DOI:
10.1039/D2NA00774F
(Paper)
Nanoscale Adv., 2023,
5, 1397-1404
Efficient red luminescence in Eu3+ doped CdSe/CdS all-inorganic quantum dots shows great potential for wLEDs†
Received
4th November 2022
, Accepted 25th January 2023
First published on 30th January 2023
Abstract
Complete inorganic quantum dots (QDs) CdSe/CdS:Eu3+ with full transmittance were proposed as red color converters for white light emitting diodes (wLEDs) using a facile one-step melt quenching method. TEM, XPS, and XRD were used to verify the successful nucleation of CdSe/CdS:Eu3+ QDs in silicate glass. The results indicated that the incorporation of Eu contributed to the nucleation of CdSe/CdS QDs in silicate glass, where the nucleation time of the CdSe/CdS:Eu3+ QDs rapidly decreased in 1 h compared with other inorganic QDs that took more than 15 h. CdSe/CdS:Eu3+ inorganic QDs exhibited bright and long-term stable red luminescence under both UV and blue light excitation; up to 53.5% quantum yield and 8.05 ms fluorescence lifetime were obtained by adjusting the Eu3+ concentration. Based on the luminescence performance and absorption spectra, a possible luminescence mechanism was proposed. Moreover, the application potential of the CdSe/CdS:Eu3+ QDs in wLEDs was studied by coupling the CdSe/CdS:Eu3+ QDs and commercial Intematix G2762 green phosphor on a InGaN blue LED chip. Warm white light (5217 K) with 89.5 CRI and 91.1 lm W−1 luminous efficacy could be achieved. Additionally, 91% of the NTSC color gamut was obtained, demonstrating the great potential of the CdSe/CdS:Eu3+ inorganic QDs as a color converter for wLEDs.
Introduction
White LED (wLED) is one of the most important optical devices in people's daily lives. To date, most commercial wLEDs have been obtained using an InGaN-based blue LED chip to excite commercial Y3Al5O12:Ce3+ (YAG:Ce3+; yellow) phosphor or Lu3Al5O12:Ce3+ (LuAG:Ce3+; green) and CaAlSiN3:Eu2+ (CASN:Eu2+; red) composite phosphors.1,2 However, the absence of red components in the first white light scheme resulted in a low color rendering index (CRI) and cool white light. However, the low luminous efficiency and transparency of red phosphors used in the second white light scheme resulted in low luminous efficacy for the wLED.3 In comparison with red phosphor, the red QD was regarded as the most potential luminescent material to replace red phosphor and as a color converter for wLED because of its high quantum efficiency, narrow full width at half maximum (FWHM), high fluorescence efficiency, and wide color gamut.4–6 However, the disadvantages of agglomeration, weak thermal stability and atmospheric sensitivity of colloidal QDs (CQDs) should be addressed before their practical applications in wLEDs.7 Recently, synthesizing all-inorganic QD was proposed to overcome the disadvantages of CQDs by encapsulating QDs in a silicate glass matrix because of non-reactivity, moisture and thermal resistance of inorganic glasses.8,9 To date, various semiconductor and perovskite inorganic QDs have been successfully synthesized within a silicate glass matrix via a thermal nucleation and growth process using the melt-quenching method, which absolutely presents their promising characteristics and optical performance depending on the element composition, QD particle size, and structure.10–12 CsPbX3 (X = Cl/Br/I) halide perovskite QDs in glass are widely studied inorganic QDs because of their high quantum yield, narrow FWHM, and tunable emission.13,14 However, the low quantum efficacy (<14%) and transmittance (<10%) of the red halide perovskite QD glass (CsPbI3) limit its application potential in wLED although the halide perovskite QDs with full emission color from blue to red have been achieved by adjusting the ratio of halide elements.15,16 Even though great endeavors have been made and reported, the quantum efficiency of red-emitting CsPbI3 QDs could be partly improved by incorporating specific elements, such as fluorine and Eu3+, into the QD glass matrix. Under the high temperature and poor transmittance of CsPbI3 QD glass, the volatile characteristics of halide element I were also huge challenges for its practical application in optical devices.17–19 Except for inorganic perovskite QDs, inorganic IIB-VIA group semiconductor QDs, such as PbS, PbSe, CdSe, CdS, and ZnS, have attracted considerable attention in recent years.20–23 The advantages of high transparency, a long fluorescence lifetime, and easy precipitation in the glass matrix also show great application potential in optical devices. However, similar to the red perovskite QDs, the IIB-VIA group red QDs show relatively low quantum efficiency, which hinders their feasibility as color converters for wLEDs. The preparation of novel red-emitting all-inorganic QDs with high quantum efficiency and transmittance is still an urgent task.
To address the low quantum efficiency of IIB-VIA group red emitting inorganic QDs, we proposed and designed a novel all-inorganic transparent CdSe/CdS:Eu3+ red-emitting QDs by doping rare earth Eu3+ ions into the crystalline of CdSe/CdS co-exited QDs based on the characteristic 5D0–7F2 energy level transition of Eu3+ ions.24–26 Compared with other inorganic QDs, this CdSe/CdS:Eu3+ inorganic QD shows full transmittance and bright red fluorescence with 53.5% of QY under excitation of blue light (465 nm) and significantly reduces the preparation time (more than 10 h saved). Therefore, the CdSe/CdS:Eu3+ inorganic QD shows great potential as a color converter for wLED.
