int. J. Antimicrob. Agents, 2010, 35(4), 322–332 CrossRef PubMed
.
- N. M. Maurice, B. Bedi and R. T. Sadikot, Pseudomonas Aeruginosa Biofilms: Host Response and Clinical Implications in Lung Infections, Am. J. Respir. Cell Mol. Biol., 2018, 58(4), 428–439 CrossRef CAS PubMed
.
- H.-S. Kim, S.-H. Lee, Y. Byun and H.-D. Park, 6-Gingerol Reduces Pseudomonas Aeruginosa Biofilm Formation and Virulence via Quorum Sensing Inhibition, Sci. Rep., 2015, 5(1), 8656 CrossRef CAS PubMed
.
- M. T. T. Thi, D. Wibowo and B. H. A. Rehm, Pseudomonas Aeruginosa Biofilms, Int. J. Mol. Sci., 2020, 21(22), 8671 CrossRef CAS PubMed
.
- P. Banerjee, P. K. Sahoo, Sheenu, A. Adhikary, R. Ruhal and D. Jain, Molecular and Structural Facets of C-Di-GMP Signalling Associated with Biofilm Formation in Pseudomonas Aeruginosa, Mol. Aspects Med., 2021, 81, 101001 CrossRef CAS PubMed
.
- C. L. Wysoczynski-Horita, M. E. Boursier, R. Hill, K. Hansen, H. E. Blackwell and M. E. A. Churchill, Mechanism of Agonism and Antagonism of the Pseudomonas Aeruginosa Quorum Sensing Regulator QscR with Non-Native Ligands: Mechanism of Agonism and Antagonism of QscR, Mol. Microbiol., 2018, 108(3), 240–257 CrossRef CAS PubMed
.
- B. Kim, J.-S. ParK, H.-Y. Choi, J.-H. Kwak and W.-G. Kim, Differential Effects of Alkyl Gallates on Quorum Sensing in Pseudomonas Aeruginosa, Sci. Rep., 2019, 9(1), 7741 CrossRef PubMed
.
- M. Duplantier, E. Lohou and P. Sonnet, Quorum Sensing Inhibitors to Quench P. aeruginosa Pathogenicity, Pharmaceuticals, 2021, 14(12), 1262 CrossRef CAS PubMed
.
- F. Soukarieh, R. Liu, M. Romero, S. N. Roberston, W. Lucanto, S. Richardson, E. V. Oton, N. R. Qudus, A. Mashabi, S. Grossman, S. Ali, T. Sou, I. Kukavica-Ibrulj, R. C. Levesque, C. A. S. Bergström, N. Halliday, S. N. Mistry, J. Emsley, S. Heeb, P. Williams, M. Cámara and M. J. Stocks, Hit Identification of New Potent PqsR Antagonists as Inhibitors of Quorum Sensing in Planktonic and Biofilm Grown Pseudomonas Aeruginosa, Front. Chem., 2020, 8, 204 CrossRef CAS PubMed
.
- E. G. Suneby, L. R. Herndon and T. L. Schneider, Pseudomonas Aeruginosa LasR·DNA Binding Is Directly Inhibited by Quorum Sensing Antagonists, ACS Infect. Dis., 2017, 3(3), 183–189 CrossRef CAS PubMed
.
- M. J. Gambello, S. Kaye and B. H. Iglewski, LasR of Pseudomonas Aeruginosa Is a Transcriptional Activator of the Alkaline Protease Gene (Apr) and an Enhancer of Exotoxin A Expression, Infect. Immun., 1993, 61(4), 1180–1184 CrossRef CAS PubMed
.
- J. P. Pearson, L. Passador, B. H. Iglewski and E. P. Greenberg, Second N-Acylhomoserine Lactone Signal Produced by Pseudomonas Aeruginosa, Proc. Natl. Acad. Sci. U. S. A., 1995, 92(5), 1490–1494 CrossRef CAS PubMed
.
- U. A. Ochsner and J. Reiser, Autoinducer-Mediated Regulation of Rhamnolipid Biosurfactant Synthesis in Pseudomonas Aeruginosa, Proc. Natl. Acad. Sci. U. S. A., 1995, 92(14), 6424–6428 CrossRef CAS PubMed
.
- S. Yan and G. Wu, Can Biofilm Be Reversed Through Quorum Sensing in Pseudomonas Aeruginosa?, Front. Microbiol., 2019, 10, 1582 CrossRef PubMed
.
- A. Vetrivel, M. Ramasamy, P. Vetrivel, S. Natchimuthu, S. Kim, G.-S. Arunachalam and R. Murugesan, Pseudomonas Aeruginosa Biofilm Formation and Its Control, Biologics, 2021, 1(3), 312–336 CrossRef
.
