DOI:
10.1039/D3NJ04371A
(Paper)
New J. Chem., 2023,
47, 20358-20362
Catalytic prenylation of natural polyphenols†
Received
17th September 2023
, Accepted 19th October 2023
First published on 20th October 2023
Abstract
Prenylated polyphenols occur naturally and exhibit biological activity superior to the ubiquitous parent polyphenols. However, their low abundance limits the wider application of these derivatives. In this work, we present an expedient, single-step catalytic protocol for introducing terpene fragments into the aromatic rings of the widely available natural stilbenoids and flavonoids to upgrade their therapeutic potential.
Introduction
Flavonoids and stilbenoids are ubiquitous secondary metabolites in plants, leaves, flowers, fruits and seeds.1,2 Bioactive flavonoids acquired a proven track record of beneficial effects on human health;3 the pharmaceutical, medicinal, nutraceutical and cosmetics industries actively use flavonoids in their development.3,4 However, the most common polyphenols do not reach therapeutic plasma concentration levels due to the low solubility and poor absorption of the compounds. This low bioavailability can be improved through chemical modifications that alter the polarity and lipophilicity of the polyphenols, as well as the biological functions that depend on the structure. Among the chemical modification strategies, prenylation is often considered an essential way to boost the potency of the parent flavonoid.5–8 Prenylated analogues have been detected for most flavonoids, such as chalcones, stilbenes, flavanones, flavones, flavonols and isoflavones, with some examples shown in Fig. 1. While flavonoids are usually present in reasonable quantities in nature, the levels of prenylated flavonoids are much lower. The low abundance in nature limits their application as medicines and nutraceuticals, therefore it is important to develop facile synthetic approaches.
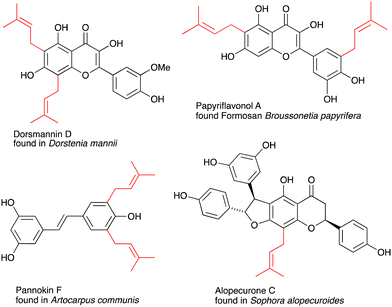 |
| Fig. 1 Selected examples of natural prenylated polyphenols. | |
Chemical synthesis of prenylated flavonoids usually relies on multistep sequences involving the protection of hydroxyls, O-prenylation followed by the Claisen rearrangement of the O- to C-isomer and deprotection.8–12 Alternative approaches are based on Suzuki–Miyaura cross-coupling, which requires prior synthesis of the respective halides or boronic acid derivatives,13,14 or the use of chemoenzymatic methods.15,16
Herein, we present the development of a simple, single-step catalytic Friedel–Crafts alkylation of unprotected natural polyphenols using prenyl alcohol and its analogues.
Results and discussion
Optimisation studies
Catalytic C–H allylation of aromatic compounds is a widely used synthetic transformation for the formation of C–C bonds.17 Of the plethora of methods for the modification of electron-rich arenes, Friedel–Crafts alkylation looks appealing from the practical viewpoint because it can employ unprotected allylic alcohols as the electrophiles.18,19 The reaction can be performed using catalytic quantities of Lewis or Brønsted acids.18,20,21
However, despite the apparent simplicity, there are very few reports of the Friedel–Crafts prenylation of polyphenolic compounds, which mainly focus on the allylation of related resorcinol analogues.22–24 The reason for this may stem from the inactivation of the Lewis catalysts by binding to the phenolic groups. Therefore, successful examples22,24,25 usually employ the excess of promoters.
With the aim to develop a catalytic system for the modification of natural polyphenols, prenylation of resveratrol 1a was selected for optimisation studies (Table 1).
Table 1 Optimisation of prenylation of resveratrol 1aa
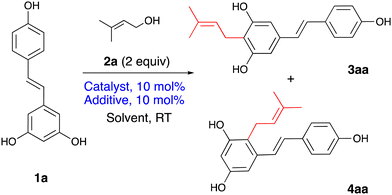
|
Entry |
Catalyst |
Additive |
Solvent |
Yieldb (%) |
Ratio 3/4 |
Conditions: 1a (1mmol), 2a (2 mmol), catalyst (10 mol%) and additive (10 mol%); in 24 mL solvent under N2 at RT overnight.