Experimental
Preparation
65SiO2–25Na2CO3–5BaO–5ZnO–10%H3BO3 (in mol%) glass system was selected as the QD glass matrix, and QD raw materials, which consisted of 0.65ZnS–0.85ZnSe–0.75CdO (in mol%), were mixed with the glass matrix materials and ground into powders for a certain time. Then, Eu2O3 was selected to provide Eu3+ and introduced into the QD crystalline based on the theoretical calculation and luminescence of the actual product. The CdSe/CdS:xEu3+ inorganic QDs were synthesized by a facile one-step melt-quenching method and by adjusting the mole concentration variation of Eu3+ ions from 0.08% to 0.8%. In detail, the mixed raw materials were melted in a muffle furnace at 1000 °C for 1 h under ambient atmosphere with a heating rate of 5 °C min−1. Subsequently, the melts were removed immediately from the muffle furnace, promptly poured into a brass mold and annealed at 400 °C for 3 h to release thermal stress. Next, the CdSe/CdS:Eu3+ QD glasses were obtained when the temperature cooled to room temperature naturally (see Fig. S1†). Moreover, a blank sample (CdSe/CdS QDs without Eu3+ ion doping) was prepared using a similar method for comparison. Finally, the as-prepared CdSe/CdS:Eu3+ QDs blended with commercial green phosphor (Intematix G2762 CIE chromaticity coordinate x = 0.25, y = 0.64) were mounted on an InGaN blue LED chip (465 nm) to assemble the wLED to examine the color conversion (The specific preparation of the wLED was supplied in ESI material part 2.†).
Characterization
To confirm the growth of the CdSe/CdS:Eu3+ QDs in glass, X-ray diffraction (XRD, D8 Advance, Bruker, Germany) measurements were carried out with CuKα radiation operating at 40 kV and 30 mA in the range of 10–80° (2 theta) at a rate of 8° s−1. Microstructure observations of CdSe/CdS:Eu3+ solid QDs were carried out using an FEI Tecnai G2 F20 TEM operated at 200 kV accelerating voltage. High-resolution TEM (HR-TEM) images were taken using an FEI aberration-corrected Titan-Cubed S-Twin transmission electron microscope operated on a HAADF mode. X-ray photoelectron spectroscopy (XPS) was carried out in a Thermo scientific 250Xi spectrometer equipped with a monochromatic Al Kα X-ray source (hν = 1486.6 eV) operated at 150 W. Photoluminescence (PL) spectra and fluorescence lifetime were recorded by applying a Hitachi F-7100 spectrophotometer equipped with continuous (450 W) xenon lamps. The quantum yield (QY) and CIE chromaticity coordinates were measured using an Edinburgh FLS 1000 spectrofluorometer. Specifically, the QY measurement should be taken in an integrating sphere and obtained by calculating the ratio of emitted photons to those absorbed by the QD samples. The absorption spectra were collected using a Lambda 950 UV-Vis spectrophotometer (PerkinElmer, USA). The optical properties of the QDs/phosphors converted wLEDs, including the color rendering index (CRI), correlated color temperature (CCT), electroluminescence (EL) spectra, and luminous efficacy (LE), were evaluated using a spectroradiometer system that consisted of an integrating sphere (PMS-50, Everfine, China) and a CCD spectrometer (CDS2100, Labsphere Inc., USA).
Results and discussion
Fig. 1a presents the TEM image of the CdSe/CdS:Eu3+ QDs samples; it can be observed that the QDs dispersed well in the inorganic glass matrix with an average diameter of 1.8 nm (Fig. S2†). The HR-TEM image of the QDs shown in Fig. 1b revealed that QDs possess interplanar distances of 0.373, 0.351, 0.358, and 0.316 nm, corresponding to the (100), (002), (100), and (101) lattice planes of CdSe and CdS.27,28 Moreover, the XRD patterns were investigated to verify the successful synthesis of the inorganic CdSe/CdS:Eu3+ QDs in glass. As illustrated in Fig. 1c, although there is no sharp diffraction peak emerging due to the nano particle size of the inorganic QDs, part of the weak characteristic diffraction peaks can be observed compared with the XRD patterns of the blank samples without Eu ion doping (see Fig. S3†), which agrees with standard CdSe JCPDS#77-2307 and CdS JCPDS#77-2306. This verifies the formation of CdSe and CdS QDs and the contribution of Eu3+ ions to the nucleation and crystalline growth of CdSe and CdS QDs in the glass matrix. By continuously increasing the mole concentrations of Eu3+ ions to 0.8%, some of the characteristic diffraction peaks of the samples disappeared, and some new diffraction peaks appeared in the XRD patterns, indicating that the ordered crystal structure of the CdSe/CdS QDs may be destroyed by incorporating Eu ions. Except for CdSe and CdS, no characteristic diffraction peak of ZnSe of ZnSeS can be observed, implying that the element of Zn does not participate in the crystallization of the QDs in glass. This may be attributed to the short synthesis time of the QD glass. Notably, although the TEM images and XRD patterns showed the existence of CdSe and CdS QDs in glass, the structure of CdSe/CdS:Eu3+ QD could not be confirmed.