- T. L. Wood, T. Gong, L. Zhu, J. Miller, D. S. Miller, B. Yin and T. K. Wood, Rhamnolipids from Pseudomonas Aeruginosa Disperse the Biofilms of Sulfate-Reducing Bacteria, npj Biofilms Microbiomes, 2018, 4(1), 22 CrossRef PubMed
.
- T. Das, S. Sabir, R. Chen, J. Farrell, F. H. Kriel, G. S. Whiteley, T. O. Glasbey, J. Manos, M. D. P. Willcox and N. Kumar, Halogenated Dihydropyrrol-2-One Molecules Inhibit Pyocyanin Biosynthesis by Blocking the Pseudomonas Quinolone Signaling System, Molecules, 2022, 27(4), 1169 CrossRef CAS PubMed
.
- A. V. Thees, K. M. Pietrosimone, C. K. Melchiorre, J. N. Marden, J. Graf, M. A. Lynes and M. Maltz-Matyschsyk, PmtA Regulates Pyocyanin Expression and Biofilm Formation in Pseudomonas Aeruginosa, Front. Microbiol., 2021, 12, 789765 CrossRef PubMed
.
- S. Hernando-Amado, M. Alcalde-Rico, T. Gil-Gil, J. R. Valverde and J. L. Martínez, Naringenin Inhibition of the Pseudomonas Aeruginosa Quorum Sensing Response Is Based on Its Time-Dependent Competition With N-(3-Oxo-Dodecanoyl)-L-Homoserine Lactone for LasR Binding, Front. Mol. Biosci., 2020, 7, 25 CrossRef CAS PubMed
.
- R. Sommer, K. Rox, S. Wagner, D. Hauck, S. S. Henrikus, S. Newsad, T. Arnold, T. Ryckmans, M. Brönstrup, A. Imberty, A. Varrot, R. W. Hartmann and A. Titz, Anti-Biofilm Agents against Pseudomonas aeruginosa : A Structure–Activity Relationship Study of C -Glycosidic LecB Inhibitors, J. Med. Chem., 2019, 62(20), 9201–9216 CrossRef CAS PubMed
.
- R. Singh and A. K. Dubey, Isolation and Characterization of a New Endophytic Actinobacterium Streptomyces Californicus Strain ADR1 as a Promising Source of Anti-Bacterial, Anti-Biofilm and Antioxidant Metabolites, Microorganisms, 2020, 8(6), E929 CrossRef PubMed
.
- H. B. Sarveswari, S. Kalimuthu, K. Shanmugam, P. Neelakantan and A. P. Solomon, Exploration of Anti-Infectives From Mangrove-Derived Micromonospora Sp. RMA46 to Combat Vibrio Cholerae Pathogenesis, Front. Microbiol., 2020, 11, 1393 CrossRef PubMed
.
- M. Sun, X. Zhang, H. Hao, W. Li and C. Lu, Nocarbenzoxazoles A–G, Benzoxazoles Produced by Halophilic Nocardiopsis Lucentensis DSM 44048, J. Nat. Prod., 2015, 78(8), 2123–2127 CrossRef CAS PubMed
.
- M. A. Pisano, M. J. Sommer and M. M. Lopez, Application of Pretreatments for the Isolation of Bioactive Actinomycetes from Marine Sediments, Appl. Microbiol. Biotechnol., 1986, 25(3), 285–288 CrossRef
.
- T. J. Mincer, P. R. Jensen, C. A. Kauffman and W. Fenical, Widespread and Persistent Populations of a Major New Marine Actinomycete Taxon in Ocean Sediments, Appl. Environ. Microbiol., 2002, 68(10), 5005–5011 CrossRef CAS PubMed
.
- E. B. Shirling and D. Gottlieb, Methods for Characterization of Streptomyces Species, Int. J. Syst. Bacteriol., 1966, 16(3), 313–340 CrossRef
.
- S. Balasubramanian, E. M. Othman, D. Kampik, H. Stopper, U. Hentschel, W. Ziebuhr, T. A. Oelschlaeger and U. R. Abdelmohsen, Marine Sponge-Derived Streptomyces Sp. SBT343 Extract Inhibits Staphylococcal Biofilm
Formation, Front. Microbiol., 2017, 8, 236 Search PubMed
.
- M. Chatterjee, S. D'Morris, V. Paul, S. Warrier, A. K. Vasudevan, M. Vanuopadath, S. S. Nair, B. Paul-Prasanth, C. G. Mohan and R. Biswas, Mechanistic Understanding of Phenyllactic Acid Mediated Inhibition of Quorum Sensing and Biofilm Development in Pseudomonas Aeruginosa, Appl. Microbiol. Biotechnol., 2017, 101(22), 8223–8236 CrossRef CAS PubMed
.