Combined isolated yield; nr: no reaction.
Catalyst: 10 mol%, additive: 20 mol%.
Catalyst: 10 mol%; additive: 30 mol%.
|
1 |
(acac)2MoCl2 |
AgOTf |
CH3CN |
13 |
40/60 |
2 |
Fe(OTf)3 |
— |
CH3CN |
48 |
1/99 |
3 |
In(OAc)3 |
— |
CH3CN |
nr |
— |
4 |
InCl3 |
— |
CH3CN |
nr |
— |
5 |
InCl3 |
AgOTf |
CH3CN |
37 |
1/99 |
6 |
AlCl3 |
— |
CH3CN |
42 |
25/75 |
7 |
AlCl3 |
AgOTf |
CH3CN |
65 |
26/74 |
8 |
AlCl3 |
AgOTf |
Et2O |
Trace |
— |
9 |
AlCl3 |
AgOTf |
MeOH |
nr |
— |
10 |
AlCl3 |
AgOTf |
DCM |
Trace |
— |
11
|
AlCl
3
|
AgOTf
|
Acetone
|
85
|
21/79
|
12 |
AlCl3 |
AgOTf |
EtOAc |
81 |
31/69 |
13c |
AlCl3 |
AgOTf |
Acetone |
59 |
24/76 |
14d |
AlCl3 |
AgOTf |
Acetone |
42 |
24/76 |
First, a catalytic system developed by us previously for the allylation of electron-rich arenes was examined.21 It employed the Lewis acidic complex (acac)2MoCl2 activated by silver triflate by replacing the chloride with a non-nucleophilic anion (entry 1), however, the yield and regioselectivity were low. Next, a series of other metal salts were examined. With Fe(OTf)3 (entry 2), 2-prenylresveratrol 4aa was obtained in a modest yield with only trace quantities of the 4-isomer 3aa. Indium(III) acetate and chloride were inactive (entries 3 and 4), but InCl3 activated by AgOTf worked similarly to Fe(OTf)3, with a slightly reduced yield (entry 5). The increased yield was obtained with the catalytic system based on the equimolar quantities of AlCl3 and AgOTf (entry 7). AlCl3 worked even without silver triflate (entry 6) but with lower product yield. Notably, AlCl3 produced a 3
:
1 mixture of regioisomers 4aa and 3aa. This could be viewed as a disadvantage, however, taking into account that the isomers are readily separable by chromatography and that synthesis of isomer 3aa, a naturally occurring arachidin-2, requires multistep sequences,26,27 this reaction can serve as a viable alternative for obtaining practical quantities of 3aa, in a single step. In this context, the molybdenum catalyst (entry 1) exhibited the highest proportion of the symmetrical 3aa in the product mixture. To boost the reaction yield, a protection strategy was explored, where the hydroxy groups were protected as silyl ethers (Scheme 1). A nearly equimolar mixture of 3aa and 4aa has been achieved, though, despite some improvement in the ratio towards 3aa, the overall yield remained modest.
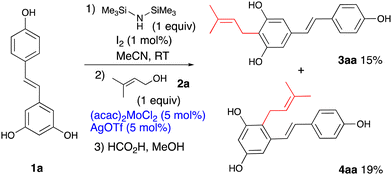 |
| Scheme 1 Prenylation of protected resveratrol. | |
Next, the reaction solvents were briefly assessed (entries 8–12) with a focus on greener solvents with lower environmental impact.28,29 Ether and methanol were found unsuitable (entries 8 and 9); as was non-green dichloromethane (entry 10). The highest yields were obtained in acetone and ethyl acetate (entries 11 and 12). An increase in the number of equivalents of AgOTf to AlCl3 proved to be detrimental to the reaction yield but the product ratio remained the same (entries 13 and 14). Thus, the conditions of entry 11 were selected as optimal.
The reaction scope
With the optimal protocol in hand, the reaction scope has been investigated using naturally occurring polyphenols. The results are presented according to the polyphenol classes.
Following the optimisation experiments, the allylation of resveratrol was assessed first. Chemical yields of the isolated pure compounds are shown in Table 2. Two entries are shown for the reaction of resveratrol with prenol. Thus, under the optimal conditions (entry 1), the isolated yields of both regioisomers 3aa and 4aa are presented. At the same time, this reaction can occur regioselectively, furnishing only 4aa (entry 2). In this instance, Fe(OTf)3 had been used as a catalyst.