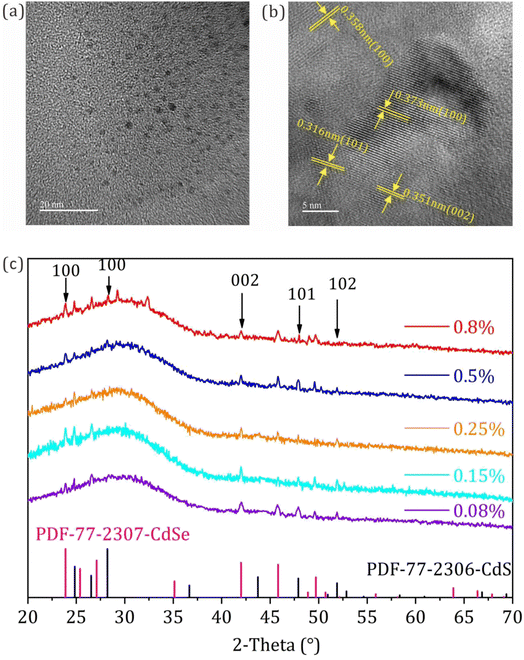 |
| Fig. 1 (a) and (b) TEM and HR-TEM images; (c) XRD patterns of the CdSe/CdS:Eu3+ QDs with different Eu3+ concentrations of Eu3+ doping. | |
XPS comparative analyses of the inorganic CdSe/CdS:Eu3+ QDs are carried out to further confirm the incorporation of Eu3+ ions. Fig. 2a illustrates a full-scan spectrum for all elements in CdSe/CdS:Eu3+ QDs. The characteristic peaks of the main elements of Cd and Se can be clearly found, but the peaks of Eu and S are too weak to be discovered because of the low levels of these two elements in the QDs. Fig. 2b–e shows high-resolution XPS narrow-sweep spectra for the elements of Eu 3d, Cd 3d, Se 3d, and S 5p. The characteristic peaks at 1163 eV and 1134 eV binding energies, which were assigned to Eu 3d3/2 and Eu 3d5/2, further verified the fact that Eu3+ was successfully introduced into QDs. Cd 3d revealed two peaks with banding energies of 412 eV and 405.4 eV, which correspond to Cd 3d3/2 and Cd 3d5/2. In addition, the single peak at 63.2 eV binding energy originated from Se 3d, and the peak at 168.7 eV was assigned to S 2p. Comparing the XPS peak intensity and location of all the QD elements, the binding energies of all the QD element XPS spectra exhibited small variations, whereas the intensity of the Eu 3d peaks increased along with the Eu3+ ion concentration from 0.08% to 0.8%. The results confirmed the fact that Eu3+ ions entered the lattice of CdSe/CdS QDs and generated enhanced Eu–Cd–Se and Eu–Cd–S bonds with the increase of Eu3+ doping.
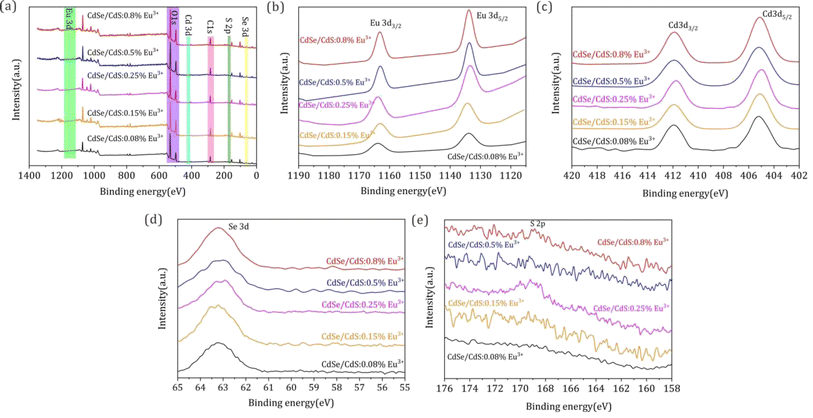 |
| Fig. 2 (a) XPS survey spectra of CdSe/CdS:Eu3+ QDs with different Eu3+ concentrations. (b)–(e) The high-resolution XPS analysis corresponds to Eu 3d, Cd 3d, Se 3d, and S 2p, separately. | |
The PL excitation and emission spectra of the CdSe/CdS:Eu3+ inorganic QDs incorporated with different concentrations of Eu3+ ions are shown in Fig. 3a and b to explore their optical properties. In compassion with the excitation spectrum of the blank sample (Fig. S4a†), sharper excitation peaks that originated from Eu3+ ions emerged from 350 to 550 nm in the excitation spectra of CdSe/CdS:Eu3+ inorganic QDs. The highest excitation peaks at 393 nm and 465 nm implied that the CdSe/CdS:Eu3+ QDs could be best excited by both UV and visible light, especially blue light. The emission band under the excitation of 465 nm comprises a continuous broad band from 575 nm to 675 nm with multiple emission peaks at 580, 592, 612, and 656 nm, thereby verifying that the CdSe/CdS:Eu3+ QDs could be excited by blue light to emit red luminescence. Specifically, except for the peak of 580 nm belonging to the yellow emission of CdSe/CdS (see Fig. S4b†), all the residual peaks at 592, 612, and 656 nm originated from the electronic energy level transition of 5D0–7F1, 5D0–7F2, and 5D0–7F3 from Eu3+.29 With the variation in Eu3+ ion concentrations from 0.08% to 0.8% mol, except for the enhancement of PL spectra intensity, the FWHM of the PL spectra, the position of the excitation, and emission peak have no variation, thereby indicating the luminescence color stability of the CdSe/CdS:Eu3+ inorganic QDs. The optical images and CIE chromaticity coordinate diagrams of the CdSe/CdS:Eu3+ inorganic QDs doped with various Eu3+ concentrations under the excitation of 465 nm in a dark environment were also presented in Fig. 3c and d to certify the enhanced red luminescence, high transparency and color stability of the CdSe/CdS:Eu3+ QDs. From the PL emission spectra and optical images, it can be concluded that the increasing Eu3+ concentration in the range of 0.08–0.8% contributes to improving the red emission of the inorganic CdSe/CdS:Eu3+ QDs. Moreover, the detailed CIE chromaticity coordinate values and QY, and color purity of the QDs samples, are inserted in Fig. 3d. All inorganic QD samples showed more than 99% color purity, and the QY also increased rapidly from 7.4% to 53.5% when the Eu3+ concentration increased to 0.8%, thereby suggesting the great potential of the CdSe/CdS:Eu3+ inorganic QDs as color converters for wLED. Additionally, the PL emission spectra, optical photos, quantum yield (QY), and CIE chromaticity coordinates at 30 days and 60 days supplied in Fig. S7 and Table S1† certified the long-term optical stability of the inorganic CdSe/CdS:Eu3+ QDs.