- D. W. Essar, L. Eberly, A. Hadero and I. P. Crawford, Identification and Characterization of Genes for a Second Anthranilate Synthase in Pseudomonas Aeruginosa: Interchangeability of the Two Anthranilate Synthases and Evolutionary Implications, J. Bacteriol., 1990, 172(2), 884–900 CrossRef CAS PubMed
.
- T. Cheng, J. Liang, J. He, X. Hu, Z. Ge and J. Liu, Novel Rhamnolipid-Producing Pseudomonas Aeruginosa ZS1 Isolate Derived from Petroleum Sludge Suitable for Bioremediation, AMB Express, 2017, 7(1), 120 CrossRef PubMed
.
-
R. Marchant and I. M. Banat, Protocols for Measuring Biosurfactant Production in Microbial Cultures, in Hydrocarbon and Lipid Microbiology Protocols, ed. T. J. McGenity, K. N. Timmis and B. Nogales, Springer Protocols Handbooks, Springer Berlin Heidelberg, Berlin, Heidelberg, 2014, pp. 119–128 Search PubMed
.
- Y. Cai, A. Hutchin, J. Craddock, M. A. Walsh, J. S. Webb and I. Tews, Differential Impact on Motility and Biofilm Dispersal of Closely Related Phosphodiesterases in Pseudomonas Aeruginosa, Sci. Rep., 2020, 10(1), 6232 CrossRef CAS PubMed
.
- J. B. Aswathanarayan and R. R. Vittal, Inhibition of Biofilm Formation and Quorum Sensing Mediated Phenotypes by Berberine in Pseudomonas Aeruginosa and Salmonella Typhimurium, RSC Adv., 2018, 8(63), 36133–36141 RSC
.
- R. A. Friesner, J. L. Banks, R. B. Murphy, T. A. Halgren, J. J. Klicic, D. T. Mainz, M. P. Repasky, E. H. Knoll, M. Shelley, J. K. Perry, D. E. Shaw, P. Francis and P. S. Shenkin, Glide: A New Approach for Rapid, Accurate Docking and Scoring. 1. Method and Assessment of Docking Accuracy, J. Med. Chem., 2004, 47(7), 1739–1749 CrossRef CAS PubMed
.
- N. Narayanan and D. T. Nair, Vitamin B12 may inhibit RNA-dependent-RNA polymerase activity of nsp12 from the SARS-CoV-2 virus, IUBMB Life, 2020, 72(10), 2112–2120 CrossRef CAS PubMed
.
- G. Q. Xu, X. Q. Gong, Y. Y. Zhu, X. J. Yao, L. Z. Peng, G. Sun, J. X. Yang and L. F. Mao, Novel 1, 2, 3-Triazole Erlotinib Derivatives as Potent IDO1 Inhibitors: Design, Drug-Target Interactions Prediction, Synthesis, Biological Evaluation, Molecular Docking and ADME Properties Studies, Front. Pharmacol., 2022, 13, 854965 CrossRef CAS PubMed
.
- G. A. Rácz, N. Nagy, J. Tóvári, Á. Apáti and B. G. Vértessy, Identification of New Reference Genes with Stable Expression Patterns for Gene Expression Studies Using Human Cancer and Normal Cell Lines, Sci. Rep., 2021, 11(1), 19459 CrossRef PubMed
.
- L. Miao, J. Xu, Z. Yao, Y. Jiang, H. Zhou, W. Jiang and K. Dong, The Anti-Quorum Sensing Activity and Bioactive Substance of a Marine Derived Streptomyces, Biotechnol. Biotechnol. Equip., 2017, 31(5), 1007–1015 CrossRef CAS
.
- N. Marappa, D. Dharumadurai, T. Nooruddin and A. Mohammad Abdulkader, Morphological, Molecular Characterization and Biofilm Inhibition Effect of Endophytic Frankia Sp. from Root Nodules of Actinorhizal Plant Casuarina Sp., S. Afr. J. Bot., 2020, 134, 72–83 CrossRef CAS
.
- S. R. Borgert, S. Henke, F. Witzgall, S. Schmelz, S. Zur Lage, S. K. Hotop, S. Stephen, D. Lübken, J. Krüger, N. O. Gomez, M. Ham, L. Jansh, M. Kalesse, A. Pich, M. Bronstrup, S. Haussler and W. Blankenfeldt, Moonlighting chaperone activity of the enzyme PqsE contributes to RhlR-controlled virulence of Pseudomonas aeruginosa, Nat.
Commun., 2022, 13(1), 1–12 Search PubMed
.
|
This journal is © The Royal Society of Chemistry 2023 |
Click here to see how this site uses Cookies. View our privacy policy here.