Table 2 Allylation of resveratrol 1aa
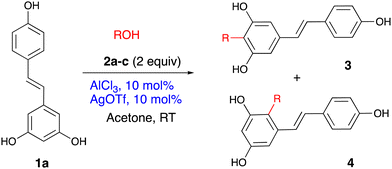
|
Entry |
Alcohol 2, R |
3, Yield (%) |
4, Yield (%) |
Conditions: 1a (1mmol), 2 (2 mmol), AlCl3 (10 mol%) and AgOTf (10 mol%); in 24 mL solvent under N2 at RT overnight, unless stated otherwise. The regioisomers were separated by flash chromatography, yields are given for isolated analytically pure compounds.
The reaction was performed in CH3CN using Fe(OTf)3 (10 mol%) as a catalyst.
|
1 |
Prenyl alcohol, 2a |
3aa, 18 |
4aa, 67 |
2 |
Prenyl alcohol, 2ab |
— |
4aa, 48 |
3 |
Geraniol, 2a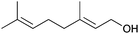 |
3ab, 22 |
4ab, 70 |
4 |
Cinnamyl alcohal, 2c |
3ac, 60 |
— |
The reaction of resveratrol with geraniol mirrored that of the prenol: a 3
:
1 mixture of products 4ab and 3ab was produced in a high overall yield of 92% (entry 3). The two compounds were readily separated by chromatography. The more abundant isomer 4ab is a bioactive macatrichocarpin F isolated from Macaranga plants.30 This compound previously was synthesised through a sequence involving O-alkylation followed by the O- to C-isomerisation.31,32 The allylation with cinnamyl alcohol produced an unknown symmetrical isomer 3ac as a single product in a 60% yield (entry 4). The reason for such a switch in the regioselectivity is unknown but may relate to the formation of a more stabilised cation intermediate.
Prenylation of flavones, chrysin 1b and luteolin 1c proved to be more challenging (Fig. 2). Despite good overall yields, mixtures of compounds were produced. Thus, the reaction of chrysin with prenol gave a mixture of mono and di-prenylation products, 3ba and 5ba, respectively. The initially formed 6-isomer 3ba likely undergoes the second alkylation to furnish 5ba. Nonetheless, the compounds can be readily separated by chromatography. In contrast, luteolin gave a 3
:
1 mixture of monosubstituted isomers 3ca and 4ca, which had similar chromatographic mobility and were difficult to separate; only isomer 3ca was isolated in pure form. Other isomer 4ca was characterised in the mixture with 3ca.
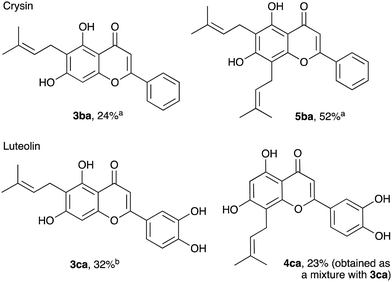 |
| Fig. 2 Allylation of flavones, chrysin 1b, and luteolin 1c. (For conditions, see Table 1, entry 11, yields are given for isolated, analytically pure compounds. a Separated chromatographically from the mixture of 3ba and 5ba. b Separated chromatographically from the mixture of 3ca and 4ca). | |
Flavonols quercetin 1d and myricetin 1e are even more densely substituted with hydroxyl groups and, therefore potentially represented even more challenging targets for the catalytic prenylation. However, they turned out to be good substrates (Scheme 2). Thus, alkylation of quercetin with prenol, geraniol and cinnamyl alcohol occurred uneventfully furnishing good yields of the respective monosubstituted derivatives 3da, 3db and 3dc as single regioisomers. Note that compound 3da is a natural polyphenol gancaonin P found in liquorice.33 The reaction of myricetin with geraniol followed the trend to afford 3ab in a 73% yield. However, the prenylation of myricetin, along with the expected 3ea (24%), showed the formation of dialkylated product 5ea (62%). So far, the attempts at reversing the ratio in favour of monoalkylation have been unsuccessful, but the products are readily separable by simple flash chromatography.