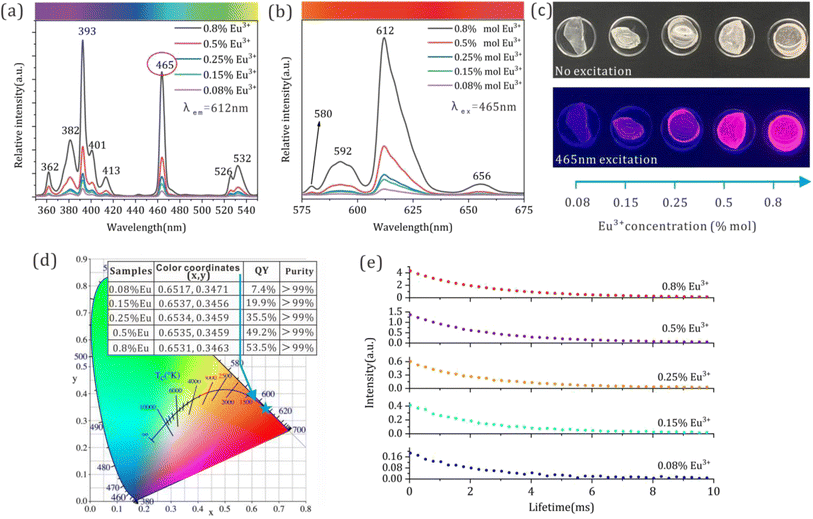 |
| Fig. 3 (a) and (b) Excitation and emission spectra; (c) and (d) optical photos and CIE-1931 chromaticity coordinate diagram of the QDs under excitation of blue light with a wavelength of 465 nm, the corresponding optical parameters including CIE chromaticity coordinate diagram values, QY and color purity were inserted; (e) fluorescence decay curve. | |
Fig. 3e presents the fluorescence lifetime decay curve of the CdSe/CdS:Eu3+ QDs. In detail, the fluorescence lifetime decay curves agree with the following double exponential equations via Gaussian fitting:30
| 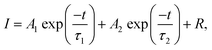 | (1) |
where
I represents the integral area of the PL emission spectrum,
t is the time,
A1 and
A2 are the fitting constants,
τ1 and
τ2 are the fast and slow decay lifetimes, respectively, and
R is the correction coefficient. The average fluorescence lifetime of CdSe/CdS:Eu
3+ QDs can be calculated using the following formula:
|  | (4) |
Compared with the CdSe/CdS QDs,31 the fluorescence lifetime of the CdSe/CdS:Eu3+ inorganic QDs was prolonged significantly from nanosecond to millisecond level. Moreover, the increased Eu3+ concentration in the range of 0.08–0.5% contributes to prolonging the fluorescence lifetime of the QD samples from 5.91 ms to 8.05 ms, accompanied by the increase in PLQY. This suggests that the doped Eu3+ ions entered the lattice of CdSe/CdS QDs and improved the interface defects between the QDs and glass matrix, and this contributed to eliminating the non-radiative transition paths to increase the fluorescence lifetime of inorganic QDs. However, by continuously increasing Eu3+ concentration to 0.8%, the fluorescence lifetime decreased to 7.83 ms owing to the concentration quenching effect.32 The unified double exponential behavior of the fluorescence lifetime decay curve suggested that the inorganic CdSe/CdS QDs matrix provided a homogenous electronic transition environment for Eu3+ ions and certified that Eu3+ ions were incorporated into the CdSe/CdS QDs lattice.
To explore the reason for the contribution of Eu3+ ions to the enhanced red emission of CdSe/CdS QDs, the absorption spectra of the CdSe/CdS:Eu3+ QDs are illustrated in Fig. 4. The absorption spectra of the CdSe/CdS:Eu3+ QDs displayed strong absorption from UV to visible light in the range of 350–550 nm with characteristic absorption peaks at 393, 465, 526 and 532 nm, which agrees with the excitation spectra. Except for the absorption peak of 393 nm belonging to CdSe/CdS, the residual characteristic absorption peaks must be assigned to the absorption of Eu3+, demonstrating that some kinds of energy upconversion may happen from CdSe/CdS to Eu3+, which leads to an improvement in red emission. Furthermore, in comparison with the PL emission and absorption spectra of CdSe/CdS:Eu3+ QDs, no overlap area can be observed, indicating that there is no energy re-absorption in the CdSe/CdS:Eu3+ QD system, which avoided lots of energy loss. This is another reason for the enhanced red emission of CdSe/CdS:Eu3+ QDs.
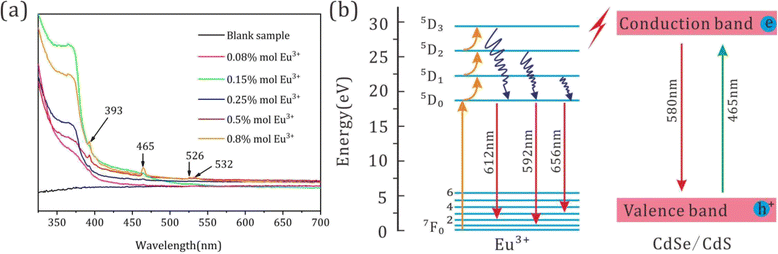 |
| Fig. 4 (a) Absorption spectra of CdSe/CdS:Eu3+ QD. (b) Energy level diagram of CdSe/CdS:Eu3+ QD glass and possible photoluminescence energy transfer mechanism. | |
Consequently, a possible luminescence mechanism of the inorganic CdSe/CdS:Eu3+ QDs is depicted in Fig. 4f. The free electrons in the valence band jump to the conduction band under the excitation of a blue light source and then return to the valence band immediately and combine with the electron hole to release the luminescence of CdSe/CdS QDs with a wavelength of 580 nm. Additionally, a luminescence energy transfer occurred from CdSe/CdS QDs to the incorporated Eu3+ ions, where the free electrons of Eu3+ ions could be stimulated to jump to an energy level of 5D0 from 7F0. Notably, the excited state electrons of Eu3+ show a special energy up-conversion process, which can jump to higher energy levels of 5D1, 5D2, and 5D3, and then release enhanced red luminescence with wavelengths of 595, 612, and 656 nm when returning from the states of 7F1, 7F2 and 7F3, corresponding to its electronic energy level transitions of 5D0–7F1, 5D0–7F2, and 5D0–7F3, respectively.
To further investigate the application feasibility of the prepared red emitting all-inorganic CdSe/CdS:Eu3+ QDs in wLED, the EL spectra of the wLED devices were measured by coupling the InGaN blue LED chip (EL spectra were supplied in Fig. S5a†) with G2762 green phosphors (PL spectra were supplied in Fig. S5b†) and CdSe/CdS:Eu3+ inorganic QDs (Fig. 5). To construct the wLED, the CdSe/CdS:Eu3+ inorganic QDs are pulverized into glass powders and combined with Intematix G2762 green phosphors to create a composite glass plate with a thickness of 0.25 mm. Subsequently, the composite glass plate is mounted on the InGaN blue LED chip under an operating current of 20 mA. Based on the QY and optical performance of the CdSe/CdS:Eu3+ QDs, two kinds of wLEDs were fabricated by selecting CdSe/CdS:0.5%Eu3+ and CdSe/CdS:0.8%Eu3+ QDs as color converters. As depicted in Fig. 5a and b, stable blue/green/red emissions can be detected in the EL spectra. Pure white light and warm white light were produced by yielding white light with tunable CCT (5333 K and 5217 K), CRI (92.1 and 89.5), and LE (88.3 and 91.1 lm W−1) (Fig. 5c and insets of Fig. 5a and b). Obviously, the warm white light of the wLED originated from the increasing red component of the EL spectra. Furthermore, the increase in Eu3+ ions doped into inorganic CdSe/CdS QDs contributes to improved LE, which is comparable to those devices based on inorganic halide perovskite CsPbI2/P-Y2O3 (61 lm W−1),33 colloidal CsPbBr1I2-TS-1 QDs and YAG:Ce3+ phosphor (95 lm W−1),34 Gd-doped CsPbBrI2 nanocrystals (90.09 lm W−1),35 and CaAlSiN3:Eu2+ phosphors glass (90.3 lm W−1)36 reported in the literature. Furthermore, the high LE value is not based on the sacrifice of the color rendering index, which is quite different from those of previously reported studies, where the increase in CRI decreases LE.37,38 This may be attributed to the luminescence energy transfer of CdSe/CdS-Eu3+ and the high transmittance of the inorganic CdSe/CdS:Eu3+ QDs.39,40 The overlap area between the excitation spectrum of the green phosphor and the PL emission spectrum of the CdSe/CdS:Eu3+ QDs in Fig. S5b–d† indicated that the luminescence of green phosphor could be absorbed by the inorganic QDs to reduce energy loss, and this is also another reason for the excellent LE performance of the wLED device. In comparison with the National Television Standards Committee (NTSC) color gamut (black line in Fig. 5c), 91% of the NTSC color gamut (white line) could be obtained by calculating the CIE-1931 area that comprises the chromaticity coordinate of the blue InGaN LED chip, G2762 green phosphor, and red inorganic CdSe/CdS:0.5%Eu3+ QDs, thereby demonstrating the excellent color reproduction of the inorganic CdSe/CdS:Eu3+ QDs and great potential in wLED and other optical display devices.