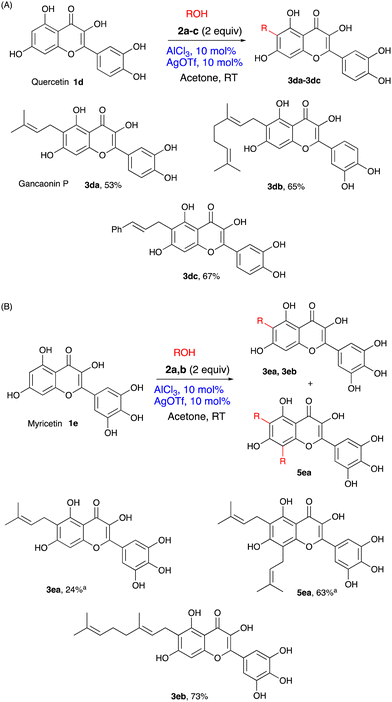 |
| Scheme 2 Allylation of flavonols, (A) quercetin 1d and (B) myricetin 1e. (For conditions, see Table 1, entry 11, yields are given for isolated, analytically pure compounds. a Separated chromatographically from the mixture of 3ea and 5ea). | |
Experimental
General procedure for prenylation of polyphenols
A polyphenol (1 mmol) was added to the solution of AlCl3 (10 mol%) and AgOTf (10 mol%) in acetone (20 mL). The mixture was stirred for 20 min. Then, a solution of prenol or other allylic alcohol (2 mmol) in acetone (4 mL) was added dropwise to this mixture under an inter atmosphere. The resulting solution was stirred at room temperature overnight. The reaction progress was monitored by TLC. The work-up involved dilution with water and extraction with EtOAc. The crude product was purified by chromatography on silica to afford the target compound. For details, see ESI.†
Conclusions
In conclusion, we developed an expedient catalytic method for the introduction of allyl groups, such as prenyl, geranyl and cinnamyl, into the aromatic rings of natural polyphenols under mild reaction conditions. Resveratrol, flavones and flavonols proved to be competent substrates for this reaction furnishing practical yields of the alkylation products, some of the synthesised analogues are occurring in nature. Chemical prenylation can modify the structure of polyphenols, potentially leading to enhanced biological activity and improved pharmacological properties as evidenced by a superior bioactivity of some naturally occurring prenylated analogues compared to the parent polyphenols. Therefore, the developed single-step method may serve as a convenient tool for upgrading the bioactivity of the abundant natural polyphenols.
Author contributions
Conceptualization, A. V. M.; funding acquisition, A. V. M. methodology, Y. D., I. K. and A. V. M. formal analysis, Y. D., I. K. and A. E. R; investigation, Y. D. and I. K.; writing – original draft, A. V. M. and A. E. R.
Conflicts of interest
There are no conflicts to declare.
Acknowledgements
Y. D. and A. V. M. thank H2020 BBI JU for the PHENOLEXA Grant 101023225. A. E. R. thanks Perm Research and Education Centre “Rational Subsoil Use”, 2023.
Notes and references
- N. Shen, T. Wang, Q. Gan, S. Liu, L. Wang and B. Jin, Plant flavonoids: Classification, distribution, biosynthesis, and antioxidant activity, Food Chem., 2022, 383, 132531 CrossRef CAS PubMed.
-
P. Gupta, J. Kour, M. Bakshi and R. Kalsi, in Nutraceuticals and Health Care, ed. J. Kour and G. A. Nayik, Academic Press, 2022, ch. 5, pp. 105–113 Search PubMed.
-
S. Chibisov, E. Kharlitskaya, R. B. Singh, A. Itharat, E. On-Saard, H.-R. Park, J. Chaudhury, S. Chakravorty, O. K. Gupta and M. M. A. Smail, in Functional Foods and Nutraceuticals in Metabolic and Non-Communicable Diseases, ed. R. B. Singh, Elsevier, 2022, ch. 44, pp. 671–689 Search PubMed.
- A. Ullah, S. Munir, S. L. Badshah, N. Khan, L. Ghani, B. G. Poulson, A. H. Emwas and M. Jaremko, Important Flavonoids and Their Role as a Therapeutic Agent, Molecules, 2020, 25, 5243 CrossRef CAS PubMed.
- B. Botta, A. Vitali, P. Menendez, D. Misiti and G. Delle Monache, Prenylated Flavonoids: Pharmacology and Biotechnology, Curr. Med. Chem., 2005, 12, 713–739 CrossRef CAS PubMed.
- S. Shi, J. Li, X. Zhao, Q. Liu and S.-J. Song, A comprehensive review: Biological activity, modification and synthetic methodologies of prenylated flavonoids, Phytochemistry, 2021, 191, 112895 CrossRef CAS PubMed.
- L. M. Dominika, J. Stefano, D. Karel and S. Dallacqua, C-prenylated flavonoids with potential cytotoxic activity against solid tumor cell lines, Phytochem. Rev., 2019, 18, 1051–1100 CrossRef.
- Y. Zhang, X. Zhang, Z. Xiao, X. Zhang and H. Sun, Hypoglycemic and hypolipidemic dual activities of extracts and flavonoids from Desmodium caudatum and an efficient synthesis of the most potent 8-prenylquercetin, Fitoterapia, 2022, 156, 105083 CrossRef CAS PubMed.
- W. Li, L. Shu, K. Liu and Q. Wang, Regioselective synthesis of C-prenylated flavonoids via intramolecular [1,3] or [1,5] shift reaction catalyzed by acidic clays, Tetrahedron Lett., 2019, 60, 151138 CrossRef CAS.
- T. Kawamura, M. Hayashi, R. Mukai, J. Terao and H. Nemoto, An Efficient Method for C8-Prenylation of Flavonols and Flavanones, Synthesis, 2012, 1308–1314 CAS.
- H. Sun, Y. Li, X. Zhang, Y. Lei, W. Ding, X. Zhao, H. Wang, X. Song, Q. Yao, Y. Zhang, Y. Ma, R. Wang, T. Zhu and P. Yu, Synthesis, α-glucosidase inhibitory and molecular docking studies of prenylated and geranylated flavones, isoflavones and chalcones, Bioorg. Med. Chem. Lett., 2015, 25, 4567–4571 CrossRef CAS PubMed.
- S. Tischer and P. Metz, Selective C-6 prenylation of flavonoids via europium(III)-catalyzed Claisen rearrangement and cross-metathesis, Adv. Synth. Catal., 2007, 349, 147–151 CrossRef CAS.
- M. Hurtová, D. Biedermann, Z. Osifová, J. Cvačka, K. Valentová and V. Křen, Preparation of Synthetic and Natural Derivatives of Flavonoids Using Suzuki–Miyaura Cross-Coupling Reaction, Molecules, 2022, 27, 967 CrossRef PubMed.
- G. Kwesiga, J. Greese, A. Kelling, E. Sperlich and B. Schmidt, The Suzuki–Miyaura Cross-Coupling–Claisen Rearrangement–Cross-Metathesis Approach to Prenylated Isoflavones, J. Org. Chem., 2023, 88, 1649–1664 CrossRef CAS PubMed.
- X. Yang, J. Yang, Y. Jiang, H. Yang, Z. Yun, W. Rong and B. Yang, Regiospecific synthesis of prenylated flavonoids by a prenyltransferase cloned from Fusarium oxysporum, Sci. Rep., 2016, 6, 24819 CrossRef CAS PubMed.
- T. Kumano, S. B. Richard, J. P. Noel, M. Nishiyama and T. Kuzuyama, Chemoenzymatic syntheses of prenylated aromatic small molecules using Streptomyces prenyltransferases with relaxed substrate specificities, Bioorg. Med. Chem., 2008, 16, 8117–8126 CrossRef CAS PubMed.
- S. Dutta, T. Bhattacharya, D. B. Werz and D. Maiti, Transition-metal-catalyzed C-H allylation reactions, Chemistry, 2021, 7, 555–605 CrossRef CAS.
- R. Ortiz and R. P. Herrera, Molecules, 2017, 22, 574 CrossRef.
- M. Rueping and B. J. Nachtsheim, Beilstein J. Org. Chem., 2010, 6(No. 6) DOI:10.3762/bjoc.6.6.
- W. Bonrath, C. Dittel, L. Giraudi, T. Netscher and T. Pabst, Rare earth triflate catalysts in the synthesis of Vitamin E and its derivatives, Catal. Today, 2007, 121, 65–70 CrossRef CAS.