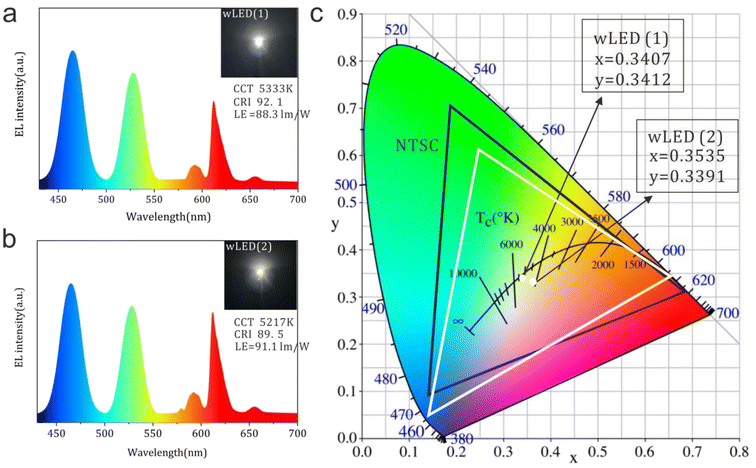 |
| Fig. 5 (a) and (b) EL spectra of the self-assembled wLED devices by coupling GaN blue chip with G2762 green phosphors and the prepared inorganic CdSe/CdS:0.5%Eu3+ QDs and CdSe/CdS:0.8%Eu3+ QDs; (c) CIE-1931 chromaticity coordinate diagram of the as-prepared LED device; inset: the corresponding optical images of the wLED devices driven by a 20 mA operation current. | |
Conclusion
Herein, we successfully fabricated a complete inorganic red emitting QD CdSe/CdS:Eu3+ with high transmittance and efficient quantum efficiency, as well as long-term stable optical performance, by incorporating Eu3+ ions into silicate glass-based CdSe/CdS QDs using a facile one-step melt quenching method. Enhanced red luminescence could be observed by increasing Eu3+ concentration under the excitation of 465 nm, and up to 53.5% of quantum yield was achieved when Eu3+ mole concentration increased to 0.8%. The improvement in the red luminescence was attributed to the energy transfer of CdSe/CdS-Eu3+ and energy up-conversion of Eu3+ in the electronic energy level transitions of 5D0–7F1, 5D0–7F2, and 5D0–7F3. A warm white light (CCT = 5217 K) with 89.5 of CRI and 91.1 lm W−1 of LE was obtained by mounting the as-prepared CdSe/CdS:0.8%Eu3+ inorganic QDs and commercial Intematix G2762 green phosphor on the InGaN blue LED chip, which is comparable to those of phosphor- and CQD-based wLEDs. Furthermore, 91% of the NTSC color gamut was obtained, demonstrating the excellent color reproduction of the inorganic CdSe/CdS:Eu3+ inorganic QDs and great potential as color converters for wLED and LED displays.
Conflicts of interest
There are no conflicts to declare.
Acknowledgements
This work was financially supported by the Natural Science Foundation of Fujian Province (No. 2020J05173), Major Science and Technology Project of Fuzhou city (No. 2021-ZD-298) and Major Science and Technology Project of Jiebang Guashuai of Fuzhou city (No. 2022-ZD-007).
References
- R. Wang, M. Y. Wang, G. Li, J. H. Zhang, Y. J. Zhang, H. Lin, E. Y. B. Pun and D. S. Li, Red-emitting improvement of CaAlSiN3:Eu2+ phosphor-in-glass: Insight into the effect of atmospheric pressure preparation on photoluminescence properties and thermal degradation, J. Lumin., 2020, 225, 117390 CrossRef CAS.
- Y. H. Nam, W. J. Chung and W. B. Im, Phosphor in glass using β-SiAlON:Eu2+, CaAlSiN3:Eu2+ and Nd-doped silicate glass for enhanced color gamut of white LED, J. Alloy Compd., 2021, 851, 156945 CrossRef.
- P. Dang, G. Li, X. Yun, Q. Zhang, D. Liu, H. Lian, M. Shang and J. Lin, Thermally stable and highly efficient red-emitting Eu3+-doped Cs3GdGe3O9 phosphors for WLEDs: non-concentration quenching and negative thermal expansion, Light: Sci. Appl., 2021, 10, 29 CrossRef CAS PubMed.
- J. S. Li, Y. Tang, Z. T. Li, J. X. Li, X. R. Ding, B. H. Yu, S. D. Yu, J. Z. Ou and H. C. Kuo, Toward 200 Lumens per Watt of Quantum-Dot White-Light-Emitting Diodes by Reducing Reabsorption Loss, ACS Nano, 2021, 15, 550–562 CrossRef CAS PubMed.
- X. Li, W. Cai, H. Guan, S. Zhao, S. Cao, C. Chen, M. Liu and Z. Zang, Highly stable CsPbBr3 quantum dots by silica-coating and ligand modification for white light-emitting diodes and visible light communication, Chem. Eng. J., 2021, 419, 129551 CrossRef CAS.
- L. Rao, Q. Zhang, M. Wen, Z. Mao, H. Wei, H. Chang and X. Niu, Solvent regulation synthesis of single-component white emission carbon quantum dots for white light-emitting diodes, Nanotechnol. Rev., 2021, 10, 465–477 CrossRef CAS.
- Z. Li, C. Song, J. Li, G. Liang, L. Rao, S. Yu, X. Ding, Y. Tang, B. Yu, J. Ou, U. Lemmer and G. Gomard, Highly Efficient and Water-Stable Lead Halide Perovskite Quantum Dots Using Superhydrophobic Aerogel Inorganic Matrix for White Light-Emitting Diodes, Adv. Mater. Technol., 2020, 1900941 CrossRef CAS.