- A. V. Malkov, P. Spoor, V. Vinader and P. Kočovský, Molybdenum(IV) Complexes as Efficient, Lewis Acidic Catalysts for Allylic Substitution. Formation of C−C and C−N Bonds, J. Org. Chem., 1999, 64, 5308–5311 CrossRef CAS PubMed.
- N. G. Jentsch, X. Zhang and J. Magolan, Efficient synthesis of cannabigerol, grifolin, and piperogalin via alumina-promoted allylation, J. Nat. Prod., 2020, 83, 2587–2591 CrossRef CAS PubMed.
- J. Zhang, W. Xiong, Y. Wen, X. Fu, X. Lu, G. Zhang and C. Wang, Magnesium dicarboxylates promote the prenylation of phenolics that is extended to the total synthesis of icaritin, Org. Biomol. Chem., 2022, 20, 1117–1124 RSC.
- Y. W. Tang, C. J. Shi, H. L. Yang, P. Cai, Q. H. Liu, X. L. Yang, L. Y. Kong and X. B. Wang, Synthesis and evaluation of isoprenylation-resveratrol dimer derivatives against Alzheimer's disease, Eur. J. Med. Chem., 2019, 163, 307–319 CrossRef CAS PubMed.
- M. Osorio, M. Carvajal, A. Vergara, E. Butassi, S. Zacchino, C. Mascayano, M. Montoya, S. Mejías, M. C. S. Martín and Y. Vásquez-Martínez, Prenylated flavonoids with potential antimicrobial activity: Synthesis, biological activity, and in silico study, Int. J. Mol. Sci., 2021, 22, 5472 CrossRef CAS PubMed.
- B. H. Park, H. J. Lee and Y. R. Lee, Total synthesis of chiricanine A, arahypin-1, trans -arachidin-2, trans -arachidin-3, and arahypin-5 from peanut seeds, J. Nat. Prod., 2011, 74, 644–649 CrossRef CAS.
- A. M. Hartung, J. A. Beutler, H. A. Navarro, D. F. Wiemer and J. D. Neighbors, Stilbenes as κ-selective, non-nitrogenous opioid receptor antagonists, J. Nat. Prod., 2014, 77, 311–319 CrossRef CAS PubMed.
- D. Prat, A. Wells, J. Hayler, H. Sneddon, C. R. McElroy, S. Abou-Shehada and P. J. Dunn, CHEM21 selection guide of classical- and less classical-solvents, Green Chem., 2015, 18, 288–296 RSC.
- F. P. Byrne, S. Jin, G. Paggiola, T. H. M. Petchey, J. H. Clark, T. J. Farmer, A. J. Hunt, C. Robert McElroy and J. Sherwood, Tools and techniques for solvent selection: green solvent selection guides, Sustain, Chem. Process., 2016, 4, 7 Search PubMed.
- M. Tanjung, L. D. Juliawaty, E. H. Hakim and Y. M. Syah, Flavonoid and stilbene derivatives from Macaranga trichocarpa, Fitoterapia, 2018, 126, 74–77 CrossRef CAS PubMed.
- T. Zhou, Y. Jiang, B. Zeng and B. Yang, The cancer preventive activity and mechanisms of prenylated resveratrol and derivatives, Curr. Res. Toxicol., 2023, 5, 100113 CrossRef PubMed.
- T. Puksasook, S. Kimura, S. Tadtong, J. Jiaranaikulwanitch, J. Pratuangdejkul, W. Kitphati, K. Suwanborirux, N. Saito and V. Nukoolkarn, Semisynthesis and biological evaluation of prenylated resveratrol derivatives as multi-targeted agents for Alzheimer's disease, J. Nat. Med., 2017, 71, 665–682 CrossRef CAS.
- T. Nomura, T. Fukai and T. Akiyama, Chemistry of phenolic compounds of licorice (Glycyrrhiza species) and their estrogenic and cytotoxic activities, Pure Appl. Chem., 2002, 74, 1199–1206 CrossRef CAS.
|
This journal is © The Royal Society of Chemistry and the Centre National de la Recherche Scientifique 2023 |
Click here to see how this site uses Cookies. View our privacy policy here.