- X. Yang, Z. Zhang, T. Ding, N. Wang, G. Chen, C. Dang, H. V. Demir and X. Sun, High-efficiency all-inorganic full-colour quantum dot light-emitting diodes, Nano energy, 2018, 46, 229–233 CrossRef CAS.
- Q. Xu, X. Li, Q. Lin, H. Shen, H. Wang and Z. Du, Improved Efficiency of All-Inorganic Quantum-Dot Light-Emitting Diodes via Interface Engineering, Front. Chem., 2020, 8, 265 CrossRef CAS PubMed.
- M. Xia, J. Luo, C. Chen, H. Liu and J. Tang, Semiconductor quantum dots-embedded inorganic glasses: fabrication, luminescent properties, and potential applications, Adv. Opt. Mater., 2019, 7(21), 1900851 CrossRef CAS.
- Y. Tong, Q. Wang, X. Liu, E. Mei, X. Liang and W. Xiang, The promotion of TiO2 induction for finely tunable self-crystallized CsPbX3 (X = Cl, Br and I) nanocrystal glasses for LED backlighting display, Chem. Eng. J., 2022, 429, 132391 CrossRef CAS.
- Y. Wang, F. Yuan, Y. Dong, J. Li, A. Johnston, B. Chen, M. I. Saidaminov, C. Zhou, X. Zheng, Y. Hou, K. Bertens, H. Ebe, D. Ma, Z. Deng, S. Yuan, R. Chen, L. K. Sagar, J. Liu, J. Fan, P. Li, X. Li, Y. Gao, M. K. Fung, Z. H. Lu, O. M. Bakr and L. Liao, All-Inorganic Quantum-Dot LEDs Based on a Phase-Stabilized α-CsPbI3 Perovskite, Angew. Chem., Int. Ed., 2021, 60, 16164 CrossRef CAS PubMed.
- H. Zhang, M. Jin, X. Liu, Y. Zhang, Y. Yu, X. Liang, W. Xiang and T. Wang, The preparation and up-conversion properties of full spectrum CsPbX3 (X = Cl, Br, I) quantum dot glasses, Nanoscale, 2019, 11, 18009 RSC.
- C. Chen, L. Nie, Y. Huang, S. Xi, X. Liu, X. Zhang, T. Shi, G. Liao, S. Liu and Z. Tang, Embedded growth of colorful CsPbX3 (X = Cl, Br, I) nanocrystals in metal-organic frameworks at Room Temperature, Nanotechnology, 2022, 33, 175603 CrossRef PubMed.
- D. Chen, Y. Liu, C. Yang, J. Zhong, S. Zhou, J. Chen and H. Huang, Promoting photoluminescence quantum yields of glass-stabilized CsPbX3 (X=Cl, Br, I) perovskite quantum dots through fluorine doping, Nanoscale, 2019, 11, 17216 RSC.
- B. Shu, Y. Chang, J. Zhang, X. Cheng and D. Yu, Synthesis and photoluminescence kinetics of Ce3+-doped CsPbI3 QDs with near-unity PLQY, Nano Res., 2021, 14(10), 3352–3357 CrossRef CAS.
- A. Swarnkar, W. J. Mir and A. Nag, Can B–site doping or alloying improve thermal- and phase-stability of all-inorganic CsPbX3 (X = Cl, Br, I) perovskites?, ACS Energy Lett., 2018, 3, 286 CrossRef CAS.
- S. Seth, T. Ahmed, A. De and A. Samanta, Tackling the defects, stability, and photoluminescence of CsPbX3 perovskite nanocrystals, ACS Energy Lett., 2019, 4, 1610 CrossRef CAS.
- C. H. Bi, S. V. Kershaw, A. L. Rogach and J. J. Tian, Improved stability and photodetector performance of CsPbI3 perovskite quantum dots by ligand exchange with aminoethanethiol, Adv. Funct. Mater., 2019, 29, 1902446 CrossRef.
- P. Liu, Y. Lou, S. Ding, W. Zhang, Z. Wu, H. Yang, B. Xu, K. Wang and X. Sun, Green InP/ZnSeS/ZnS Core Multi-Shelled Quantum Dots Synthesized with Aminophosphine for Effective Display Applications, Adv. Funct. Mater., 2021, 31, 2008453 CrossRef CAS.
- B. B. Jin, S. Y. Kong, G. Q. Zhang, X. Q. Chen, H. S. Ni, F. Zhang, D. J. Wang and J. H. Zeng, Voltage-assisted SILAR deposition of CdSe quantum dots to construct a high performance of ZnS/CdSe/ZnS quantum dot- sensitized solar cells, J. Colloid Interf. Sci., 2021, 586, 640 CrossRef CAS PubMed.
- W. Li, K. Li, X. Zhao, C. Liu and F. X. Coudert, Defective Nature of CdSe Quantum Dots Embedded in Inorganic Matrices, J. Am. Chem. Soc., 2022, 144(25), 11296–11305 CrossRef CAS PubMed.
- U. Latief, S. U. Islam, Z. Khan and M. S. Khan, Luminescent Manganese/Europium doped ZnS quantum dots: Tunable emission and their application as fluorescent sensor, J. Alloy Compd., 2022, 910, 164889 CrossRef CAS.
- C. Lu, Y. Duan, P. Li, Y. Lu, S. Xu and J. Zhang, Polychromatic tunable luminescence of Eu3+ doped CsPbBr3 quantum dot glass ceramic induced by mechanical crystallization, Ceram. Int., 2022, 48, 13826 CrossRef CAS.
- E. Erdinç, V. Naji, U. Ekim, U. Nazlıcan, Ç. E. Miray and E. E. Ali, Ultra-stable Eu3+/Dy3+ co-doped CsPbBr3 quantum dot glass nanocomposites with tunable luminescence properties for phosphor-free WLED applications, J. Alloy Compd., 2022, 909, 164650 CrossRef.
- X. Jing, D. Zhou, R. Sun, Y. Zhang, Y. n Li, X. Li, Q. n Li, H. Song and B. Liu, Enhanced Photoluminescence and Photoresponsiveness of Eu3+ Ions-Doped CsPbCl3 Perovskite Quantum Dots under High Pressure, Adv. Funct. Mater., 2021, 31, 2100930 CrossRef CAS.
- K. Han, W. B. Im, J. Heo and W. J. Chung, A complete inorganic colour converter based on quantum-dot-embedded silicate glasses for white light-emitting-diodes, Chem. Commun., 2016, 52, 3564 RSC.
- K. Han, W. B. Im, J. Heo and W. J. Chung, Compositional dependency of Cd-S-Se quantum dots within silicate glass on color conversion for a white LED, J. Am. Ceram. Soc., 2019, 102, 1703 CAS.
- R. Yuan, J. Liu, H. Zhang, Z. Zhang, G. Shao, X. Liang and W. Xiang, Eu3+-doped CsPbBr1.5I1.5 quantum dots glasses: A strong competitor among red fluorescence solid materials, J. Am. Ceram. Soc., 2018, 101, 4927 CrossRef CAS.
- P. P. Li, Y. Lu, Y. M. Duan, S. Q. Xu and J. J. Zhang, Potential application of perovskite glass material in photocatalysis field, J. Phys. Chem. C, 2021, 125, 2382 CrossRef CAS.
- P. Sara, D. Dirk, F. Sebastian, F. Axel, W. Andreas and C. B. Nadja, Aerogels from CdSe/CdS Nanorods with Ultra-long Exciton Lifetimes and High Fluorescence Quantum Yields, Adv. Mater., 2015, 27, 6152 CrossRef PubMed.
- J. Sun, W. Zheng, P. Huang, M. Zhang, W. Zhang, Z. Deng, S. Yu, M. Jin and X. Chen, Efficient Near-Infrared Luminescence in Lanthanide-Doped Vacancy-Ordered Double Perovskite Cs2ZrCl6 Phosphors via Te4+ Sensitization, Angew. Chem., Int. Ed., 2022, 61, e202201993 CAS.
- X. Li, Y. Wei, P. Dang, X. Xiao, H. Xiao, G. Zhang, G. Li and J. Lin, Enhancing the stability of perovskite quantum dots CsPbX3 (X=Cl, Br, I) by encapsulation in porous Y2O3 nanoparticles for WLED applications, Mater. Res. Bull., 2022, 146, 111592 CrossRef CAS.
- J. Li, Z. Chen, J. Liu, E. Mei, X. Liang, J. Liu and W. Xiang, Novel and stable CsPbX3-TS-1 (X= Br, I) nanocomposites for light-emitting diodes, Chem. Eng. J., 2021, 426, 131327 CrossRef CAS.
- Q. He, Y. Zhang, Y. Yu, Y. Chen, M. Jin, E. Mei, X. Liang, L. Zhai and W. Xiang, Ultrastable Gd3+ doped CsPbBrI2 nanocrystals red glass for high efficiency WLEDs, Chem. Eng. J., 2021, 411, 128530 CrossRef CAS.
- Y. Zhang, Z. Zhang, X. Liu, G. Shao, L. Shen, J. Liu, W. Xiang and X. Liang, A high quantum efficiency CaAlSiN3:Eu2+ phosphor-in-glass with excellent optical performance for white light-emitting diodes and blue laser diodes, Chem. Eng. J., 2020, 401, 125983 CrossRef CAS.
- T. Deng, E. Song, Y. Zhou and J. Yuan, Implementation of high color quality, high luminous warm WLED using efficient and thermally stable Rb3AlF6:Mn4+ as red color converter, J. Alloy Compd., 2019, 795, 453 CrossRef CAS.
- X. Liu, X. Qian, P. Zheng, X. Chen, Y. Feng, Y. Shi, J. Zou, R. Xie and J. Li, Composition and structure design of three-layered composite phosphors for high color rendering chip-on-board light-emitting diode devices, J. Adv. Ceram., 2021, 10(4), 729–740 CrossRef CAS.
- Z. Yang, L. Zhao, M. Li, Q. Yang, Z. Fang, L. Yang, X. Yu, J. Qiu, Y. Yang and X. Xu, Perovskite quantum dots growth in situ in transparent medium for short wavelength shielding, J. Am. Ceram. Soc., 2020, 103, 4150 CrossRef CAS.
- P. M. Tan, N. X. Ca, N. T. Hien, H. T. Van, P. V. Do, L. D. Thanh, V. H. Yen, V. P. Tuyen, Y. Peng and P. T. Tho, New insights on the energy transfer mechanisms of Eu-doped CdS quantum dots, Phys. Chem. Chem. Phys., 2020, 22, 6266 RSC.
|
This journal is © The Royal Society of Chemistry 2023 |
Click here to see how this site uses Cookies. View our privacy policy here.